Abstract
Pathogenesis-related (PR) proteins are induced in response to pathogen attack. In the present study, the induction of PR proteins in response to the fungal pathogen Macrophomina phaseolina was investigated in 15-day- and 1-month-old plants of Vigna aconitifolia with resistant and susceptible cultivars. Inoculation of the fungal pathogen resulted in the enzyme activity gradually increased throughout the experimental period of 168 h compared to control. However, the activation of β-1,3-glucanase and chitinase was more rapid and to a greater extent in the resistant FMM-96 cultivar as compared to susceptible RM0-40 and CZM-3 cultivars. Furthermore, the western blot analysis revealed the presence of 33- and 30-kDa bands of β-1,3-glucanase and chitinase in induced moth bean plants, respectively. The possible implications of these findings as part of the general defense response of moth bean plants against the fungal pathogen (M. phaseolina) have been discussed.
1. Introduction
Plants provide long-lasting systemic immunity to secondary infection by a range of biotrophic and necrotrophic pathogens (Grant & Lamb Citation2006) through the production of antimicrobial secondary metabolic compounds known as phytoalexins and by the activation and synthesis of defense-related proteins (Castro & Fontes Citation2005). Antoniw et al. (Citation1980) coined the term ‘pathogenesis-related (PR) proteins (PRs),’ which have been defined as ‘proteins encoded by the host plant but induced only in pathological or related situations.’ They are low-molecular weight proteins (6–43 kDa), selectively extractable and stable at low pH 3 and thermostable (Van Loon Citation1999). Two well-known examples of PR proteins are β-1,3-glucanase (EC 3.2.1.39) and chitinase (EC 3.2.1.14). β-1,3-glucanase also known as laminarinase enhances fungal resistance in crop plants (Kirubakaran & Sakthivel Citation2007). These endoglucanases catalyze the hydrolytic cleavage of the (1,3)-β-D-glucosidic linkages in (1,3)-β-glucans and act primarily on glucans present in the fungal cell wall. The main substrate of chitinase is chitin, a natural homopolymer of β-1,4-linked N-acetyl-D-glucosamine (NAG) residues (Kasprzewska Citation2003).
Moth bean [V. aconitifolia (Jacq.) Marechal] (family Leguminoceae) (Verdcourt Citation1970) is an economically important kharif-season crop grown in arid and semiarid regions particularly in northwestern states of India such as Rajasthan. It is a major commercial crop of India, and its contribution in area and production is 5.9 and 1.6%, respectively (Kumar Citation2002). The plant fixes atmospheric nitrogen into the soil, and it is known for its higher protein content and good amino acid balance.
Macrophomina phaseolina (Tassi) Goid is one of the most damaging seed and soil-borne fungal pathogen of moth bean-growing areas in India (Singh & Srivastava Citation1988). M. phaseolina has a wide host range and is responsible for causing losses on more than 500 cultivated and wild plant species (Mihail & Taylor Citation1995).
In our earlier work, we have demonstrated that PR proteins (β-1,3-glucanase and chitinase) activity increased in Eruca sativa after the fungal pathogen (Alternaria brassicicola) inoculation (Gupta et al. Citation2012). In Sorghum bicolor too, there was higher expression of chitinase gene after the pathogen (M. phaseolina) inoculation (Sharma et al. Citation2013).
In the present study, induction of PR proteins in response to the fungal pathogen M. phaseolina was investigated in 15-day- and 1-month-old plants of Vigna aconitifolia in different time intervals. The biochemical approach described in this article for the defense mechanism in moth bean provides the basis for further efforts concentrating on the isolation and characterization of elements involved in perception and in the early steps of intracellular signal transduction.
2. Materials and methods
2.1. Plant material and growth conditions
Three different cultivars of moth bean namely, var. FMM-96 (resistant), and RMO-40 and CZM-3 (susceptible) were procured from S.K.N. College of Agriculture, Jobner (Rajasthan, India) and from CAZRI, Jodhpur (Rajasthan, India). Seeds were surface sterilized using 0.1% HgCl2 and grown in pots containing steam-sterilized garden soil in a plant growth chamber with a photoperiod of 14-h light (photosynthetically active radiation, 35 µmol photons·m−2·s−1, provided by cool-white Philips fluorescent tubes) and 10-h dark, 28 ± 2°C temperature and 60% relative humidity. Two age-groups of plants, i.e. 15 days and 1 month, were taken for experiments.
2.1.1. Activation of fungal culture and mode of inoculation
The fungal strain of M. phaseolina (Microbial Type Culture Collection and Gene Bank No. 2165) was obtained from IMTECH, Chandigarh. The lyophilized fungal strain was activated on potato dextrose broth. The flasks were incubated in an incubator shaker (28 ± 2°C) for 120 h at 120 rpm. Activated fungal strain was then streaked on potato dextrose agar slants. Fungal spore suspension was prepared in sterilized water at a concentration of 105 spores/ml under aseptic conditions and kept in the incubator shaker (28 ± 2°C, 120 rpm) for 1 h to obtain a uniformly distributed spore suspension. For inoculation, the leaf and stem surfaces of the plants were injured mildly with abrasive (saw dust paper) to facilitate the entry of spores. The fungal spores on plant surfaces were sprayed with a sprayer. The plants were sprayed with autoclaved distilled water without fungal spores but injured mildly with abrasive served as control.
2.2. Determination of PR proteins
The activities of two PR proteins namely, β-1,3-glucanase and chitinase were determined using the method of Abeles et al. (Citation1970).
2.2.1. Extraction of β-1,3-glucanase and chitinase
One gram leaf and stem portion were taken from control and inoculated moth bean plants of all the three cultivars namely, RMO-40 and CZM-3 (susceptible), and FMM-96 (resistant) at different time intervals (0, 2, 4, 24, 48, 72, 96, 120, 144, and 168 h) of fungal spore inoculation. The tissue was homogenized in a prechilled mortar pestle in 4 ml of 0.05 M of potassium acetate buffer (pH 5) for β-1,3-glucanase and 0.1 M sodium citrate buffer (pH 5) for chitinase. The homogenate of β-1,3-glucanase and chitinase was filtered through two layers of cheese cloth premoistened in 0.05 M of potassium acetate buffer (pH 5) and 0.1 M sodium citrate buffer (pH 5), respectively. Then filtrate was centrifuged at 10,000 g in a cooling centrifuge for 10 min at 4°C. The supernatant was collected and used for the assay.
2.2.2. Assay of β-1,3-glucanase
The extract from both control and inoculated plants were taken for the assay. The reaction mixture (1 ml) consisted of 50-µl sample, 450-µl buffer (0.05 M potassium acetate, pH 5), and 500 µl of 2% laminarin as a substrate. The mixture was incubated for 1 h at 40°C. After incubation, the released glucose was assayed using the method of Nelson (Citation1944) and Somogyi (Citation1952). The β-1,3-glucanase activity (µkat·mg−1protein) was calculated using the standard curve of glucose.
In a centrifuge tube, 0.1 ml of reaction mixture was taken and 1.9 ml of double deionized water was added. Then 1 ml of alkaline copper tartrate reagent was added. Mixture was incubated in boiling water for 10 min at 100°C and then cooled at room temperature. Then 2 ml of arsenomolybdate reagent was added, and the mixture was incubated for 10 min and optical density was recorded at 620 nm.
2.2.3. Assay of chitinase
Colloidal chitin was prepared according to Berger and Reynolds (Citation1958). Chitinase activity was measured by the release of NAG using colloidal chitin as a substrate according to the method of Reissig et al. (Citation1955). The chitinase activity (µkat·mg−1 protein) was calculated using the standard curve of NAG.
In a 1.5-ml eppendorf tube, 0.4-ml enzyme solution was taken and 10 µl of 0.01 M sodium acetate buffer (pH 5) was added. The reaction was carried out for 1.5 h at 37°C in a shaking water bath by adding 0.1 ml of (10 mg/ml) colloidal chitin. Reaction was stopped by centrifugation at 10,000 g for 3 min. Then 0.3 ml of supernatant was taken in a glass tube containing 30 µl of 1 M potassium phosphate buffer (pH 7.1) and incubated with 89.5 µl of snail gut enzyme for 1 h, so that pH of buffer mixture became pH 6.8. The pH of the reaction mixture was increased to 8.9 by the addition of 70 µl of 1 M sodium borate buffer (pH 9.8). Mixture was incubated in a boiling water bath for exactly 3 min and then rapidly cooled in an ice water bath. Then 2 ml of p-dimethylaminobenzaldehyde reagent was added and the mixture was incubated for 20 min at 37°C. The absorbance was read at 585 nm against enzyme blank in an ultraviolet–visible spectrophotometer.
2.3. Determination of protein
The protein content of different extracts that were used for the assay of β-1,3-glucanase and chitinase specific activity was determined using the method of Lowry et al. (Citation1951).
2.4. Western blot
For western blot samples of 0, 4, 24, and 96 h of 15-day-old plants were taken for experiment after pathogen inoculation. Protein extract was prepared by grinding 1-g leaves with 4 ml of 0.02 M Tris–HCl buffer (pH 8.0) for 1–2 min at 4°C. The homogenate was centrifuged at 10,000 g for 20 min at 4°C, and supernatant was used for sodium dodecyl sulfate polyacrylamide gel electrophoresis (SDS–PAGE) (Gupta et al. Citation2012). Protein extract was separated on 10% SDS–PAGE (Laemmli Citation1970). After electrophoresis, the proteins were electroblotted onto 0.45-mm nitrocellulose membrane (Whatman). The electrophoretic transfer of proteins from gel to membrane was carried out in a Blueflash-L Serva semi-dry transblot apparatus (96 mA, 60 min) using Tris–glycine buffer containing methanol (0.048 M Tris–HCl, pH 9.4, 0.039 M glycine, 0.037% [w/v] SDS, and 20% [v/v] methanol). The nitrocellulose membrane was taken out and incubated for 1 h with continuous shaking at room temperature in 5% Tris-buffered saline and Tween 20 (TBST; 0.01 M Tris (pH 7.5) and 0.15 M NaCl) and defatted milk to block the nonspecific binding sites. The membrane was then incubated overnight in primary antibodies (anti-rabbit class I β-1,3-glucanase and anti-tobacco class I chitinase antibody) (Agrisera, Sweden) in the concentration of 1:1000 stock in TBST. After incubation, membrane was washed with TBST two times for 10 min each. The membranes were then incubated in secondary antibody for 2 h. Alkaline phosphatase-conjugate goat anti-rabbit (IgG-ALP) (Genei, India) was used as a secondary antibody at a dilution of 1:8000. After washing, membrane was incubated with alkaline phosphatase color development reagent containing 5-bromo-4-chloro-3-indolyl phosphate and nitroblue tetrazolium. Immediately after color development, the membrane was washed in distilled water and air-dried.
2.5. Statistical analysis
The data (mean values) were statistically compared by the method of analysis of variance (Gomez & Gomez Citation1984) under a completely randomized design. The data were analyzed statistically with SPSS-17 statistical software (SPSS Inc.).
3. Results
PR proteins accumulate rapidly at the intracellular and extracellular level under various biotic and abiotic treatments (Graham et al. Citation2003). The PR proteins such as β-1,3-glucanases and chitinases play multiple roles in plant self-defense (Ham et al. Citation1991).
Our results show that in general β-1,3-glucanase and chitinase activities were higher in the inoculated-resistant plants as compared to the inoculated-susceptible and uninoculated control plants. In 15-days- and 1-month-old plants, maximum increase in the activity of β-1,3-glucanase was observed at 24 h after pathogen inoculation in all the three cultivars beyond which a gradual decrease in activity was observed. The β-1,3-glucanase activity of 15-day-old plants was 2.24-, 2.40-, and 3.45-fold higher in RMO-40, CZM-3, and FMM-96, respectively, than their corresponding control (). In one-month-old plants, the β-1,3-glucanase activity was 2.65-, 2.56-, and 2.94-fold higher in RMO-40, CZM-3, and FMM-96, respectively, than the control ().
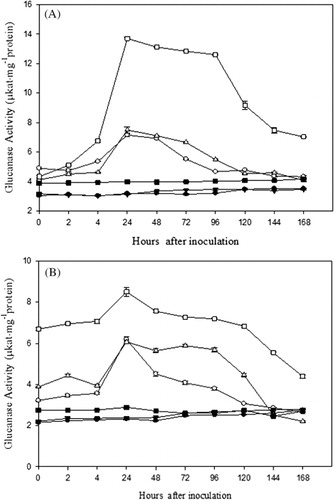
A significant increase in chitinase activity in all the three cultivars of 15-day- and 1-month-old plants of moth bean was observed at 24 h after pathogen inoculation. Thereafter a gradual decrease in activity was observed. The chitinase activity of 15-day-old plants was 4.94-, 4.76-, and 11.76-fold higher in RMO-40, CZM-3, and FMM-96, respectively, than their control (). The activation of chitinase was more rapid and higher in plants of resistant cultivar than in susceptible cultivar. In one-month-old plants, the chitinase activity was 14.11-, 14.35-, and 17.31-fold higher in RMO-40, CZM-3, and FMM-96, respectively, than the control ().
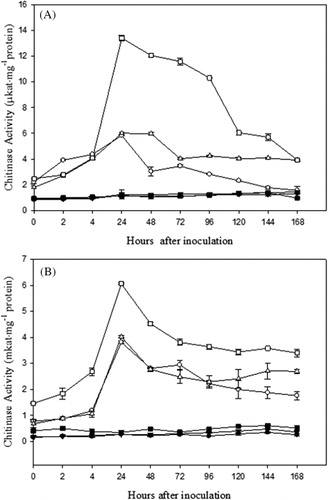
Western blot analysis revealed 33- and 30-kDa bands of β-1,3-glucanase and chitinase proteins, respectively, which were induced in pathogen-inoculated plants of moth bean, confirming the role of β-1,3-glucanase and chitinase in the disease reaction. The intensity of β-1,3-glucanase protein increased with time, and highly intense bands (33 kDa) were observed at 24 h after inoculation in all the three cultivars, FMM-96, RMO-40, and CZM-3 (). In the case of chitinase activity, induction of a 30-kDa band of chitinase protein was observed, and its intensity was also higher at 24 h in all the three cultivars, FMM-96, RMO-40, and CZM-3, after pathogen inoculation (). Control plants of both resistant and susceptible varieties showed that these PR proteins are in low amount as compared to pathogen-inoculated plants.
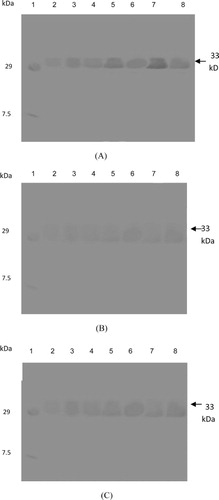
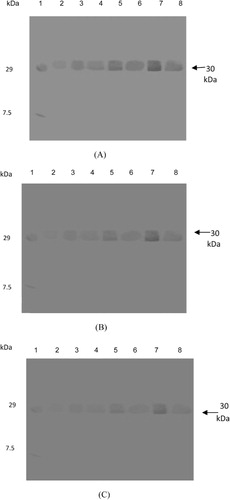
Although, faint β-1,3-glucanase and chitinase bands were observed in uninoculated control plants but the intensity of bands remarkably increased after pathogen inoculation. These observations suggest that the activity of PR proteins such as β-1,3-glucanase and chitinase in moth bean was induced after pathogen inoculation and further the increased levels of PR proteins may contribute to the resistance of these cultivars to charcoal root rot disease.
The β-1,3-glucanase and chitinase activities were determined in two age-groups of plants, that is 15 days and 1 month. The enzyme activities were found to be higher in 15-day-old plants as compared to 1-month-old plants.
The results were highly significant for β-1,3-glucanase activity in both 15-day- (p = 2.2 × 10−170) and 1-month-old (p = 1.1 × 10−159) plants as well as for chitinase activity in both 15-day- (p = 5.4 × 10−153) and 1-month-old (p = 1.64 × 10−124) plants.
4. Discussion
β-1,3-glucanase and chitinase are well-studied PR proteins which are produced by plants in response to pathogen attack (Theis & Stahl Citation2004). These enzymes are important determinants of the resistance of plants to fungal diseases (Funnell et al. Citation2004). It has been demonstrated that genetically engineered overexpression of PR proteins can increase resistance in plants (Velazhahan & Muthukrishnan Citation2004). The activation of defense mechanisms in plants is considered to be consequent upon an initial recognition event in which the host plant detects molecular components of the pathogen, known as elicitors (Van't Slot & Knogge Citation2002). In this study, we present evidence that in V. aconitifolia inoculated with M. phaseolina, the activities of chitinase and β-1,3-glucanase increase significantly in the resistant genotype in contrast with the susceptible genotype. This is in accordance with the results of Ramesh Sundar et al. (Citation2008) who investigated the induction of PR) proteins in sugarcane (Saccharum officinarum L.) leaves and suspension-cultured cells in response to treatment with a glycoprotein elicitor isolated from Colletotrichum falcatum (the red rot pathogen). Treatment of leaves and cells with the elicitor resulted in a marked increase in the activities of chitinase and β-1,3-glucanase in a red rot resistant (BO 91) than a susceptible (CoC 671) sugarcane cultivar. Similar results were obtained in wheat plants after the inoculation of leaf rust pathogen (Puccinia recondita f. sp. tritici). Resistance was associated with high constitutively expressed chitinase activity and induced β-1,3-glucanase activity (Anguelova-Merhar et al. Citation2001).
The results from our study imply that the application of fungal pathogen induces signaling process that begins upstream activation of PR proteins. Our results are also in agreement with Nazeem et al. (Citation2008) who reported an increasing trend in the β-1,3-glucanase activity over a period of 3–5 days after infection by fungus (Phytophthora capsici) with relatively higher levels in tolerant Kalluvally variety than the susceptible Panniyur-1 in Piper nigrum plants. Potato leaves infected with Phytophthora infestans also showed high glucanase activity (McDowell & Dangl Citation2000).
In an earlier study by us, Gupta et al. (Citation2012) reported the activity of β-1,3 glucanase and chitinase higher in 10-day-old plants than in the 1-month-old plants of E. sativa after pathogen inoculation. This may be due to the higher metabolic rate and growing cells of young plants as compared to the mature plants. They also showed the strong evidence of systemic resistance for airborne communication, and young plants were more effective emitters of cues as well as more responsive receivers of volatile cues.
Western blot analysis revealed the presence of a 33-kDa band of β-1,3-glucanase and 30-kDa band of chitinase in induced moth bean plants. This is similar to the results observed by Shrestha et al. (Citation2008) that healthy plants had low to undetectable levels of 28- and 35-kDa chitinases. However, after infection with Rhizoctonia solani, the level of 28- and 35-kDa chitinases increased substantially in all cultivars tested indicating that these were induced after infection. Pepper plants inoculated with P. capsici showed the accumulation of β-1,3-glucanases and chitinases in stem tissues. The 34-kDa β-1,3-glucanase isolated from a pepper stem inhibited the hyphal growth of P. capsici in vitro (Kim & Hwang Citation1997). Furthermore, it has been concluded that plants with elevated levels of chitinase and β-1,3-glucanase expression are more resistant to fungal pathogens (Datta et al. Citation2001).
Our findings showed that plants with elevated levels of chitinase and β-1,3-glucanase expression are more resistant to fungal pathogens. The results, therefore, suggest a possible role of PR proteins in defense mechanism of V. aconitifolia against the charcoal root rot disease.
5. Conclusion
This study revealed the role of PR proteins (β-1,3-glucanase and chitinase) in the defense response of moth bean with respect to charcoal root rot infection. The high native activity of β-1,3-glucanase and chitinase in the resistant cultivar, the increased higher activity upon inoculation in the resistant than susceptible cultivar, and the positive reaction in the western blot analysis confirmed the positive role of these enzymes in imparting disease resistance. Chitinases and β-1,3-glucanases have been purified and characterized from several of plant sources. Genes encoding these enzymes have also been cloned from a variety of plants. Currently, there is an immense interest in delineating the molecular events from pathogen recognition to the expression of these genes. In an effort to enhance the disease resistance, PR genes have been used to transform a variety of plant sources. Based on the results obtained through our study, it can be concluded that both chitinase and β-1,3-glucanase have a distinct role in imparting disease resistance in moth bean against M. phaseolina.
References
- Abeles FB, Bosshart RP, Forrence LE, Habig WE. 1970. Preparation and purification of glucanase and chitinase from bean leaves. Plant Physiol. 47:129–134. doi:10.1104/pp.47.1.129
- Anguelova-Merhar VS, Van der Westhuizen AJ, Pretorias ZA. 2001. β-1,3-Glucanase and chitinase activities and the resistance response of wheat to leaf rust. J Phytopathol. 149:381–384. doi:10.1046/j.1439-0434.2001.00638.x
- Antoniw JF, Ritter CE, Pierpoint WS, Van Loon LC. 1980. Comparison of three pathogenesis-related proteins from plants of two cultivars of tobacco infected with TMV. J Gen Virol. 47:79–87. doi:10.1099/0022-1317-47-1-79
- Berger LR, Reynolds DM. 1958. The chitinase system of a strain of Streptomyces griseus. Biochem Biophys Acta. 29:522–534. doi:10.1099/0022-1317-47-1-79
- Castro MS, Fontes W. 2005. Plant defense and antimicrobial peptides. Protein Pept Lett. 12:13–18.
- Datta K, Tu JM, Oliva N, Ona I, Velazhahan R, Mew TW, Muthukrishnan S, Datta SK. 2001. Enhanced resistance to sheath blight by constitutive expression of infection related rice chitinase in transgenic elite Indica rice cultivars. Plant Sci. 160:405–414. doi:10.1016/S0168-9452(00)00413-1
- Funnell DL, Lawrence CB, Pedersen JF, Schardl CL. 2004. Expression of the tobacco β-1,3-glucanase, PR-2d, following induction of SAR with Peronospora tabacina. Physiol Mol Plant Pathol. 65:285–296. doi:10.1016/j.pmpp.2005.02.010
- Gomez KA, Gomez AA. 1984. Statistical procedures for agricultural research. New York (NY): Wiley.
- Graham MY, Weidner J, Wheeler K, Pelow ML, Graham TL. 2003. Induced expression of pathogenesis-related protein genes in soybean by wounding and the Phytophthora sojae cell wall glucan elicitor. Physiol Mol Plant Pathol. 63:141–149. doi:10.1016/j.pmpp.2003.11.002
- Grant M, Lamb C. 2006. Systemic immunity. Curr Opin Plant Biol. 9:414–420. doi:10.1016/j.pmpp.2003.11.002
- Gupta P, Ravi I, Sharma V. 2012. Induction of β-1,3-glucanase and chitinase activity in the defense response of Eruca sativa plants against the fungal pathogen Alternaria brassicicola. J Plant Interact. 8:155–161. doi:10.1080/17429145.2012.679705
- Ham KS, Kauffmann S, Albersheim P, Darvill AG. 1991. Host pathogen interactions. XXXIX. A soybean pathogenesis related protein with β-1,3-glucanase activity releases phytoalexin elicitor-active heat-stable fragments from fungal walls. Mol Plant Microbe Interact. 4:545–552.
- Kasprzewska A. 2003. Plant chitinases – regulation and function. Cell Mol Biol Lett. 8:809–824.
- Kim YJ, Hwang BK. 1997. Isolation of a basic 34 kiloDalton β-1,3-glucanase with inhibitory against Phytophthora capsici from pepper stems. Physiol Mol Plant Pathol. 50:103–115. doi:10.1006/pmpp.1996.0073
- Kirubakaran SI, Sakthivel N. 2007. Cloning and over expression of antifungal barley chitinase gene in Escherichia coli. Protein Exp Purif. 52:1159–1166. doi:10.1016/j.pep.2006.08.012
- Kumar D. 2002. New concept of moth bean improvement. In: Kumar D, Singh NB, editors. Moth bean in India. Jodhpur: Scientific Publishers; p. 31–47.
- Laemmli UK. 1970. Cleavage of structural proteins during the assembly of the head of bacteriophage T4. Nature. 227:680–685. doi:10.1038/227680a0
- Lowry OH, Roserbrough NJ, Farr AL, Randell RJ. 1951. Protein measurement with the Folin-phenol reagent. J Biol Chem. 193:265–275.
- McDowell JM, Dangl JL. 2000. Signal transduction in the plant immune response. Trends Biochem Sci. 25:79–82. doi:10.1016/S0968-0004(99)01532-7
- Mihail JD, Taylor SJ. 1995. Interpreting variability among isolates for Macrophomina phaseolina in pathogenicity, pycnidium production and chlorate utilization. Can J Bot. 10:1596–1603. doi:10.1139/b95-172
- Nazeem PA, Achuthan CR, Babu TD, Parab GV, Girija D, Keshavachandran R, Samiyappan R. 2008. Expression of pathogenesis related proteins in black pepper (Piper nigrum L.) in relation to Phytophthora foot rot disease. J Trop Agr. 46:45–51.
- Nelson N. 1944. A photometric adaption of the Somogyi method for the determination of glucose. J Biol Chem. 153:375–380.
- Ramesh Sundar A, Velazhahan R, Nagarathinam S, Vidhyasekaran P. 2008. Induction of pathogenesis-related proteins in sugarcane leaves and cell-cultures by a glycoprotein elicitor isolated from Colletotrichum falcatum. Biol Plantarum. 52:321–328. doi:10.1007/s10535-008-0066-8
- Reissig JL, Strominger JL, Leloir LF. 1955. A modified colorimetric method for the estimation of N-acetylamino sugars. J Biol Chem. 217:959–966. doi:10.1007/s10535-008-0066-8
- Sharma I, Kumari N, Sharma V. 2013. Defense gene expression in Sorghum bicolor against Macrophomina phaseolina in leaves and roots of susceptible and resistant cultivars. J Plant Interact. doi:10.1080/17429145.2013.832425
- Shrestha CL, Ona II, Muthukrishnan S, Mew TW. 2008. Chitinase levels in rice cultivars correlate with resistance to the sheath blight pathogen Rhizoctonia solani. Eur J Plant Pathol. 120:69–77. doi:10.1007/s10658-007-9199-4
- Singh SK, Srivastava HP. 1988. Symptoms of Macrophomina phaseolina infections on moth bean seedling. Ann Arid Zone. 27:151–152.
- Somogyi M. 1952. Notes on sugar determination. J Biol Chem. 195:19–23.
- Theis T, Stahl U. 2004. Antifungal protein: target, mechanism and prospective applications. Cell Mol Life Sci. 61:437–455. doi:10.1007/s00018-003-3231-4
- Van Loon LC. 1999. Occurrence and properties of plant pathogenesis-related proteins. In: Datta SK, Muthukrishnan S, editors. Pathogenesis-related proteins in plants. Boca Raton (FL): CRC Press LLC, p. 1–19.
- Van't Slot KAE, Knogge W. 2002. A dual role for microbial pathogen-derived effector proteins in plant disease and resistance. Crit Rev Plant Sci. 21:229–271. doi:10.1080/0735-260291044223
- Velazhahan R, Muthukrishnan S. 2004. Transgenic tobacco plants constitutively overexpressing a rice thaumatin-like protein (PR-5) show enhanced resistance to Alternaria alternata. Biol Plant. 47:347–354. doi:10.1080/0735-260291044223
- Verdcourt B. 1970. Studies in leguminosae-papilionoideae for the flora of tropical east Africa IV. Kew. Bull. 24:235–307.