Abstract
Fasciation, a frequent phenomenon in Cactaceae, has been attributed to various causes. The present study reports on phytoplasma-induced fasciation in Euphorbia coerulescens (Euphorbiaceae), Orbea gigantea (Asclepiadaceae), Opuntia cylindrica (Cactaceae), and Senecio stapeliiformis (Asteraceae). DNA was extracted from symptomless and fasciated tissues and amplified by nested PCR using universal primers P1/P7 followed by R16F2n/R16R2 produced amplicons of 1.2 Kb. The nucleotide sequence analyses of the amplicons indicated that fasciated plants were infected by phytoplasma. Phylogenetic analysis placed the cacti fasciation phytoplasmas in 16SrII group. The hormonal content of symptomless and fasciated tissues including indole-3-acetic acid (IAA), kinetin (Kin), N6-benzyladenine (BA), abscisic acid (ABA), and gibberellic acid (GA3) was quantified by high performance liquid chromatography (HPLC). The results indicated that fasciation in O. gigantea was correlated with the accumulation of Kin and IAA increasing five and two times, respectively, as compared to symptomless tissue. However, there was no consistent pattern of hormones in other fasciated species (E. coerulescens, O. cylindrica, and S. stapeliiformis), suggesting that different plant species might have different mechanism to develop fasciation associated with phytoplasma infection.
Keywords:
Introduction
Fasciation, cristation, or abnormal cohesion of organs is a widespread phenomenon that may randomly affect vascular plants both in vitro and in vivo. It occurs in the morphology of plant organs and typically involves broadening of the shoot apical meristem, flattening of the stem, and changes in leaf arrangement (Iliev & Kitin Citation2011). It has been noted that many biotic and abiotic causes lead to the development of fasciated forms including fungal and nematode infections (Stange et al. Citation1996) and mineral deficiency; zinc deficiency is known to cause fasciation (Rance et al. Citation1982), temperature fluctuation (Binggeli Citation1990), chemical mutagens (Soroka & Lyakh Citation2009), and mutations (El-Banna et al. Citation2013). Fasciation is a frequent phenomenon in Cactaceae and in many other succulents’ family like in Euphorbiaceae, Crassulaceae, and Asclepiadaceae. A pure fasciation is also completely different than monstruosity, where axils (areoles) are multiple-activated and the apical meristem loses dominance. All column-like and globular cactican be touched by this anomaly growth form which remains, however, rare. According to origin, fasciations are classified as physiological or genetic, but comparatively little is known on their epigenetic or genetic nature at the molecular level (Iliev & Kitin Citation2011). Previous studies on fasciation of cacti and succulent plants focused mainly on the causative factors of fasciation (Snyder & Weber Citation1966; Boke & Ross Citation1978; Werner Citation1988; Bourque & Pierson Citation1998). Recently, tissue anatomy, protein pattern, and RAPD polymorphisms in fasciated cacti and succulent species indicated that occurrence of fasciation is an epigenetic mutation of tissues (El-Banna et al. Citation2013).
Phytoplasmas are diverse and cell wall-less bacteria associated with several hundreds plant species worldwide (McCoy et al. Citation1989; Lee et al. Citation2000). Symptoms of yellowing, witches’ broom, phyllody, virescence, dwarfing, and irregular growth of leaves are associated with several phytoplasma-caused diseases (Lee et al. Citation2000; Duduk & Bertaccini Citation2006). Phytoplasma has been reported to infect many plants in Egypt such as Catharanthus roseus (Omar et al. Citation2008), eggplants, tomato plants, and squash (Omar & Foissac Citation2012). These infected plants exhibited stunting, leaf yellows, and flower development abnormalities (Omar & Foissac Citation2012). Phytoplasmas can be detected by PCR assays using specific and universal primers designed on the basis of 16S rRNA gene (Deng & Hiruki Citation1990; Ahrens & Seemüller Citation1992; Davis & Lee Citation1993). The extremely conserved 16S rRNA gene is the most widely used for phylogenetic studies of prokaryotes (Martini et al. Citation2007). Phylogenetic analysis of 16S rRNA gene sequence revealed the phylogenetic status of phytoplasma and classified them as the class Mollicutes (Lee et al. Citation2000).
Fasciated mutants are evaluated in plant breeding programs for their ornamental characteristics and are widely cultivated by commercial growers (Krusmann Citation1995; Van Gelderen & Van Hoey Smith Citation1997; Dirr Citation1998). Some fasciations are perceived as real living sculptures and are sought after by horticulturists and collectors. Although, fasciated plants are very attractive when potted, and on the one hand, have ornamental values (Vallicelli Citation2010), on the other hand, they can be phytoplasma-host and cause infection to many food crops (Omar et al. Citation2008). The objective of this study was to detect and categorize the phytoplasma in Euphorbia coerulescens (Euphorbiaceae), Orbea (Stapelia) gigantea (Asclepiadaceae, Leach Citation1975), Opuntia cylindrica (Cactaceae), and Senecio stapeliiformi (Asteraceae), using 16S rRNA gene sequence. We also determined different hormones content in symptomless versus fasciated tissues in order to improve the understanding of the physiological mechanism of fasciation associated with phytoplasma.
Materials and methods
Plant materials
Fasciated and symptomless stem segments from the same plant of E. coerulescens, O. gigantea, O. cylindrica, and S. stapeliiformis were used in this study (). The samples were kept at −80°C for further studies. The plants were taken from a local nursery located in El-Gharbia governorate and grown in 20-cm-diameter clay pots containing a mixture of clay:sand (1:1, v/v). Plants were irrigated once a week during the summer season and once a month during the winter season. The plants were grown under 50% shade net and a compound fertilizer (19:19:19; N:P2O5:K2O) at 1 g L−1 was applied once a month.
DNA extraction
Total DNA of four cacti plants (E. coerulescens, O. gigantea, O. cylindrica, and S. stapeliiformi) having fasciation symptoms and other four asymptomatic were extracted from 1 g of fresh phloem-rich tissue areas of cladodes, which initially were mashed in liquid nitrogen with a mortar and pestle, using i-genomic plant DNA extraction Mini Kit (iNtRON Biotechnology, Inc., Cat. No. 17371) according to the manufacturer's instructions.
Condition, amplification of PCR, and 16S rDNA gene
A 1.8 kb product covering the partial sequence of the 16S rDNA gene, the intergenic spacer region, and the 5′-end region of the 23S rRNA gene regions of the phytoplasma genome was amplified using the universal primer pair P1/P7 (Deng & Hiruki Citation1991; Schneider et al. Citation1995). The first amplification were diluted with sterile distilled water (1:10), and 1 µl of each dilution was used as DNA template for amplification by nested PCR using primer pair R16F2n (5′-GAA ACG ACT GCT AAG ACT GG-3′) and R16R2 (5′-TGA CGG GCG GTG TGT ACA AAC CCC G-3′), which was used for amplification of the 1200 bp fragment of the phytoplasma16S rDNA (Lee et al. Citation1993). PCR was performed for 34 cycles, which were conducted in an automated thermal cycler (C1000TM Thermal Cycler, Bio-RAD).The following parameters were used: the primary denaturation step at 94°C for 3 min, 34 cycles with denaturation at 94°C for 1 min, annealing at 55°C for 1 min, and extension at 72°C for 2 min, followed by a final extension step at 72°C for 7 min. Each PCR mixture (25 µl) was as follows: (1 µl) of 25 ng nucleic acid, 1 µl of each primer (10 pmol), (12.5 µl) of GoTag®Colorless Master Mix (Promega Corporation, USA), and 9.5 µl of Nuclease free water (Promega). Fifteen microliter of all PCR products was analyzed by electrophoresis through a 1% agarose gel, stained with ethidium bromide, and DNA bands were visualized using an ultraviolet transilluminator.
The nested PCR products of four cacti samples were sent for sequencing directly. The sequence was in two directions with primers R16F2 and R16R2 in a sequenciator ABI. ISM 3700 (Applied Biosystems, USA). Raw sequence chromatograms were assembled and edited using GAP4 (Bonfield et al. Citation1995) to correct ambiguous bases or remove low-quality stretches from the termini of the sequences. Similarities to known sequences were detected using the BLASTN algorithms (Altschul et al. Citation1997) against the GenBank database at http://www.ncbi.nlm.nih.gov/blast. Multiple alignments were performed using ClustalW (Thompson et al. Citation1994), and the phylogenetic analyses were conducted with MEGA4 using the neighbor-joining method (Tamura et al. Citation2007). The evolutionary distances were computed using the Maximum Composite Likelihood method (Tamura et al. Citation2004). Partial 16SrDNA gene sequences of four phytoplasma strains obtained from E. coerulescens fasciation phytoplasma, O. gigantean fasciation phytoplasma, O. cylindrica fasciation phytoplasma, and S. stapeliiformis fasciation phytoplasma have been deposited in the EMBL/GenBank/DDBJ databases under accession numbers, HG421070, HG421073, HG421071, and HG4210702, respectively. The 16S rDNA gene sequences of different groups of phytoplasmas used for comparisons were retrieved from GenBank (www.ncbi.nlm.nih.gov).
HPLC hormones estimation
The analysis of hormones was performed by high performance liquid chromatography (HPLC) according to Shindy and Smith (Citation1975) with modifications. In different samples, 5 g fresh weight was ground and soaked in 80% (v/v) aqueous methanol and allowed to extract for 72 h. Then they were filtered through Whatman no. 42 paper. The filter paper and the residue were returned to the flask with a fresh volume of methanol and filtered again. The procedure was repeated once more, and the combined extracts were evaporated to the aqueous phase using a rotary evaporator. The aqueous phase was partitioned into two with equal volume (15 ml each). One partition was adjusted to pH 8.6 with 1% NaOH and extracted thrice with ethyl acetate. The combined ethyl acetate fraction was evaporated to dryness, dissolved in 1 ml of HPLC methanol, and used for quantification of basic hormones such as BA and Kin at 269 nm. The other partition of aqueous phase was adjusted to pH 2.8 with 1% HCl, extracted thrice with ethyl acetate. It was evaporated to dryness, dissolved in 1 ml of HPLC methanol, and used for quantification of acidic hormones such as IAA, ABA, and GA3 at 254 nm. The extract was filtered through a membrane filter (0.45 µm) before injecting (10 µl) into a HPLC system (Waters 746, HPLC 510 pump; Tunable absorbance detector, Waters Associates, Millford, MA, USA) equipped with µ bonda pack C18 300 mm × 3.9 mm column. A mobile phase consisting of methanol HPLC containing 2% of glacial acetic acid was used at a flow rate of 1.0 ml min−1. The concentrations of hormones in sample were computed from the response ratio of target compound and the appropriate internal Sigma standard (IAA – 12273; GA3 – 48880; ABA – A1049; Kin – 48130; BA – 13151).
Statistical analysis
Data were presented as means ± SE. Mean separation was by Duncan's multiple range test (DMRT) at 5% level.
Results and discussion
Morphological features
Morphological differences were clearly visible in fasciated plants compared with symptomless plants (). The symptomless form of E. coerulescens had regular shape, while the cristated form had irregular shapes. The symptomless form of O. cylindrica was regular and has a cylindrical shape, but the fasciated form has spherical and irregular shape. Dense and small spines were found in the fasciated form compared to the symptomless form. Symptomless form of O. gigantea was elongated and has sharp spines, while fasciated forms had curled spherical shape with protrusions. The symptomless form of S. stapeliiformis was elongated and had polygonal shape, while the fasciated form was flat. Fasciation is typically characterized by the development of a flattened organs and plant parts, most commonly on stem and inflorescences. CLAVATA1 mutant of Arabidopsis plants have enlarged apical vegetative and floral meristems, leading to fasciation, altered phylotaxis, and extra floral organs and whorls (Clark et al. Citation1993). Similarly, fasciation in pea is characterized by abnormal enlargement of the stem apical meristem leading to distortions in shoot structure (Sinjushin & Gostimsky Citation2006, Citation2008). Fasciated shoots of Betula pendula induced in vitro as well as Prunus avium and Fraxinus excelsior formed flattened stems with densely arranged lanceolate leaves that were dramatically increased in size (Kitin et al. Citation2005; Mitras et al. Citation2009; Iliev & Kitin Citation2011).
The fasciation is thought to be genetically determined in dicot species (Karakaya et al. Citation2002), while in Lilium henryi, it was associated with the presence of Rotylenchus and ditylenchus nematode species (Stumm-Tegethoff & Linskens Citation1985). In Arabidopsis plant, two genes have been identified to cause fasciation (Reboredo & Silvares Citation2007). Also, fasciation has been experimentally produced by X-rays and chemical mutagens (Reboredo & Silvares Citation2007).
DNA amplification
The PCR amplification using primer pair P1/P7 specific of phytoplasma 16S rRNA from four symptomatic cacti plants (E. coerulescens, O. gigantea, O. cylindrica, and S. stapeliiformi) did not show any bands as the first PCR (data not shown). The same primers failed to produce any PCR products when the DNA from the four symptomless plants was used as control. The nested PCR amplification using primer pair R16F2n/R16R2 generated DNA fragments of 1.2 kb of the four strains. They were observed by electrophoresis and corresponded to the amplification of the 16S rRNA gene according with the DNA positive control. No band was observed in the negative control (data not shown).
Sequences and phylogenetic analysis
Four 16S rDNA (R16F2n/ R16R2 nested PCR) products were sequenced and compared with other 16S rDNA of groups and subgroups reported in GenBank as well as each other. The four strains were given the following names: E. coerulescens fasciation phytoplasma (EcFP), O. gigantea fasciation phytoplasma (OgFP), O. cylindrica fasciation phytoplasma (OcFP), and S. stapeliiformis fasciation phytoplasma (SsFP). The results revealed that the four cacti isolates are not identical even though they shared identities that ranged from 99.4 to 99.9, and they also shared high identities from 99.5 to 99.9 with Papaya yellow crinkle phytoplasma (White et al. Citation1998), eggplant phyllody phytoplasma (Omar & Foissac Citation2012) – which was previously classified as 16SrII-D subgroup – and Peanut witches-broom phytoplasma of 16SrII-A (Gundersen et al. Citation1994), and more than 99.2% with Euphorbia heterophylla phytoplasma (not classified); however, they have identities that ranged from 98.6 to 99.4 with Cactus witches-broom phytoplasma different subgroups according to Cai et al. (Citation2008). Lower identities of 94.1–95.3 were with the Euphorbia dendroides fasciation phytoplasma, Euphorbia linifolia fasciation phytoplasma, and Euphorbia pulcherrima phytoplasma; hence, they belong to different 16Sr subgroups. The phylogenetic analysis of the four strains of phytoplasma showed that they clustered together with 16Sr II subgroup members. E. coerulescens fasciation phytoplasma isolate (EcFP), S. stapeliiformis fasciation phytoplasma isolate (SsFP), and O. cylindrica fasciation phytoplasma isolate (OcFP) were more closely related to Papaya yellow crinkle phytoplasma and Eggplant phyllody phytoplasma, while O. gigantea fasciation phytoplasma isolate (OgFP) stood out of this subclade (). Phytoplasmas belonging to Peanut witches’ broom group (16SrII) and Aster yellows group (16SrI) were detected in Opuntia species showing witches’ broom symptoms (Wei et al. Citation2007; Cai et al. Citation2008). Phytoplasma representing different groups have been reported to be associated with stem fasciation in different plant species in Lilium martagon (16SrI-C) by Bertaccini et al. (Citation2005), while in Petunia spp., the phytoplasma belongs to the AY group (Chung & Huh Citation2008); in Senna surattensis, it belongs to 16SrXII and 16SrV (Wu et al. Citation2012), in Cichorium intybus, it belongs to 16SrV-B (Li et al. Citation2012); in Crotalaria spectabilis, it belongs to the 16SrI-B subgroup (Kumar et al. Citation2010); and in Asparagus officinalis (16SrI-B) in Czech Republic (Fránová & Petrzik Citation2010). The 16SrII-D phytoplasmas are considered to be a serious problem in many crops (chickpea, sesame, mungbean, faba bean, tomato, eggplant, squash, papaya, and pale purple coneflower), in many countries, causing phyllody and virescence symptoms (Akhtar et al. Citation2010; Alfaro-Fernández et al. Citation2012; Omar & Foissac Citation2012; Pallavi et al. Citation2012) and stem fasciation in Linum usitatissimum (16SrII-D) in Pakistan (Akhtar et al. Citation2013).
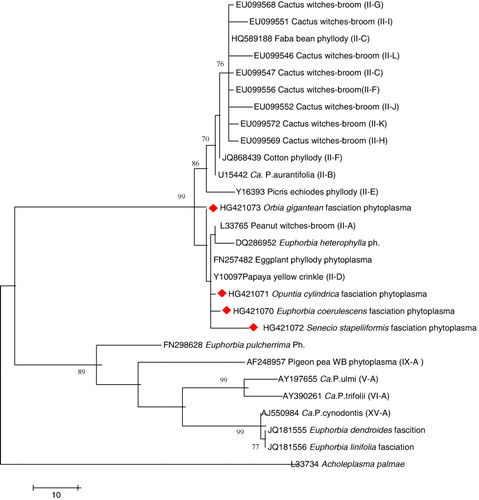
Hormonal content in symptomless versus fasciated tissues
Hormonal content of symptomless and fasciated tissues of E. coerulescens, O. cylindrica, O. gigantea, and S. stapiliiformis is shown in . Fasciated tissues of E. coerulescens had lower content of BA, Kin, IAA, GA3, and ABA than that of symptomless tissues. In O. gigantea, Kin and IAA contents were significantly increased in fasciated tissues as compared to symptomless tissue. However, fasciated tissues had low content of GA3 and ABA as compared to symptomless tissue. BA content showed no significant difference between fasciated and symptomless tissues. Phytoplasma-associated disorders are classified among the auxonic diseases of plants. The phytoplasma symptoms such as growth aberrations indicate perturbations in plant hormonal balance. It has been reported that in phytoplasma-infected micropropagated periwinkle shoots, the level of IAA increased 10 times compared with the control (Pertot et al. Citation1998). The development of green flowers, a symptom of phytoplasma diseases, was correlated with the accumulation of high levels of cytokinin in the floral parts (Davey et al. Citation1981). Nicolaison and Horvath (Citation2008) studied gene expression in E. pulcherrima infected with a branch-inducing phytoplasma. They identified four differentially expressed genes after phytoplasma infection. Three of them (histidine-containing phosphotransmitter, an α-expansin, and a protein with similarity to the gibberellin-regulated GAST-1) showing high homology with genes involved in the biosynthesis or the perception of plant hormones or other growth regulators, such as cytokinin and gibberellins, were shown to be upregulated.
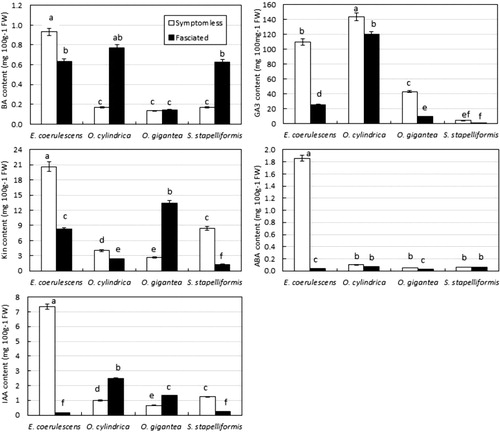
The circumferences of fasciated tissues are several times greater than those of the symptomless ones due to increases in rates of cell growth and cell division (Iliev & Kitin Citation2011). These fasciation characteristics indicate perturbations in plant hormonal balance. There is evidence for a hormonal basis in pathological fasciation caused by Rhodococcus fascians, which has a plasmid-borne virulence gene fas-1 encoding an isopentenyl transferase that produces cytokinin (Crespi et al. Citation1994; Stange et al. Citation1996). Changes in hormone metabolism also correlated with fasciation in hybrid Populus transformed with the Agrobacterium rhizogenes rolC gene, and fasciated tissues had a high level of free cytokinins (Nilsson et al. Citation1996). It has also been shown that plants of Helianthus annuus with fasciated stems (STF) had higher endogenous free IAA levels (Fambrini et al. Citation2006). The observed phenotype and the higher levels of auxin detected suggest that the STF gene is necessary for the proper initiation of primordia and for the establishment of a phyllotactic pattern through control of both shoot apical meristem arrangement and hormonal homeostasis (Fambrini et al. Citation2006). Furthermore, the increased hormonal levels point to a possible hormonal control mechanism of the development of fasciated shoot apical meristem phenotypes. A possible genetic mechanism which operates through a hormonal imbalance restricted to the meristem and its immediate vicinity in fasciated stems has been suggested (Boke & Ross Citation1978). Previous reports demonstrated that exogenously applied cytokinins induced fasciation in Kalanchoe blossfeldiana (Varga et al. Citation1988), B. pendula (Iliev Citation1996), Mammillaria elongata (Papafotiou et al. Citation2001), and P. avium (Kitin et al. Citation2005). Also, it has been reported that fasciation in cotton is conditioned by GA3 and genotype (Nadjimov et al. Citation1999).
Conclusion
The present study confirmed the presence of four new phytoplasma strains in cacti and succulent species. Phytoplasmas have been identified as members of 16SrII group. Our results also indicated that fasciation in O. gigantea was correlated with the accumulation of Kin and IAA which increased five and two times, respectively, as compared to symptomless tissue. However, there was no consistent pattern of hormones in other fasciated species suggesting that different plant species might have different mechanism to develop fasciation associated with phytoplasma infection.
References
- Ahrens U, Seemüller E. 1992. Detection of DNA of plant pathogenic mycoplasmalike organisms by a polymerase chain reaction that amplifies a sequence of the 16S rRNA gene. Phytopathology. 82:828–832. 10.1094/Phyto-82-828
- Akhtar KP, Dickinson M, Hodgetts J, Abbas G, Asghar MJ, Shah TM, Atta BM, Ahmad M, Haq MA. 2010. The phytoplasma disease ‘mung bean phyllody’ is now present in Pakistan. Plant Pathol. 59:399. 10.1111/j.1365-3059.2009.02134.x
- Akhtar KP, Dickinson M, Shah TM, Sarwar N. 2013. Natural occurrence, identification and transmission of the phytoplasma associated with flax phyllody and stem fasciation in Pakistan. Phytoparasitica. 41:383–389. 10.1007/s12600-013-0299-8
- Alfaro-Fernández A, Ali MA, Abdelraheem FM, Saeed EAE, Ambrosio MIFS. 2012. Molecular identification of 16SrII-D subgroup phytoplasmas associated with chickpea and faba bean in Sudan. Eur J Plant Pathol. 133:791–795. 10.1007/s10658-012-9975-7
- Altschul SF, Madden TL, Zhang J, Zhang Z, Miller W, Lipman DJ. 1997. Gapped BLAST (Basic Local Alignment Search Tool) and PSI-BLAST: a new generation of protein database search programs. Nucleic Acids Res. 25:3389–3402. 10.1093/nar/25.17.3389
- Bertaccini A, Franova J, Botti S, Tabanelli D. 2005. Molecular characterization of phytoplasmas in lilies with fasciation in the Czech Republic. FEMS Microbiol Lett. 249:79–85. 10.1016/j.femsle.2005.06.001
- Binggeli P. 1990. Occurrence and causes of fasciation. Cecidology. 5:57–62.
- Boke NH, Ross RG. 1978. Fasciation and dichotomous branching in Echinocereus (Cactaceae). Am J Bot. 65:522–530. 10.2307/2442585
- Bonfield JK, Smith KF, Staden R. 1995. A new DNA sequence assembly program. Nucleic Acids Res. 24:4992–4999. 10.1093/nar/23.24.4992
- Bourque DP, Pierson E. 1998. Crested saguaros: what is the rhyme or reason? Plant Mol Biol Rep. 16:217–218. 10.1023/A:1007500107375
- Cai H, Wei W, Davies RE, Chen H, Zhao Y. 2008. Genetic diversity among phytoplasmas infecting Opuntia species: virtual RFLP analysis identifies new subgroups in the peanut witches,-broom phytoplasma group. Int J Syst Evol Microbiol. 58:1448–1457. 10.1099/ijs.0.65615-0
- Chung BN, Huh KY. 2008. Occurrence of petunia flattened stem caused by phytoplasma. Plant Pathology J. 24:279–282. 10.5423/PPJ.2008.24.3.279
- Clark SE, Running MP, Meyerowitz EM. 1993. CLAVATA1, a regulator of meristem and flower development in arabidopsis. Development. 119:397–418.
- Crespi M, Vereecke D, Temmerman W, Van Montagu M, Desomer J. 1994. The fas operon of Rhodococcus fascians encodes new genes required for efficient fasciation of host plants. J Bacteriol. 176:2492–2501.
- Davey JE, Van Staden J, De Leeuw GTN. 1981. Endogenous cytokinin levels and development of flower virescence in Catharanthus roseus infected with phytoplasmas. Physiol Plant Pathol. 19:193–200. 10.1016/S0048-4059(81)80021-5
- Davis RE, Lee I-M. 1993. Cluster-specific polymerase chain reaction amplification of 16S rDNA sequences for detection and identification of mycoplasmalike organisms. Phytopathology. 83:772–776. 10.1094/Phyto-83-772
- Deng SJ, Hiuki C. 1990. The use of cloned DNA probes for diagnosis of noncultivable plant mollicutes. Proc Jpn Acad. 66B:58–61. 10.2183/pjab.66.58
- Deng S, Hiruki C. 1991. Genetic relatedness between two non-culturable mycoplasmalike organism revealed by nucleic acid hybridization and polymerase chain reaction. Phytopathology. 81:1475–1479. 10.1094/Phyto-81-1475
- Dirr M. 1998. Manual of woody landscape plants: their identification, ornamental characteristics, culture, propagation and uses. 5th ed. Champaign (IL): Stipes Publishing LLC.
- Duduk B, Bertaccini A. 2006. Corn with symptoms of reddening: new host of stolbur phytoplasma. Plant Dis. 90:1313–1319. 10.1094/PD-90-1313
- El-Banna AN, El-Nady MF, Dewir YH, El-Mahrouk ME. 2013. Stem fasciation in cacti and succulent species – tissue anatomy, protein pattern and RAPD polymorphisms. Acta Biol Hung. 64:305–318. 10.1556/ABiol.64.2013.3.4
- Fambrini M, Bonsignori E, Rapparini F, Cionini G, Michelotti V, Bertini D, Baraldi R, Pugliesi C. 2006. Stem fasciated, a recessive mutation in sunflower (Helianthus annuus), alters plant morphology and auxin level. Ann Bot-London. 98:715–730. 10.1093/aob/mcl153
- Fránová J, Petrzik K. 2010. Asparagus officinalis: a new host of ‘Candidatus phytoplasma astries’. J Phytopathol. 158:317–320. 10.1111/j.1439-0434.2009.01615.x
- Gundersen DE, Lee IM, Rehner SA, Davis RE, Kingsbury DT. 1994. Phylogeny of mycoplasmalike organisms (phytoplasmas): a basis for their classification. J Bacteriol. 176:5244–5254.
- Iliev I. 1996. In vitro propagation of Betula pendula Roth. ‘Joungii’. In: Iliev I, Zhelev P, Aleksandrov P, editor. Propagation of ornamental plants. Sofia: Ministry of the Education, Science and Technology Publishing House; p. 44–54.
- Iliev I, Kitin P. 2011. Origin, morphology, and anatomy of fasciation in plants cultured in vivo and in vitro. Plant Growth Regul. 63:115–129. 10.1007/s10725-010-9540-3
- Karakaya HC, Tang YH, Cregan PB, Knap HT. 2002. Molecular mapping of the fasciation mutation in soybean, Glycine max (Leguminosae). Am J Bot. 65: 522–530.
- Kitin P, Iliev I, Scaltsoyiannes A, Nellas C, Rubos A, Funada R. 2005. A comparative histological study between normal and fasciated shoots of Prunus avium generated in vitro. Plant Cell Tiss Org Cult. 82:141–150. 10.1007/s11240-004-8153-9
- Krusmann G. 1995. Manual of cultivated conifers. Portland (OR): H-D Warda, Timber Press.
- Kumar S, Singh V, Lakhanpaul S. 2010. First report of Crotalaria spectabilis fasciation associated with ‘Candidatus phytoplasma asteris’ in India. Plant Dis. 94:1265. 10.1094/PDIS-06-10-0404
- Leach LC. 1975. The lectotype species of Stapelia L. and the reinstatement of Orbea Haw. (Asclepiadaceae). Kirkia. 10:287–291.
- Lee IM, Davis RE, Gundersen-Rindal DE. 2000. Phytoplasma: phytopathogenic mollicutes. Annu Rev Microbiol. 54:221–255. 10.1146/annurev.micro.54.1.221
- Lee IM, Hammond RW, Davis RE, Gundersen DE. 1993. Universal amplification and analysis of pathogen 16S rDNA for classification and identification of mycoplasma like organisms. Phytopathol. 83:834–842. 10.1094/Phyto-83-834
- Li Z-N, Zhang L, Bai Y-B, Liu P, Wu Y-F. 2012. Detection and identification of the elm yellows group phytoplasma associated with Puna chicory flat stem in China. Can J Plant Pathol. 34:34–41. 10.1080/07060661.2012.659679
- Martini M, Lee I-M, Bottner KD, Zhao Y, Botti S, Bertaccini A, Harrison NA, Carraro L, Marcone C, Khan AJ, Osler R. 2007. Ribosomal protein gene-based phylogeny for finer differentiation and classification of phytoplasmas. Int J Syst Evol Microbiol. 57:2037–2051. 10.1099/ijs.0.65013-0
- McCoy RE, Caudwell A, Chang CJ, Chen TA, Chiykowski LN, Cousin MT, Dale JL, de Leeuw GTN, Golino DA, Hackett KJ, et al. 1989. Plant diseases associated with mycoplasmalike organisms. In: Whitcomb RF, Tully JG, editors. The mycoplasmas, vol. 5. New York (NY): Academic Press; p. 545–640.
- Mitras D, Kitin P, Iliev I, Dancheva D, Scaltsoyiannes A, Tsaktsira M, Nellas C, Rohr R. 2009. In vitro propagation of Fraxinus excelsior L. by epicotyls. J Biol Res. 11:37–48.
- Nadjimov UK, Scott IM, Fatkhullaeva GN, Mirakhmedov MS, Nasirullaev BU, Musaev DA. 1999. Conditioning of fasciation by gibberellin and genotype in cotton (Gossypiumhirs utum L.). J Plant Growth Regul. 18:45–48. 10.1007/PL00007045
- Nicolaison M, Horvath DP. 2008. A branch inducing phytoplasma in Euphorbia pulcherrima is associated with changes in expression of host genes. J Phytopathol. 156:403–407. 10.1111/j.1439-0434.2007.01372.x
- Nilsson O, Moritz T, Sundberg B, Sandberg G, Olsson O. 1996. Expression of the Agrobacterium rhizogenes rolC gene in a deciduous forest tree alters growth and development and leads to stem fasciation. Plant Physiol. 112:493–502.
- Omar AF, Emeran AA, Abass JM. 2008. Detection of phytoplasma associated with periwinkle virescence in Egypt. Plant Pathol J. 7:92–97. 10.3923/ppj.2008.92.97
- Omar AF, Foissac X. 2012. Occurrence and incidence of phytoplasmas of the 16SrII-D subgroup on solanaceous and cucurbit crop in Egypt. Eur J Plant Pathol. 133:353–360. 10.1007/s10658-011-9908-x
- Pallavi MS, Ramappa HK, Shankarappa KS, Rangaswamy KT, Wickramaarachchi WART, Maruthi MN. 2012. Detection and molecular characterization of phytoplasma associated with chickpea phyllody disease in south India. Phytoparasitica. 40:279–286. 10.1007/s12600-012-0221-9
- Papafotiou M, Balotis G, Louka P, Chronopoulos J. 2001. In vitro plant regeneration of Mammillaria elongata normal and cristate forms. Plant Cell Tiss Org Cult. 65:163–167. 10.1023/A:1010601328667
- Petrot I, Musetti R, Pressacco L, Osler R. 1998. Changes in indole-3-acetic acid level in micropropagated tissues of Catharanthus roseus L. infected by the agent of the clover phyllody and effect of exogenous auxins on phytoplasma morphology. Cytobios. 95:13–23.
- Rance SJ, Cameron DM, Williams ER. 1982. Correction of crown disorders of Pinus caribaea var. hondurensis by application of zinc. Plant Soil. 65:293–296. 10.1007/BF02374661
- Reboredo F, Silvares C. 2007. Fasciation phenomena and mineral balance in Spartium junceum L. Phyton 47:123–132.
- Schneider B, Seemüller E, Smart CD, Kirkpatrick BC. 1995. Phylogenetic classification of plant pathogenic mycoplasmalike organisms or phytoplasmas. In: Razin S, Tully JG, editors. Molecular and diagnostic procedures in mycoplasmalogy, vol. 1. New York (NY): Academic Press; p. 369–380.
- Shindy WW, Smith OE. 1975. Identification of plant hormones from cotton ovules. Plant Physiol. 55:550–554. 10.1104/pp.55.3.550
- Sinjushin AA, Gostimsky SA. 2006. Fasciation in pea: basic principles of morphogenesis. Russ J Dev Biol. 37:375–381. 10.1134/S1062360406060063
- Sinjushin AA, Gostimsky SA. 2008. Genetic control of fasciation in pea (Pisum sativum L.). Russ J Genet. 44:807–814. 10.1134/S1022795408060100
- Snyder EE, Weber DJ. 1966. Causative factors of cristation in the Cactaceae. Cactus Succulent J. 38:27–32.
- Soroka A, Lyakh V. 2009. Genetic variability in sunflower after mutagen treatment of immature embryos of different ages. Helia. 32:33–46. 10.2298/HEL0951033S
- Stange RR, Jeffares D, Young C, Scott DB, Eason JR, Jameson PE. 1996. PCR amplification of the fas-1 gene for the detection of viriulent strains Rhodococus fascians. Plant Pathol. 45:407–417. 10.1046/j.1365-3059.1996.d01-154.x
- Stumm-Tegethoff BFA, Linskens HF. 1985. Stem fasciation in Lilium henryi caused by nematodes. Acta Bot Neerl. 34:83–93.
- Tamura K, Dudley J, Nei M, Kumar S. 2007. MEGA4: Molecular Evolutionary Genetics Analysis (MEGA) software version 4.0. Mol Biol Evol. 24:1596–1599. 10.1093/molbev/msm092
- Tamura K, Nei M, Kumar S. 2004. Prospects for inferring very large phylogenies by using the neighbor-joining method. Proc Natl Acad Sci USA. 101:11030–11035. 10.1073/pnas.0404206101
- Thompson JD, Higgins DG, Gibson TJ. 1994. CLUSTAL W: improving the sensitivity of progressive multiple sequence alignment through sequence weighting, position-specific gap penalties and weight matrix choice. Nucleic Acids Res. 22:4673–4680. 10.1093/nar/22.22.4673
- Vallicelli V. 2010. Cactuspedia. http://www.cactuspedia.info/. Accessed 20 May 2010.
- Van Gelderen DM, Van Hoey Smith JRP. 1997. Conifers: the illustrated encyclopedia, vols 1 and 2. Portland (OR): Timber Press.
- Varga A, Thoma H, Bruinsma J. 1988. Effects of auxins and cytokinins on epigenetic instability of callus-propagated Kalanchoe blossfeldiana Poelln. Plant Cell Tiss Org Cult. 15:223–231. 10.1007/BF00033646
- Wei W, Cai H, Chen H, Davis RE, Zhao Y. 2007. First report of a natural infection of Opuntia sp. by a ‘Candidatus Phytoplasma asteris’-related phytoplasma in China. Plant Dis. 4:461. 10.1094/PDIS-91-4-0461C
- Werner R. 1988. Unusual growth in succulent Euphorbias. ‘Fasciation & Dichotomus Branching’. Euphorbia J, Strawberry Press, Mill, CA. 5:7–17.
- White DT, Blackall LL, Scott PT, Walsh KB. 1998. Phylogenetic positions of phytoplasmas associated with dieback, yellow crinkle and mosaic diseases of papaya, and their proposed inclusion in ‘Candidatus Phytoplasma australiense ‘and a new taxon’, Candidatus Phytoplasma australasia’. Int J Syst Bacteriol. 48:941–951. 10.1099/00207713-48-3-941
- Wu W, Cai H, Wei W, Davis RE, Lee I-M, Chen H, Zhao Y. 2012. Identification of two new phylogenetically distant phytoplasmas from Senna surattensis plants exhibiting stem fasciation and shoot proliferation symptoms. Ann Appl Biol. 160:25–34. 10.1111/j.1744-7348.2011.00517.x