Abstract
It has been reported that nitrate availability was able to modify the detrimental effects induced by excess ultraviolet-B (UV-B) radiation on plants. In this study, the medical plant Catharanthus roseus was subjected to UV-B stress and altered levels of nitrate nutrition to investigate their influence in a sole or combined way on growth and alkaloid productions. Our results showed that the UV-B stress obviously inhibited growth and led to damages of oxidative stress and lipid peroxidation. The simultaneous supply of higher nitrate nutrition could largely alleviate the inhibitory effects and damage symptom under the UV-B stress in C. roseus. Meanwhile, the UV-B-absorbing compounds as well as three alkaloids, vinblastine, vindoline, and catharanthine, were observed to have a remarkable elevation. These compounds were considered to serve as protectants of UV-B radiation. It was concluded that an increased nitrogen (N) supply could not only alleviate the inhibitory effect of UV-B stress on plant growth, but also enhance the accumulation of pharmaceutically used alkaloid in these plants. We proposed that the enrichment of N nutrition might provide more N source for alkaloid synthesis induced by UV-B radiation, eventually resulting in an increase of alkaloids accumulation.
Introduction
Elevated ultraviolet-B (UV-B) radiation produces a wide diversity of morphological and physiological damages to plant growth and metabolism, photosynthetic performance, and chloroplast membrane components (Ali et al. Citation2010; Lidon & Ramalho Citation2011; Pandey et al. Citation2011; Fukaya et al. Citation2013; Singh et al. Citation2013). UV-B-absorbing compounds such as flavonoids are considered as a critical barrier for protecting plants from UV-B stress (Nunez-Olivera et al. Citation2009; Fabon et al. Citation2012). Recent studies have suggested that UV-B-induced flavonoid accumulation in plant leaves is dependent upon nitrate reductase-mediated nitric oxide signaling (Zhang et al. Citation2011). Increasing reports showed that nitrate availability was able to modify plant response to UV-B, and the analysis of individual UV-absorbing compounds represented a useful complement to the global estimation of UV protection (Lau et al. Citation2006; Nunez-Olivera et al. Citation2009).
Catharanthus roseus, an endemic plant of Madagascar, produces a large amount of secondary metabolites of great pharmacological interest, for example, vinblastine (St-Pierre et al. Citation1999; Tang et al. Citation2009; Han et al. Citation2013). Under natural conditions, environmental stresses stimulate the production of secondary metabolites including terpenoid indole alkaloids (TIAs) that serve putative protective functions (Yu & De Luca Citation2013). Such metabolites absorb UV-B light and serve putatively to protect the plant from harmful radiation (Zhang & Bjorn Citation2009). Previous studies have shown that near-UV light in the UV-B spectrum stimulated the production of monomeric and dimeric alkaloids in C. roseus shoots (Hirata et al. Citation1991).
Nitrogen (N) is essential for plant growth and is taken up by plants principally in the forms of nitrate (Ali et al. Citation2010; Guo et al. Citation2012). Plant growth has been illustrated to be favored in nitrate-replete solution, where plants display stimulated shoot growth, delayed senescence, and increased tolerance to environmental stress (Morrison et al. Citation2010). Recent reports found that nitrate was one of the important nutritional factors affecting the accumulation of alkaloids in C. roseus (Tang et al. Citation2011). The hypothesis is that supplement with replete N nutrition to plants will provide adequate N-containing compounds for alkaloid synthesis (St-Pierre et al. Citation1999). However, excessive N deposition will decrease the biomass, damage the leaf epidermis, and reduce the resistance of leaf epidermis, causing the leaves to change color and fall off (Smith & Stitt Citation2007). Hence, investigation on how nitrate interacts with other environmental cues to regulate alkaloid production in plants is required.
There are a variety of environmental factors in the natural environment which simultaneously combine to affect plant growth (Smith & Stitt Citation2007). Previous studies have showed that enhanced UV-B radiation on N metabolism in plants has important implications (Shukla & Kakkar Citation2002; Morrison et al. Citation2010). Plants are more sensitive to UV radiation under decreased N supply, and it is considered that N limits the ability to repair the damage caused by UV-B radiation (Lau et al. Citation2006). However, so far the specific mechanism of N involvement in response to UV-B stress is unclear. Thus, in this study we used UV-B radiation and nitrate as dual factors to study the variations of phenotype and alkaloids of C. roseus. The potential impact of UV-B stress on plant under different N supplies was assessed.
Materials and methods
Plant materials and growth conditions
C. roseus seeds were germinated in pots of sand (particle size 0.6–1.5 mm diameter), and kept moist by covering with a transparent sheet until germination. After germination, the seedlings were irrigated with half-strength Hoagland solution (pH 6.0), adjusted by addition of HCl/KOH.
Treatment experiments
The seedlings were randomized to the treatments when four pairs of leaves had appeared (about one month). Each group treatment consisted of 50 seedlings. The treatments were: half-strength Hoagland nutrient solution (CK); 50% of additional nitrate N in CK solution (CK + N); approximately double UV-B radiation intensity of growth chamber in CK solution (CK + UV); double UV-B radiation of growth chamber intensity and about 50% of additional nitrate N in CK solution (CK + UV + N).
The experiment was conducted in growth chambers (S10H, CONVIRON, Canada) set as 12/12 h light/night regime at an irradiance of 450 µmol m−2 s−1 at 28°C during the day and 26°C at night and 65% relative humidity.
The increase of UV-B radiation was realized using four UV-B tubes (305 nm, 40 w, Beijing Electric Light Source Institute) on both sides, and the light intensity of the UV-B radiation was 10.8 µW cm−2 nm−1. The UV-B fluorescent tubes were wrapped in cellulose acetate foil to eliminate shorter wavelengths in the UV range. The cellulose acetate foil was replaced twice each week with a fresh piece that had been pre-exposed for 12 h to avoid degradation effects. The N supply was increased by improving the concentrations of calcium nitrate and potassium nitrate in the Hoagland nutrient.
Phenotypic assay and biomass measurement
Individual plants were harvested for morphological and physiological determinations 7 or 15 d after the treatments were applied. Morphological measurements, height, root length, and leaf angle were also measured on these plants. Then, the whole plant was dried at 80°C for 48 h to determine the dry weight.
Determination of alkaloid content
Leaf samples were taken after 7 and 15 days, and dry leaf powder (0.3 g) was dissolved in 10 mL absolute methanol (analytical grade) for the extraction of vinblastine, vindoline, and catharanthine. Low-frequency ultrasonication (250 W, 40 kHz) was used to extract the alkaloids for 20 min. The methanol extract was centrifuged at 8000 rpm for 10 min, concentrated to 1 mL, and analyzed by high performance liquid chromatography (HPLC) (Jasco, VG, England) equipped with a Waters ODS C18 reversed-phase column (250 × 4.6 mm, 5 µm) and a photodiode-array detector at 220 nm. Sample injection volume was 10 µL at a flow rate of 1.5 mL min−1.
Determination of H2O2
Hydrogen peroxide (H2O2) concentration was determined according to Tang et al. (Citation2009), using N-ethyl-N-(2-hydroxy-3-sulfopropyl)-3-methylaniline sodium dihydrate (TOOS) as a staining solution. About 150 µL staining solution and 50 µL sample solution were added to each well of a 96-well plate and incubated at room temperature for 20 min. The absorbance was read at 492 nm using a Stat Fax 3200 microplate reader and H2O2 was determined from a calibration curve (Tang et al. Citation2009).
Malondialdehyde content
About 0.5 g leaf powder ground in pre-cooled mortar was dissolved in 5 mL of cold 10% trichloroacetic acid (TCA) to extract the malondialdehyde (MDA). Extract was centrifuged at 4000 rpm for 10 min, then the mixture containing 2 mL of 0.5% TBA and supernatant was boiled for 15–30 min in a boiling water bath and then quickly cooled in cold water. Finally, the absorbance of the supernatant centrifuged for 15 min at 8000 rpm was measured at 532, 450, and 600 nm.
The formula was:
Enzyme assays
For the detection of antioxidant enzyme activities, 0.5 g leaf tissue was homogenized at 0–4°C in 5 mL Tris HCl buffer (50 mM, pH 7.0) containing 1 mM ethylene diamine tetraacetic acid (EDTA)-Na2 and 1% w/v soluble polyvinylpyrrolidone. Homogenates were centrifuged (10,000 × g, 30 min, 4°C) and the total soluble enzyme activities were measured in the supernatant at 25°C, by a UV spectrophotometer (UV-160A, Shimadzu Corporation, Japan). Protein concentration in the enzyme extracts was determined according to Lowry et al. (Citation1951) using defatted bovine serum albumin (BSA) as a standard.
Superoxide dismutase (SOD, E.C.1.15.1.1) activity was determined by the modified method of Beauchamp and Fridovich (Citation1971). The reaction mixture contained 50 mM sodium phosphate buffer (pH 7.8), 130 mM methionine, 0.75 mM nitroblue tetrazolium (NBT), 1 mM EDTA, 0.02 mM riboflavin, and enzyme extract containing 50 µg protein (100 µL). The activity of SOD was tested at 560 nm, using a UV spectrophotometer. One unit of SOD was defined as the amount of enzyme that produced 50% inhibition of NBT reduction under assay conditions.
Activity of peroxidase (POD, E.C.1.11.1.7) was assayed according to Rathmell and Sequeira's (Citation1974) study. The formation of the conjugate product of Guaiacol was measured at 460 nm. The reaction mixture contained 1.8 mL acetic acid buffer (100 mM, pH 5.4), 1 mL 0.25% Guaiacol, 100 µL 0.75% H2O2, and 100 µL of leaf extract. The increase within 3 min was measured as the conjugate was formed using an extinction coefficient of 26.6 mM−1 cm−1 for the conjugate.
Catalase (CAT, E.C. 1.11.1.6) activity was determined as described by Patterson et al. The decomposition of H2O2 was followed at 240 nm in a quartz cuvette (extinction coefficient of H2O2 was 0.04 mM−1 cm−1). The reaction mix consisted of 2.7 mL 50 mM sodium phosphate buffer (pH 7.0), 100 µL 300 mM H2O2 solution, and 200 µL plant extract equivalent to 25 µg protein in a final volume of 3 mL (Patterson et al. Citation1984). The changing curve of the reaction within 3 min was measured.
Ascorbate peroxidase (APX, EC 1.11.1.11) activity was determined in the reaction mixture containing 2.2 mL 50 mM potassium phosphate buffer (pH 7.0), 0.3 mL 5 mM ascorbate (ASC), 0.3 mL 1 mM H2O2, and 0.2 mL enzyme extract, and measured the changes of A290 (extinction coefficient 2.8 mM−1 cm−1) for 1 min. The reaction was started by the enzyme extract. Correction was done for the low, nonenzymatic oxidation of ASC by H2O2.
Determination of UV-B-absorbing compounds
UV-B-absorbing compounds were extracted according to the acidified methanol protocol (Fabon et al. Citation2012). Application of fiber-optic measurements and the concentration of UV-B-absorbing compounds were evaluated on whole-leaf extracts with spectroscopy. Two 1-cm2 area samples were cut from a leaf adjacent to the leaf used for microprobe measurement. One sample was used for analyzing UV-B-absorbing compound concentration, while the other was used for measuring dry weight (60°C for 48 h) to allow concentrations to be expressed. Concentrations of these compounds were estimated by measuring absorbance at 305 nm with a UV/visible spectrophotometer (UV 160U, Shimadzu).
Statistical analysis
Results were subjected to analysis of variance (ANOVA) to determine the significant differences between treatments. When ANOVA was performed, Duncan's honestly significant difference (HSD) post hoc tests were conducted to determine the differences between the individual treatments (SPSS 17.0, SPSS Inc., USA). SPSS was also used to calculate Pearson's correlation coefficients.
Results
Morphology characteristics and biomass allocation under UV-B radiation and the effect of N nutrition
The changes of the phenotype and biomass of C. roseus seedling were investigated after 15 d of four kinds of treatments. As shown in , there was a significant decrease in plant height, leaf inclined angle (LIA), and biomass in plants under CK + UV condition compared with those treated with CK or CK + N. However, the treatment of CK + UV + N efficiently recovered this reduction. No significant alteration was detected in leaf number among four treatments. These results indicated that replete N used in this research largely alleviated the harmful effect of UV-B radiation on plant growth.
Table 1. The effects of enhanced UV-B radiation and nitrogen supply on growth characteristics of C. roseus seedlings.
H2O2 and MDA
Seedlings exposed to excessive UV-B for seven days had elevated leaf H2O2 content, which was significantly increased by 30.8% compared with the treatment of CK or CK + N (). Further enhancement of N supply significantly reduced (p < 0.05) the content of H2O2 compared to enhanced UV-B radiation alone, but still higher than that in CK or CK + N plants. In addition, plants under CK + N condition had a relatively lower H2O2 content, indicating that the sufficient N source was helpful for plant to control the redox station.
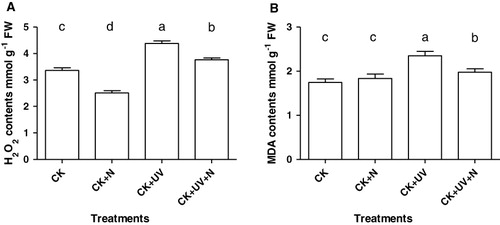
As a reflection of plant senescence and stress damage, the change of MDA content was consistent with H2O2 production during the experiment. There was a significant increase in MDA content when the plants were exposed to UV-B stress for seven days, while the content of leaf MDA was obviously reduced in the CK + UV + N-treated plants due to the presence of increased nitrate nutrition.
Antioxidant activity
Activities of four enzymes that were measured (SOD, POD, CAT, and APX) displayed the same pattern of changes in response to the treatments (). In reponse to UV-B, antioxidant enzyme activities greatly decreased (p < 0.05) in leaves after seven days, probably because excessive UV-B radiation had seriously damaged the plant's antioxidant system. After the application of exogenous N together with UV-B, enzyme activities were increased almost twofold compared to UV-B alone and were even a little higher than the nonstressed control. The addition of exogenous N stimulated antioxidant enzyme activities to resist oxidation, reducing the destructive effects of UV radiation on the plant's antioxidant system.
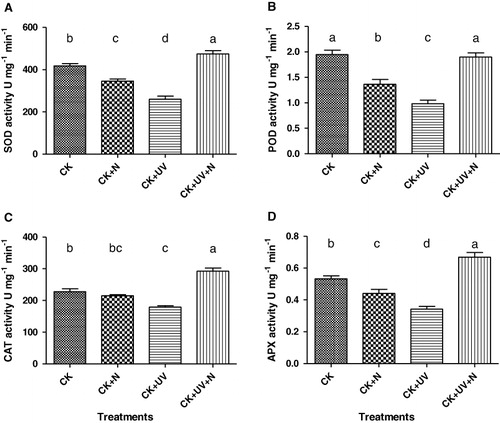
UV-B-absorbing compounds
After 15 d of treatment, UV-B-absorbing compounds in C. roseus leaf increased significantly (p < 0.05) compared with the control (p < 0.05, ). Increased N supply further significantly enhanced (p < 0.05) the accumulation of UV-B-absorbing compounds under UV-B stress. This is another example of how exogenous N could improve the plant's defense against UV radiation performance.
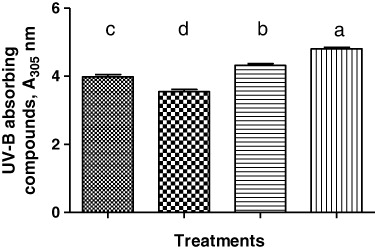
Alkaloid contents
The three alkaloids' (vindoline, catharanthine, and vinblastine) contents in the leaf of C. roseus were measured after enhanced UV-B radiation and N supply treatments for 7 and 15 d (). Vinblastine content was detected by HPLC as previously described. As shown in the control treatment, vinblastine content decreased with the growth of C. roseus. UV-B radiation alone or combined with N supply both increased vinblastine content. For UV-B-exposed plants, vinblastine content was largely improved compared with the nonstressed control after seven days of treatment. But when the seedlings were exposed to UV-B radiation combined with N after 15 d, the accumulation level reached three times that of control plants. In contrast to vinblastine, vindoline and catharanthine contents increased with plant maturation. UV-B radiation increased contents of both compounds. This effect was further enhanced when compared with N treatment. It was interesting to observe that plants under CK + N condition accumulated slightly lower alkaloids during the experimental process.
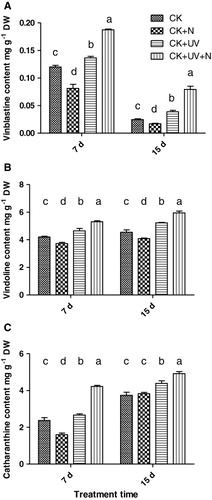
Correlation analysis
Correlation analysis for the contents of the three alkaloids was performed to determine the relationships among them (). Vinblastine and catharanthine were significantly positively correlated (p < 0.01) after seven days. There was also a positive correlation (p < 0.05) between vindoline and vinblastine in the whole experiment. After 7 and 15 d, vindoline and catharanthine were strongly correlated, respectively. In short, the three alkaloids were strongly interrelated probably because vindoline and catharanthine are precursors of vinblastine.
Table 2. Pearson's correlation matrix of three indole alkaloids in the leaves of C. roseus.
Discussion and conclusion
The medical plant C. roseus originates from a tropical region and now is widely cultivated in southern China for the production of some alkaloids such as vinblastine (Tang et al. Citation2009). Under field conditions, exposure to substantial UV-B radiation and nutrition deficiency are two commonly depressing factors for growth of this plant practically. The interactive effects of these two factors on C. roseus still remain to be investigated. Therefore, we compared the sole or combined influence of UV-B and nitrate supply on growth and alkaloid accumulation in this study.
It has been normally observed that exposure to excess UV-B radiation can reduce plant height, leaf area, biomass accumulation, and allocation of carbon resource (Agrawal et al. Citation2006; Ren et al. Citation2010; Singh et al. Citation2013). Among the morphological responses of plants to UV-B stress, LIA and root/shoot ration (RSR) are regarded as typical responses (Fukaya et al. Citation2013). The reduced LIA and RSR in UV-stressed C. roseus seedlings were obviously observed in our study. Moreover, this altered the response induced by UV-B stress could be alleviated by increased nitrate supply. It was proposed that increased nitrate was able to help plants allocate more energy to the aerial parts to withstand or repair radiation damage (Morrison et al. Citation2010).
Plants under the conditions of excess UV-B radiation produce serious oxidative stress (Lidon & Ramalho Citation2011; Singh et al. Citation2012; Singh et al. Citation2013). Our research supported this view because the contents of H2O2 and MDA in the UV-stressed plants increased significantly compared to that in CK or CK + N. But in the combined treatment of UV-B radiation and N supply, H2O2 and MDA contents decreased significantly. This result indicated that the adequate N source supplied could reduce the oxidative sensitivity of C. roseus to UV-B radiation. In this condition, the promoted activities of antioxidant enzymes assayed here might allow for the reduction of oxidative damages (Correia et al. Citation2012).
Under UV-B stress condition, the availability of N source is critical for plants to accumulate UV-B-absorbing compounds to decrease photo inhibition induced by UV-B radiation (Fabon et al. Citation2012). It is reported that UV-B-induced flavonoid accumulation in plant leaves is dependent upon nitrate reductase-mediated nitric oxide signaling (An et al. Citation2005; Zhang et al. Citation2011), confirming the pivotal role of nitrate in this process.
Alkaloid metabolism and accumulation in C. roseus are reported to actively respond to adverse environment conditions such as potassium nutrition (Chang et al. Citation2014), N nutrition (Guo et al. Citation2012), and salinity (Tang et al. Citation2011). Researchers found that UV-B-induced signaling events led to the enhanced production of alkaloid in C. roseus cell suspension cultures (Ramani & Chelliah Citation2007). The UV-B light-responsive regions identified in the promoter of the tryptophan decarboxylase gene provided molecular mechanism for this signaling process (Ouwerkerk et al. Citation1999). These findings raise the question that what is the definitive role of alkaloid in plant response to UV-B radiation or other environmental factors.
The alkaloid, such as vinblastine or catharanthine, may act as a UV-B-absorbing compound and free radical scavenger to reduce oxidative stress of the UV-B radiation (Hirata et al. Citation1991; St-Pierre et al. Citation1999; Ramani & Chelliah Citation2007). The recent report supported this hypothesis because they found that catharanthine could be transported via a unique catharanthine transporter to the leaf surface to cope with environmental changes (Yu & De Luca Citation2013). The extra nitrate included in the nutrition solution further promoted alkaloid contents in UV-stressed plants in our study and this might be due to the increased N-containing compounds for biosynthesis of alkaloids under high nitrate condition.
Acknowledgments
We sincerely thank Matthew Paul from Rothamsted Research for his constructive and critical comments on this manuscript. This study was financially supported by the Fundamental Research Funds for the Central Universities [DL12BA02 and DL11CA04] and the Natural Science Foundation of Heilongjiang province [C201117].
References
- Agrawal SB, Rathore D, Singh A. 2006. Combined effects of enhanced ultraviolet-B radiation and mineral nutrients on growth, biomass accumulation and yield characteristics of two cultivars of Vigna radiata L. J Environ Biol. 27:55–60.
- Ali B, Hayat S, Hayat Q, Ahmad A. 2010. Cobalt stress affects nitrogen metabolism, photosynthesis and antioxidant system in chickpea (Cicer arietinum L.). J Plant Interact. 5:223–231. 10.1080/17429140903370584
- An L, Liu Y, Zhang M, Chen T, Wang X. 2005. Effects of nitric oxide on growth of maize seedling leaves in the presence or absence of ultraviolet-B radiation. J Plant Physiol. 162:317–326. 10.1016/j.jplph.2004.07.004
- Beauchamp C, Fridovich I. 1971. Superoxide dismutase: improved assays and an assay applicable to acrylamide gels. Anal Biochem. 44:276–287. 10.1016/0003-2697%2871%2990370-8
- Chang B-W, Cong W-W, Chen Q, Zu Y-G, Tang Z-H. 2014. The influence of different forms and concentrations of potassium nutrition on growth and alkaloid metabolism in Catharanthus roseus seedlings. J Plant Interact. 9:370–377. 10.1080/17429145.2013.838312
- Correia CM, Coutinho JF, Bacelar EA, Goncalves BM, Bjorn LO, Moutinho Pereira J. 2012. Ultraviolet-B radiation and nitrogen affect nutrient concentrations and the amount of nutrients acquired by above-ground organs of maize. Sci World J. 2012:608954 10.1100/2012/608954
- Fabon G, Monforte L, Tomas-Las-Heras R, Nunez-Olivera E, Martinez-Abaigar J. 2012. Dynamic response of UV-absorbing compounds, quantum yield and the xanthophyll cycle to diel changes in UV-B and photosynthetic radiations in an aquatic liverwort. J Plant Physiol. 169:20–26. 10.1016/j.jplph.2011.08.010
- Fukaya M, Uesugi R, Ohashi H, Sakai Y, Sudo M, Kasai A, Kishimoto H, Osakabe M. 2013. Tolerance to solar ultraviolet-B radiation in the citrus red mite, an upper surface user of host plant leaves. Photochem Photobiol. 89:424–431. 10.1111/php.12001
- Guo XR, Zu YG, Tang ZH. 2012. Physiological responses of Catharanthus roseus to different nitrogen forms. Acta Physiologiae Plantarum. 34:589–598. 10.1007/s11738-011-0859-9
- Han M, Heppel SC, Su T, Bogs J, Zu Y, An Z, Rausch T. 2013. Enzyme inhibitor studies reveal complex control of methyl-D-erythritol 4-phosphate (MEP) pathway enzyme expression in Catharanthus roseus. PLoS One. 8:e62467 10.1371/journal.pone.0062467
- Hirata K, Horiuchi M, Ando T, Asada M, Miyamoto K, Miura Y. 1991. Effect of near-ultraviolet light on Alkaloid production in multiple shoot cultures of Catharanthus roseus. Planta Med. 57:499–500. 10.1055/s-2006-960184
- Lau TSL, Eno E, Goldstein G, Smith C, Christopher DA. 2006. Ambient levels of UV-B in Hawaii combined with nutrient deficiency decrease photosynthesis in near-isogenic maize lines varying in leaf flavonoids: flavonoids decrease photoinhibition in plants exposed to UV-B. Photosynthetica. 44:394–403. 10.1007/s11099-006-0042-5
- Lidon FC, Ramalho JC. 2011. Impact of UV-B irradiation on photosynthetic performance and chloroplast membrane components in Oryza sativa L. J Photochem Photobiol B. 104:457–466. 10.1016/j.jphotobiol.2011.05.004
- Lowry OH, Rosebrough NJ, Farr AL, Randall RJ. 1951. Protein measurement with the Folin phenol reagent. J Biol Chem. 193:265–275.
- Morrison KM, Simmons SJ, Stapleton AE. 2010. Loci controlling nitrate reductase activity in maize: ultraviolet-B signaling in aerial tissues increases nitrate reductase activity in leaf and root when responsive alleles are present. Physiol Plant. 140:334–341. 10.1111/j.1399-3054.2010.01406.x
- Nunez-Olivera E, Otero S, Tomas R, Martinez-Abaigar J. 2009. Seasonal variations in UV-absorbing compounds and physiological characteristics in the aquatic liverwort Jungermannia exsertifolia subsp. cordifolia over a 3-year period. Physiol Plant. 136:73–85. 10.1111/j.1399-3054.2009.01215.x
- Ouwerkerk PB, Hallard D, Verpoorte R, Memelink J. 1999. Identification of UV-B light-responsive regions in the promoter of the tryptophan decarboxylase gene from Catharanthus roseus. Plant Mol Biol. 41:491–503. 10.1023/A:1006321100550
- Pandey M, Srivastava AK, Suprasanna P, D'Souza SF. 2011. Thiourea mediates alleviation of UV-B stress-induced damage in the Indian mustard (Brassica juncea L.). J Plant Interact 7:143–150. 10.1080/17429145.2011.561934
- Patterson BD, Payne LA, Chen YZ, Graham D. 1984. An inhibitor of catalase induced by cold in chilling-sensitive plants. Plant Physiol. 76:1014–1018. 10.1104/pp.76.4.1014
- Ramani S, Chelliah J. 2007. UV-B-induced signaling events leading to enhanced-production of Catharanthine in Catharanthus roseus cell suspension cultures. BMC Plant Biol. 7:61. 10.1186/1471-2229-7-61
- Rathmell WG, Sequeira L. 1974. Soluble peroxidase in fluid from the intercellular spaces of tobacco leaves. Plant Physiol. 53:317–318. 10.1104/pp.53.2.317
- Ren J, Duan B, Zhang X, Korpelainen H, Li C. 2010. Differences in growth and physiological traits of two poplars originating from different altitudes as affected by UV-B radiation and nutrient availability. Physiol Plant. 138:278–288. 10.1111/j.1399-3054.2009.01328.x
- Shukla UC, Kakkar P. 2002. Effect of dual stress of ultraviolet-B radiation and cadmium on nutrient uptake of wheat seedlings. Comm Soil Sci Plant Anal. 33:1737–1749. 10.1081/CSS-120004819
- Singh S, Agrawal M, Agrawal SB. 2013. Differential sensitivity of spinach and Amaranthus to enhanced UV-B at varying soil nutrient levels: association with gas exchange, UV-B-absorbing compounds and membrane damage. Photosynth Res. 115:123–138. 10.1007/s11120-013-9841-2
- Singh VP, Srivastava PK, Prasad SM. 2012. Differential effect of UV-B radiation on growth, oxidative stress and ascorbate–glutathione cycle in two cyanobacteria under copper toxicity. Plant Physiol Biochem. 61:61–70. 10.1016/j.plaphy.2012.09.005
- Smith AM, Stitt M. 2007. Coordination of carbon supply and plant growth. Plant Cell Environ. 30:1126–1149. 10.1111/j.1365-3040.2007.01708.x
- St-Pierre B, Vazquez-Flota FA, De Luca V. 1999. Multicellular compartmentation of Catharanthus roseus alkaloid biosynthesis predicts intercellular translocation of a pathway intermediate. Plant Cell. 11:887–900.
- Tang ZH, Liu YJ, Guo XR, Zu YG. 2011. The combined effects of salinity and nitrogen forms on Catharanthus roseus: the role of internal ammonium and free amino acids during salt stress. J Plant Nutrition Soil Sci. 174:135–144. 10.1002/jpln.200900354
- Tang ZH, Yang L, Zu YG, Guo XR. 2009. Variations of vinblastine accumulation and redox state affected by exogenous H2O2 in Catharanthus roseus (L.) G. Don. Plant Growth Regul. 57:15–20. 10.1007/s10725-008-9320-5
- Yu F, De Luca V. 2013. ATP-binding cassette transporter controls leaf surface secretion of anticancer drug components in Catharanthus roseus. Proc Natl Acad Sci USA. 110:15830–15835. 10.1073/pnas.1307504110
- Zhang WJ, Bjorn LO. 2009. The effect of ultraviolet radiation on the accumulation of medicinal compounds in plants. Fitoterapia. 80:207–218. 10.1016/j.fitote.2009.02.006
- Zhang M, Dong JF, Jin HH, Sun LN, Xu MJ. 2011. Ultraviolet-B-induced flavonoid accumulation in Betula pendula leaves is dependent upon nitrate reductase-mediated nitric oxide signaling. Tree Physiol. 31:798–807. 10.1093/treephys/tpr070