Abstract
Hydrogen peroxide (H2O2) functions as a signal molecule in plants under abiotic and biotic stresses. Leaves of detached maize (Zea mays L.) seedlings were used to study the function of H2O2 pretreatment in osmotic stress resistance. Low H2O2 concentration (10 mM) which did not cause a visual symptom of water deficit (leaf rolling) was applied to the seedlings. Exogenous H2O2 alone increased leaf water potential, endogenous H2O2 content, abscisic acid (ABA) concentration, and metabolite levels including soluble sugars, proline, and polyamines while it decreased lipid peroxidation and stomatal conductance. Osmotic stress induced by polyethylene glycol (PEG 6000) decreased leaf water potential and stomatal conductance but enhanced lipid peroxidation, endogenous H2O2 content, the metabolite levels, and ABA content. H2O2 pretreatment also induced the metabolite accumulation and improved water status, stomatal conductance, lipid peroxidation, ABA, and H2O2 levels under osmotic stress. These results indicated that H2O2 pretreatment may alleviate water loss and induce osmotic stress resistance by increasing the levels of soluble sugars, proline, and polyamines thus ABA and H2O2 production slightly decrease in maize seedlings under osmotic stress.
Introduction
Drought stress is the primary cause of crop loss across the world, reducing average yields in most major crop plants. Under drought or water-deficit stress condition, plants exhibit a wide range of biochemical and physiological responses at the molecular, cellular, and whole-plant levels, such as changed leaf water potential (ψ leaf) and stomatal conductance (g s), increased abscisic acid (ABA) content, and the accumulation of metabolites including soluble sugars, proline, and amines such as glycine betaine and polyamines. These metabolites accumulate under water-deficit stress and function as osmolytes that contribute to the maintaining of cell turgor, antioxidants, or scavengers that help plants to avoid and/or tolerate stresses (Mahajan & Tuteja Citation2005; Seki et al. Citation2007; Fariduddin et al. Citation2013). The changes in these metabolites at the cellular level are associated with protecting cellular function with maintaining the structure of cellular components. ABA is also essential for various stress responses, including stomatal closure, stress-responsive gene expression, and metabolic changes (Seki et al. Citation2007).
The production of reactive oxygen species (ROS) is a common phenomenon in plants under biotic and abiotic stress conditions. ROS can induce cellular damage by degradation of proteins, inactivation of enzymes, alterations in the gene, and interfere in various pathways of metabolic importance (Mahajan & Tuteja Citation2005). To scavenge the ROS, plants possess antioxidant enzymes, including superoxide dismutase, ascorbate peroxidase, guaiacol peroxidase, catalase, glutathione reductase, dehydroascorbate reductase, and monodehydroascorbate reductase, and antioxidants such a reduced glutathione and ascorbate. Hydrogen peroxide (H2O2), a major kind of ROS plays an important role in signal transduction for abiotic stress tolerance, although H2O2 is toxic at high concentrations (Foyer & Noctor Citation2009). Some authors suggested that the application of H2O2 at low concentrations could improve plant tolerance to abiotic stresses such as drought (He et al. Citation2009), heat (Gao et al. Citation2010), and heavy metal stresses (Hu et al. Citation2009). Hydrogen peroxide induced increases in antioxidant enzyme activities of plants, which are subjected to abiotic stress factors, has also been reported earlier. For instance, Liu et al. (Citation2010) reported that pretreatment of two cucumber varieties with H2O2 improved osmotic stress resistance by activating antioxidant system. However, to the best of our knowledge, there is little information available on the effects of H2O2 pretreatment on metabolite levels including soluble sugars, proline, and polyamines and ABA content in plants under osmotic stress.
Despite a large number of reports detailing plant tolerance mechanisms to osmotic stress, plant responses to H2O2 pretreatment under osmotic stress are not still obvious. The aim of this paper was to investigate effects of H2O2 pretreatment for improving osmotic stress tolerance of maize seedlings and the role of some metabolites such as proline, soluble sugars, and polyamines and phytohormone ABA in this process.
Materials and methods
Plant material, growth, and H2O2/osmotic stress treatment
Maize (Zea mays L.) seeds were provided from Black Sea Agricultural Research Institute, Samsun, Turkey, and were surface sterilized with 0.1% HgCl2 for 3 min followed by repeated washings with sterilized distilled water. Seeds were sown in plastic pots (16 cm height, 18 cm top, and 12 cm bottom diameter) containing peat and sand (5:1). Seedlings were grown in a growth chamber with the following parameters: 16 h light and 8 h darkness at 25 ± 2°C, relative humidity 60 ± 5%, and photon flux density at the surface of the leaves 400 µmol m−2s−1. When the fourth leaves were fully expanded, the seedlings were excised 2 cm high from soil and rinsed in distilled water in test tubes wrapped with aluminum foil for 1 h to relieve the effects of wound stress due to excision. After that the seedlings were pretreated with distilled water with or without 10 mM H2O2 for 6 h, and then they were submitted to the osmotic stress treatments with 3% polyethylene glycol 6000 (PEG 6000) containing osmotic potential of −0.3 MPa for 12 h or kept in distilled water for 12 h in the growth chamber with an air temperature of 25°C ± 2 and relative humidity 50 ± 2%. Hence, the seedlings were submitted to four treatments: treated with distilled water (Control), pretreated with H2O2 and not osmotic stressed (H2O2), osmotic-stressed only (PEG), and pretreated with H2O2 and osmotic stressed (H2O2 + PEG). After the treatments, samples of the second leaves were used for the following analyses.
Measurements of leaf water status
Leaf water potential (ψ leaf) was measured with a PSYPRO thermocouple psychrometer (Wescor, USA). Discs about 6 mm in diameter were cut from the youngest fully expanded leaves of the plants and sealed in the C-52 psychrometer chamber. Samples were equilibrated for 60 min before the readings were recorded by a water potential data logger in the psychrometric mode.
Degree of leaf rolling (%), a visual indication of internal plant water deficit (Kadioglu & Terzi Citation2007), was measured as a percentage reduction in leaf width of leaf midportion by the rolling according to Saglam et al. (Citation2008).
Stomatal conductance
Stomatal conductance (g s) was monitored by an AP4 dynamic diffusion porometer (Delta T Devices, UK) after calibration with a standard calibration plate following manufacturer's instructions.
Lipid peroxidation
Lipid peroxidation was measured in the term of malondialdehyde (MDA) content (ε = 155 mM−1 cm−1), a product of lipid peroxidation, following the method of Heath and Packer (Citation1968). Leaf samples (0.5 g) were homogenized in 10 ml of 0.1% (w/v) trichloroacetic acid (TCA). The homogenate was centrifuged at 15,000g for 5 min. To the 1 ml aliquot of the supernatant, 4 ml of 0.5% (w/v) thiobarbituric acid (TBA) in 20% (w/v) TCA was added. The mixture was heated at 95°C for 30 min and then quickly cooled in an ice bath. After centrifugation at 10,000g for 10 min, the absorbance of the supernatant was recorded at 532 and 600 nm. The MDA content was determined using a standard curve and expressed as nmol MDA g−1 dry weight.
H2O2 content
Endogenous H2O2 content was determined according to modified method of Velikova et al. (Citation2000). Leaves (0.25 g) were ground in 3 ml of 5% TCA with 0.1 g activated charcoal at 0°C. The homogenate was centrifuged at 12,000g for 15 min. To 0.5 ml aliquot of the supernatant, 0.5 ml of 10 mM potassium phosphate buffer (pH 7.0) and 0.75 ml of 1 M KI were added. The absorbance was measured at 390 nm, and H2O2 content was expressed as µmol g−1 dry weight.
Determination of proline and total soluble sugars
Determination of proline content was achieved according to the method of Bates et al. (Citation1973). Dry leaf sample (0.2 g) was homogenized in 10 ml of 3% (w/v) aqueous sulfosalicylic acid solution. Homogenate was centrifuged at 10,000g for 10 min. Supernatant was filtered through Whatman No 2 filter paper. Later, 1 ml filtrate was mixed with equal volumes of glacial acetic acid and ninhydrin reagent (1.25 g ninhydrin, 30 ml of glacial acetic acid, 20 ml 6 M H3PO4), and incubated for 1 h at 100°C. The reaction was stopped by placing the test tubes in an ice bath. The samples were rigorously mixed with 3 ml toluene. After 50 min, the light absorption of the toluene phase was estimated at 520 nm on a UV-VIS spectrophotometer. The proline concentration was determined using a standard curve. Free proline content was expressed as µg proline g−1 dry wt.
Total soluble sugar content was determined by phenol sulfuric acid method (Dubois et al. Citation1956). Dry leaves (0.1 g) were extracted with 5 ml of 80% ethanol by boiling the samples in glass tubes in a 95°C water bath for 10 min. After extraction, the tubes were centrifuged at 580g for 5 min, and the supernatants of the extractions were used for sugar analysis. To 900 µl of distilled water, 100 µl of the sample was added, and the mixture was shaken vigorously. To 1 ml of sample, 1 ml of 5% phenol, and 5 ml of H2SO4 were added, and the mixture was stirred. After cooling at room temperature for 15 min, the absorbance of the sample was recorded at 490 nm.
Determination of polyamines
Extraction of leaf samples and High Performance Liquid Chromatography (HPLC) determination of biogenic amines (putrescine (Put), spermine (Spm), and spermidine (Spd)) were carried out according to the procedures developed by Ben-Gigirey et al. (Citation1998). To extract biogenic amines, 10 ml of 0.4 M perchloric acid containing a known amount of 1,7-diaminoheptane as an internal standard was added to 5 g of leaf samples, and the mixture was homogenized for 3 min. The homogenate was centrifuged at 3000g at 4°C for 10 min. The supernatant was collected, and the residue was extracted twice with the same volume of 0.4 M perchloric acid solution. All supernatants were combined, and the final volume was adjusted to 25 ml with 0.4 M perchloric acid. The extract was filtered through Whatman paper No. 1. One milliliter of each of the extract was used for the HPLC analysis followed by derivatization with dansyl chloride.
Derivatization of biogenic amines was carried out according to developed method of Ben-Gigirey et al. (Citation1998). One milliliter of each extracted sample or standard amine solution was mixed with 200 µl of 2 M sodium hydroxide and 300 µl of sodium hydrogen carbonate. Two milliliters of a dansyl chloride solution (10 mg/ml in acetone) was added to the mixture and then incubated in water bath at 40°C for 45 min. One hundred microliters of 25% ammonium hydroxide were added to stop the reaction and to remove residual dansyl chloride. After 30 min incubation at room temperature, the final volume was adjusted to 5 ml by adding acetonitrile. Finally, the mixture was centrifuged at 2500g for 5 min, and the supernatant was filtered through 0.2 µm-pore-size filter (Pall Co., Acrodisc Syringe Filters, 25 mm, 0.2 µm, Woongki Science Co., Seoul, Korea). The filtered supernatant was kept at −25°C until assayed by HPLC. All samples were subjected to HPLC injection.
Quantitative analyses of polyamines were carried out using a HPLC unit (Shimadzu, LC 20 AT/Prominence, Japan), consisted of two pumps and a UV–VIS detector. Separation was achieved using a C18 Supelco column 5 µm (250 mm × 4.6 mm). The mobile phase was ammonium acetate (0.1 M; solvent A) and acetonitrile (solvent B) at the flow rate 1 ml min−1 with gradient elution program for 35 min. The sample volumes injected were 10 µl. The samples were monitored at 254 nm. Each HPLC run took about 35 min, and afterward the column was conditioned again with the mixture of 65% solvent A and 35% solvent B.
Determination of ABA
Leaves were grounded in liquid nitrogen, homogenized in distilled water at a ratio of 1:7 (w/v), and placed overnight in the dark at 4°C. The extracts were centrifuged at 10,000g for 10 min at 4°C, and the resulting supernatant was diluted four times in standard TBS buffer. ABA in these extracts was quantified using the Phytodetek ABA enzyme linked immunosorbent assay (ELISA) kit (Agdia/Linaris) according to the manufacturer's instructions.
Statistical analysis
All experiments were repeated three times with five biological replicates. Variance analysis of mean values was performed with Duncan Multiple Comparison test (one-way analysis of variance [ANOVA]) using SPSS software for Microsoft Windows (Ver. 13.0, SPSS Inc., USA). Statistical significance of the means between control and treatments were evaluated at the 5% (P < 0.05) probability level.
Results
ψleaf, gs, and leaf rolling
Low H2O2 concentration (10 mM) was preferred for exogenous H2O2 pretreatment because leaf rolling used as a visual indication of water deficit was observed in concentrations higher than 10 mM H2O2 (data not presented). Exogenous H2O2 alone significantly increased ψleaf but decreased gs value in the seedlings compared to the control. Osmotic stress supplied by application of 3% PEG 6000 decreased ψleaf and gs (), and thus induced leaf rolling (). Leaf rolling was observed about 120 min after PEG treatment. In course of time, leaf rolling degree (%) increased (). Pretreatment of H2O2 (H2O2 + PEG) improved ψleaf and gs values (). Hence, leaf rolling delayed in pretreated seedlings (H2O2 + PEG) compared to osmotic-stressed only ones (PEG) (). The degree of leaf rolling (%) was also low in pretreated seedlings ().
Note: The seedlings were submitted to four treatments: treated with distilled water (control), pretreated with H2O2 and not osmotic stressed (H2O2), osmotic-stressed only (PEG), pretreated with H2O2 and osmotic stressed (H2O2 + PEG). Vertical bars represent standard deviation of the means of six replicates. Different letters denote significant differences among different treatments at p < 0.05.
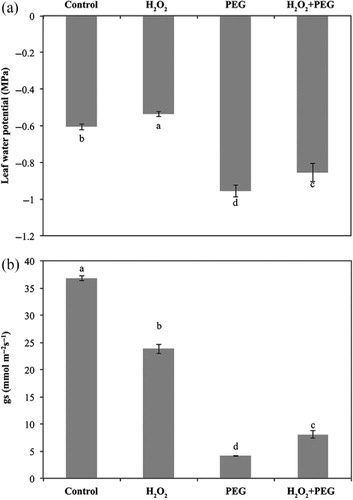
Table 1. Effect of exogenous H2O2 on leaf rolling in detached maize seedlings under osmotic stress conditions.
Lipid peroxidation and H2O2 content
As shown in , exogenous H2O2 alone decreased MDA content. The exposure of osmotic stress greatly increased MDA content in maize seedlings. H2O2 pretreatment also alleviated lipid peroxidation under osmotic stress.
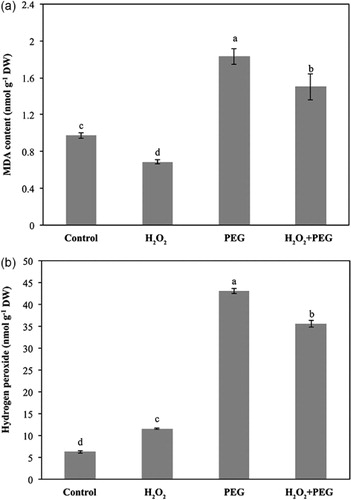
Foliar H2O2 content after exogenous application was slightly elevated in comparison to control seedlings. Osmotic stress caused a sharp H2O2 accumulation in the seedlings. The H2O2 pretreatment brought a substantial decline of endogenous H2O2 level under osmotic stress ().
Proline and total soluble sugars
The proline contents of H2O2-treated only seedlings (H2O2) interestingly increased as compared to the control seedlings. PEG exposure with H2O2 pretreatment also increased proline content. There is no significant increase in pretreated seedlings (H2O2 + PEG) compared to osmotic-stressed only ones ().
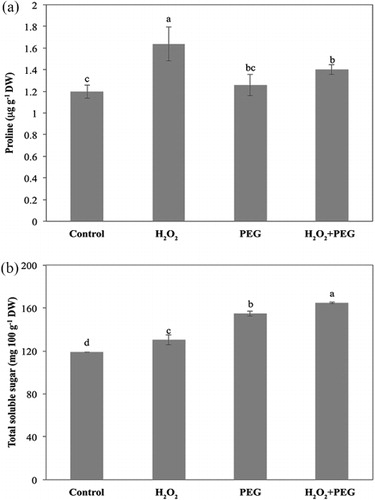
The total soluble sugar content of the control seedlings was measured as 118.9 ± 0.2 mg 100 g−1 dry weight. Exogenous H2O2 alone (H2O2) significantly increased total soluble sugar content. Osmotic stress enhanced the soluble sugar content more than H2O2-treated only group. H2O2 pretreatment also apparently induced the soluble sugar production in maize seedlings under osmotic stress ().
Polyamines
H2O2 treatment alone (H2O2) induced polyamine accumulation as compared to the control group. Osmotic stress treatment alone (PEG) also caused a significant increase in polyamine contents in maize leaves. For instance, the increases in Put, Spd, and Spm contents in osmotic-stressed only seedlings were approximately 57, 81 and 29% of the control, respectively. Furthermore, H2O2 pretreatment increased overproduction of polyamines under osmotic stress. Thus, H2O2 pretreatment increased leaf Put, Spd, and Spm contents by 72, 106, and 68% of the control under the stress, respectively ().
ABA
ABA content clearly increased in the H2O2-treated only and osmotic-stressed only groups compared to the control. When the H2O2-osmotic-stressed seedlings (H2O2 + PEG) was compared with the osmotic-stressed only (PEG) seedlings, ABA content in H2O2 + PEG was slightly lower than those of osmotic-stressed seedlings ().
Discussion
The present study explores the ameliorative effect of H2O2 on maize seedlings subjected to osmotic stress and the role of some metabolites in this process. Results of the present study showed that H2O2 treatment only caused increased ψ leaf thanks to stomatal closure partially, and osmolytes accumulation, maintaining turgor potential and osmotic adjustment. Indeed, it is known that one way to maintain the turgor potential is by osmotic adjustment. Osmotic adjustment is the net accumulation of solutes in a cell of a plant in response to a fall in the water potential of the cell's environment. As a consequence of this net accumulation, the osmotic potential of the cell is lowered, which in turn attracts water into the cell, and this tends to maintain turgor pressure (Kirkham Citation2011). Also we determined that the leaves pretreated with 10 mM H2O2 (H2O2 + PEG) exhibited improved ψ leaf and g s as compared to osmotic-stressed only leaves (PEG). As a result of decreased water loss with H2O2 pretreatment, leaf rolling, a visual indication of water loss in plants, retarded and the rolled leaves slightly opened under osmotic stress ( and ). Similar results were previously observed in osmotic-stressed wheat seedlings (He et al. Citation2009) and drought-stressed soybean plants (Ishibashi et al. Citation2011), but in those studies, parameter of leaf rolling was not used to evaluate water status in the plants. Here, we can express that the ameliorative effect of H2O2 on maize plants under osmotic stress can be visually observed by leaf rolling. H2O2 pretreatment may limit water loss by increasing the levels of metabolite involved in osmotic adjustment such as soluble sugar, proline, and polyamines under osmotic stress and thus contribute to the stress acclimation of maize plants.
We observed that exogenous applications of H2O2 at low concentration significantly decreased lipid peroxidation of maize plants under both osmotic-stressed and nonstressed conditions. Similar results in membrane damage were reported in wheat seedlings under drought by He et al. (Citation2009) and in cucumber plants under osmotic stress by Liu et al. (Citation2010). Compared with the untreated seedlings, decrease in MDA level with 10 mM H2O2 pretreatment under osmotic stress might be an induction that exogenous H2O2 at low concentrations alleviated membrane damages by inducing the metabolite levels involved in osmotic adjustment.
The role H2O2 in stomatal closure was described previously (e.g. Pei et al. Citation2000; Zhang et al. Citation2001). Accordance with g s values, we also determined that endogenous H2O2 content slightly increased in H2O2-treated only seedlings compared to control. The increase might be a result from penetration of exogenous H2O2 to leaf tissues. Also, we observed that endogenous H2O2 level increased under osmotic stress. However, H2O2 content was significantly low in plants subjected to a combined treatment with H2O2 and osmotic stress in comparison with the PEG treatment. He et al. (Citation2009) found the same results in drought-stressed wheat seedlings had arisen from H2O2-treated seeds. H2O2 accumulation was also alleviated in cucumber leaves under osmotic stress in the case of foliar spray of H2O2 (Liu et al. Citation2010). It was known that the endogenous H2O2 concentration depended on the balance between the rates of its production and its enzymatic and nonenzymatic utilization. Thus, our results indicated the induction of ROS scavenging compounds such as proline and polyamines in seedlings pretreated with H2O2 under osmotic stress.
As known, plants exhibit the accumulation of metabolites including proline, soluble sugars, and amines such as glycine betaine and polyamines under the various stress conditions (Seki et al. Citation2007). Proline can protect plants from the stress through different mechanisms, including osmotic adjustment, detoxification of ROS, protection of membrane integrity, and stabilization of proteins (Seki et al. Citation2007; Patade et al. Citation2011). On the other hand, to the best of our knowledge, only single study investigated the relationship among H2O2-pretreatment, the metabolites, ABA, and drought tolerance in plants (Abass & Mohamed Citation2011). As different from our study, these researches were used in the seeds of common bean for H2O2-pretreatment, and they were similarly recorded that H2O2-pretreatment of the seeds enhanced the level of some metabolites such as proline, soluble sugars, and polyamines in the shoots under drought condition. Our present data showed that proline content of H2O2-treated only seedlings was higher than the other treatments (). Based on known properties of the proline, the increases might be ascribed in detoxification of exogenous H2O2. The H2O2 pretreatment under osmotic stress also induced proline accumulation compared to control seedlings. Thus, we suggested that exogenous H2O2 enhanced proline content to alleviate deleterious effects of the osmotic stress.
It is well known that sugar accumulation contributes to regulation of internal osmolarity and protection of the biomolecules and membranes (Seki et al. Citation2007). In the current study, osmotic stress increased the total soluble sugar content in maize leaves (). Furthermore, we found that the highest soluble sugar content was in H2O2-pretreated plants under osmotic stress conditions. As mentioned above, Abass and Mohamed (Citation2011) recorded that H2O2-pretreatment of common bean seeds led to a significant accumulation of soluble sugars in the shoots under drought stress. In line with these data, we could express that exogenous H2O2 applications might protect the water status of the tissues by increasing soluble sugars involved in osmotic regulation.
The results showed that the main polyamines, Put, Spd, and Spm significantly increased under the stress alone or in combination with pretreatment of H2O2. Generally, plant species are endowed with a great capacity to enhance polyamine biosynthesis in response to abiotic stresses including drought (Erdei et al. Citation1996). For the first time, in the present study, the obtained results showed that H2O2 pretreatment increased the overproduction of polyamines including Put, Spd, and Spm in osmotic-stressed maize seedlings treated with H2O2 at the vegetative stage. Similar to our results, Abass and Mohamed (Citation2011) recorded the same results in common bean plants arising from H2O2-treated seeds grown under drought stress. So, we concluded here that as a result of H2O2 applications, increased polyamine content might protect the membranes by scavenging of ROS including H2O2. Indeed, we determined that exogenous H2O2 at low concentrations lessened membrane damages.
We also found that ABA content increased in H2O2-treated only seedlings compared to the control group. Accordingly, increased ABA slightly decreased gs value. ABA was known as a factor responsible for stomatal closure (Seki et al. Citation2007). On the other hand, ROS-induced ABA accumulation in root tips of wheat seedlings was recorded by Zhao et al. (Citation2001). As similar to results of Hassine et al. (Citation2009), ABA accumulation in H2O2-treated only seedlings also stimulated synthesis of the osmolytes. Again, ABA, the key phytohormone involved in response to abiotic stress mainly triggers the drought protection (Mahajan & Tuteja Citation2005). Here, we determined that PEG treatment increased the content of ABA in maize leaves, while H2O2 pretreatment under osmotic stress conditions relieved the production of ABA. Accordingly, gs values were affected by PEG and/or H2O2 applications. Also, our findings showed that H2O2 application improved water status under osmotic stress thus ABA content decreased compared to osmotic-stressed only plants due to alleviating of the stress. As similar to our findings, Zhang et al. (Citation2007) recorded that ABA was gradually degraded upon removal of drought stress. On the other hand, high osmolyte level in H2O2 + PEG seedlings compared to PEG in spite of decreased ABA showed that other mechanisms induced by H2O2 might also induce osmolyte synthesis under osmotic stress. Therefore, the interactions among H2O2, ABA, and osmolytes should be studied further.
In summary, the results of this study indicate that delay in leaf rolling may be used for a visual symptom for early detection of stress relief. Furthermore, exogenous H2O2 treatment can enhance tolerance of maize seedlings to osmotic stress by increasing some metabolite and phytohormone levels and by decreasing lipid peroxidation and endogenous H2O2 content. The protection mechanism of H2O2 pretreatment against osmotic stress should be investigated in molecular level further.
Acknowledgments
This work is supported by Turkish National Science Foundation (Project No. 111T511). We thank Prof. Dr. Sevim Köse (Faculty of Marine Science, Karadeniz Technical University) and Assist. Prof. Dr. Serkan KORAL (Faculty of Fisheries, Rize University) for their assistance with analysis of HPLC.
References
- Abass MS, Mohamed HI. 2011. Alleviation of adverse effects of drought stress on common bean (Phaseolus vulgaris L.) by exogenous application of hydrogen peroxide. Bangladesh J Bot. 40:75–83.
- Bates LS, Waldren RP, Teare ID. 1973. Rapid determination of free proline for water stress studies. Plant Soil. 39:205–207. 10.1007/BF00018060
- Ben-Gigirey B, De Sousa JMVB, Villa TG, Barros-Velazquez J. 1998. Changes in biogenic amines and microbiological analysis in albacore (Thunnus alalunga) muscle during frozen storage. J Food Prot. 61:608–615.
- Dubois M, Gilles KA, Hamilton JK, Rebers PA, Smith F. 1956. Colorimetric method for determination of sugars and related substances. Anal Chem. 28:350–356. 10.1021/ac60111a017
- Erdei L, Szegletes Z, Barabás K, Pestenácz A. 1996. Responses in polyamine titer under osmotic and salt stress in sorghum and maize seedlings. J Plant Physiol. 147:599–603. 10.1016/S0176-1617(96)80052-6
- Fariduddin Q, Varshney P, Yusuf M, Ahmad A. 2013. Polyamines: potent modulators of plant responses to stress. J Plant Interact. 8:1–16. 10.1080/17429145.2012.716455
- Foyer CH, Noctor G. 2009. Redox regulation in photosynthetic organisms: signaling, acclimation, and practical implications. Antioxid Redox Signal. 11:861–905. 10.1089/ars.2008.2177
- Gao Y, Guo YK, Lin SH, Fang YY, Bai JG. 2010. Hydrogen peroxide pretreatment alters the activity of antioxidant enzymes and protects chloroplast ultrastructure in heat-stressed cucumber leaves. Sci Hortic. 126:20–26. 10.1016/j.scienta.2010.06.006
- Hassine AB, Ghanem ME, Bouzid S, Lutts S. 2009. Abscisic acid has contrasting effects on salt excretion and polyamine concentration of an inland and a coastal population of the Mediterranean xero-halophyte species Atriplex halimus. Ann Bot. 104:925–936. 10.1093/aob/mcp174
- He L, Gao Z, Li R. 2009. Pretreatment of seed with H2O2 enhances drought tolerance of wheat (Triticum aestivum L.) seedlings. Afr J Biotechnol. 8:6151–6157.
- Heath RL, Packer L. 1968. Photoperoxidation in isolated chloroplast. I. Kinetics and stoichiometry of fatty acid peroxidation. Arch Biochem Biophys. 125:189–198. 10.1016/0003-9861(68)90654-1
- Hu Y, Ge Y, Zhang C, Ju T, Cheng W. 2009. Cadmium toxicity and translocation in rice seedlings are reduced by hydrogen peroxide pretreatment. Plant Growth Regul. 59:51–61. 10.1007/s10725-009-9387-7
- Ishibashi Y, Yamaguchi H, Yuasa T, Iwaya-Inoue M, Arima S, Zheng SH. 2011. Hydrogen peroxide spraying alleviates drought stress in soybean plants. J Plant Physiol. 168:1562–1567. 10.1016/j.jplph.2011.02.003
- Kadioglu A, Terzi R. 2007. A dehydration avoidance mechanism: leaf rolling. Bot Rev. 73:290–302. 10.1663/0006-8101(2007)73[290:ADAMLR]2.0.CO;2
- Kirkham MB. 2011. Elevated carbon dioxide: impacts on soil and plant water relations. Boca Raton, FL: CRC Press.
- Liu ZJ, Guo YK, Bai JG. 2010. Exogenous hydrogen peroxide changes antioxidant enzyme activity and protects ultrastructure in leaves of two cucumber ecotypes under osmotic stress. J Plant Growth Regul. 29:171–183. 10.1007/s00344-009-9121-8
- Mahajan S, Tuteja N. 2005. Cold, salinity and drought stresses: an overview. Arch Biochem Biophys. 444:139–158. 10.1016/j.abb.2005.10.018
- Patade VY, Bhargava S, Suprasanna P. 2011. Salt and drought tolerance of sugarcane under iso-osmotic salt and water stress: growth, osmolytes accumulation, and antioxidant defense. J Plant Interact. 6:275–282. 10.1080/17429145.2011.557513
- Pei ZM, Murata Y, Benning G, Thomine S, Klüsener B, Allen GJ, Grill E, Schroeder JI. 2000. Calcium channels activated by hydrogen peroxide mediate abscisic acid signaling in guard cells. Nature. 406:731–734. 10.1038/35021067
- Saglam A, Kadioglu A, Terzi R, Saruhan N. 2008. Physiological changes in them in post-stress emerging Ctenanthe setosa plants under drought conditions. Russ J Plant Physiol. 55:48–53. 10.1134/S1021443708010056
- Seki M, Umezawa T, Urano K, Shinozaki K. 2007. Regulatory metabolic networks in drought stress responses. Curr Opin Plant Biol. 10:296–302. 10.1016/j.pbi.2007.04.014
- Velikova V, Yordanov I, Edreva A. 2000. Oxidative stress and some antioxidant systems in acid rain-treated bean plants. Protective role of exogenous polyamines. Plant Sci. 151:59–66. 10.1016/S0168-9452(99)00197-1
- Zhang M, Duan L, Tian X, He Z, Li J, Wang B, Li Z. 2007. Uniconazole-induced tolerance of soybean to water deficit stress in relation to changes in photosynthesis, hormones and antioxidant system. J Plant Physiol. 164:709–717. 10.1016/j.jplph.2006.04.008
- Zhang X, Zhang L, Dong F, Gao J, Galbraith DW, Song CP. 2001. Hydrogen peroxide is involved in abscisic acid-induced stomatal closure in Vicia faba. Plant Physiol. 126:1438–1448. 10.1104/pp.126.4.1438
- Zhao Z, Chen G, Zhang C. 2001. Interaction between reactive oxygen species and nitric oxide in drought-induced abscisic acid synthesis in root tips of wheat seedlings. Aust J Plant Physiol. 28:1055–1061.