Abstract
The amino acid, protein, carbohydrate, and mineral element contents and composition of the xylem, phloem, and leaves of healthy and insect-damaged Caragana korshinskii plants were analyzed to evaluate the changes in the nutrient content of C. korshinskii after damage by Chlorophorus caragana. The amino acid content decreased in the leaf and phloem but increased in the xylem in response to damage, while the protein content did not change in the leaf, increased in the xylem, and decreased in the phloem. The carbohydrate content increased slightly in the leaf but decreased in the xylem and phloem. The six mineral elements analyzed, namely, phosphorous, potassium, magnesium, zinc, manganese, and iron decreased in the xylem, phloem, and leaf. The present results may provide a basis for understanding the mechanisms underlying the effect of C. caragana on the loss of viability of C. korshinskii.
Introduction
Caragana korshinskii is a type of deciduous shrub that belongs to the Caragana genus (Leguminosae). Most species of Caragana are resistant to both cold and high temperatures, as well as drought, and possess strong budding ability. The plant makes an excellent windbreak, and is also used for sand fixation and water and soil conservation in semidesert, desert, desert steppe, and loess hilly-gully regions in the west of China. Moreover, C. korshinskii has high economic value and can be used for manufacturing paper, fiber processing, firewood, stall food for animals, and other uses. Currently, C. korshinskii is one of the most important plant resources for vegetation restoration, ecological reconstruction, and regional economic development in the arid and semiarid areas of China.
C. korshinskii is damaged throughout its growth cycle by various pests (Cui et al. Citation2011) among which Chlorophorus caragana is the most important. C. caragana belongs to the Chlorophorus genus (Cerambycidae, Coleoptera), and its larvae bore into the stems of C. korshinskii. Most stems are bored to the point of being hollow, causing the whole tree to wither and even die. In heavily damaged areas, it is very common to see the heavily damaged C. korshinskii blown down by wind (Zong et al. Citation2012).
In recent years, C. caragana has broken out in large areas in the Zhongwei, Lingwu, and Yanchi cities of Ningxia in China. In a single plant, the population density is two to three larvae, and about 47.7–80.5% of plants are commonly damaged in 2009, which constitutes a huge threat to the large areas of planted and naturally growing C. korshinskii forests (Cao Citation2010, Cao et al. Citation2010)
Plants synthesize organic compounds (i.e. fats, proteins, etc.,) and store starch through the photosynthetic reactions of leaves to meet the needs of their own growth and other life activities. These substances are transported into trees through vascular bundles. When the vascular bundle is damaged, not only the transportation of nutrients from the leaf to the rest of the tree is impeded, but the transportation of water and mineral elements absorbed by the roots is also suppressed, which can result in the weakness or even death of the tree (Furuhashi et al. Citation2013).
The vascular bundle on stem of C. korshinskii was destroyed after the damage of C. caragana, which would lead to lack of water, mineral elements, and nutrients and, eventually, decreasing the growth vigor of the plants. Thus, it is necessary to investigate those contents change in different sections of the plant, for that will provide the valuable data underlying the death or the injury mechanism of plant C. korshinskii.
Recent studies of Caragana plants have focused on the examination of their chemical composition, bioactivity, and pharmacological activity (Zhang & Gao Citation1997; Liu et al. Citation2004; Yang et al. Citation2008). The nutrient composition of C. korshinskii has been studied to some degree. Although there are several studies addressing the biochemical composition of roots, old branches, lateral branches, and twigs (Liu et al. Citation2008), the activities of starch hydrolytic enzymes and starch mobilization in roots of C. korshinskii, following aboveground partial shoot removal (Fang et al. Citation2008), and variations of foliar carbon isotope discrimination and nutrient concentrations (Zhao et al. Citation2006), the effect of C. caragana damage on the nutrient composition of C. korshinskii has not been reported.
In the present study, a planted C.korshinskii forest was used to investigate the changes in nutrient (amino acids, proteins, and carbohydrates) and mineral element (phosphorous, potassium, and magnesium) content in different parts (i.e. leaf, xylem, and phloem) from healthy and damaged C. korshinskii to provide a theoretical basis for elucidating the mechanism underlying the loss of viability of C. korshinskii in response to damage caused by C. caragana.
Materials and methods
Materials
C. korshinskii samples were collected from a forest planted in the fixed sand dunes of Shapotou, Zhongwei city in Ningxia. The C. korshinskii trees were 7 years old, with an average height of 2.1 m and 2.4 cm in DBH.
Design of experiments
In August, 2009, healthy and damaged forests were selected on the basis of a comprehensive sampling of the forest under the same site conditions. Within the damaged forest, 81% of the C. korshinskii plants was affected by C. caragana, and the population density was 2.4 larvae per plant. Three clusters of C. korshinskii were selected randomly from the healthy forest, and one plant from each cluster was selected for a total of three trees. The growth, tree height, and basal diameter of each plant were similar. Then, three clusters of C. korshinskii were selected randomly from the damaged forest, and one plant from each cluster was selected for a total of three trees. Tree height, basal diameter, and the state of health of each plant were similar. Lastly, three positions in the trunk (i.e. 0–20, 20–40, and 40–60 cm) were selected in the six plants, and leaves were collected as samples and stored at −80 °C in liquid nitrogen.
Methods of nutrient determination
The samples were analyzed at the Analysis Laboratory of Beijing Nutrition Resources Institute. Amino acid composition was determined by the ion exchange chromatography (GB/T 5009.124-Citation2003), and protein was analyzed by the first method (GB/T 5009.5-Citation2003). The fat composition of the samples was determined by the Soxhlet extraction method (GB/T 5009.6-Citation2003). Sucrose content was analyzed by high-performance liquid chromatography (HPLC) (GB/T 22221-Citation2008). The ash content was determined by combustion (GB/T 5009.4–Citation2003) and moisture was measured using the direct drying method (GB/T 5009.3-Citation2003). The zinc content was determined by atomic absorption spectrometry (GB/T 5009.14-Citation2003), phosphorus was detected by spectrophotometry (GB/T 5009.87-Citation2003), and potassium was detected by flame emission spectrometry (GB/T 5009.91-Citation2003). Iron, magnesium, and manganese were analyzed by atomic absorption spectrophotometry (GB/T 5009.90-Citation2003). Carbohydrate content was calculated according to the following formula: 100% – (protein + moisture + ash + fat)%.
Statistical analysis
The experimental data were analyzed using Microsoft Excel 2003 (Microsoft). Graph production and tests of statistically significant differences were performed using Prism 5, t-test (GraphPad Software, Inc., La Jolla, CA, USA).
Results
Effect of insect damage on the amino acid content of C. korshinskii tissues
Amino acid composition analysis identified 18 amino acids in the xylem, phloem, and leaves of C. korshinskii, namely, Aspartate (ASP), Threonine (THR), Serine (SER), Glutamate (GLU), Glycine (GLY), Alanine (ALA), Valine (VAL), Methionine (MET), Isoleuine (ILE), Leucine (LEU), Tyrosine (TYR), Phenylalanine (PHE), Lysine (LYS), Histidine (HIS), Arginine (ARG), Proline (PRO), Tryptophan (TRP), and Cysteine (CYS). The amino acid content of the different tissues of insect-damaged C. korshinskii was significantly different from that of healthy plants (–).
Note: Data show the mean ± standard deviation (n = 3).
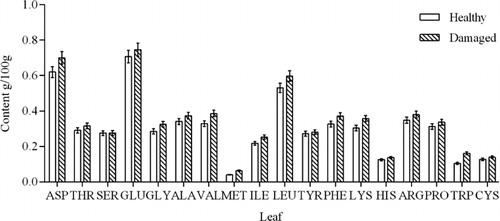
Note: Data show the mean ± standard deviation (n = 3) and the different small letters indicate significant differences compared with controls (P < 0.05).
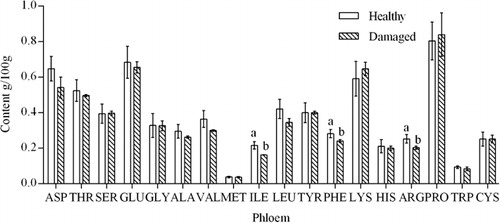
Note: Data show the mean ± standard deviation (n = 3).
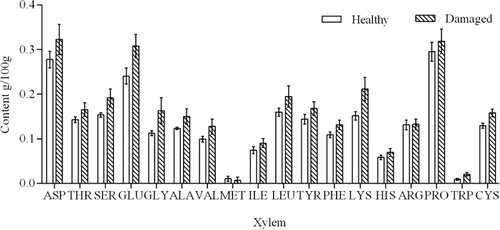
Plant leaves are the principal sites of nutrient synthesis. The differences in the amino acid composition of leaves between healthy and insect-damaged C. korshinskii are shown in . This graph shows that all amino acids showed an increasing trend associated with damage caused by C. caragana. Damaged C. korshinskii plants showed an increase of approximately 10% in the majority of amino acids compared to healthy plants. Tryptophan and Methionine showed the greatest increase, which reached over 30%. However, these changes did not reach statistical significance.
The phloem is the main transportation channel for photo assimilates in the plant. The amino acid contents of healthy and insect-damaged C. korshinskii phloem are shown in . The effect of C. caragana on the amino acid content of the phloem followed an opposite trend than that observed in the leaves. The majority of amino acids in insect-damaged C. korshinskii decreased to different degrees with the exception of serine, lysine, and proline, which showed a slight increase. Isoleucine (P = 0.012), phenylalanine (P = 0.040), and arginine (P = 0.029) showed a significant decrease by over 10%.
The xylem is an important channel for the long distance transport of amino acids. The xylem sap of plants contains different amino acids. In the xylem of insect-damaged C. korshinskii, only the methionine content decreased by 52%, while other amino acids showed an increasing trend (). Glycine (P = 0.164), lysine (P = 0.101), and tryptophan (P = 0.268) contents increased by more than 40%. However, the changes in amino acids between healthy and damaged plants did not reach statistical significance.
Effect of insect damage on the protein and carbohydrate contents of tissues of C. korshinskii
Damage by C. caragana resulted in alterations in the protein and carbohydrate contents of C. korshinskii, with a similar pattern of changes in all plant parts analyzed. Compared with healthy C. korshinskii, the leaves of damaged plants did not show significant changes in protein content, but the protein content of the xylem showed an increasing trend, while the phloem had a significant reduction by 15% in protein (P = 0.0126). As the main source of nutrition for insects, carbohydrates in the xylem (P = 0.0152) and phloem (P = 0.224) decreased in the insect-damaged C. korshinskii ().
Effect of insect damage on the mineral content of tissues of C. korshinskii
Plants need minerals for photosynthesis and to transform nutrients into the molecules needed for defense functions. The phosphorus, potassium, magnesium, zinc, manganese, and iron contents of healthy C. korshinskii were compared with those of insect-damaged C. korshinskii (). Several mineral elements decreased in the phloem, xylem, and leaves of damaged plants compared to healthy C. korshinskii. In particular, in the xylem, potassium (P = 0.0544) and magnesium (P = 0.0091) had a significant reduction by more than 30% in comparison with healthy plants.
Note: Data show the mean ± standard deviation (n = 3), the different capital letters indicate highly significant differences compared with controls (P < 0.01), and the different small letters indicate significant differences compared with controls (P < 0.05).
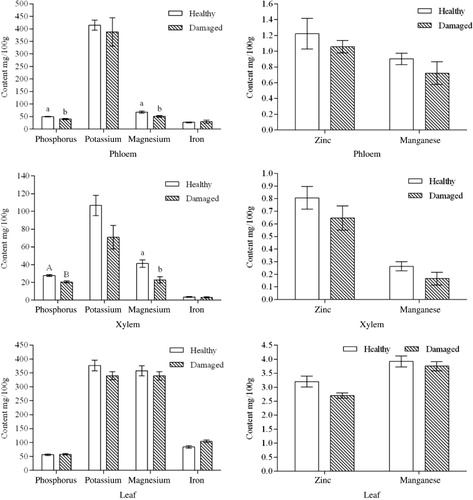
Discussion
Plants have evolved several defense mechanisms against herbivorous insects. Plants use chemical defenses mediated by the synthesis of secondary metabolites directed against insects. In addition, plants release volatiles that trigger signaling reactions to induce neighboring plants to activate their defense mechanisms. These defense-associated substances have a close relation with the nutrients in plants. Plant nutrients can be activated by a variety of physiological and biochemical processes and used as raw materials for the synthesis of defense compounds, thus enhancing the ability of a plant to resist insect attacks.
Changes in the amino acid and protein contents in C. korshinskii after insect damage
Amino acids are the raw materials for the synthesis of alkaloids and insect-resistant proteins. Damage by C. caragana triggers induced-resistance reactions in C. korshinskii. As the leaf is the principal site of amino acid synthesis, insect attack activates various physiological and biochemical processes that result in the accumulation of an increased amount of amino acids for the synthesis of defensive compounds. Compared with healthy plants, the amino acids in the leaves of damaged C. korshinskii increased to a certain degree, which indicated that the insect damage had not exceeded the tolerance range of C. korshinskii, and the plant was able to effectively activate a defense response against the pest.
The xylem and phloem are the long-distance channels of plants for the transport of amino acids, which are relocated to different parts to participate in defense responses. However, damage to the xylem caused by C. caragana impeded the transport function of C. korshinskii, which resulted in the accumulation of amino acids in the xylem of damaged plants compared with healthy plants.
The induced resistance activated in the plant in response to insect damage increased the demand for nutrients in the whole plant. However, because the xylem was blocked, the phloem served as the main transport channel, and its transport capacity could not meet the increasing demand. Compared with healthy plants, the increased amounts of amino acids in the phloem of damaged plants were transferred to other parts of the plant, which decreased the overall content in the phloem. The changes in the contents of proteins and amino acids showed similar trends.
Changes in the carbohydrate content of C. korshinskii after insect damage
Amino acids are synthesized from photosynthetic products in plants. The content of photosynthetic products such as carbohydrates changed in the leaves, xylem, and phloem of C. korshinskii in response to insect damage in a similar way to the amino acid content. On the one hand, carbohydrates generated by the photosynthetic process need to be used for the synthesis of defensive compounds to resist pests. On the other hand, the photosynthetic efficiency and the accumulation of carbohydrates were decreased because photosynthesis was inhibited and the transportation of water and mineral elements was blocked due to insect damage.
In this study, although the carbohydrate content of insect-damaged C. korshinskii leaves was similar to that of healthy plants, the content of the whole plant decreased significantly. There was a dynamic equilibrium between carbohydrate synthesis and the generation of downstream compounds for defense, and the content changes were the result of the regulation of the synthesis of defense substances by C. korshinskii.
Changes in the mineral element contents of C. korshinskii after insect damage
Mineral elements, which are necessary for the generation of photosynthate, are absorbed by roots and transported upward. In the present study, transportation of phosphorus, potassium, magnesium, and other mineral elements was found to be blocked because the xylem of C. korshinskii was damaged by C. caragana. In particular, the base of roots was damaged significantly, resulting in a lower content of minerals in the xylem, leaves, and phloem than in healthy plants. Moreover, the blocked transportation of mineral elements played an important role in inhibiting photosynthesis in insect-damaged C. korshinskii.
In summary, amino acids, proteins, carbohydrates, and other nutrients changed to different degrees after damage by C. caragana. Damage to the xylem not only blocked the transportation of mineral elements (phosphorus, potassium, etc.), which affected the synthesis of photosynthate, but also impeded the delivery of photosynthate and the generation of insect-resistance substances, thus causing changes in the nutrient content of different plant tissues. The reactions mediating the conversion of nutrients into insect-resistance substances in C. korshinskii and the effects of damage by C. caragana on transport functions and on the nutrient content in different tissues should be analyzed further.
Acknowledgment
The research was supported by the Fundamental Research Funds for the Central Universities (No. TD2011-06).
References
- Cao CJ. 2010. Studies on the bio-ecological characteristics and monitoring techniques of Chorophorus sp. [PhD Thesis]. Beijing: Beijing Forestry University.
- Cao CJ, Luo YQ, Cui YQ, Lei YS, Pu ZX, Zong SX. 2010. Study on damage characteristics and population dynamics of Chlorophorus sp. (Coleoptera: Cerambycidae). Ecol Sci. 29:127–130.
- Cui YQ, Sheng ML, Luo YQ, Zong SX. 2011. Emergence patterns of Orgyia ericae Germar parasitoids. Rev Colomb Entomol. 37: 240–243.
- Fang XW, Li JH, Xiong YC, Xu DH, Fan XW, Li FM. 2008. Responses of Caragana Korshinskii Kom. to shoot removal: mechanisms underlying regrowth. Ecol Res. 23:863–871. 10.1007/s11284-007-0449-x
- Furuhashi T, Kojima M, Sakakibara H, Fukushima A, Hirai MY, Furuhashi K. 2013. Morphological and plant hormonal changes during parasitization by Cuscuta japonica on Momordica charantia. J Plant Interact. 8:1–13. 10.1080/17429145.2013.816790
- GB/T. 5009.124-2003 2003. Determination of amino acids in foods. Beijing: Standardization Administration of the People's Republic of China.
- GB/T 5009.3-2003 2003. Determination of moisture in foods. Beijing: Standardization Administration of the People's Republic of China.
- GB/T 5009.4-2003 2003. Determination of ash in foods. Beijing: Standardization Administration of the People's Republic of China.
- GB/T 5009.5-2003 ( The first method) 2003. Determination of protein in foods. Beijing: Standardization Administration of the People's Republic of China.
- GB/T 5009.6-2003 2003. Determination of Fat in Foods. Beijing: Standardization Administration of the People's Republic of China.
- GB/T 5009.14-2003 ( The first method) 2003. Determination of zinc in foods. Beijing: Standardization Administration of the People's Republic of China.
- GB/T 5009.87-2003 2003. Determination of phosphorus in foods. Beijing: Standardization Administration of the People's Republic of China.
- GB/T 5009.90-2003 2003. Determination of iron, magnesium and manganese in foods. Beijing: Standardization Administration of the People's Republic of China.
- GB/T 5009.91-2003 2003. Determination of potassium and sodium in foods. Beijing: Standardization Administration of the People's Republic of China.
- GB/T 22221–2008 2008. Determination of fructose glucose sucrose maltose lactose in foods—high-performance liquid chromatography. Beijing: Standardization Administration of the People's Republic of China.
- Liu Y, Feng JC, Wu YL, Wang LJ. 2008. Biochemical composition of Caragana Korshinskii Kom. in Helingeer County of Inner Mongolia. J Arid Resour Environ. 22:159–162.
- Liu HX, Lin WH, Yang JS. 2004. Research progress of chemical composition and pharmacological effect on Caragana spp. Chin Pharm J.39:327–330.
- Yang ZD, Li T, Yang MJ, Li S. 2008. Research progress of chemical composition and bioactivity on Caragana spp. Chin Tradit Pat Med. 30:1678–1681.
- Zhang HS, Gao XX. 1997. Study on the microscopic structure and fiber state and chemical component of little leaf peashrup. J Neimenggu For Coll. 19:41–45.
- Zhao LJ, Xiao HL, Liu XH. 2006. Variations of foliar carbon isotope discrimination and nutrient concentrations in Artemisia Ordosica and Caragana Korshinskii at the southeastern margin of China's Tengger Desert. Environ Geol. 50:285–294. 10.1007/s00254-006-0209-1
- Zong SX, Xie GL, Wang WK, Luo YQ, Cao CJ. 2012. A new species of Chlorophorus Chevrolat (Coleoptera Cerambycidae) from China with description of biology. Zootaxa. 3157:54–60.