Abstract
A pot experiment was conducted to investigate the organic phosphorus (P) (phytate) utilization of Zea mays L. with different nitrogen (N) forms (NH4+ and NO3−) when both arbuscular mycorrhizal (AM) fungus (Funelliformis mosseae) and phosphate-solubilizing bacterium (PSB, Pseudomonas alcaligenes) are present. The soil was supplied with either KNO3 or (NH4)2SO4 (200 mg kg−1 N) with or without phytin (75 mg P kg−1). Results showed that the application of NH4+ to the soil in a plant–AM fungus–PSB system decreased rhizosphere pH and increased phosphatase activity. It also enhanced the mineralization rate of phytin, which resulted in the release of more inorganic P. The application of NO3− promoted mycorrhizal colonization and hyphal length density in the soil. The inorganic P in the hyphosphere decreased, but more P was transferred to the plant through the mycorrhizal hyphae. Hence, in addition, the application of the two different N forms did not significantly alter the content of plant P. The plant supplied with different N fertilizers acquired P through different mechanisms associated with other microbes. NH4+ application promoted phytin mineralization by decreasing soil pH, whereas NO3− application increased inorganic P uptake by strengthening the mycorrhizal pathway.
Introduction
Organic phosphorus (P) accounts for approximately 50% of the total P and even up to 80% in a range of soils (McLaughlin et al. Citation1990; Turner et al. Citation2005). Organic P considerably contributes to plant P nutrition because of its reserve of P which is potentially available to plants after hydrolyzation by phosphatases (Turner & Haygarth Citation2005; Fransson & Jones Citation2007; Turner Citation2008). Thus, the manipulation of biological functions to mobilize and utilize organic P is important.
Plants can mineralize organic P by excreting phosphatases to the rhizosphere. Their phosphatase activities are affected by various environmental factors, such as soil pH. Different forms of nitrogen (N) fertilizer such as NH4+ and NO3− have distinctive influence on rhizosphere pH. Our previous study demonstrated that rhizosphere acidification mediated by NH4+ can enhance the phytin utilization of maize under sterile conditions (Ding et al. Citation2011). However, plants in agricultural ecosystems are associated with soil microbes, such as arbuscular mycorrhizal (AM) fungi and phosphate-solubilizing bacteria (PSB). AM fungi, which form associations with the roots of more than 80% of land plant species, can improve plant P nutrition by releasing H+ to mobilize insoluble phosphates and enlarging the P-absorbing zone around the rhizosphere (Smith & Read Citation2008; Sathiyadash et al. Citation2012). AM fungi also affect organic P mineralization, transformation, or turnover (Feng et al. Citation2003; Richardson et al. Citation2009). PSB exist widely in the soil and may constitute up to 40% of the cultivable population of soil bacteria (Kucey Citation1983), which increase soil-available P for plant uptake through the mineralization of organic P (Rodríguez & Fraga Citation1999). Although both AM fungi and PSB help plants cope with P deficiency by mobilizing organic P (Smith & Read Citation2008; Jung & Tamai Citation2013), the factors that affect this coping process remain unknown.
In this study, we structured a plant–AM fungus–PSB system by inoculating soil near the root of maize with both AM fungus and PSB. The soil in this system was supplied with different N forms (NH4+ and NO3−). Previous studies (Li et al. Citation1991; Ding et al. Citation2011) reported that the addition of different N forms can decrease or increase soil pH in the rhizosphere and hyphosphere (the volume of soil influenced by the AM fungal hyphae, see Jansa et al. Citation2013). We hypothesized that the bioavailability of organic P (phytin) and phosphatase activity from the root and/or PSB in the rhizosphere and hyphosphere increase under low pH mediated by NH4+ fertilizer. Then the inorganic P concentration was increased and improved plant P uptake. The phytin utilization rate of maize was improved by NH4+ than by NO3− in the plant–AM fungus–PSB system.
Materials and methods
Biological materials and soil
The P-efficient maize (Zea mays L.) genotype 181, which was developed for cultivation in calcareous soils in the Northeastern China, was used as the experimental plant. The P uptake-related root physiological traits of genotype 181, such as proton and acid phosphatase excretion under P-deficient conditions, have been previously described (Liu et al. Citation2004). The AM fungal inoculum used was Funelliformis mosseae (FM) (Nicol. and Gerd.) Gerdemann and Trappe (BEG 167). FM was kindly supplied by Professor Youshan Wang from the Institute of Plant Nutrition and Soil Science, Beijing Academy of Agriculture and Forestry Sciences. Pseudomonas alcaligenes (PA) M20, which utilizes phytin as the sole P source and has antibiotic resistance to ampicillin and tetracycline, was selected as the bacterial inoculum. PA was kindly provided by Professor Sanfeng Chen from the China Agricultural University, Beijing, China.
Samples of a moderately acid (pHwater 6.4) clay soil were collected in Tai'an, Shandong Province, China. Each sample contained 7.27 g kg−1 organic matter, 7.2 mg kg−1 mineral N (NO3− and NH4+, as analyzed using continuous-flow analysis [TRAACS 2000]), 3.3 mg kg−1 Olsen-P, and 97.6 mg kg−1 NH4Cl-exchangeable K. The soil samples were air-dried, sieved (2 mm), and then mixed with fine river sand. The mixture was supplemented with 50 mg kg−1 Mg (as MgSO4), 5 mg kg−1 Cu (as CuSO4), 5 mg kg−1 Mn (as MnSO4), and 5 mg kg−1 Zn (as ZnSO4), and sterilized by γ-ray (radiation dosimetry: 10 kGy 60Co γ-ray, Beijing Radiation Application Research Center, Beijing, China).
Plant growth condition and experimental design
The microcosm unit used in the experiment was a plastic pot with a diameter of 15 cm and a height of 20 cm. Each pot received 2 kg of the soil and sand mixture. The culture medium had two organic P levels after it was added to the pots. Half of the pots were added with 75 mg kg−1 organic P in the form of phytin (P0410, Tokyo Chemical Industry, Tokyo, Japan). The other half were not supplied with organic P. Each organic P application level in pots included two N fertilizer forms. Half of the total number of pots were supplied with (NH4)2SO4 (200 mg N kg−1 soil), and the other half were supplied with KNO3 (200 mg N kg−1 soil). The nitrification inhibitor 3, 4-dimethylpyrazole phosphate (1 mg kg−1 soil) was added to the soil to prevent microbial nitrification.
Maize seeds were disinfected with 10% (v/v) H2O2 for 10 min and 70% (v/v) ethanol for 3 min, after which they were rinsed eight times with sterile deionized water. After imbibing in water at 27°C in the dark for 2 days, three seedlings were transferred to the pots. They thinned into one seedling 3 days after their transfer. In each pot, 1000 FM fungal spores isolated by wet sieving were added. PA was cultured in liquid LB medium at 37°C for 24 h at 180 rpm and then diluted to 109 colony-forming units (CFUs) mL−1 as the inoculum. In each pot, 10 mL of PA suspension was added to the soil around the roots. A small PVC box (4 cm × 4 cm × 4 cm) containing 100 g soil was placed in the middle of the pot. One side of each box was covered by a 30-µm mesh to separate fungal hyphae from plant roots in the soil. The soil in the box was collected as hyphosphere soil.
The experiment was set up in a randomized block design in a greenhouse for 6 weeks from May to June in 2010 at the temperature range of 24–30°C under natural light intensity. The soil samples were treated with two organic P levels (0 or 75 mg kg−1 P as phytin) and two N forms [200 mg N kg−1 of (NH4)2SO4 or KNO3]. Each treatment was replicated four times, and each pot had one maize plant. During the experiment, the soil moisture was maintained at 18–20% humidity (w/w, c. 70% of water holding capacity), as determined gravimetrically by weighing the pots every 2 days and adding water as necessary.
Harvests and sample analyses
The maize plants were separated into shoot and root tissues. The rhizosphere soil was collected by removing the soil that adhered to the roots via shaking and then mixed in a blender to obtain a uniform mixture for subsequent analysis (see below). The soil in the small box was mixed uniformly to serve as the hyphosphere soil. The shoot and root P in the shoots and roots was determined through the vanadomolybdate yellow method (Jackson Citation1958) after digestion with H2SO4 and 30% H2O2 (Thomas et al. Citation1967).
Mycorrhizal colonization and hyphal length density
The mycorrhizal colonization of roots was measured following the method described by Trouvelot et al. (Citation1986). External mycorrhizal hyphae were extracted from two 5 g soil samples from the hyphosphere soil in the small box, using the membrane filter technique (Staddon et al. Citation1999). Hyphal length was assessed using the gridline intercept method at 200× magnification and then converted to hyphal length density (m g−1 DW [dry weight] soil).
Rhizosphere pH and phosphatase activity
The pH of the rhizosphere soil was measured in a 1:5 soil to water (w/v) mixture by a pH meter. The realistic phosphatase activities in the rhizosphere and hyphosphere soil samples were measured using buffers with a pH according to the measured pH in each soil. A 1-mL aliquot of each liquefied agar sample was pipetted into a 5-mL Eppendorf tube containing 2 mL of a 50 mM acetate buffer with the mean rhizosphere pH of a similar rhizosphere. Subsequently, 0.4 mL of the substrate (150 mM p-nitrophenyl phosphate; Sigma St. Louis, MO, USA) in an acetate buffer was added. The mixture was incubated at 30°C for 30 min. The reaction was terminated by adding 2.5 mL of 0.5 M NaOH. After the mixture was centrifuged for 5 min at 1500× g, the phosphatase activity in the rhizosphere was quantified by comparing its absorption at 410 nm with a standard curve of diluted p-nitrophenol phosphate solutions (Carreira et al. Citation2000).
Rhizosphere P concentration
Samples of air-dried rhizosphere soil (2 g) were extracted in 50 mL polypropylene tubes using 10 volumes of sterile deionized water. The soil samples were extracted at 25 ± 1°C for 30 min in a reciprocal shaker at 300 rpm and then centrifuged at 10,300× g for 15 min. The P concentrations of the soil extracts were determined from a 1-mL subsample of each solution. The inorganic P concentration was determined by measuring the inorganic concentration of solutions using malachite green reagent (Irving & McLaughlin Citation1990). The total P concentration was determined by autoclaving the samples at 121°C for 40 min with 0.6 M H2SO4 and 3.3% ammonium persulfate (Schoenau & Huang Citation1991) and then conducting the same analysis as that for inorganic P concentration. Corrections for volume loss during autoclaving were made as necessary, based on gravimetric analyses. The organic P concentration in the extracts was calculated deducting the inorganic P concentration from the total P concentration.
Hyphosphere and rhizosphere PSB number
The abundance of PSB in the rhizosphere and hyphosphere soil samples was determined using bacterial plate counts. Approximately 2.5 g of fresh soil was added to 25 mL sterile 0.9% w/v normal saline and then agitated on a rotary shaker for approximately 30 min. Ten-fold serial dilutions of the resulting suspension were made in sterile normal saline, and 100 µL of the samples was spread-plated in triplicate on Luria–Bertani agar supplemented with ampicillin (10 µg mL−1) and tetracycline (10 µg mL−1). The agar plates were incubated at 37°C for 2 days before counting the colonies.
Statistical analysis
The significant effects of N form, organic P level, and their interaction on the variables were determined by standard two-way analysis of variance (ANOVA) using SPSS software, version 16.0 (SPSS Inc., Table S1Footnote1). The differences between the treatments were determined by t-test (P < 0.05).
Results
The P values of two-way ANOVA analyses results are shown in Table S1. They indicated the effects of N form, phytin, and their interactions on the determined variables.
Plant dry weight and P content
The plant shoot DW was significantly increased by phytin addition in the soil () with both N forms, while the increase was higher in the soil with NH4+ than that in the soil with NO3−. However, only root DW was increased in the NH4+ treatments with phytin addition. In addition, phytin significantly increased plant shoot P content under both N forms but only significantly increased root P content in the soil with NH4+ (). N forms had no significant influence on both shoot and root P contents regardless of the phytin addition.
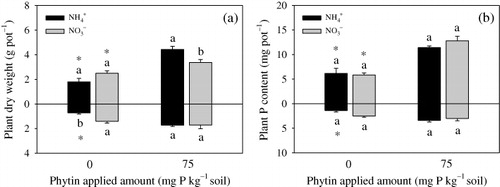
Rhizosphere pH and phosphatase activity
Comparing the soil pH at the beginning of the experiment, rhizosphere pH decreased with NH4+ but increased with NO3− () regardless of the addition of phytin. However, rhizosphere pH increased with the addition of phytin in the NO3− treatment but had no changes in the NH4+ treatment.
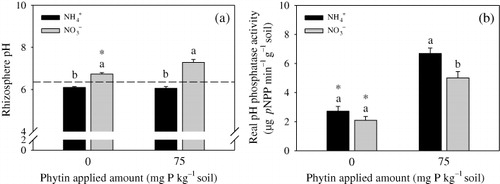
Phosphatase activity significantly increased in the soil with phytin in NH4+ or NO3− treatment (). Without phytin, the soil samples treated with NH4+ or NO3− showed no significant difference in phosphatase activity; with phytin, the soil sample treated with NH4+ had higher phosphatase activity than that treated with NO3−.
Inorganic and organic P concentrations
Without phytin, the rhizosphere soil treated with NH4+ had higher inorganic P concentration than that treated with NO3− (). With phytin, there was no significant difference of inorganic P concentration between the rhizosphere soil treated with NO3− and NH4+. With or without phytin, the rhizosphere soil treated with NH4+ had lower organic P concentration than that treated with NO3− ().
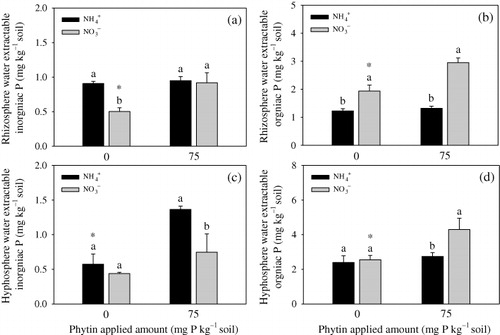
Hyphosphere inorganic P concentration in the NH4+ treatment was higher than that in the NO3− treatment with phytin addition (). It increased significantly with the addition of phytin in the NH4+ treatment. Hyphosphere organic P concentration in the NH4+ treatment was lower than that in the NO3− treatment with phytin addition (). However, it increased with the addition of phytin only in the NO3− treatment.
Mycorrhizal colonization, hyphae length density, and bacterial number
Root mycorrhizal colonization was enhanced by the addition of phytin regardless of N-applied forms (). Whether or not with phytin addition, the colonization in the NO3− treatment had higher colonization than that in the NH4+ treatment. Hyphae length density (collected from the small box) was promoted by the addition of phytin in both N forms (). NO3− treatment had a higher root length density than NH4+ treatment irrespective of phytin addition.
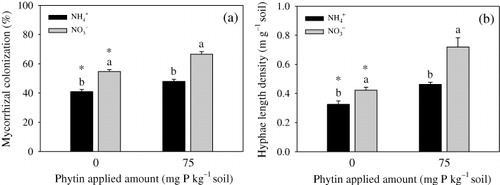
Bacterial numbers in the rhizosphere and hyphosphere were more than 108 CFUs g−1 soil. Addition of phytin and different N forms had no significant influence on bacterial number.
Discussion
Plant P uptake from phytin affected by different N forms
Because application of NH4+ and NO3− fertilizer had opposite changes in rhizosphere pH, we expected that these changes could influence the activity of phosphatases from roots and/or bacteria, which finally affected phytin mineralization and plant P uptake in a plant–AM fungus–PSB system. In the present study, plant P contents increased significantly when 75 mg P kg −1 soil phytin was added, but there was no significant difference between the NH4+ and NO3− treatment regardless of the phytin application (). These results showed that the added phytin was utilized by maize, while the plant P uptake was not affected by different N forms. It was different from a previous study that plant P uptake of maize was increased by the application of NH4+ compared to NO3− (Ding et al. Citation2011). These different results suggested that the effect of pH regulation on plant P uptake in the sterile condition was not consistent with the plant–AM fungus–PSB system.
Phytin utilization mediated by different N forms
In the present experiment, the rhizosphere pH was increased by application of the NO3− and decreased by application of NH4+ compared with the initial soil pH (). With phytin, the soil treated with NH4+ had higher phosphatase activity than that treated with NO3− (). Water-soluble organic P concentration in the NH4+ treatment was lower than that in the NO3− treatment. These results suggested that application of NH4+ fertilizer enhances phosphatase activity in the rhizosphere by decreasing the soil pH to a certain level and then promoting the mineralization rate of phytin. Phosphatase activities can be regulated by changing soil pH conditions (George et al. Citation2008). Similarly, a number of studies have shown that phosphatase activities in rhizosphere and hyphosphere soils can be enhanced by applying NH4+ under sterile conditions (Ding et al. Citation2011; Wang et al. Citation2013). The present results showed that the phosphatase activity was increased when NH4+ was added in the plant–AM fungus–PSB system. These data indicated that the total phosphatases released from the root and bacteria have a high efficiency of hydrolyzation under relative low pH.
Mycorrhizal P uptake pathway mediated by different N forms
AM fungal hyphae enlarge the absorption area of roots and function as the second P uptake pathway in plants (Smith & Read Citation2008). The mycorrhizal colonization rate and hyphal length in the soil are the two main indexes used to evaluate the properties of mycorrhizal fungi. The present study showed that the mycorrhizal colonization and hyphal length density were higher in the soil treated with NO3− than in the soil treated with NH4+. This finding suggested that NO3− could enhance mycorrhizal formation and extra-hyphae growth which may be unrelated to the change of pH. The hyphosphere soil treated with NO3− had higher water-extractable organic P content than that with the NH4+ treatment, but lower inorganic P content than that treated with NH4+. This finding indicates that the increased hyphal length density in the NO3− -supplied soil promotes the uptake and subsequent adsorption of inorganic P by the plant. Extra-hyphae can absorb P from the soil of 7 cm from the root surface (Li et al. Citation1991). A previous study found that the amount of plant P uptake has a positive relationship with the hyphal length density in the soil (Kabir et al. Citation1998). The higher mycorrhizal colonization rate in the soil treated with NO3− suggested that the extra-hyphae could transfer more P to the plant via the AM fungi.
The bacterial number of inoculated PSB exceeded 108 CFUs mg−1 soil in the rhizosphere and hyphosphere (). The roots and hyphae elicit a ‘priming effect’ on the bacteria and enhance their activity of phytin mineralization (Kuzyakov Citation2002) and P immobilization by transforming into matrix-bound phosphate (MBP) (Wu et al. Citation2007). The NH4+ and NO3− treatment elicited no significant changes in the number of PSB. This result suggested that the application of different N forms may have same influence on MBP content immobilized by the added PSB.
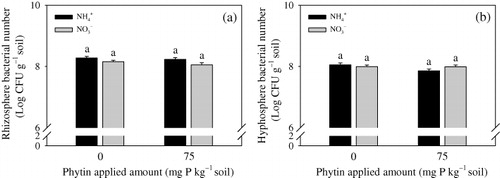
In conclusion, the application of NH4+ and NO3− could promote plant P uptake by enhancing organic P mineralization or mycorrhizal P uptake in the plant–AM fungus–PSB system. The application of NH4+ fertilizer to the soil increased phosphatase activity by reducing pH in the rhizosphere. Furthermore, it enhanced the mineralization rate of phytin and increased the amount of available P. The application of NO3− fertilizer strengthened plant P uptake via the mycorrhizal pathway. Furthermore, it increased mycorrhizal colonization and extra-hyphal length density which decreased inorganic P in the hyphosphere and transferred more P to the plant via the mycorrhizal hyphae. With phytin, the soil samples treated with both forms of N showed no significant difference in plant P contents. However, plants in natural and agricultural ecosystems are associated not with one but various various fungi (and among them also several AM fungal species) and bacteria. Thus, such plants are more complex than the systems in the present study. Future research should focus on the interaction of plants with more types of AM fungi and bacteria.
Supplemental_Material.doc
Download MS Word (31.5 KB)Acknowledgments
We acknowledge the financial support from the National Natural Science Foundation of China (31201693, 31372139), the Specialized Research Fund for the Doctoral Program of Higher Education (20120008130001), the Science Foundation of Guangdong province (S2012040006938), and Guangdong Academy of Sciences (qnjjsq201102; rcjj201202).
Notes
1. Supplementary Content may be viewed online at http://dx.doi.org/10.1080/17429145.2013.861028.
References
- Carreira J, Garcia-Ruiz R, Lietor J, Harrison A. 2000. Changes in soil phosphatase activity and P transformation rates induced by application of N-and S-containing acid-mist to a forest canopy. Soil Biol Biochem. 32:1857–1865. 10.1016/S0038-0717(00)00159-0
- Ding X, Fu L, Liu C, Chen F, Hoffland E, Shen J, Zhang F, Feng G. 2011. Positive feedback between acidification and organic phosphate mineralization in the rhizosphere of maize (Zea mays L.). Plant Soil. 349:13–24. 10.1007/s11104-011-0783-1
- Feng G, Song Y, Li X, Christie P. 2003. Contribution of arbuscular mycorrhizal fungi to utilization of organic sources of phosphorus by red clover in a calcareous soil. Appl Soil Ecol. 22:139–148. 10.1016/S0929-1393(02)00133-6
- Fransson AM, Jones DL. 2007. Phosphatase activity does not limit the microbial use of low molecular weight organic-P substrates in soil. Soil Biol Biochem. 39:1213–1217. 10.1016/j.soilbio.2006.11.014
- George TS, Gregory PJ, Hocking P, Richardson AE. 2008. Variation in root-associated phosphatase activities in wheat contributes to the utilization of organic P substrates in vitro, but does not explain differences in the P-nutrition of plants when grown in soils. Environ Exp Bot. 64:239–249. 10.1016/j.envexpbot.2008.05.002
- Irving GCJ, McLaughlin MJ. 1990. A rapid and simple field test for phosphorus in Olsen and Bray no. 1 extracts. Commun Soil Sci Plant Anal. 21:2245–2255. 10.1080/00103629009368377
- Jackson ML. 1958. Soil chemical analysis. Englewood Cliffs (NJ): Prentice Hall.
- Jansa J, Bukovská P, Gryndler M. 2013. Mycorrhizal hyphae as ecological niche for highly specialized hypersymbionts – or just soil free-riders? Front Plant Sci. 4:134. 10.3389/fpls.2013.00134
- Jung NC, Tamai Y. 2013. Polyphosphate (phytate) formation in Quercus acutissima–Scleroderma verrucosum ectomycorrhizae supplied with phosphate. J Plant Interact. 8:291–303. 10.1080/17429145.2013.816789
- Kabir Z, O'Halloranb IP, Fylesa JW, Hamela C. 1998. Dynamics of the mycorrhizal symbiosis of corn (Zea mays L.): effects of host physiology, tillage practice and fertilization on spatial distribution of extra-radical mycorrhizal hyphae in the field. Agric Ecosyst Environ. 68:151–163. 10.1016/S0167-8809(97)00155-2
- Kucey R. 1983. Phosphate-solubilizing bacteria and fungi in various cultivated and virgin Alberta soils. Can J Soil Sci. 63:671–678. 10.4141/cjss83-068
- Kuzyakov Y. 2002. Review: factors affecting rhizosphere priming effects. J Plant Nutr Soil Sci. 165:382–396. 10.1002/1522-2624(200208)165:4%3C382::AID-JPLN382%3E3.0.CO;2-#
- Li XL, George E, Marschner H. 1991. Phosphorus depletion and pH decrease at the root-soil and hyphae-soil interfaces of VA-mycorrhizal white clover fertilized with ammonium. New Phytol. 119:397–404. 10.1111/j.1469-8137.1991.tb00039.x
- Liu Y, Mi G, Chen FJ, Zhang JH, Zhang FS. 2004. Rhizosphere effect and root growth of two maize (Zea mays L.) genotypes with contrasting P efficiency at low P availability. Plant Sci. 167:217–223. 10.1016/j.plantsci.2004.02.026
- McLaughlin M, Baker T, James T, Rundle J. 1990. Distribution and forms of phosphorus and aluminum in acidic topsoils under pastures in south-eastern Australia. Soil Res. 28:371–385. 10.1071/SR9900371
- Richardson AE, Hocking PJ, Simpson RJ, George TS. 2009. Plant mechanisms to optimise access to soil phosphorus. Crop Pasture Sci. 60:124–143. 10.1071/CP07125
- Rodríguez H, Fraga R. 1999. Phosphate solubilizing bacteria and their role in plant growth promotion. Biotechnol Adv. 17:319–339.
- Sathiyadash K, Muthukumar T, Uma E, Pandey RR. 2012. Mycorrhizal association and morphology in orchids. J Plant Interact. 7:238–247. 10.1080/17429145.2012.699105
- Schoenau JJ, Huang WZ. 1991. Anion-exchange membrane, water and sodium bicarbonate extractions as soil tests for phosphorus. Commun Soil Sci Plant Anal. 22:465–492. 10.1080/00103629109368432
- Smith SE, Read DJ. 2008. Mycorrhizal symbiosis. San Diego (CA): Academic Press.
- Staddon PL, Fitter AH, Graves JD. 1999. Effect of elevated atmospheric CO2 on mycorrhizal colonization, external mycorrhizal hyphal production and phosphorus inflow in Plantago lanceolata and Trifolium repens in association with the arbuscular mycorrhizal fungus Glomus mosseae. Glob Change Biol. 5:347–358. 10.1046/j.1365-2486.1999.00230.x
- Thomas RL, Sheard RW, Moyer JR. 1967. Comparison of conventional and automated procedures for nitrogen, phosphorus, and potassium analysis of plant material using a single digestion. Agron J. 59:240–243. 10.2134/agronj1967.00021962005900030010x
- Trouvelot A, Kough JL, Gianinazzi-Pearson V. 1986. Mesure du taux de mycorhization VA d'un système radiculaire. Recherche de méthodes d'estimation ayant une signification fonctionnelle. In: Physiological and genetical aspects of mycorrhizae. Paris, France: INRA Press.
- Turner BL, Cade-Menun BJ, Condron LM, Newman S. 2005. Extraction of soil organic phosphorus. Talanta. 66:294–306. 10.1016/j.talanta.2004.11.012
- Turner BL, Haygarth PM. 2005. Phosphatase activity in temperate pasture soils: potential regulation of labile organic phosphorus turnover by phosphodiesterase activity. Sci Total Environ. 344:27–36. 10.1016/j.scitotenv.2005.02.003
- Turner BS. 2008. The body and society: explorations in social theory. London, UK: Sage.
- Wang F, Jiang RF, Kertesz M, Zhang FS, Feng G. 2013. Arbuscular mycorrhizal fungal hyphae mediating acidification can promote phytate mineralization in the hyphosphere of maize (Zea mays L.). Soil Biol Biochem. 65:69–74. 10.1016/j.soilbio.2013.05.010
- Wu JS, Huang M, Xiao HA, Su YR, Tong CL, Huang DY, Syers JK. 2007. Dynamics in microbial immobilization and transformations of phosphorus in highly weathered subtropical soil following organic amendments. Plant Soil. 290:333–342. 10.1007/s11104-006-9165-5