Abstract
Indole-3-acetic acid (IAA)-producing bacteria Kocuria turfanensis strain 2M4 was isolated from the rhizospheric soil of halotolerant plant Suaeda fruticosa from a unique saline desert of Little Rann of Kutch, Gujarat, India. Rhizobacteria was bright orange pigmented, gram-positive, coccoid, non-endospore forming, and aerobic in nature. 16S rRNA gene sequence analysis showed that 2M4 isolate matched best with type strain of K. turfanensis HO-9042T. Isolate optimally produced 38 µg ml−1 IAA when growth medium was supplemented with 600 µg ml−1 of L-tryptophan. Thin layer chromatography and Fourier transform infrared spectroscopy analysis were performed to corroborate IAA production. To characterize rhizobacterial isolate as a plant growth-promoting bacteria, it was tested for phosphate solubilization where it solubilized maximum 12 µg ml−1 phosphate in presence of fructose, produced 53% siderophore units under iron-free minimal MM9 medium and produced 1.8 µmol ml−1 ammonia in peptone water broth. Plant growth promotion by test isolate was studied on groundnut (Arachis hypogaea L.) under non-saline and saline soil. There was increase by 18% in total plant length and 30% in fresh biomass observed under non-saline control soil. Under saline soil, test isolate showed 17% increase in total length of the plant and 13% increase in fresh biomass.
Introduction
The genus Kocuria was proposed by Stackebrandt et al. (Citation1995) which was earlier classified in the genus Micrococcus. The members of this genus are gram-positive, coccoid, aerobic, non-encapsulated, and non-endospore forming belonging to the order of Actinomycetales. This genus of Actinobacteria has been isolated from different sources such as air, fermented sea food, mammalian skin, soil, the rhizoplane, freshwater, seawater, marine sediment, and desert soil (Kaur et al. Citation2011). At present, 17 species belonging to Kocuria genus have validly published names, available at http://www.bacterio.cict.fr/k/kocuria.html. The genus Kocuria includes several halotolerant strains including K. kristinae, K. rhizophila, and K. marina which can tolerate up to 10% NaCl in growth medium (Kovacs et al. Citation1999; Kim et al. Citation2004). K.'varians, K. rhizophila, K. flava, and other unnamed species of Kocuria have been reported to produce large amount of indole-3-acetic acid (IAA) (Saharan & Nehra Citation2011; Vicene et al. Citation2012). IAA is a common natural auxin and a product of L-tryptophan metabolism of microorganisms. It influences several parameters in the plant, namely elongation and cell division, apical dormancy, and differentiation of vascular tissues (Aloni et al. Citation2006; Ali et al. Citation2010).
IAA production is one of the important trait of plant growth-promoting bacteria widely known as PGPR. This term PGPR was first coined by Kloepper and Schroth (Citation1978) addressing the group of bacteria that helps to enhance plant growth by inhabiting rhizosphere of plant. Commonly known traits of PGPR include phytohormone production such as IAA, production of ammonia from nitrogenous organic matter, solubilization of phosphate, production of siderophores which helps in suppression of plant pathogenic bacteria, and so on (Ganeshan & Kumar Citation2005; Ahmad et al. Citation2008; Ali et al. Citation2011). Most routinely studied PGPR species producing IAA belongs to Pseudomonas, Bacillus, Azotobacter, Azospirrulam, and Rhizobia genus (Aeron et al. Citation2011; Bhattacharyya & Jha Citation2012). Despite of well-documented history for species belonging to Kocuria genus as IAA producers, they have been poorly investigated for their potential as PGPR.
In this present work, we isolated efficient IAA-producing strain ‘2M4’ from the rhizosphere of Suaeda fruticosa, native of saline desert of Little Rann of Kutch, Gujarat, India. 2M4 isolate produced bright orange-colored colonies on nutrient agar and could tolerate up to 8% NaCl in the growth medium (Goswami et al. Citation2013a). 16S rRNA gene sequence analysis showed the isolate belonged to Kocuria genus. Further biochemical characterization of Kocuria sp. 2M4 matched with the type strain of Kocuria turfanensis HO-9042T as described by Zhou et al. (Citation2008). Kocuria sp. 2M4 was further tested for other plant growth-promoting traits including phosphate solubilization under the presence of various sugars, ammonia production, and siderophore production. To test the plant growth-promoting ability of Kocuria sp. 2M4, talc-based bioformulation was prepared and its efficacy was tested on the seedling growth of groundnut (Arachis hypogaea L.) in presence and absence of NaCl stress under pot trials.
Materials and methods
Isolation and screening for IAA-producing bacteria
Rhizospheric soil of halotolerant plant S. fruticosa from a unique saline desert of Little Rann of Kutch, Gujarat, India (23°51′N, 71°28′E) was collected. Soil sample was suspended in standard normal saline in the ratio of 1:9 and vortex for 20 min at room temperature. The suspension of soil in normal saline was serially diluted and its 0.1 ml aliquot was inoculated on modified nutrient agar (peptic digest of animal tissue 5.0 g L−1, sodium chloride 5.0 g L−1, beef extract 1.5 g L−1, yeast extract 1.50 g L−1, and final pH at 25°C was 7.4 ± 0.2) procured from HiMedia Laboratories, supplemented with 5% NaCl using spread plant technique and incubated for 24 h at 35 ± 2°C. Morphologically distinct colonies were subcultured to obtain pure cultures.
To screen the halotolerant organisms producing IAA, all the isolates obtained were grown in the nutrient broth supplemented with 5% NaCl and 500 µg ml−1 of L-tryptophan. Spectrophotometric estimation of IAA was performed as per the method developed by Brick et al. (Citation1991) with modifications adapted by Goswami et al. (Citation2013b). 72-h old culture supernatants of the bacterial isolates were mixed with Salkowski reagent (50 ml, 35% of perchloric acid, 1 ml 0.5 M FeCl3 solution) in the ratio of 1:1. Development of pink color indicates the production of IAA and its optical density was recorded at 530 nm. Concentration of IAA produced was estimated against standard curve of IAA (HiMedia) in the range of 10–100 µg ml−1. We have already published this part of research involving isolation and screening of IAA-producing bacteria (Goswami et al. Citation2013a). Present focus is to identify, characterize, and test plant growth-promoting ability of novel isolate designated 2M4 which showed bright orange colored colonies on nutrient agar and produced IAA.
Identification of bacterial isolate using 16S rRNA gene sequence
16S rRNA gene sequence analysis of IAA-producing bacterial isolate was performed to identify the organism. To amplify 16S rRNA gene, universal forward primer: 5′-AGAGTTTGATCCTGGCTCAG-3′ and reverse primer: 5′-AAGGAGGTGATCCAGCCGCA-3′ from 1st BASE (Agile Lifescience Technologies India Pvt. Ltd.) were used. Amplified gene product ∼1.6 kb was sequenced at 1st BASE. Gene sequence homology was tested using BLASTn search program (http://www.ncbi.nlm.nih.gov). Gene sequence obtained was aligned by ClustalW using MEGA 4.0 software (Tamura et al. Citation2007) and a neighbor-joining tree with bootstrap value 500 was constructed. The gene sequence was submitted to GenBank and accession number was assigned.
Biochemical characterization
Morphological analyses were performed by using a standardized method (Murray et al. Citation1994). Physiological tests including growth at different temperatures, pH, and NaCl concentrations were performed by growing the strain in nutrient broth under appropriate test conditions. Biochemical tests described in Bergey's Manual of Systematic Bacteriology were performed as per the methods described by Shi et al. (Citation2012). All the media and reagents required to perform biochemical tests were obtained from HiMedia. Sugar utilization tests were performed using HiCarbo™ Kit-KB009 from HiMedia.
Characterization of bacterial IAA
Correlation between IAA produced and supplemented L-tryptophan was determined. Isolate 2M4 was grown in nutrient broth with varying concentrations of supplemented L-tryptophan in the range of 0–600 µg ml−1 and IAA was determined at 24 h, 48 h, 72 h, 96 h, and 120 h as per the spectrophotometric method described earlier.
To confirm the production of IAA by isolate 2M4, IAA was extracted from the 72-h old culture supernatant and analyzed using thin layer chromatography (TLC) and Fourier transform infrared spectroscopy (FTIR). To extract the IAA produced by isolate 2M4, 72-h old culture supernatant (as sample) and un-inoculated medium supplemented with L-tryptophan (as control) were acidified to pH 2.5 using 1 N HCl and extracted thrice using equal volume of ethyl acetate. Fraction of ethyl acetate was air dried and redissolved in one-tenth of the culture supernatant volume in methanol.
TLC analysis for confirmation of IAA production
For TLC analysis, standards of L-tryptophan, IAA, and indole-3-butyric acid (IBA) were prepared in methanol (200 µg ml−1), 30 µl of bacterial methanolic extract along with each standard were spotted on aluminum-backed silica gel 60 F254 TLC foils (4 × 6 cm) of 0.25-mm thickness (Merck, Darmstadt, Germany). Spots were allowed to develop using the mobile phase isopropanol:ammonia:water (16:3:1 [v/v]). After running, TLC foils were dried and observed under 256- and 360-nm UV light.
FTIR analysis for confirmation of IAA production
In order to confirm the production of IAA by bacterial isolate based on information about its chemical bonds and molecular structure, FTIR analysis was carried out. Methanolic extract was completely dried and mixed with spectral grade potassium bromide, and FTIR spectral analysis of IAA was recorded at the transmission mode from 400–4000 cm−1 using Thermo Scientific Nicolet FTIR 6700.
Assessment of phosphate solubilization ability
The method developed by Pikovskaya (Citation1948) was used for quantitative estimation of tricalcium phosphate solubilization. Phosphate solubilization ability of test isolate was determined in presence of different sugars (conc. 1%) including glucose, fructose, sucrose, and mannitol in the modified Pikovskaya's medium. The concentration of the soluble phosphate was estimated from the supernatant by stannous chloride method (King Citation1932) after seven days of incubation at 30 ± 2°C. Drop in the pH caused by organic acid production due to sugar utilized by test isolate was determined.
Ammonia production
Nesslerization reaction described by Cappucino and Sherman (Citation1992) was employed to estimate ammonia. Bacterial isolate was grown in peptone water broth for five days at 29 ± 2°C. Culture supernatant (0.2 ml) was mixed with Nessler's reagent (1 ml) and volume of this mixture was made up to 8.5 ml by the addition of ammonia-free distilled water. Development of brown to yellow color was measured at 450 nm using spectrophotometer. The concentration of ammonia was estimated using the standard curve of ammonium sulfate in the range of 0.1–1 µmol ml−1.
Quantitative estimation siderophore
Siderophore produced by isolate was quantified using chrome azurol S (CAS) shuttle assay described by Payne (Citation1994). Test isolate was grown in iron-free MM9 minimal medium (HiMedia) supplemented with 1% (w/v) glucose. CAS assay solution was mixed with culture supernatant in equal proportion and allowed to stand for 20 min. Presence of siderophore removes the iron from the dye complex and causes reduction in the intensity of blue color which is recorded at 630 nm. For the measurements, minimal medium was used as blank and % siderophore units were calculated by following formula – [(Ar − As)/Ar] 100 = % siderophore units, where Ar = absorbance of reference (minimal media + CAS assay solution) and As = absorbance of sample.
Pot study
Talc-based bioformulation of test isolate was prepared as per the method described by Goswami et al. (Citation2013b). Briefly, a loopful of bacterial culture was inoculated into nutrient broth and incubated in a rotary shaker at 150 rpm for 48 h at 27 ± 2°C. Talc powder was taken in a metal tray and its pH was adjusted to neutral by the addition of 15 g of CaCO3/kg of talc followed by the addition of 10 g CMC to 1 kg of neutralized talc, mixed well, and autoclaved. Four hundred milliliter of 48-h grown bacterial suspension with colony forming unit of 2.07 × 108/ml was mixed with carrier–cellulose mixture under aseptic conditions. After drying (approximately 35% moisture content), bioformulation was stored in sterile plastic bag. Efficacy of bioformulation was tested on the seeds of hybrid variant Gujarat Junagadh Groundnut-2 (GJG-2) of groundnut. The purpose of using talc-based bioformulation was to allow bacterial cells to adhere seed coat due to the presence of CMC which acts as a binding agent. Second, talc-based bioformulation improves the viability of bacterial cells and shelf life of the biofertilizer. Pot study was carried out in the month of February where the average temperature was below 35°C, night temperature ranging from 14°C to 19°C and average humidity was less than 30%. Soil used for the experiment was sterilized by autoclaving and it was obtained from farm (22°59′N, 72°82′E) located near CHARUSAT University, Anand, Gujarat which can support the growth of groundnut. Pot trials were performed in two sets of soil where first set of soil was unmodified soil (did not contained NaCl supplementation) whereas, second set of soil was modified by supplementation of additional 50 mM NaCl. Electrical conductivity of soil was measured to determine innate soil salinity. Chemical properties and salinity of the soil were determined as per the methods described by Ibekwe et al. (Citation2010). For pH and EC1:5 (electrical conductivity of salt solution at 1:5 soil:water dilution) measurement method given by Walkley and Black (Citation1934), available phosphorus was estimated by the method given by Olsen et al. (Citation1954), available potassium by the method given by Schollenberger and Simon (Citation1945). Estimation of magnesium and sulfate content of the soil samples was performed using an atomic absorption spectrophotometer as described by Chapman and Pratt (Citation1978), all the tests were performed by Gujarat Laboratories, Ahmedabad. Seeds of hybrid variant GJG-2 of groundnut were used for pot experiment. Seeds were surface sterilized by gently shaking in 70% ethanol for 2 min followed by HgCl2 (1%) for 3 min and finally rinsed twice by sterile distilled water before been sowed in pots containing biofertilizer (0.5 g bioformulation per kilograms soil). After 15 days of germination, all the plantlets were uprooted and various vegetative parameters were measured. These parameters were compared with PGPR-treated and non-treated plantlets under control and saline conditions.
Statistical analysis
Analysis of variance (ANOVA) was carried out using triplicate value to identify significant difference in each vegetative parameter between treated and non-treated seeds for both control and saline conditions. Mean values of triplicates were compared at significance levels of 5%, 1%, and 0.1% least square difference (LSD).
Results
Isolation
Out of all the isolates obtained from the rhizosphere of S. fruticosa on nutrient agar amended with 5% NaCl, only single isolate designated 2M4 was able to produce IAA above 35 µg ml−1 (Goswmi et al. Citation2013b) 2M4 isolate produced bright orange pigmented small, convex colonies with entire margin. 2M4 was gram-positive, coccoid, did not produce endospore, and it was aerobic in nature. Further experiments were performed using 2M4 isolate.
Identification of 2M4 isolate using 16S rRNA gene sequence analysis
Phylogenetic analysis was performed to identify 2M4 isolate. Phylogenetic tree was constructed by aligning 16S rRNA gene sequences of species belonging to genus Kocuria with validly published names along with 16S rRNA gene sequence of 2M4. Type strains belonging to Kocuria genus used to construct phylogenetic tree were K. himachalensis, K. rosea, K. polaris, K. aegyptia, K. turfanensis, K. flava, K. palustris, K. kristinae, K. carniphila, K. marina, K. varians, and K. rhizophila. Phylogenetic analysis showed that 16S rRNA gene sequence 2M4 matched best with gene sequence of type strain K. turfanensis HO-9042T under GenBank ID-DQ531634. Based on biochemical characterization and phylogenetic analysis, 2M4 isolate was designated as K. turfanensis strain 2M4 and its 16S rRNA gene sequence was submitted GenBank nucleotide sequence database under the accession number JX679496.
Biochemical characterization of 2M4
Physiological tests to study the growth of 2M4 under various pH, salinity, and temperature showed that the isolate can grow opulently within the pH range of 6–9; however, optimum pH for the growth was 8. Isolate could grow best when 2% (w/v) NaCl was supplemented in nutrient broth and grew up to 6% NaCl supplementation (). 2M4 can survive being dormant up to 10% NaCl supplementation. 2M4 isolate grew optimally at 29 ± 2°C. Growth was not observed above 40°C and below 22°C.
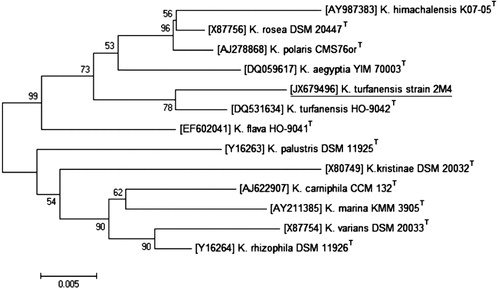
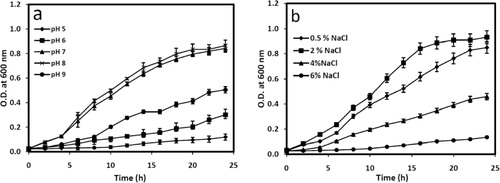
Biochemical tests of 2M4 are shown in , where it was found negative for amylase, gelatinase, urease, oxidase, indole production, methyl red test, and Voges–Proskauer test. 2M4 was found positive for catalase, slow positive for esculin hydrolysis, and nitrate reduction. Substrates used for growth and acid production by 2M4 were xylose, maltose, fructose, dextrose, trehalose, sucrose, L-arabinose, glycerol, inositol, mannitol, rhamnose, melezitose, and citrate. 2M4 could not utilize lactose, galactose, raffinose, melibiose, mannose, inulin, sodium gluconate, salicin, dulcitol, adonitol, arabitol, erythritol, α-methyl-d-glucoside, α-methyl-d-mannoside, xylitol, ortho-Nitrophenyl-β-galactoside (ONPG), and sorbose.
Table 1. Biochemical characterization of 2M4 isolate.
Characterization of IAA produced by 2M4
It was found that IAA produced was L-tryptophan dependent. Production of IAA increases with increase in supplemented L-tryptophan concentration in the medium. Maximum IAA produced by 2M4 isolate was 38 µg ml−1 when the growth medium was supplemented with 600 µg ml−1 of L-tryptophan after 96 h of incubation at 29 ± 2°C under constant agitation. IAA production increased till 96 h of incubation, and there after it reached plateau. However, 4 µg ml−1 of IAA was produced when growth medium was not supplemented with L-tryptophan ().
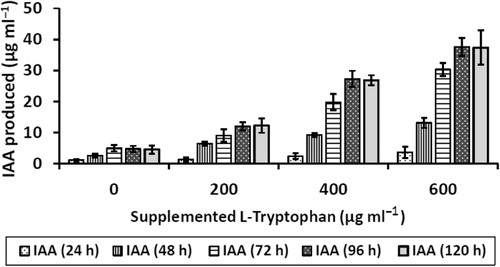
TLC analysis
Development of spots on TLC foils is observed under short and long UV light (). TLC foil contains three standards namely L-tryptophan, IAA, and IBA on first three spots from left, respectively. Spots 4 and 5 were loaded with extracted IAA from 2M4 cell-free supernatant in methanol. R f value of L-tryptophan, IAA, and IBA were 0.29, 0.35, and 0.44, respectively. Spots 4 and 5 has R f value of 0.35 were visible under short and long UV, indicating presence of IAA. TLC analysis is a qualitative test to determine the presence of IAA with greater surety than traditional spectrophotometric method.
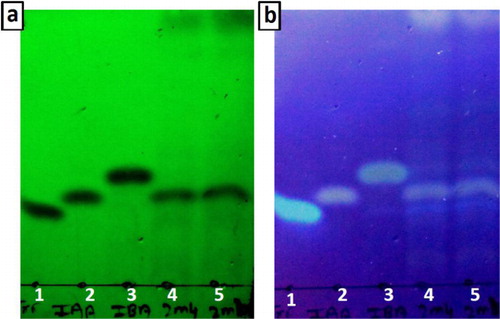
FTIR analysis
Characterization of IAA based on FTIR spectra was performed as per Kamnev et al. (Citation2001). shows the FTIR spectra of extracted IAA from 2M4. Characteristic (N–H) stretching of indole moiety is observed at 3389 cm−1 (N–H) bending, and wagging was observed at 1652 cm−1 and 517 cm−1, respectively. Stretching for (C–N) bond of indole ring was observed at 1247 cm−1. For aromatic ring, (C–H) stretching and banding was observed at 3055 cm−1 and 740 cm−1, respectively. Furthermore, (C = C) banding and in-ring (C–C) stretching was observed at 1558 cm−1 and 1488 cm−1, respectively. Alkyl (–CH2) asymmetric stretching, symmetric stretching, and bending was observed at 2940 cm−1, 2925 cm−1, and 1457 cm−1, respectively. Strong bond of (C = O) in IAA appears at 1701 cm−1. FTIR spectra confirm the presence of IAA.
Phosphate solubilization by 2M4
Phosphate solubilization ability of 2M4 was determined in Pikovskaya's medium along with modifications where glucose was replaced by fructose, sucrose, and mannitol. Tri-calcium phosphate solubilized by 2M4 isolate in presence of different sugars is described . Maximum phosphate solubilized was 12 µg ml−1 in presence of fructose, followed by 9 µg ml−1 in presence of sucrose. However, in presence of glucose and mannitol phosphate solubilized was reduced to 3.5 µg ml−1 and 5.5 µg ml−1, respectively. It was observed that pH of the medium was more acidic in the presence of sugars which solubilized phosphate in greater amounts ().
Ammonia and siderophore production
It was observed that 2M4 isolate produced ammonia in peptone water and produced siderophore in iron-free MM9 minimal medium supplemented with 1% glucose. Maximum production of ammonia and siderophores was 1.8 µmol ml−1 and 53% units, respectively, after 72 h of incubation at 30 ± 2°C with constant agitation. However, ammonia and siderophore produced in the respective growth medium did not increase after 72 h of incubation ().
Pot study
As 2M4 isolate showed multiple traits of PGPR including production of IAA, ammonia, siderophore, and solubilization of phosphate its efficacy as a PGPR was tested in vivo for the test plant of groundnut under non-saline and saline conditions. Chemical properties of soil used for pot study are shown in . Electrical conductivity (EC1:5) of natural unmodified soil was found to be 0.21 mS cm−1 which indicates inherent salinity of soil to be equivalent to 32 mM NaCl and after modification by the addition of 50 mM NaCl to these soil EC1:5 increased to 0.64 mS cm−1 which indicates inherent salinity of soil to be equivalent to 82 mM NaCl. EC is indirect measure to determine the salinity of soil.
Table 2. Analysis of chemical properties of soil.
Comparing the vegetative parameters of control plants (PGPR non-treated) under non-saline and saline soil, it was found that total length of the plant was reduced by 42% and fresh biomass was reduced by 43% for plant grown under saline conditions. Test plant (PGPR treated) grown in absence of NaCl showed significant enhancement in the growth where there was an increase by 18% in total plant length, 26% in root length, 30% in fresh biomass, and 31% in dry biomass. Test plant (PGPR treated) in presence of supplemented 50 mM NaCl showed 17% increase in total length of the plant, root length increased by 19%, fresh biomass by 13%, and dry biomass by 17%. shows the comparative analysis of various vegetative parameters of control and test plants grown in non-saline and saline soil.
Table 3. Effect of 2M4-based bioformulation treatment on the seedling growth of groundnut after 15 days after been sown in pots with and without NaCl supplementation. Values are the mean of triplicates with standard error of mean.
Discussion
Isolation source of K. turfanensis strain 2M4 was S. fruticosa's rhizosphere, a plant belonging to Little desert of Kutch. This is the unique saline desert with salinity up to 12% (w/w) where majority of land in this desert contain 60% clay, unlike other deserts which contains high sand content (Gupta & Ansari Citation2012). Little Rann of Kutch is nominated to be a ‘biosphere reserve’ by UNESCO's Man and Biosphere program (CitationUNESCO World Heritage Centre). Under present study attempt was made to isolate unique rhizobacteria, as this region is least explored for isolating rhizobacteria from the plants adapted to its unique saline ecosystem.
To characterize any rhizobacteria as PGPR it should be attributed to the production of diverse metabolites including IAA, siderophores, ammonia, and activities such as phosphate solubilization (Glick Citation1995; El-Deeb et al. Citation2012). IAA production is the most prominent trait observed by K. turfanensis strain 2M4. IAA is the phytohormone which influences several parameters in plant, namely elongation and cell division, apical dormancy, and differentiation of vascular tissues (Kloepper & Schroth Citation1978). Godinho et al. (Citation2010) have reported that Kocuria rosea isolated from sand dunes of Goa produced IAA 26 µg ml−1, Vicene et al. (Citation2012) reported unnamed Kocuria sp. PWN-228A isolated from Pinewood pinaster produced 35 µg ml−1. This shows that few species of Kocuria has been reported for IAA production. Ahmad et al. (Citation2008) reported that several species of Azotobacter, Pseudomonas, and Bacillus produced IAA up to 20 µg ml−1, 25 µg ml−1, and 10 µg ml−1, respectively. Under present study, it can be construed that K. turfanensis strain 2M4 can produce greater amount IAA when compared with several well-known PGPR.
IAA is a product of L-tryptophan metabolism of microorganisms. So for several PGPR, IAA production is directly dependent on availability of L-tryptophan (Ahmad et al. Citation2008). Under present study, K. turfanensis strain 2M4 showed increased IAA production when concentration of supplemented L-tryptophan was increased. However, IAA is not the only metabolite produced by L-tryptophan metabolism. IBA, indole-3-pyruvate, indole-3-acetamide, tryptamine, and indole-3-acetonitrile can be produced other than IAA by L-tryptophan metabolism (Godinho et al. Citation2010). Furthermore, there have been reports where these L-tryptohan metabolites react with Salkovaski reagent and give color reaction (Glickmann & Dessaux Citation1995; Szkop & Bielawski Citation2013). So to confirm IAA production by K. turfanensis strain 2M4, TLC, and FTIR were performed. Moreover, it has been reported that concentration of IAA produced tends to be greater than other metabolites produced by L-tryptophan metabolism (Glickmann & Dessaux Citation1995; Kang et al. Citation2006).
Other than IAA production K. turfanensis strain 2M4 was also found to solubilize phosphate, produce siderophore, and produce ammonia from peptone. Phosphate and nitrogen are the two main nutrients required by plant. Organic acid secretion by rhizobacteria has been major factor responsible for phosphate solubilization (Buch et al. Citation2010). Rhizobacterial population on the root surface is 10 times higher than in bulk soil. These rhizobacteria utilizes sugars mainly glucose, fructose, sucrose, xylose, and L-arabinose which are present in root exudates metabolize it and produce organic acid which aid in solubilization of phosphate (Archana et al. Citation2012). Surprisingly, biochemical analysis of K. turfanensis strain 2M4 showed that it can utilize all these important sugars to produce organic acid. However, phosphate solubilization assays showed that maximum solubilization of phosphate occurred in presence of fructose and pH of the medium was more acidic as compared to other sugars indicating greater organic acid production in presence of fructose. Ammonia production is another important trait of PGPR where an organism can break down complex nitrogenous materials like peptones and release ammonia in soil. Released ammonia is taken up by plant as a nutrient source. Soils which are rich in nitrogen, there can be an accumulation of ammonia creating alkaline condition of the soil which suppresses the growth of certain fungi (Jha et al. Citation2012). Under present study, ammonia produced by K. turfanensis strain 2M4 is at parity with several PGPR strains including Bacillus, Pseudomonas, Rhizobium, Azotobacter, and Enterobacter (Joseph et al. Citation2007; Jha et al. Citation2012).
Siderophores are the compounds which can chelate iron and cause iron deficiency on growing pathogens in the plant rhizosphere, indirectly suppressing the growth of these pathogens. The importance of siderophore production in species of Pseudomonas is vividly described by Jan et al. (Citation2011), where it induced systemic resistance in watermelon against gummy stem rot while the siderophore-negative mutants failed to induce resistance. Haas and Défago (Citation2005) reported that strains of Pseudomonas act as a strong biocontrol PGPR because of its efficacy to produce siderophores. They further reported that strains of Pseudomonas can suppress Fusarium oxysporum, Gaeumannomyces graminis, Rhizoctonia solani, and so on. Siderophore production trait was observed in K. turfanensis strain 2M4 suggests that this strain may aid in suppressing phytopathogen in the rhizosphere.
Several published reports suggest that if an organism shows in vitro PGPR traits such as IAA production, phosphate solubilization, ammonia production, and siderophore production then it is inevitable for that organism to shows plant growth promotion in vivo (El-Deeb et al. Citation2012, Citation2013; George et al. Citation2013). Deepa et al. (Citation2010) showed that strains of Enterobacter aerogens and Enterobacter cloacae which produced IAA, siderophores, hydrogen cyanide (HCN), and solubilized showed growth promotion in cowpea (Vigna unguiculata L.). Similarly, Jha et al. (Citation2012) showed the growth promotion in Jatropha curcas by multi-trait PGPR with ACC deaminase activity Enterobacter cancerogenus MSA2. Goswami et al. (Citation2013b) reported growth promotion in green gram (Vigna radiata) and chickpea (Cicer arietinum L.) by Pseudomonas spp. strain OG isolated from marine water. Madhaiyan et al. (Citation2006) reported enhancement in growth of groundnut (Arachis hypogaea L.) by Methylobacterium spp. which also showed biocontrol activity against its fungal pathogen Aspergillus niger and Sclerotium rolfsii. Under present study, K. turfanensis strain 2M4 possessing important PGPR traits showed growth promotion in groundnut (Arachis hypogaea L.) under saline soil. Thus, from the evidences obtained K. turfanensis strain 2M4 isolated from saline desert can be considered as a PGPR.
Acknowledgments
Authors are thankful to Department of Science and Technology (DST), New Delhi, India for the fellowship and financial aid. Authors are also thankful to Dr. K. C. Patel R&D Centre, Charotar University of Science and Technology (CHARUSAT) for providing necessary facilities.
References
- Aeron A, Kumar S, Pandey P, Maheshwari DK. 2011. Emerging role of plant growth promoting rhizobacteria in agrobiology. In: Maheshwari DK, editor. Bacteria in agrobiology: crop ecosystems. Berlin: Springer Heidelberg; p. 1–36.
- Ahmad F, Ahmad I, Khan MS. 2008. Screening of free living rhizospheric bacteria for their multiple plant growth promoting activities. Microbiol Res. 263:173–181. 10.1016/j.micres.2006.04.001
- Ali B, Sabri AN, Hasnain S. 2010. Rhizobacterial potential to alter auxin content and growth of Vigna radiata (L.). World J Microbiol Biotechnol. 26:1379–1384. 10.1007/s11274-010-0310-1
- Ali SZ, Sandhya V, Grover M, Linga VR, Bandi V. 2011. Effect of inoculation with a thermotolerant plant growth promoting Pseudomonas putida strain AKMP7 on growth of wheat (Triticum spp.) under heat stress. J Plant Interact. 6:239–246. 10.1080/17429145.2010.545147
- Aloni R, Aloni E, Langhans M, Ulrich CI. 2006. Role of cytokinin and auxin in shaping root architecture: regulating vascular differentiation, lateral root initiation, root apical dominance and root gravitropism. Ann Bot. 97:883–893. 10.1093/aob/mcl027
- Archana G, Buch A, Kumar GN. 2012. Pivotal role of organic acid secretion by rhizobacteria in plant growth promotion. In: Satyanarayana T, Johri BN, editors. Microorganisms in sustainable agriculture and biotechnology. Netherlands: Springer; p. 35–53.
- Bhattacharyya PN, Jha DK. 2012. Plant growth-promoting rhizobacteria (PGPR): emergence in agriculture. World J Microbiol Biotechnol. 28:1327–1350. 10.1007/s11274-011-0979-9
- Brick JM, Bostock RM, Silverstone SE. 1991. Rapid in-situ assay for indole acetic acid production by bacteria immobilized on nitrocellulose membrane. Appl Environ Microbiol. 57:535–538.
- Buch A, Archana G, Naresh Kumar G. 2010. Heterologous expression of phosphoenolpyruvate carboxylase enhances the phosphate solubilizing ability of fluorescent pseudomonads by altering the glucose catabolism to improve biomass yield. Bioresour Technol. 101:679–687. 10.1016/j.biortech.2009.08.075
- Cappucino JC, Sherman N. 1992. Microbiology: a laboratory manual. 3rd ed. New York: Benjamin/Cumming.
- Chapman HD, Pratt PF. 1978. Methods of analysis for soils, plants and waters. Berkeley: Division of Agricultural Sciences, University of California; p. 3034.
- Deepa CK, Dastager SG, Pandey A. 2010. Isolation and characterization of plant growth promoting bacteria from non-rhizospheric soil and their effect on cowpea (Vigna unguiculata (L.) Walp.) seedling growth. World J Microbiol Biotechnol. 26:1233–1240. 10.1007/s11274-009-0293-y
- El-Deeb B, Bazaid S, Gherbawy Y, Elhariry H. 2012. Characterization of endophytic bacteria associated with rose plant (Rosa damascena trigintipeta) during flowering stage and their plant growth promoting traits. J Plant Interact. 7:248–253. 10.1080/17429145.2011.637161
- El-Deeb B, Fayez K, Gherbawy Y. 2013. Isolation and characterization of endophytic bacteria from Plectranthus tenuiflorus medicinal plant in Saudi Arabia desert and their antimicrobial activities. J Plant Interact. 8:56–64. 10.1080/17429145.2012.680077
- Ganeshan G, Kumar MA. 2005. Pseudomonas fluorescens, a potential bacterial antagonist to control plant diseases. J Plant Interact. 1:123–134. 10.1080/17429140600907043
- George P, Gupta A, Gopal M, Thomas L, Thomas GV. 2013. Multifarious beneficial traits and plant growth promoting potential of Serratia marcescens KiSII and Enterobacter sp. RNF 267 isolated from the rhizosphere of coconut palms (Cocos nucifera L.). World J Microbiol Biotechnol. 29:109–117. 10.1007/s11274-012-1163-6
- Glick BR. 1995. The enhancement of plant-growth by freeliving bacteria. Can J Microbiol. 41:109–117. 10.1139/m95-015
- Glickmann E, Dessaux Y. 1995. A critical examination of the specificity of the salkowski reagent for indolic compounds produced by phytopathogenic bacteria. Appl Environ Microbiol. 61:793–796.
- Godinho A, Ramesh R, Bhosle S. 2010. Bacteria from sand dunes of Goa promoting growth in Eggplant. World J Agric Sci. 6:555–564.
- Goswami D, Dhandhukia P, Patel P, Thakker JN. 2013a. Screening of PGPR from saline desert of Kutch: growth promotion in Arachis hypogea by Bacillus licheniformis A2. Microbiol Res. doi:10.1016/j.micres.2013.07.004
- Goswami D, Vaghela H, Parmar S, Dhandhukia P, Thakker J. 2013b. Plant growth promoting potentials of Pseudomonas spp. strain OG isolated from marine water. J Plant Interact. 8:281–290.
- Gupta V, Ansari AA. 2012. Geomorphic portrait of the Little Rann of Kutch. Arab J Geosci. doi: 10.1007/s12517-012-0743-y.
- Haas D, Défago G. 2005. Biological control of soil-borne pathogens by Fluorescent Pseudomonas. Nat Rev Microbiol. 3:307–319. 10.1038/nrmicro1129
- Ibekwe AM, Poss JA, Grattan SR, Grieve CM, Suarez D. 2010. Bacterial diversity in cucumber Cucumis sativus rhizosphere in response to salinity, soil pH, and boron. Soil Biol Biochem. 42:567–575.
- Jan AT, Azam M, Ali A, Haq Q. 2011. Novel approaches of beneficial Pseudomonas in mitigation of plant diseases – an appraisal. J Plant Interact. 6:195–205. 10.1080/17429145.2010.541944
- Jha CK, Patel B, Sarf M. 2012. Stimulation of the growth of Jatropha curcas by the plant growth bacterium Enterobacter cancerogenus MSA2. World J Microbiol Biotechnol. 28:891–899. 10.1007/s11274-011-0886-0
- Joseph B, Patra RR, Lawerence R. 2007. Characterization of plant growth promoting rhizobacteria with chickpea (Cicer arietinum L.). Int J Plant Prod. 1:141–151.
- Kamnev AA, Shchelochkov AG, Perfiliev YD, Tarantilis PA, Polissiou MG. 2001. Spectroscopic investigation of indole-3-acetic acid interaction with iron (III). J Mol Struct. 563:565–572. 10.1016/S0022-2860(00)00911-X
- Kang BR, Yang KY, Cho BH, Han TH, Kim IS, Lee MC, Kim YC. 2006. Production of indole-3-acetic acid in the plant-beneficial strain Pseudomonas chlororaphis O6 is negatively regulated by the global sensor kinase GacS. Curr Microbiol. 52:473–476. 10.1007/s00284-005-0427-x
- Kaur C, Kaur I, Raichand R, Bora TC, Mayilraj S. 2011. Description of a novel actinobacterium Kocuria assamensis sp. nov., isolated from a water sample collected from the river Brahmaputra, Assam, India. A Van Leeuw J Microb. 99:721–726. 10.1007/s10482-010-9547-9
- Kim SB, Nedashkovskaya OI, Mikhailov VV, Han SK, Kim KO, Rhee MS, Bae KS. 2004. Kocuria marina sp. nov., a novel actinobacterium isolated from marine sediment. Int J Syst Evol Microbiol. 54:1617–1620. 10.1099/ijs.0.02742-0
- King JE. 1932. The colorimetric determination of phosphorus. Biochem J. 26:292–297.
- Kloepper JW, Schroth MN. 1978. Plant growth-promoting rhizobacteria on radishes. In: Gilbert-Clarey T, editor. Proceedings of the 4th international conference on plant pathogenic bacteria; p. 879–882.
- Kovacs G, Burghardt J, Pradella S, Schumann P, Stackebrandt E, Marialigeti K. 1999. Kocuria palustris sp. nov. and Kocuria rhizophila sp. nov., isolated from the rhizoplane of the narrow-leaved cattail (Typha angustifolia). Int J Syst Bacteriol. 49:167–173. 10.1099/00207713-49-1-167
- Madhaiyan M, Reddy BS, Anandham R, Senthilkumar M, Poonguzhali S, Sundaram SP, Sa T. 2006. Plant growth–promoting Methylobacterium induces defense responses in groundnut (Arachis hypogaea L.) compared with rot pathogens. Curr Microbiol. 53:270–276. 10.1007/s00284-005-0452-9
- Murray RGE, Doetsch RN, Robinow F. 1994. Determinative and cytological light microscopy. In: Gerhardt P, Murray RGE, Wood WA, Krieg NR, editors. Methods for general and molecular bacteriology. Washington, DC: American Society for Microbiology; p. 21–41.
- Olsen SR, Cole CV, Watanabe FS, Dean LA. 1954. Estimation of available phosphorus in soils by extraction with sodium bicarbonate. US Department of Agriculture Circular No. 939. Washington, DC: US Government Printing Office.
- Payne SM. 1994. Detection, isolation and characterization of siderophores. Method Enzymol. 235:329–344.
- Pikovskaya RI. 1948. Mobilization of phosphorus in soil in connection with the vital activity of some microbial species. Mikrobiologiya. 17:362–370.
- Saharan BS, Nehra V. 2011. Plant growth promoting rhizobacteria: a critical review. Life Sci Med Res. 21:1–30.
- Schollenberger CJ, Simon RH. 1945. Determination of exchange capacity and exchangeable bases in soil-ammonium acetate method. Soil Sci. 59:13–24. 10.1097/00010694-194501000-00004
- Shi W, Takano T, Liu S. 2012. Anditalea andensis gen. nov., sp. nov., an alkaliphilic, halotolerant bacterium isolated from extreme alkali–saline soil. A Van Leeuw J Microb. 102:703–710. 10.1007/s10482-012-9770-7
- Stackebrandt E, Koch C, Gvozdiak O, Schumann P. 1995. Taxonomic dissection of the genus Micrococcus: Kocuria gen. nov., Nesterenkonia gen. nov., Kytococcus gen. nov., Dermacoccus gen. nov., and Micrococcus Cohn 1872 gen. emend. Int J Syst Bacteriol. 45:682–692. 10.1099/00207713-45-4-682
- Szkop M, Bielawski W. 2013. A simple method for simultaneous RP-HPLC determination of indolic compounds related to bacterial biosynthesis of indole-3-acetic acid. A Van Leeuw J Microb. 103:683–691. 10.1007/s10482-012-9838-4
- Tamura K, Dudley J, Nei M, Kumar S. 2007. MEGA4: molecular evolutionary genetics analysis (MEGA) software version 4.0. Mol Biol Evol. 24:1596–1599. 10.1093/molbev/msm092
- UNESCO World Heritage Centre. Nomination entry. http://whc.unesco.org/en/tentativelists/2105/
- Vicene CS, Nascimento F, Espada M, Barbosa P, Mota M, Glick BR, Oliveira S. 2012. Characterization of bacteria associated with pinewood nematode Bursaphelenchus xylophilus. PLoS ONE. 7:e46661. doi: 10.1371/journal.pone.004666
- Walkley A, Black IA. 1934. An examination of the Degtjareff method for determining soil organic matter and a proposed modification of the chromic acid titration method. Soil Sci. 34:29–38. 10.1097/00010694-193401000-00003
- Zhou G, Luo X, Tang Y, Zhang L, Yang Q, Qiu Y, Fang C. 2008. Kocuria flava sp. nov. and Kocuria turfanensis sp. nov., airborne actinobacteria isolated from Xinjiang, China. Int J Syst Evol Microbiol. 58:1304–1307. 10.1099/ijs.0.65323-0