Abstract
The mechanism of the toxicity of plant lectins is not clearly understood. The insecticidal activity of plant lectins results from effects on insect metabolism by interfering with gut enzymatic function. Thus, a greater understanding of the mechanisms of plant lectin toxicity in insects is required. This study reports the effects of dietary ingestion of the glucose-mannose binding lectin Concanavaline A (Con A) on bird cherry-oat aphid (Rhopalosiphum padi L.) enzymes involved in protein digestion [aminopeptidase N and cathepsin L (CatL)], sugar (α- and β-glucosidases), and phosphorus (alkaline and acid phosphatase) metabolism. An aphid bioassay test using artificial diets containing Con A is also presented. An increase in the concentration of Con A generally suppressed the activity of glucosidases and phosphatases, and increased the activity of CatL in apterae morphs. Bird cherry-oat aphid performance was affected by the presence of Con A in artificial diets. The lectin added to the liquid diet increased the pre-reproductive period, mortality, and the average time of generation development (T) and decreased fecundity and the intrinsic rate of natural increase (rm). Aphicidal activity of Con A might be linked to its interference in the activity of digestive enzymes.
1. Introduction
Aphids are a serious insect problem in many regions of the world. They feed on phloem sap and infect plants with pathogenic viruses (Guerrieri & Digilio Citation2008). Among the numerous aphid species, the bird cherry-oat aphid, Rhopalosiphum padi L., belongs to the family Aphididae, characterized in part, by phytophagous phloem-feeders with a rapid generation time. R. padi is highly polyphagous, with a large number of hosts from the Poaceae family, including all the major cereals (Blackman & Eastop Citation2000). It has been extensively researched since being discovered to vector Yellow Dwarf Viruses (YDVs) in the 1950s, dramatically increasing its status as an economically important pest (Finlay & Luck Citation2011). Aphid populations are usually controlled with chemical insecticides (Boatman et al. Citation2007), but increasing resistance and their side effects on the environment and non-target organisms including humans has stimulated the research on alternative methods of aphid control. The alternative strategy to synthetic insecticides for aphid control includes the use of natural and biodegradable compounds, such as plant extracts, essential oils, saponins, flavonoids, and lectins (Napoleão et al. Citation2012; Ghodke et al. Citation2013).
Plant lectin-based strategies for the production of insect-resistant transgenic crops are currently receiving significant attention for the control of aphids, as they are one of the best-available toxins and display the widest array of molecular targets (Jaber et al. Citation2010). Toxicity assays with artificial diets containing plant lectins or transgenic plants overexpressing specific lectins have demonstrated the harmful effects of lectins on biological parameters of insects, including larval weight, mortality, feeding inhibition (Sprawka & Goławska Citation2010), metabolism, honeydew excretion, pupation, delay in total developmental duration, adult emergence, and fecundity (Carlini & Grossi-de-Sá Citation2002). The results of this research show that specific plant lectins have entomotoxic properties but do not exhibit the same level of toxicity against different insect species, which depend largely on the insect species and the lectin used. Moreover, despite current interest in the insecticidal properties of lectins, their mode of action is not clearly understood. Previous studies have suggested that lectins have different mechanisms of toxicity (Vasconcelos & Oliveira Citation2004) and that the insecticidal activity of lectins results partly from effects on insect metabolism by interfering with gut enzymatic function (Carlini & Grossi-de-Sá Citation2002; Macedo et al. Citation2007). Most of the digestive enzymes or transport proteins secreted in the midgut of insects or proteins embedded in the epithelial cell membrane contain glycan structures, which are potential targets for plant lectins (Vandenborre et al. Citation2011a). Until recently, it was believed that N-linked glycans in insects were exclusively of the high mannose or paucimannose-type. This motivated great interest, especially in mannose-specific plant lectins, as possible insecticidal or insect-deterring molecules for the development of new pest-management strategies (Michiels et al. Citation2010). However, the sensitivity of different insect species to the insecticidal effects of lectin ingestion is variable and the binding of a particular lectin to the gut of a given insect species does not necessarily imply toxicity. Therefore, if strategies based on the use of transgenic crops that express specific lectins are to be adopted, more information on their precise modes of activity will be required to ensure durability in the field (Jaber et al. Citation2007).
One of the most widely studied and perhaps one the most promising plant lectins for aphid control is jackbean lectin (Concanavalin A, Con A). Con A is a homotetramer: each subunit (26.5 KDa, 235 amino acids, heavily glycated) binds a metallic atom (usually Mn2 + or Ca2+) and its tertiary structure has been elucidated. Con A specifically binds α-D-mannosyl and α-D-glucosyl residues (two hexoses differing only in the alcohol group on carbon 2) at a terminal position of ramified structures from B-glycans (in α-mannose, or hybrid and bi-antennary glycan complexes). It has four binding sites, corresponding to the four subunits (Rüdiger & Gabius Citation2001). Bioassays with Con A have shown the highest toxicity among the tested lectins against several insect species (Carlini & Grossi-de-Sá Citation2002) and have led to the selection of Con A for further studies. Con A is known to be toxic to several aphid species: Acythosiphon pisum (Harris), Macrosiphon albifrons (Essig), Aphis gossypii (Glover), Myzus persicae (Sulzer), Macrosiphum euphorbiae (Thomas), and Aulacorthum solani (Kaltenbach), although the effect of lectin on these species is variable (Sauvion et al. Citation2004; Jaber et al. Citation2010).
To establish whether lectins act by disrupting the digestive capacity of wingless females of R. padi and thus further our understanding of the mechanisms of lectin toxicity in insects, this study reports the effects of dietary ingestion of the model glucose-mannose-binding lectin Con A on R. padi enzymes involved in protein digestion [aminopeptidase N (APN) and cathepsin L (CatL)], sugar (α- and β-glucosidases), and phosphorus (alkaline (AkP) and acid (AcP) phosphatases) metabolism. An aphid bioassay test on artificial diets with Con A is also presented.
2. Material and methods
2.1. Insect culture
The bird cherry-oat aphid was obtained from a stock culture kept at the Siedlce University of Natural Sciences and Humanities, Poland. Wingless females were used in all experiments and were reared on winter wheat (Triticum aestivum L. cv. Tonacja) and maintained in an environment chamber at 21 ± 1°C, 16 h light:8 h dark photoperiod and 70% relative humidity.
2.2. Chemicals
The substrate Z-Phe-Arg-pNA was obtained from Bachem (Saffron Walden, UK). Lectin Con A was purchased from MP Biomedicals (CN.150710). All other dietary components and chemical reagents were obtained from Sigma (Sigma Chemical Co., Poznań, Poland) and were of analytical or best-available grade.
2.3. Aphid artificial diet assays
An artificial diet suitable for the development and reproduction of R. padi was prepared according to Kieckhefer and Derr (Citation1976). Con A was incorporated into the diet at four concentrations of 50, 500, 1000, and 1500 µg·cm−3. Control diets (without Con A) were also included. The diets were sterilized by filtration through 0.45 µm Millipore filters. The prepared diets (500 mm3) were introduced between two layers of Parafilm® (sandwich layers), which were placed on plastic rings (height = 1.5 cm−3, Ø = 3.5 cm−3). Wingless females were placed within rings prepared in this way, on a control diet, securing their basis with blotting cloth and were left to produce nymphs overnight. Adults were then removed and the nymphs were maintained on the diet for a further 24 h. A total of 50 nymphs (10 replicates, five insects per replicate) were used for each treatment for the bioassays. Feeding chambers were kept at 21 ± 1°C, 16 h light:8 h dark photoperiod and 70% relative humidity, and fresh diet sachets (sandwich layers) were provided every three days to avoid fungal and bacterial contamination. Larval development time (pre-reproductive period), daily fecundity, and aphid mortality were monitored daily for 15 days. Population parameters were used to determine the influence of Con A on the growth potential of bird cherry-oat aphids. The average time of generation development (T) and the intrinsic rate of natural increase (rm) were calculated using equations of Wyatt and White (Citation1977):
2.4. Effect of Con A on enzyme activities
The effect of Con A on aphid enzymes was determined using starved adult apterous aphids (60 per gel), placed on an artificial diet.
There were two groups of artificial diet: (1) without lectin (control) and (2) with the tested jackbean lectin at four different concentrations; 50, 500, 1000, and 1500 µg·cm−3 (three replicates for each concentration of lectin and control). After seven days of exposure to the diet, aphids were collected and the entire guts were dissected under the binocular microscope and placed in cold buffers recommended for each enzyme: for α-glucosidase and β-glucosidase, 0.1 M phosphate buffer pH 5.8; for AkP, 0.15 M ammonium buffer pH 10; for AcP, 0.2 M citrate buffer pH 5.0; for APN, 0.1 M Tris–HCl buffer pH 7.0 containing 10 mM Triton X-100; for CatL, Mc Ilvaine'a buffer pH 7.0 and homogenised with a small homogeniser at 0°C. The homogenates were centrifuged at 10,000 g for 15 min and the supernatants used to assay the activity of the enzymes studied.
2.5. Enzymatic assays and protein determination
The activity of α- and β-glucosidases was determined using the Katagiri (Citation1979) method, modified by Chararas and Chipoulet (Citation1982). Activities of α- and β-glucosidases were measured with p-nitrophenyl-α-D-glucopyranoside (pNαG) or p-nitrophenyl-β-D-glucopyranoside (pNβG) as substrates, respectively. The amount of p-nitrophenol formation from substrates was determined in a mixture consisting of gut homogenates in 0.1 M phosphate buffer (pH 5.8) and pNαG (15 mM in 0.1 M phosphate buffer pH 5.8) or pNβG (50 mM in 0.1 M phosphate buffer pH 5.8) in a total volume of 0.5 cm3. The mixture was incubated for 60 min at 30°C and the reaction was stopped by the addition of sodium carbonate (2%). Optical density was measured at 405 nm after 10 min. Controls without enzyme were examined. A standard curve of absorbance against the amount of p-nitrophenol released was constructed, to calculate the amount of p-nitrophenol released during the α- and β-glucosidases assays. Activity of α- and β-glucosidases was expressed as µmol p-nitrophenol released min−1 mg−1 protein.
The activity of AkP and AcP was determined using the Neumann and van Vreedendaal (Citation1967) and Deloach and Mayer (Citation1979) methods, based on the amount of p-nitrophenol released from the synthetic substrate p-nitrophenylphosphate (pNPP). The mixture for determining the activity of AcP, which contained gut homogenates, was 0.2 M citrate buffer (pH 5.0) in which the following were dissolved: 5 mM MgCl2, 3 mM p-nitrophenylphosphate, and 0.1% TritonX-100, and was incubated for 30 min at 30°C and the reaction was stopped by the addition of KOH (6M).
The mixture for determining the activity of AkP contained p-nitrophenylphosphate (0.1%) and ammonium buffer (0.15 M pH 10) was incubated for 15 min at 30°C. After adding gut homogenates, the mixture was incubated for a further 30 min and the reaction stopped by the addition of NaOH (1 M) and made to a total volume of 2 cm3 with water. Controls without enzyme were also included. Optical density was measured at 405 nm. The activity of AkP and AcP was expressed as µmol p-nitrophenol released min−1 mg−1 protein.
The activity of aminopeptidse N activity and CatL was determined spectrophotometrically based on the amount p-nitroaniline liberated from L-leucine-p-nitroanilide (L-Leu-p-NA) and from benzoyloxycarbonyl-L-phenylalanyl-L-arginine-p-nitroanilide (Z-Phe-Arg-pNA) as substrates, respectively.
APN was determined by the method of Erlanger et al. (Citation1961), modified by Baker and Woo (Citation1981). The incubation solution contained gut homogenates, a substrate of 1 mM concentration (initially solubilised in dimethylformamide, DMF) in 100 mM Tris–HCl buffer in which 5 mM MgCl2·6H2O was dissolved. After incubation at 30°C for 30 min, the reaction was stopped by the addition of cold 10% trichloracetic (TCA).
The activity of CatL was assayed according to Wagner et al. (Citation2002). Gut homogenates were pre-incubated in a buffer containing 5 mM DTT (dithiothreitol), 1 mM EDTA (disodium ethylenediaminetetraacetate dihydrate), and 0.1% Brij 35 for 15 min at 25°C. The reaction was initiated by adding the substrate (10 mM substrate solution in DMF). The reaction mixture was incubated for 30 min at 37°C. The reaction was stopped by the addition of cold 10% TCA. Controls without enzyme or without substrate were included. The absorbance was measured at 410 nm. The concentration of p-nitroaniline was calculated using an extinction coefficient 8800 M−1 cm−1. The activity of APN and CatL was expressed as nmol p-nitroaniline released per minute per milligram protein. The determination of enzyme activities was performed in three replicates.
The protein concentration in the gut homogenates was determined using the method of Bradford (Citation1976).
2.6. Statistical analysis
The effect of the different Con A concentrations on bird cherry-oat aphid performance and the influence of Con A on enzyme activity were subjected to a two-tailed unpaired Student's t-test. Relationships between the concentration of Con A and population parameters and enzyme activities were calculated using the Pearson correlation. We used Statistica for Windows v.7.0 (Statsoft Citation2003) for all statistical analyses.
3. Results
3.1. Rhopalosiphum padi performance on artificial diets with Con A
Bird cherry-oat aphid performance was affected by the presence of Con A in artificial diets. The lectin added to the liquid diet increased the pre-reproductive period, mortality, the average time of generation development (T), and decreased fecundity, and the intrinsic rate of natural increase (rm) ().
Table 1. Values of population parameters (means ± SD), for bird cherry-oat aphid on the diets with Con A.
Moreover, there were significant differences for all tested population parameters of R. padi between the control and tested concentrations of Con A (P < 0.001, P < 0.01, P < 0.05, t test). Only for the 50 µg cm−3 concentration of Con A significant differences were not found (P > 0.05, t test) (). Highly significant differences were also found for mortality. Bird cherry-oat aphid showed a significant mortality in comparison to control insects (P < 0.001, P < 0.01 Student's t-test) () at all concentrations of phytohaemagglutinin (PHA) tested.
Table 2. Toxicity of Con A on R. padi.
A significant relationship was observed between different concentrations of Con A and population parameters: aphid fecundity (R = −0.92, P < 0.001, Pearson correlation), larval development (R = 0.90, P < 0.001, Pearson correlation), average time of generation development (R = 0.90, P < 0.001, Pearson correlation), intrinsic rates of natural increase (R = −0.95, P < 0.001, Pearson correlation), and mortality (R = 0.95, P < 0.001, Pearson correlation) ().
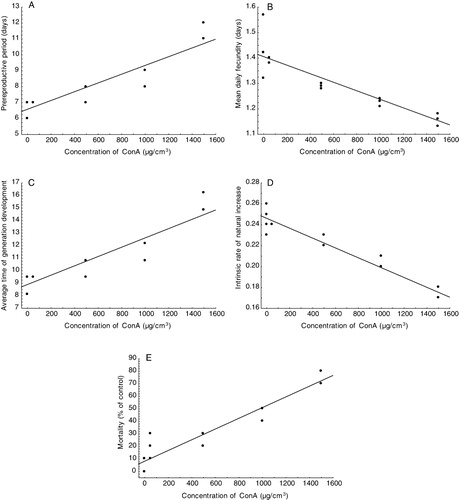
3.2. Effect of Con A on digestive enzyme activity
The activity of α-glucosidase was significantly (P < 0.01; Student's t-test, ) reduced by Con A, with the greatest inhibition at 24%. There was a highly significant and negative correlation between the increase in the concentration of Con A and the decrease in activity of α-glucosidase (R = −0.99, P < 0.001, Pearson correlation). In contrast to α-glucosidase, the effect on β-glucosidase was not as clear; it was significantly induced (P < 0.01; Student's t-test, ) by the lower concentrations of Con A, but inhibited by higher concentrations (). There was no significant correlation between β-glucosidase activity in the control and at all tested concentrations of Con A (R = 0.73, P > 0.05, Pearson correlation). The same was observed for AkP. The lowest concentrations of Con A caused an increase in AkP activity, but higher concentrations induced a decrease in activity (). There was no significant correlation for the activity of AkP between the control and all tested concentrations of Con A (R = −0.21, P > 0.05, Pearson correlations). Treatment with Con A caused a significant reduction (approximately 30%) in AcP activity (P < 0.05; P < 0.01, t test, ). There was a highly significant and negative correlation between an increase in the concentration of Con A and the decrease in AcP activity (R = −0.97, P < 0.001, Pearson correlations). Although the presence of Con A caused a significant increase in CatL activity in apterae morphs (approx. 90%), it did not affect the activity of a second proteinase, APN (). There was a significant correlation between the increase in the concentration of Con A and the activity of CatL (R = 0.82, P < 0.01, Pearson correlation), however, there was no significant correlation in the activity of APN between the control and all tested concentrations of Con A (R = −0.46, P > 0.05, Pearson correlation).
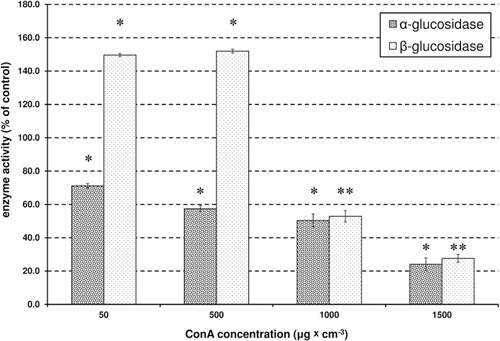
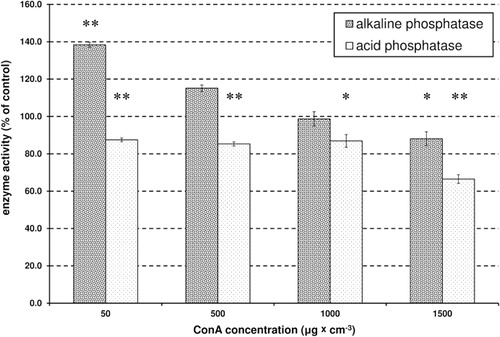
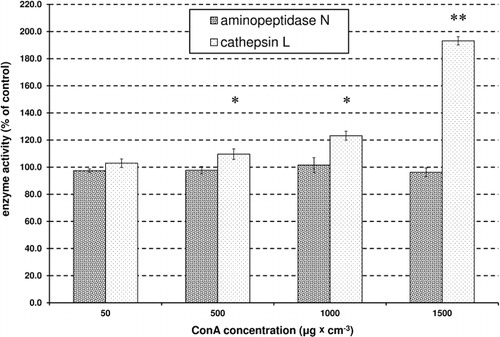
4. Discussion
The insecticidal properties of plant lectins have been identified for several lectin–insect interactions but are not well understood. As insects in normal circumstances take up plant lectins through feeding, the midgut is a critical site affected by these compounds. Therefore, it is necessary to explain the mode of action of lectins at the level of target tissues (the digestive tract). Since glycoproteins are major constituents of the insect digestive tract membranes, it is plausible that the insect gut contains specific ligand-binding molecules that are plant lectin targets (Macedo et al. Citation2007; Vanderborre et al. Citation2010, Citation2011b). Digestive enzymes belong to this class of targets (Carlini & Grossi-de-Sá Citation2002). Previous studies have shown that the interaction between lectins and digestive enzymes might destabilize the insect metabolism (Singh et al. Citation2006). Lectins may affect the enzyme activities binding to these at place other than their substrate binding site. Lectins may also increase the number of enzyme active sites by altering their accessibility to the substrate. Moreover, lectins could bind to the substrates, increasing the enzyme activity, binding to both enzymes and their substrates and may increase the affinity among them. However, the antinutrient activity of plant lectins is primarily explained by the predominant binding of lectins to glycan receptors at the intestinal surface and blocking them from enzyme (Bandyopadhyay et al. Citation2001).
Moreover, immunohistochemical and electron microscopy studies revealed that Con A binds to the midgut and causes morphological changes in the epithelial cells (Sauvion et al. Citation2004) with pathological consequences, such as apoptosis of epithelial cells, which may explain their cytotoxicity (Hamshou et al. Citation2010, Citation2013). In view of this observation, we decided to investigate the effect of Con A on various insect hydrolytic enzymes such as glucosidases, phosphatases, and proteases that are involved in digestion, development, growth, and detoxification. This study showed that lectins might cause changes in the activity of digestive enzymes. The results showed that an increase in the concentration of Con A suppressed the activity of glucosidases and phosphatases. Only the lowest concentrations of Con A (50 and 500 µg·cm−3) induced β-glucosidase and AkP activity. Moreover, the addition of Con A to the artificial diet caused an increase in the activity of CatL, but had no effect on the activity of the second proteinase, APN. This observation is supported by the findings of Cristofoletti et al. (Citation2006), who showed that APN binds Con A, but the kinetics parameters of APN associated with Con A were unaffected. This indicates that the catalytic site and the mannosylated site(s) are far apart within the enzyme molecule. There is little information known concerning lectin interactions with insect enzymes, especially for the influence of Con A on hydrolytic enzymes. Fitches and Gatehouse (Citation1998) observed a decrease in the activities of soluble and brush-border membrane enzymes (α-glucosidase and AkP) in the midgut of Lacanobia oleracea L. when examining larvae chronically exposed to lectins Galanthus nivalis L. and Canavalia ensiformis L. (Con A) for 16 days. Similar results were obtained by Kaur et al. (Citation2006) and Singh et al. (Citation2006, Citation2008) when they treated larvae of the melon fruit fly, Bactrocera cucurbitae (Coquillett) with Arisaema jacquemontii (Blume), Glycine max L., and Arisaema curvatum (Kunth) lectins. Larvae fed on an artificial diet containing lectins showed a significant decrease in AcP and AkP, catalase, and glutathione S-transferase activity, whereas esterase activity markedly increased compared to larvae fed on diets without lectins. Macedo et al. (Citation2007) also showed that Bauhinia monandra (Kurz) leaf lectin influenced the α-amylase activity of Callosobruchus maculates F. in vitro.
Li et al. (Citation2009) using microarrays and quantitative real-time PCRs to determine the transcriptional responses of Drosophila melanogaster (Meigen) third-instar larval midguts to Wheat germ agglutinin feeding, revealed that lectin caused the differential expression of 61 transcripts involved in cytoskeleton organization, digestion, detoxification, or energy metabolism. The differences for digestive enzymes and energy metabolism might also relate to reduced weight and changes in feeding behavior (via feedback mechanisms) (Michiels et al. Citation2010). Additionally, our previous work pointed out negative effects of PHA on various hydrolytic enzymes. We found that exposure of the adult apterae Sitobion avenae F. to PHA had reduced α-glucosidase and phosphatase activity and an increased activity of β-glucosidase. This differential effect of Con A and PHA on enzyme activity might be due to differences in sugar specificities; PHA has specificity for D-galactose/N-acetyl-D-galactosamine (GalNAc) residues (Goldstein & Portez Citation1986), whereas Con A specifically binds α-D-mannosyl and α-D-glucosyl residues (Rüdiger & Gabius Citation2001).
It is well established that α-glucosidase catalyses both the production of monosaccharides and the synthesis of glucose-dominated oligosaccharides by using glucose and therefore plays a central role in carbon nutrition and osmoregulation (sucrase and transglucosidase activity) in aphids (Price et al. Citation2007). β-glucosidase is an important enzyme for insect metabolism and for insect–plant interactions, in addition to hydrolysing several carbohydrates, thus liberating monosaccharides that can be absorbed (Terra et al. Citation1996). Phosphorylation is one of the metabolic pathways for ecdysteroids inactivation, which controls the reproduction and/or embryonic development in various insects (Rees Citation1995). Because a one a ways of ecdysteroid mobilization is the hydrolysis of ecdysteroid conjugates (Němec & Ženka Citation1996), the reduction in activity of phosphatases might interfere with aphid embryogenesis, which in turn, affects aphid population development and survival. Therefore, it is possible that the reduction in activity of these enzymes might cause starvation, which affects insect survival, development, and fecundity, leading to insect death within a few days. Artificial diet-feeding assays have demonstrated that Con A is toxic to the bird cherry-oat aphid. Particularly, Con A has an inhibitory effect on fecundity (an important parameter when trying to limit the growth of the insect population) and induced an increase in the pre-reproductive period of R. padi. These results were reflected in population parameter values, which showed that the rate of population increase was negatively correlated with the concentration of Con A in diets, but an increase in the mean generation time was positively correlated with the increased concentrations of Con A. Moreover, laboratory tests showed that Con A has an inhibitory effect on aphid development, which is enough to greatly affect insect survival. The mannose/glucose-specific lectin Con A has been used a lot in feeding experiments on pest herbivores. When fed to larvae of tomato moth, Lacanobia oleracea in artificial diet or on Con A-expressing potato plants, larval development was retarded, 90% mortality was scored and decrease in larval weigh also showed (Gatehouse et al. Citation1999). Toxicity of Con A to Meligethes aeneus F. was also reported by Melander et al. (Citation2003). Similarly, when Con A was added to artificial diets and fed to tara planthopper, Tarohpagous proserpina a corrected mortality of 93% was noted (Powell Citation2001). Moreover, artificial diet studies have shown that this lectin has insecticidal activity, particularly toward Homoptera including the rice brown planthopper, Nilaparvata lugens (Powell et al. Citation1993), and the pea aphid Acyrthosiphon pisum (Rahbe & Febvay Citation1993; Rahbe et al. Citation1995). The effects of Con A on the peach-potato aphid, Myzus persicae also show that the lectin has deleterious effects on growth and development in this insect when administrated in artificial diet, resulting in decreased fecundity. In agreement with the diet bioassay results, Con A-expressing potatoes decreased the fecundity of M. persicae by up to 45% (Sauvion et al. Citation1996; Gatehouse et al. Citation1999).
Moreover, it should be noted that Con A toxicity to bird cherry-oat aphid might not be related to the inhibition of APN activity. Recently, Cristofoletti et al. (Citation2006) suggested that despite Con A binding to APN, enzyme activity was unaffected. It is postulated that APN is somehow associated with putative amino acid binding sites on the plasma membrane. Consequently, this toxicity must result from a decrease in amino acid absorption with deleterious effects on the putative associated proteins thought to bind to amino acids. Accordingly, several lectin interactions with digestive enzymes might be part of the antinutritive mechanism.
Moreover, treatment with Con A caused an increase in the level of CatL activity. In aphids, CatL is possibly involved in the degradation of toxic proteins in the phloem sap and in the processing of exogenous ingested polypeptides (Deraison et al. Citation2004). The results suggest that this enzyme is involved in the metabolism of Con A.
In conclusion, the present results support the hypothesis, that insecticidal activity of plant lectins on insects may involve effects on enzymes in the gut. Con A had dose-dependent detrimental effects on feeding and development in the bird cherry-oat aphid when ingested via an artificial diet, through an identified mode of action. This is due to inhibitory effects of Con A on aphid digestive enzymes, resulting in a suppression of feeding (leading to starvation) and the associated effects of reduced fecundity and survival. However, because the mechanism of the lectin effect on digestive enzyme activity is unclear, further investigation into lectin interactions with enzymes at the biochemical (glycosylation patterns in insects, detailed lectin-insect enzymes interactions) or genomic level is necessary, which should be investigated individually. Technologies, such as microarray analysis and qRT-PCR, and advances in protein interaction analysis provide new opportunities that will enable researchers to investigate lectin–molecule enzyme interactions in more detail.
References
- Baker JE, Woo SM. 1981. Properties and specificities of a digestive aminopeptidase from larvae of Attagenus megatoma (Coleoptera: dermestidae). Comp Biochem Physiol B. 69:189–193. doi:10.1016/0305-0491(81)90229-7
- Bandyopadhyay S, Roy A, Das S. 2001. Binding of garlic (Allium sativum) leaf lectin to the gut receptors of homopteran pests is correlated to its insecticidal activity. Plant Sci. 161:1025–1033. doi:10.1016/S0168-9452(01)00507-6
- Blackman R, Eastop V. 2000. Aphids on the world's crops an identification and information guide. 2nd ed. Chichester: John Wiley & Sons.
- Boatman ND, Parry HR, Bishop JD, Cuthbertson AGS. 2007. Impacts of agricultural change for farmland biodiversity. In: Hester R, Harrison RM, editors. Biodiversity under threat. Issues in environmental science and technology. Vol. 25. London (UK): Royal Society of Chemistry Publishing; p. 1–32.
- Bradford MM. 1976. A rapid and sensitive method for the quantitation of microgram quantities of protein utilizing the principle of protein-dye binding. Anal Biochem. 72:258–254. doi:10.1016/0003-2697(76)90527-3
- Carlini CR, Grossi-de-sá MF. 2002. Plant toxic proteins with insecticidal properties. A review on their potentialities as bioinsecticides. Toxicon. 40:1515–1539. doi:10.1016/S0041-0101(02)00240-4
- Chararas C, Chipoulet J.-M. 1982. Purification by chromatography and properties of β-glucosidase from the larvae of Phoracantha semipunctata. Comp Biochem Physiol B. 72:559–564. doi:10.1016/0300-9629(82)90122-0
- Cristofoletti PT, Mendonca de sousa FA, Rahbe Y, Terra WR. 2006. Characterization of membrane-bound aminopeptidase purified from Acyrthosiphon pisum midgut cells. FEBS J. 273:5574–5588. doi:10.1111/j.1742-4658.2006.05547.x
- Deloach JR, Mayer RT. 1979. The pupal instar of Stomoxys calcitrans: developmental changes in acid phosphatase, cytochrome oxidase and lysosomal glycosidases. Insect Biochem. 9:653–659. doi:10.1016/0020-1790(79)90105-7
- Deraison C, Darboux I, Dupotets L, Gorojankina T, Rahbé Y, Juanin L. 2004. Cloning and characterization of a gut-specific cathepsin L from the aphid Aphis gossypii. Insect Mol Biol. 27:397–403.
- Erlanger BF, Kokowsky N, Cohen W. 1961. The preparation and properties of two new chromogenic substances of trypsin. Arch Biochem Biophys. 95: 271–278. doi:10.1016/0003-9861(61)90145-X
- Finlay KJ, Luck JE. 2011. Response of the bird cherry-oat aphid (Rhopalosiphum padi) to climate change in relation to its pest status, vectoring potential and function in a crop–vector–virus pathosystem. Agr Ecosyst Environ. 144:405–421. doi:10.1016/j.agee.2011.08.011
- Fitches E, Gatehouse JA. 1998. A comparison of the short and long term effects of insecticidal lectins on activities of soluble and brush border enzymes of tomato moth larvae (Lacanobia oleracea). J Insect Physiol. 44:1213–1224. doi:10.1016/S0022-1910(98)00090-0
- Gatehouse AMR, Dawidson GM, Stewart JN, Gatehouse LN, Kumar A, Geoghegan IE, Birch ANE, Gatehouse JA. 1999. Concanavalin A inhibits development of tomato moth (Lancanobia oleracea) and peach-potato aphid (Myzus persicae) when expressed in transgenic potato plants. Mol Breeding. 5:153–165. doi:10.1023/A:1009681705481
- Ghodke AB, Chavaan SG, Sonawane BV, Bharose AA. 2013. Isolation and in vitro identification of proteinase inhibitors from soybean seeds inhibiting helicoverpa gut proteases. J Plant Interact. 8:170–178. doi:10.1080/17429145.2012.668952
- Goldstein IJ, Portez RD. 1986. Isolation, physicochemical characterization, and carbohydrate-binding specificity of lectins. In: Leiner IE, Sharon N, Goldstein IJ, editors. The Lectins, properties, functions and applications in biology and medicine. New York: Academic Press; p. 234–260.
- Guerrieri E, Digilio MC. 2008. Aphid-plant interactions: a review. J Plant Interact. 3:223–232. doi:10.1080/17429140802567173
- Hamshou M. 2013. High entomotoxicity and mechanism of the fungal GalNAc/Gal-specific Rhizocotonia solani lectin in pest insects. J Insect Physiol. 59:295–305. doi:10.1016/j.jinsphys.2012.12.003
- Hamshou M, Smagghe G, Shahidi-Noghabi S, De Geyter E, Lannoo N, Van Damme EJM. 2010. Insecticidal properties of Sclerotinia sclerotiorum agglutinin and its interaction with insect tissues and cells. Insect Biochem Mol Biol. 40:883–890. doi:10.1016/j.ibmb.2010.08.008
- Jaber K, Francis F, Paquereau L, Fournier D, Haubruge E. 2007. Effect of a fungal lectin from Xerocomus chrysenteron (XCL) on the biological parameters of aphids. Commun Agric Appl Biol Sci. 72:629–638.
- Jaber K, Haubruge É, Francis F. 2010. Development of entomotoxic molecules as control agents: illustration of some protein potential uses and limits of lectins (Review). Biotechnol Agron Soc Environ. 14:225–241.
- Katagiri C. 1979. α-D-glucosidase in the serum of American cockroach Periplaneta Americana. Insect Biochem. 9:199–204. doi:10.1016/0020-1790(79)90051-9
- Kaur M, Singh K, Rup PJ, Kamboj SS, Saxena AK, Sharma M, Bhagat M, Sood SK, Singh J. 2006. A tuber lectin from Arisaema helleborifolium schott with anti-insect and anti-proliferative properties. J Biochem Mol Biol. 4:432–440. doi:10.5483/BMBRep.2006.39.4.432
- Kieckhefer RW, Derr RF. 1976. Rearing three species of cereal aphids on artifical diets. J Econ Entomol. 60:663–665.
- Li H-M, Sun L, Mittapalli O, Muir WM, Xie J, Wu J, Schemerhorn BJ, Sun W, Pittendrigh BR, Murdock LL. 2009. Transcriptional signatures in response to wheat germ agglutinin and starvation in Drosophila melanogaster larval midgut. Insect Mol Biol. 18:21–31. doi:10.1111/j.1365-2583.2008.00844.x
- Macedo MLR, Freire MGM, Silva MBR, Coelho LCBB. 2007. Insecticidal action of Bauhinia monandra leaf lectin (BmoLL) against Anagasta kuehniella (Lepidoptera: Pyralidae), Zabrotes subfasciatus and Callosobruchus maculates (Coleoptera: Bruchidae). Comp Biochem Physiol A. 146:486–498.
- Melander M, Ahman I, Kamnert I, Strömdahl A-C. 2003. Pea lectin expressed transgenically in oilseed rape reduces growth rate of pollen beetle larvae. Transgenic Res. 12:555–567. doi:10.1023/A:1025813526283
- Michiels K, Van Damme EJM, Smagghe G. 2010. Plant-insect interactions: what can we learn from plant lectins? Arch Insect Biochem. 73:193–221. doi:10.1002/arch.20351
- Napoleão TH, Pontual EV, De Albuquerque Lima T, De Lima Santos ND, Sá RA, Coelho L CBB, Navarro DMAF, Paiva, PMG. 2012. Effect of Myracrodruon urundeuva leaf lectin on survival and digestive enzymes of Aedes aegypti larvae. Parasitol Res. 110:609–616. doi:10.1007/s00436-011-2529-7
- Němec V, Zenka J. 1996. Activity of phosphatases and esterases in the aphid, Acyrthosiphon pisum (Hemiptera: Sternorrhyncha: Aphididae), and in the gut wall of Galleria mellonella (Lepidoptera: Pyralidae) larvae and pupae. Eur J Entomol. 93:37–44.
- Neumann H, Van Vreedendaal M. 1967. An improved alkaline phosphatase determination with p-nitrophenyl phosphate. Clin Chimi Acta. 17:183–187. doi:10.1016/0009-8981(67)90117-9
- Powell KS. 2001. Antimetabolic effects of plants lectins towards nymphal stages of the planthoppers Tarophagous proserpina and Nilaparvata lugens. Entomol Exp Appl. 99:71–77. doi:10.1046/j.1570-7458.2001.00803.x
- Powell KS, Gatehouse AMR, Hilder VA, Gatehouse JA. 1993. Antimetabolic effects of plants lectins and plant and fungal enzymes on the nymphal stages of two important rice pests, Nilaparvata lugens and Nephotettix cinciteps. Entomol Exp Appl. 66:119–126. doi:10.1111/j.1570-7458.1993.tb00699.x
- Price DRG, Karley AJ, Ashford DA, Isaacs HV, Pownall ME, Wilkinson HS, Gatehouse JA, Douglas AE. 2007. Molecular characterisation of candidate gut sucrase in the pea aphid Acyrthosiphon pisum. J Biochem Mol Biol. 37:307–317.
- Rahbe Y, Febvay G. 1993. Protein toxicity to aphids: an in vitro test on Acyrthosiphon pisum. Entomol Exp Appl. 67:149–160. doi:10.1111/j.1570-7458.1993.tb01663.x
- Rahbe Y, Sauvion N, Febvay G, Peumans WJ, Gatehouse AMR. 1995. Toxicity of lectins and processing of ingested proteins in the pea aphid Acyrthosiphon pisum. Entomol Exp Appl. 76:143–155. doi:10.1111/j.1570-7458.1995.tb01956.x
- Rees HH. 1995. Ecdysteroid biosynthesis and inactivation in relation to function. Eur J Entomol. 92:9–39.
- Rüdiger H, Gabius H-J. 2001. Plant lectins: occurrence, biochemistry, functions and applications. Glycoconjugate J. 18:598–613.
- Sauvion N, Nardon G, Febvay G, Gatehouse AMR, Rahbeé Y. 2004. Binding of the insecticidal lectin Concanavalin A in pea aphid, Acyrthosiphon pisum (Harris) and induced effects on the structure of midgut epithelial cells. J Insect Physiol. 50:1137–1150. doi:10.1016/j.jinsphys.2004.10.006
- Sauvion N, Rahbe Y, Peumans WJ, Van Damme EJM, Gatehouse JA, Gatehouse AMR. 1996. Effects of GNA and other mannose binding lectins on development and fecundity of the peach-potato aphid Myzus persicae. Entomol Exp Appl. 79:285–293. doi:10.1111/j.1570-7458.1996.tb00836.x
- Singh K, Kaur M, Rup MK, Singh J. 2006. Exporation for anti-insect properties of lectin from seeds of soyabean, Glycine max L. using Bactrocera cucurbitae (Coquillett) as model. Phytoparasitica. 34:463–473 doi:10.1007/BF02981200
- Singh K, Kaur M, Rup MK, Singh J. 2008. Effects of plant lectin from cobra lily, Arisaema Curvatum Kunth on development of melon fruitly, Bactrocera cucurbitae (Coq.). J Environ Biol. 29:911–916.
- Sprawka I, Goławska S. 2010. Effect of the lectin PHA on the feeding behavior of the grain aphid. J Pest Sci. 83:149–155. doi:10.1007/s10340-009-0281-x
- StatSoft Inc. 2003. Statistica (Data Analysis Software System). Version 07. [cited 2012 June 15]. Available from: www.statsoft.com.
- Terra WR, Ferreira C, Jordao BP, Dillon RJ. 1996. Digestive enzymes. In: Lehane MJ, Billingsley PF, editors. Biology of the insect midgut. London: Chapman & Hall; p. 153–193.
- Vanderborre G, Van Damme EJM, Ghesquière B, Menschaert G, Hamshou M, Rao RN, Gevaert K, Smagghe G. 2010. Glycosylation signatures in drosophila: fishing with lectins. J Proteome Res. 9:3235–3242. doi:10.1021/pr1001753
- Vandenborre G, Smagghe G, Van Damme EJM. 2011a. Plant lectins as defense protein against phytophaguos insect. Phytochemistry. 72:1538–1550. doi:10.1016/j.phytochem.2011.02.024
- Vanderborre G, Smagghe G, Ghesquière B, Menschaert G, Rao RN, Gevaert K, Van Damme EJM. 2011b. Diversity in protein glycosylation among insect species. PLoS One. 6:e16682. doi:10.1371/journal.pone.0016682
- Vasconcelos IM, Oliveira JTA. 2004. Antinutritional properties of plant lectins. Toxicon. 44:1737–1747. doi:10.1016/j.toxicon.2004.05.005
- Wagner W, Mohrlen F, Schnetter W. 2002. Characterization of the proteolytic enzymes in the midgut of the European Cockchafer, Melontha melontha (Coleoptera: Scarabaeidae). J Biochem Mol Biol. 32:803–814.
- Wyatt I, White PF. 1977. Simple estimation of intrinsic increase rates for aphids and tetranychid mites. J Appl Ecol. 14: 757–776. doi:10.2307/2402807