Abstract
The present study deals with the isolation and characterization of exopolysaccharides (EPS) produced by the plant growth-promoting rhizobacteria (PGPR) from arid and semiarid regions of Pakistan, and to investigate the drought tolerance potential of these PGPR on maize when used as bioinoculant alone and in combination with their respective EPS. Three bacterial strains Proteus penneri (Pp1), Pseudomonas aeruginosa (Pa2), and Alcaligenes faecalis (AF3) were selected as EPS-producing bacteria on the basis of mucoid colony formation. All these strains were gram negative, motile, and positive for catalase. Strain Pp1 was positive for oxidase test and was phosphate solubilizing, while Pa2 and AF3 were negative. The isolated strains were sequenced using 16SrRNA. Total soluble sugar, protein, uronic acid, emulsification activity, and Fourier-transformed infrared spectroscopy of EPS were determined. Drought stress had significant adverse effects on growth of maize seedlings. Seed bacterization of maize with EPS-producing bacterial strains in combination with their respective EPS improved soil moisture contents, plant biomass, root and shoot length, and leaf area. Under drought stress, the inoculated plants showed increase in relative water content, protein, and sugar though the proline content and the activities of antioxidant enzymes were decreased. The Pa2 strain isolated from semiarid region was most potent PGPR under drought stress. Consortia of inocula and their respective EPS showed greater potential to drought tolerance compared to PGPR inocula used alone.
Keywords:
Introduction
Microorganisms of soil play important role in the maintenance of quality and health of soil (Jeffries et al. Citation2003). Plant growth promotion was observed with bacteria that produce moderate levels of indole acetic acid, IAA including Azospirillum sp., Alcaligenes faecalis (AF3), Klebsiella sp., Enterobacter cloacae, Acetobacter diazotrophicus, Rhizobium (Costacurta & Vanderleyden Citation1995). Nakbanpote et al. (Citation2013) reported salt-tolerant and plant growth-promoting bacteria isolated from Zn/Cd contaminated soil. Similarly, Vardharajula et al. (Citation2011) demonstrated the induction of drought tolerance in maize by drought-tolerant plant growth-promoting Bacillus spp. Karnwal (Citation2009) reported best plant growth-promoting activity of Pseudomonas fluorescens AK1 and Pseudomonas aeruginosa AK2. AF3 are known to colonize the rhizosphere of rice plants, and part of the bacteria can penetrate into root cells and fix dinitrogen there.
Exopolysaccharides (EPS) are the active constituents of soil organic matter (Gouzou et al. Citation1993). EPS are most important part of extracellular matrix that often represent 40–95% of bacterial weight (Flemming & Wingender Citation2001). Bacteria produce EPS in two forms: (1) slime EPS and (2) capsular EPS (Vanhooren & Vandamme Citation1998). EPS are found in a wide variety of complex structures (Kumon et al. Citation1994). The important roles exhibited by EPS are (1) Protective, (2) surface attachment, (3) biofilm formation, (4) microbial aggregation, (5) plant–microbe interaction, and (6) bioremediation (Manca de Nadra et al. Citation1985). Some physical and chemical properties of EPS are useful in industries for stabilizing, thickening, coagulating, gelling, suspending, film forming, and water-retention capability in different industries like detergents, textile, paper, paints, adhesive, beverages, and food (Sutherland Citation1996). Some EPS-producing bacteria like Pseudomonas have the ability to survive even under drought stress due to the production of their EPS (Sandhya et al. Citation2009a, Citation2009b). EPS of bacteria are hydrated compounds with 97% of water in polymer matrix which impart protection against desiccation (Wingender et al. Citation1999; Hunter and Beveridge Citation2005; Bhaskar & Bhosle Citation2005). The EPS protect these bacteria from desiccation under drought stress by enhancing the water retention and by regulation of organic carbon source's diffusion (Roberson & Firestone Citation1992; Chenu Citation1993; Chenu & Roberson Citation1996). Due to enzymatic activities of EPS, they help in heavy metal transformation and degradation of organic recalcitrant (Van-Hullebusch et al. Citation2004; Pal & Paul Citation2008). Water availability in the soil also affects the soil structure (Roberson & Firestone Citation1992). Plants treated with EPS-producing bacteria Azospirillum showed resistance to water stress (Bensalim et al. Citation1998) through improvement in the soil structure (Sandhya et al. Citation2009a) and soil aggregation (Bashan et al. Citation2004). Under drought stress, inoculation of sunflower with EPS-producing bacterial strain YAAF34 showed increase in root tissue (Alami et al. Citation2000).
Maize has an important position in the cropping system of Pakistan being the third in grain production after wheat and rice. It is the source of food not only for human but also for livestock, poultry, and used as a good forage crop. Requirement of water for maize (135 mm/month) at the time of seedlings is 4.5 mm/day which increase up to 195 mm/month that is 6.5 mm/day during hot and windy conditions (Jamieson et al. Citation1995). The maize inoculated with Pseudomonas spp. strains has increased plant biomass, relative water contents (RWCs) of leaves, leaf water potential, root adhering soil/root tissues ratio, stability of soil aggregates, and decreased the leaf water loss (Chen & Dai Citation1994). Plants inoculated with EPS-producing bacteria showed higher concentration of sugars, proline, and free amino acids under drought stress conditions. Attenuated total reflection Fourier-transformed infrared (ATR-FTIR) spectroscopy gives us information at molecular level for inorganic and organic constituents of bacterial EPS. It permits to investigate the functional groups in close proximity that are dipolar (Braun et al. Citation1989). It is noninvasive, in situ method of spectroscopy that helps in the investigation of EPS constituents, functional group chemistry, and conformational changes without disturbing intermolecular associations of the constituents e.g. protein–polysaccharide association (Anselm et al. Citation2004).
The present investigation was aimed to characterize the EPS-producing bacteria from arid and semiarid areas of Pakistan and evaluation of their potential on maize when used as bioinoculant alone and in combination with their EPS.
Materials and methods
Collection of soil samples
Soil samples for the isolation of bacteria were collected from soil of two rainfed areas of Pakistan, including Ghotki Sindh and Kallar sayedan. The soil was taken from 6 inches depth. Ghotki Sindh lies between 26–28° North and 68–70° East (Bhatti et al. Citation2001; Qureshi Citation2004). It is arid area with extreme temperature range, severe drought with high wind and scanty rainfall, and average rainfall of 100–250 mm mostly during July and September. Temperature ranged from 40°C to 52°C in summer and below 0°C in winter (Qureshi & Bhatti Citation2005). Kallar Sayedan is located in Rawalpindi district. It lies at an altitude of 27–33° north and longitude of 16–73° east, with 8% of soil moisture. It belongs to semiarid area.
The pH and electrical conductivity (EC) of soil samples were measured by the method of McKeague (Citation1978) and McLean (Citation1982), respectively.
Isolation and growth of bacteria from soil
Isolation of bacteria from soil samples collected from Ghoti and Kallar Sayedan areas of Pakistan was done by serial dilution method. During this method, 10 g of soil sample was suspended in 9 ml of distilled water. Soil suspension was centrifuged at 3000 rpm for 10–15 min. Decimal dilutions were made. Aliquots (20 µl) from three dilutions 10−1, 10−5, and 10−7 were spread on Luria Bertini (LB) agar plates, incubated for 48 h at 28°C. After 24 h distinct bacterial colonies were streaked on LB agar plates, 3–4 times until single colony was obtained.
Extraction purification and characterization of EPS
EPS-producing bacteria were cultured in optimized mineral salts medium with 12.6% K2HPO4, 18.2% KH2PO4, 10% NH4NO3, 1% MgSO4.7H2O, 0.6% MnSO4, 1% CaCl2.2H2O, 0.06% FeSO4.2H2O, 1% sodium molybdate, 1.5% NaCl, and 0.2% of glucose in 1 l of distilled water for 10 days (Bramchari & Dubey Citation2006).
After incubation for 10 d, the bacterial cultures (250 ml) were centrifuged at 15,000 rpm for 20 min at 4°C. The EPS were extracted from the supernatant by the addition of twofold ice cold ethanol (95%), the solution was chilled at 4°C for complete precipitation. EPS were collected from above solution (Kumar et al. Citation2011). EPS extracts were washed with 70–100% ethanol–water mixture; redissolved in distilled water and dialyzed with dialysis tubing (molecular weight cut-off of 13 kDa; Sigma-Aldrich Chemie GmbH, Seelze, Germany) against distilled water at 4°C for 24 h to remove excess salts from EPS. Extracted EPS were lyophilized with Labonco lyophilizer at 3000 psi and stored at room temperature (Bramchari & Dubey Citation2006). To determine the solubility of EPS, small quantities of lyophilized EPS were suspended in 2 ml of benzene, water, chloroform, acetone, ethanol, and methanol. The mixture was vortexed and allowed to stabilize for some time and the pellet formation was observed.
Gram staining and motility test of the bacteria
Vincent method (Citation1970) was used for the preparation of slides of isolated bacterial cultures for gram staining.
Catalase test
Bacterial cultures (24 h) were used for catalase (CAT) test. Single bacterial colony was placed on glass slide and a drop of 30% hydrogen peroxide (H2O2) was added. Appearance of gas bubbles indicated the presence of CAT enzymes in the bacteria (McFadden Citation1980).
Oxidase test
For the determination of oxidase in the bacterial strains (1% N,N,N,N-tetramethylphenylenediamine), Kovacks's reagent was used (Kovacs Citation1956). The reagent was mixed with hot water and kept in dark. Filter paper strip was dipped in Kovack's solution and air dried. Cultures (24-h-old) were placed on this paper strip. Bacterial strains positive for oxidase show lavender color that slowly changes into dark purple and then black (Steel Citation1961).
Phosphorus solubilization index
Pikoviskaya's media were poured in Petri plates under sterilized conditions. With the help of sterilized tooth picks, a pinpoint inoculation was done on these agar plates under sterilized conditions. The plate was incubated at 28°C for 7 days. Formation of clear halozone was the indication of phosphorus solubilization on plates. Index of phosphorus solubilization was calculated by colony diameter and halo zone diameter (Edi Premono et al. Citation1996).
Quantification of available phosphates solubilized by EPS-producing bacteria
Quantification of available phosphates solubilized by bacteria was done using the phosphomolybdate blue color method (Murphy & Riley Citation1962). Pikoviskaya's broth (pH 7) and solution of tricalcium phosphate (0.3 g/100 ml) were autoclaved mixed and two loops full of phosphate-solubilizing strains were inoculated in each of the flask under aseptic conditions. The culture in the flask was placed on the rotary shaker at 12,000 rpm for 12 days. Thereafter, the inocula suspension was centrifuged at 10,000 rpm for 15 min. The supernatant obtained was used for determining the available phosphorus through spectrophotometer at 882 nm and calibrated against standard curve of phosphorus.
DNA extraction
Extraction of genomic DNA of bacterial strain was carried out by using the GenElute Bacterial Genomic DNA Kit.
Polymerase chain reaction
The genomic DNA of plant growth-promoting rhizobacteria (PGPR) was amplified by the method described by Weisburg et al. (Citation1991). The polymerase chain reaction (PCR) was carried out by using forward (fd1) primer having nucleotide sequence AGAGTTTGATCCTGGCTCAG and reverse (rd1) primer (AAGGAGGTGATCCAGCC). The reactions were carried out in a thermocycler (Biometra, Germany). Each reaction volume (25 µl) contained 1 µl of template DNA, 0.2 mM dNTP mix, 1.5 mM MgCl2, 5 µl of 10× Taq buffers, 1 unit of Taq DNA polymerase, and 10 pmols of each primer. The volume was raised to 25 µl by autoclaved cold water. After denaturation at 95°C for 2 min, 30 rounds of temperature cycling (94°C for 30 sec, 55°C for 30 sec, and 72°C for 2 min) were followed by incubation at 72°C for 10 min. Then, 5 µl of amplified PCR products was electrophoresed on 1.2% (w/v) agarose gel, in 1× Tris Borate EDTA (TBE) buffer at 80 V, and then stained with ethidium bromide (0.01 g/ml). Gel was visualized under UV transilluminator lamp (S. No. 76S/64069, Bio RAD, Italy) and photographed. The 1 Kb DNA ladder (Fermentas, Germany) was used as marker. The PCR product was excised from gel, purified by using gel purification kits (JET quick, Gel Extraction Spin Kit, GENOMED) and sequenced on automated sequencer. The sequences were compared with standard databases by BLAST (NCBI) software.
Sequencing
The purified PCR products of approximately 1400 bp were sequenced by using two sets of primers 27F AgA gTT TgA TCM TGG CTC Ag, 1492R TAC ggY TAC CTT gTT ACg ACT T, 518F CCA gCA gCC gCg gTA ATA Cg, and 800R TAC CAg ggT ATC TAA TCC. Sequencing was performed by using Big Dye terminator cycle sequencing kit v.3.1 (Applied BioSystems, USA). Sequencing products were resolved on an Applied Biosystems model 3730XL automated DNA sequencing system (Applied BioSystems, USA) at the Macrogen, Inc., Seoul, Korea.
Inoculation of maize (Zea mays L.) with EPS-producing bacteria
The cultures were allowed to grow in an orbital shaker (ECELLA E23, USA) at 120 rpm for 48 h at 30°C, thereafter centrifuged at 3000 rpm for 15–20 min. These cultures at optical density 600 nm (OD600) equivalent to 1 were used as bioinoculant. Effect of EPS-producing bacteria on the growth of maize was studied by the inoculation of maize seeds with EPS bacterial cells alone and in combination with their respective EPS. Seeds of maize cv.Agaiti-2002 collected from National Agricultural Research Centre, Islamabad were surface sterilized with 95% ethanol followed by shaking with 10% clorox for 2–3 min and successively washed with sterilized water. For seeds inoculation with bacterial cells, 48-h-old cultures were prepared in LB broth media, and for inoculation of seeds with cells and their respective EPS, 10-d-old cultures were used. Sterilized seeds were soaked in both 48-h-old cultures and 10-d-old cultures of bacteria for 3–4 h. Seeds were sown in pots containing autoclaved mixture of soil and sand with the ratio of 3:1, respectively. Soil and sand mixture was autoclaved three times for complete sterilization. Pots were placed in growth chamber with average day and night temperature of 25°C and 18°C, respectively. After 1 week of seed germination, the seedlings were subjected to drought stress by withholding water supply for 10 d, the nonstressed plants were kept well watered. After 10 days of drought stress, plants were harvested for further analysis. The age of plant was 21 d at the time of harvest.
Emulsification activity of EPS
To determine emulsification activity, a modified method of Rosenberg et al. (Citation1979) was used. Lyophilized EPS (0.5) was dissolved in 0.5 ml of distilled water by heating for 15–20 min, cooled at room temperature. Phosphate buffer saline (PBS) was added to the EPS solution to make a total volume of 2 ml. Hexadecane (0.5 ml) was added in the mixture and was vortexed for 1 min. The absorbance was taken immediately after vortex (A 0) at 540 nm on spectrophotometer. The mixture was incubated at room temperature and absorbance was measured after 30 min and 60 min (A t ). After incubation at room temperature for 30 and 60 min, fall in absorbance was recorded. Emulsifying activity of EPS was calculated as percentage retention after incubation time t = A t /A 0 × 100. A control was prepared as a mixture of PBS (2 ml) and hexadecane (0.5 ml).
To determine the solubility of EPS, small quantities of lyophilized EPS were suspended in 2 ml of benzene, water, chloroform, acetone, ethanol, and methanol. The mixture was vortexed and allowed to stabilize for some time and the pellet formation was observed.
The total soluble sugar, protein, and uronic acid of EPS were determined. Suspension of EPS was prepared by resuspending 2 g of lyophilized EPS in 10 ml of distilled water. Quantification of sugar was done by phenol–sulfuric acid (PSA) method (Dubois et al Citation1956). Quantification of protein was made by Lowry method (Lowry et al. Citation1951) using bovine serum albumin as standard, and absorbance was measured by spectrophotometer at 500 nm. Quantification of uronic acid was carried out by carbazole assay (Taylor & Buchanan- Smith Citation2001). To analyze the functional groups of EPS, FTIR spectroscopy of lyophilized EPS was done by single reflection ATR accessories.
Determination of effects of PGPR and their EPS on physiological parameters of soil and plants
After harvesting the plants, moisture content of rhizosphere soil of both stressed plants and nonstressed plants was measured. Fresh weight of soil sample (20 g) collected from 6-inch rhizosphere of plants was dried in oven for 72 h at 70°C. Dry weight of soil was recorded and moisture content was calculated as:
The method of Beauchamp and Frodovich (Citation1971) was used for superoxide dismutase (SOD) estimation. Estimation of peroxidase (POD) enzyme was done by the method of Vetter et al. (Citation1958) and modified by Gorin and Heidema (Citation1976). CAT activity was estimated by the method of Chandlee and Scandalios (Citation1984).
Statistical analyses of data
The data recorded in laboratory and pot experiments were subjected to statistical analysis with statistix version 8.1. Means and standard errors of the means were calculated. Results were evaluated by analysis of variance (ANOVA). The differences between the means of inoculated and control treatments were tested using the least significant differences test (p < 0.05).
Results
pH and EC of soil samples
EC and pH both were higher for the soil from Sindh. pH of soil from Ghotki Sindh was 8.72 and from Kallar Saydan was 8.10. EC of soil sample from Ghoti Sindh was 210 and from Kallar Saydan was 160.
Isolation and identification of EPS-producing bacteria
Ten bacterial strains were isolated from three soil samples. All bacterial colonies were streaked on LB agar plates. Three bacterial strains (Proteus penneri [Pp1], Pseudomonas aeruginosa [Pa2], and AF3) were selected for EPS production on the basis of mucoid colony morphology and other biochemical tests. On LB agar plates, bacterial strains Pp1 produced green mucoid colony with soapy odor and Pa2 produced yellow green mucoid colony with grape-like odor, whereas AF3 gave nonpigmented colonies with pleasant fruity odor. All colonies were circular ranging from 0.3 to 0.5 cm with entire margin. All bacterial isolate were gram negative; Pp1 and Pa2 were bacilli and AF3 was cocci. Pp1 isolate was positive for CAT and negative for oxidase, whereas Pa2 and AF3 were positive for both oxidase and CAT tests.
Alignment of 16S rRNA sequence
For the isolate Pp1 obtained from arid region of Ghoti Sindh, the total length of sequence with 1537 nucleotide was obtained. The comparison of the nucleotide sequence with data nucleotide bank showed highest sequence similarity with 1464/1469 and 99% with that of Pp1 strain (ACC No.: JN092595.1).
For the isolate Pa2 obtained from semiarid region of Kallar Sayedan, the total length of sequence with 1498 nucleotide was obtained. The comparison of the nucleotide sequence with data nucleotide bank showed highest sequence similarity with 1465/1465 and 100% with that of Pa2 strain (ACC No.: AY792969.1).
For the isolate Af3 obtained from semiarid region of Kallar Sayedan, the total length of sequence with 1462 nucleotide was obtained. The comparison of the nucleotide sequence with data nucleotide bank showed highest sequence similarity with 1460/1461 and 99% with that of AF3 strain (ACC No.: AB680626.1).
Phosphorus solubilization by bacterial isolates
Only Pp1 strain, that is, Pp1 formed a clear halo zone around the colony indicating the phosphorus solubilized from tricalcium phosphate added in the medium. The index of P solubilization was 2.11 cm and the total amount of P solubilized by Pp1 was 0.49 µg/ml.
Emulsification activity of EPS
Emulsification activity gives the strength of EPS in retaining the emulsion breaks. Dialyzed EPS have greater activity of emulsification than nondialyzed EPS (). The nondialyzed EPS of strain Pp1 retained 42% and 10% after 30 and 60 min, respectively, while dialyzed EPS had 47% and 34%, respectively. Nondialyzed EPS of Pa2 retained similar emulsification activity at 30 min but at 60 min, the activity was 50% lower, while dialyzed ones had almost similar emulsification activity, that is, 45% and 34%, respectively. Similarly in case of AF3 strain, nondialyzed EPS retained 37% and 19% after 30 and 60 min while dialyzed EPS had 46% and 33% emulsification activity.
Solubility and chemical composition of EPS
The lyophilized EPS were soluble in water and insoluble in benzene, acetone, chloroform, and ethanol. Similar results were reported by Vimala and Lalithakumari (Citation2003). The chemical composition of EPS revealed () that sugar content was 97% in Pp1 and 98% in EPS of Pa2 and AF3 strains. The protein content was 97% in Pp1 and 98% higher than control in EPS of Pa2 and AF3 strains. There were no significant differences in sugar and uronic acid contents of the isolates. Uronic acid content was lower than that of sugar in EPS of all strains.
Table 1. Chemical characterization, sugar, protein, and uronic acid contents of EPS.
Fourier-transformed infrared spectroscopy of EPS
The FTIR spectrum of EPS of bacterial isolates showed various functional groups (–). The H-bonded hydroxyl group typical of EPS (3273–3278 cm−1) as well as C–H group (at 2923 to 3016) and at 3016.78 cm−1, 2908.98 cm−1 representing aliphatic CH symmetric and asymmetric stretching in CH3 and CH2, bands corresponding to fatty acid region; the C = O asymmetric stretching of –NH-CO-R and/or N-H bonding of H2N-CO-R (Amide I; at 1633), the C-O-C group vibrations in the cyclic structures of carbohydrates that represent polysaccharide region were commonly present in all three strains. The peaks of N-H bonding of –NH- (Amide II) as protein region, the P = O stretching of phosphate PO43 − representing nucleic acid region (1377–1379); the O–H bonding in carboxylic acid and C-OH stretching of phenolic (OH) group were also identified but were not common to all strains (–).
Table 2. Potential functional groups in EPS of PP1 bacterial strain.
Table 3. Potential functional groups in EPS of Pa2 bacterial strain.
Table 4. Potential functional groups in EPS of AF3 bacterial strain.
Table 5. Emulsifying activity of EPS.
Growth and physiological attributes
Plant growth was significantly enhanced by inoculation of EPS-producing bacterial strains. After exposure to 3 d, drought stress uninoculated plants started wilting but inoculated plants with bacterial cells alone resisted stress up to 7 days prior to wilting, while seeds inoculated with bacterial cells with EPS delayed wilting till 10 d. Soil inoculated with all strains in combination with their respective EPS exhibited increase in soil moisture contents. Treatment of Pp1 and Pa2 with their respective EPS showed 68% and 67% increase in soil moisture, respectively. Pa2 strains in combination with their respective EPS showed 68% soil moisture content in stress and 74% in unstressed over uninoculated control. Seeds inoculated with all strains in combinations with its EPS showed greater increase in leaf area as compared to control both in stressed and unstressed conditions. Inoculations of strain Pa2 with its EPS resulted in 130% increase under unstressed and 127% under drought-stressed under nonstress conditions. In this study, RWCs of all the treatment were found to be significant as compared with the uninoculated control. Treatment of Pa2 in combination with their respective EPS showed 45% increase in RWCs over control (). The plants inoculated with Pseudomonas putida strain GAP-P45 EPS-producing bacteria showed increase in soil aggregation, root adhering soil, and high RWC of leaves (Sandhya et al. Citation2009b). RWC of leaves is the index of plant water status that helps in the evaluation of tolerance to drought stress, and reduction in the RWCs causes closure of stomata.
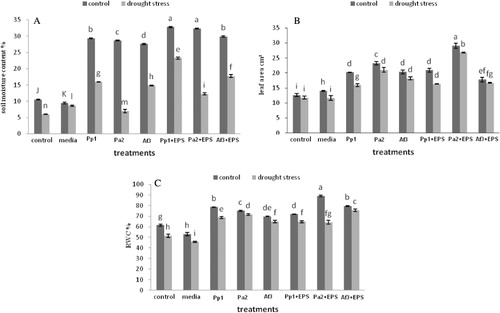
Root length and shoot length in all the treatments were increased in both drought-stressed and unstressed conditions while treatment of Pa2 and their respective EPS showed maximum increase in shoot length by 76% under drought-stressed and 72% under unstressed conditions. Treatment of AF3 and their respective EPS showed maximum increase in root length as 50% in nonstress and 42% in stress over noninoculated control. Root and shoot fresh weights of all treated plants were also significantly higher in comparison with uninoculated control. Dry weight of roots and shoots was also higher in plants inoculated with EPS-producing bacteria. Plants inoculated with strain Pp1 have same value of fresh and dry weight of shoots in both stress and nonstress conditions, which show the resistance to drought effect ().
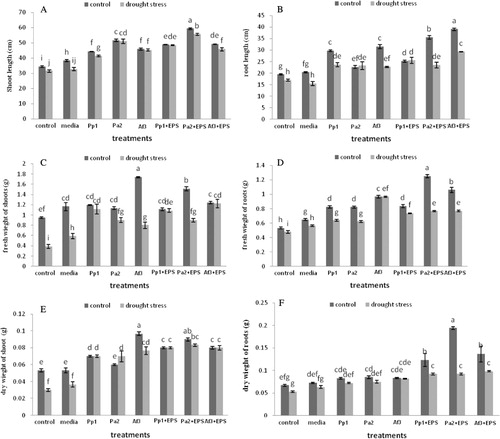
Response of maize seedlings to drought
Inoculation with EPS-producing strains significantly improved protein and sugar concentration in the leaves under stress conditions (). All the treatments decreased the proline content and activity of antioxidant enzymes in leaves. Pa2 + EPS had maximum decrease in proline content and activities of antioxidant enzyme of leaves as compared to uninoculated control ().
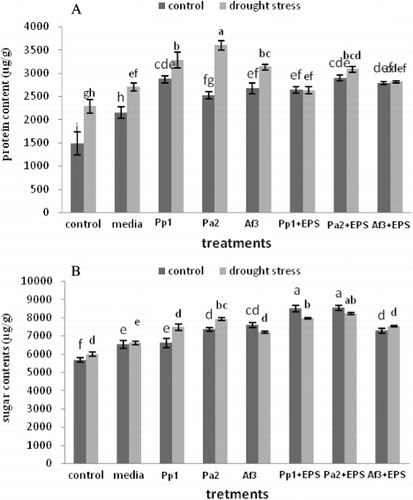
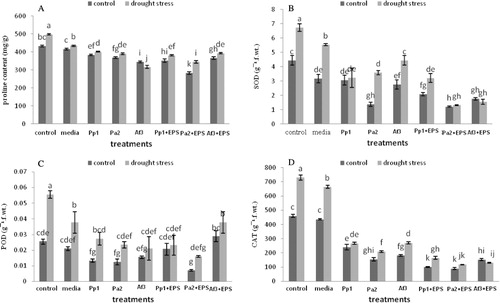
The plants inoculated with EPS-producing bacteria showed decreased activity of ascorbate peroxidase (APX), CAT, and glutathione peroxidase (GPX) enzymes under drought stress play their role in plants to tolerate the stress.
Discussion
The bacterial strains Pp1, Pa2, and Af3 produced mucoid colony on LB agar media. Roberson and Firestone (Citation1992) and Junkins and Doyle (Citation1992) demonstrated Pa2 and Escherichia coli as EPS-producing organisms on the basis of mucoid colony. The production of pigmentation in all bacterial strains was also detected during the selection process both in LB agar and LB broth media. Nair et al. (Citation1992) and Arrage et al. (Citation1993) demonstrated that pigmentation by EPS-producing bacteria was related to resistance in these bacteria against toxic substances in contaminated water.
The EPS extract contained carbohydrates (neutral sugars), proteins, and very small amount of uronic acids. Bramchari and Dubey (Citation2006) demonstrated that the production of EPS by Vibrio harveyi composed of neutral sugars, proteins, and uronic acids which imparts acidic nature to EPS.
FTIR spectrum of EPS obtained during the present study revealed cyclic carbohydrates of polysaccharides, proteins, and uronic acid. Schmitt and Flemming (Citation1998) and Sheng et al. (Citation2006) arbitrarily divided the spectrum obtained from FTIR into several characteristic bands representing different compounds. According to them, their EPS have cyclic carbohydrates of polysaccharides, uronic acid, and proteins. The presence of acidic functional groups of uronic acid (glucuronic acid) in EPS are involved in metal sequestration (Bhaskar & Bhosle Citation2005; Bramchari & Dubey Citation2006; Pal & Paul Citation2008). Hydroxyl and amine groups of EPS were predominant in lead sequestration and thus protect E. cloacae strain P2B from lead toxicity (Pal & Paul Citation2008). The presence of acidic sugars in the EPS may be important, helpful in the heavy metal-binding properties of this polymer. The chemical composition of EPS produced by Pa2 was analyzed by Grobe et al. (Citation1995). FTIR analysis of the three bacterial EPS also indicated the presence of many charged groups. The charged groups of the EPS are involved in biological and physiological functioning of the EPS (Sutherland Citation2001) and the EPS-producing rhizospheric bacteria. It is inferred that these EPS would also play an important role in mechanical and structural stability of the salt-affected soils and soil aggregation around plant roots (Amellal et al. Citation1998; Alami et al. Citation2000).
Soil moisture content was 10–12% in the untreated control plants which declined to 5–7% under drought stress. Maximum moisture content of soil was recorded for the rhizosphere soil inoculated with PGPR strains in combination with their respective EPS both under drought-stress and unstressed conditions. Treatment of Pp1 and Pa2 with their respective EPS showed maximum increase in soil moisture by 68% and 67% increase, respectively. The high affinity of EPS for water provides protection to bacteria under drought stress. EPS hold the water in the soil surrounding the plant roots and soil dries more slowly, also protect the bacteria from desiccation and fluctuations in water potential (Hepper Citation1975). Roberson and Firestone (Citation1992) suggested that the increase in EPS production by Pseudomonas during desiccation is required to ensure the protection of the bacterial strain in soil. The EPS type play direct bearing on the drought tolerance of inoculated plants. The strains Pp1 and AF3 having both phosphate group and phenolic group were more responsive to drought stress. The Pp1 has greater emulsification ability in both short-term (30 min.) and long-term (60 min.) incubation of dialysis further this strain has P solubilization potential; hence was8 found to exhibit greater retention of moisture content in the rhizosphere soil of maize inoculated along with its EPS. The RWC which is an index of drought tolerance and a measure of water status of plants, the PGPR Af3 was more effective with its EPS which was also reflected in the fresh weight of shoot which showed no significant effect of drought stress on the shoot fresh weight as compared to its unstressed control. This strain had SO4 group and also -OH bonding of carboxylic acid. There was no difference in the production of biomass (as evidenced by shoot dry weight) between the unstressed and drought-induced seedlings in any of the treatment with PGPR and its corresponding EPS. Although the roots were longer in the drought-stressed plants of AF3 and their respective EPS treatment, the fresh and dry weights of the roots have similar magnitude of increase over untreated stressed plants in all the three treatments. The leaves of the plant inoculated with Pa2 showed maximum protein content under drought stress which corresponds with the higher protein in its EPS. The activity of antioxidant enzyme SOD was higher specifically in Pa2 and its EPS-treated plant leaves, whereas POD was greater in Af3 and its EPS-treated plant leaves. The SOD is the principal enzyme acting as reactive oxygen species (ROS) scavenger, whereas POD assists in neutralizing the H2O2 produced during SOD interaction with ROS. These differences can be attributed to the difference in the functional group of EPS of the corresponding PGPR.
The RWC of all the treatment was found to be significantly higher compared with the uninoculated control. Treatment of Pa2 showed maximum increase in RWC of plant leaves. Plants having higher yield under drought stress need to maintain higher RWC. The decrease in RWC in plants under drought stress may depend on reduction in plant vigor, and has been observed in many plants (Liu et al. Citation2002). The plants inoculated with P. putida strain GAP-P45 EPS-producing bacteria showed high RWC of leaves (Sandhya et al. Citation2009b). Bacterial EPS have an ability of water holding and cementing due to which EPS-producing bacteria helped to maintain the moisture content of soil and flow of water across the plant roots due to the formation of soil aggregates (Tisdall & Oadea Citation1982; Roberson & Firestone Citation1992).
The development of optimal leaf area is important for photosynthesis and dry matter yield. Pa2 with its EPS gives maximum increase in leaf area under nonstress condition and also under drought condition. Root length in all the treatments was higher in both drought stress and nonstress conditions. The importance of root systems in acquiring water has long been recognized. The development of root system increases the water uptake and maintains requisite osmotic pressure in Phoenix dactylifera (Djibril et al. Citation2005). Pseudomonas spp. increased total microbial activity, shoot and root length, and total dry weight (Ahn et al. Citation2007). The inoculation with EPS-producing bacteria could cause the development of much better root system which subsequently increases the shoot growth (Nemat et al. Citation2012). All treatments have increased shoot length in both drought stress and nonstress plants than noninoculated control. Inoculation of Pa2 shows maximum increase in shoot length. Inoculation of P. putida strain GAP-P45 increased total shoot length as studied by Sandhya et al. (Citation2010).
Root and shoot fresh weight of all treated plants was significantly higher in comparison with uninoculated control. Dry weight of roots and shoots was also higher in plants inoculated with EPS-producing bacteria. Plants inoculated with strain PP1 have same value of fresh and dry weight of shoots in both stress and nonstress conditions which show the resistance to drought effect. Plant growth-promoting bacteria influence growth of plant through various mechanisms and several researchers have reported healthy effect of PGPR inoculation on various crops (Khalid et al. Citation2004). Plants inoculated with strain Pa2 showed maximum increase in root fresh and dry weight. Zahir et al. (Citation2008) also reported improved fresh and dry weight of plants following inoculation with Pseudomonas fluorescens biotype G (ACC-5), and P. putida biotype A (Q-7).
Protein content was significantly greater in all the treatments as compared to the uninoculated control. Application of Pa2 showed maximum increase in protein content of leaves. The increased protein content prevents denaturation and decomposition of the cellular molecules and components especially during a biotic stress conditions (Campbell & Close Citation1997). Inoculation of EPS-producing Pseudomonas sp. increased soluble sugar content in drought stress seedlings than uninoculated seedlings which indicate that possibly Pseudomonas sp. helps in the hydrolyses of starch content, subsequently more sugar was made available for osmotic adjustment to alleviate the effect of drought stress. In case of uninoculated seedlings under drought stress plant growth was affected due to the absence of PGP Pseudomonas sp. that in turn affected the biosynthesis and decreasing content of soluble sugar and starch. The observed corresponding increase in sugar and protein content of maize seedlings inoculated with the PGPR was attributed to the content of protein and sugar of EPS produced by the PGPR. Sandhya et al. (Citation2010) demonstrated that the adverse effects of drought stress on plant growth under uninoculated condition may be attributed to decrease in the content of starch and sugar.
The uninoculated control plants, compared to the inoculated plants, under drought conditions had increased activity of antioxidant enzymes SOD, POD, and CAT, and concentration of proline. Plants inoculated with Pa2 exhibited maximum decrease in the activity of antioxidant enzymes superoxidase SOD, POD, and CAT, and concentration of proline. Plants inoculated with PGPR strains under drought stress decreased the activities of antioxidant enzymes. It is interesting to note that drought stress and antioxidant enzyme activity have significant interaction, but inoculation with PGPR lessen the adverse effect of drought stress on the antioxidant enzymes activity (Han and Lee Citation2005), indicating that the effect of stress was less pronounced in the maize seedlings inoculated with EPS-producing bacteria (Sandhya et al. Citation2010). At cellular level most of the damages take place due to oxidative damage under drought stress, this injury is the result of imbalance between the production of ROS and their detoxification. Stress proteins play their role in plants to tolerate the stress; these proteins are water soluble (Wahid Citation2007). Soluble sugars are the important osmolytes that help in the osmotic adjustment in plants under drought stress. An increased level of soluble sugars was observed in plants under drought stress (Dekánková et al. Citation2004). Inoculation of plant seedlings with Psedomonas spp. increased the proline contents under drought stress; it may be due to upregulation of biosynthesis pathway of proline to keep the proline in higher concentration, that helped in maintaining the water status of cell and protect the membranes under drought stress (Yoshiba et al. Citation1997).
Conclusion
It is inferred from present study that the bacterial isolates Pp1, Pa2, and Af3 as EPS-producing bacteria can induce drought tolerance in addition to their ability as PGPR. The mechanism they adapt was to neconomize the water budget of the plants by increasing the RWC of leaves of inoculated plants to counteract the oxidative and osmotic stresses commonly prevalent under drought stress.
Acknowledgment
Authors are greatly indebted to Dr Muhammadi for his cooperation and expert opinion in experimental side.
References
- Ahn TS, Ka JO, Lee GH, Song HG. 2007. Microcosm study for revegetation of barren land with wild plants by some plant growth-promoting rhizobacteria. J Microbiol Biotechnol. 17:52–57.
- Alami Y, Champolivier L, Merrien A, Heulin T. 2000. The role of Rhizobium sp. rhizobacterium that produces exopolysaccharide in the aggregation of the rhizospherical soil of the sunflower: Effects on plant growth and resistance to hydric constraint. OCL – Oleagineux Corps Gras Lipides. 6:524–528.
- Amellal N, Burtin G, Bartoli F, Heulin T. 1998. Colonization of wheat rhizosphere by EPS producing Pantoea agglomerans and its effects on soil aggregation. Appl Environ Microbiol. 64:37040–3747.
- Anselm O, Jon C. 2004. Spectroscopic study of extracellular polymeric substances from Bacillus subtilis. Biomacromolecules. 5:1219–1230.
- Arrage AA, Phelps TJ, Benoit, RE, White, DC. 1993. Survival of subsurface microorganisms exposed to UV radiation and hydrogen peroxide. Appl Environ Microbiol. 59:3545–3550.
- Bates L, Waldren RP, Teare ID. 1973. Rapid determination of free proline for water-stress studies. Plant Soil. 39:205–207. 10.1007/BF00018060
- Bashan Y, Holguin G, de-Bashan LE. 2004. Azospirrilum-plant relationships: physiological, molecular, agricultural and environmental advances. Can J Microbiol. 50:521–577.
- Beauchamp C, Fridovich I. 1971. Superoxide dismutase. Improved assays and an assay applicable to acrylamide gel. Anal Biochem. 44:276–287. 10.1016/0003-2697(71)90370-8
- Bensalim S, Nowak J, Asiedu SK. 1998. A plant growth promoting rhizobacterium and temperature effects on performance of 18 clones of potato. Am J Potato Res. 75:145–152. 10.1007/BF02895849
- Bhaskar PV, Bhosle, NB. 2005. Microbial extracellular polymeric substances in marine biogeochemical processes. Curr Sci. 88:45–53.
- Bhatti GR, Qureshi R, Shah M. 2001. Ethnobotany of Qadan Wari of Nara Desert. Pak J Bot. 33:801–812.
- Bramchari PV, Dubey SK. 2006. Isolation and characterization of exopolysaccharides produced by Vibrio harveyi strain VB23. Lett Appl Microbiol. 43:571–577. 10.1111/j.1472-765X.2006.01967.x
- Braun D, Böhringer B, Eidam N. 1989. ATR-FTIR spectroscopy as a tool for studies of polymer-polymer miscibility. Polymer Bull. 21:63–68. 10.1007/BF00700270
- Campbell SA, Close TJ. 1997. Dehydrins: genes, proteins, and association with phenotypic traits. New Phytol. 137:61–74. 10.1046/j.1469-8137.1997.00831.x
- Chandlee JM, Scandalios JG. 1984. Analysis of variants affecting the catalase development program in maize scutellum. Theor Appl Genet. 69:71–77. 10.1007/BF00262543
- Chen J, Dai JY. 1994. Correlation among photosynthesis, lipid peroxidation and ultrastructural changes of mesophyll cells in corn leaves under water stress. Maize Sci. 2:36–40. Chinese
- Chenu C. 1993. Clay or sand polysaccharide associations as models for the interface between microorganisms and soil: water-related properties and microstructure. Geoderma. 56:143–156. 10.1016/0016-7061(93)90106-U
- Chenu C, Roberson EB. 1996. Diffusion of glucose in microbial extracellular polysaccharide as affected by water potential. Soil Biol Biochem. 28:877–884. 10.1016/0038-0717(96)00070-3
- Costacurta A, Vanderleyden J. 1995. Synthesis of phytohormones by plants associated bacteria. Crit Rev Microbiol. 21:1–18. 10.3109/10408419509113531
- Dekánková K, Luxová M, Gašparíková O, Kolarovič L. 2004. Response of maize plants to water stress. Biologia. 13:151–155.
- Djibril S, Mohamed OK, Diaga D, Diégane D, Abaye BF, Mauriceand S, Alain B. 2005. Growth and development of date palm (Phoenixdactylifera L.) seedlings under drought and salinity stresses. Afr J Biotechnol. 4:968–972.
- Dubois M, Gilles KA, Hamilton JK, Rebers PA, Smith F. 1956. Colorimetric method for determination of sugars and related substances. Anal Chem. 28:350. 10.1021/ac60111a017
- Edi Premono M, Moawad AM, Vlek PLG. 1996 Effect of phosphate-solubilizing Pseudomonas putida on the growth of maize and its survival in the rhizosphere. Indones J Crop Sci. 11:13–23.
- Flemming HC, Wingender J. 2001. Relevance of microbial extracellular polymeric substances (EPSs)-parts I: structural and ecological aspects. Water Sci Technol. 43:1–8.
- Gorin N, Heidema FT. 1976. Peroxidase activity in golden delicious apples as a possible parameter of ripening and senescence. J Agric Food Chemis. 24:200–201. 10.1021/jf60203a043
- Gouzou L, Burtin G, Philippy R, Bartoli F, Heulin T. 1993. Effect of inoculation with Bacillus polymyxa on soil aggregation in the wheat rhizosphere: preliminary examination. Geoderma. 56:479–490. 10.1016/0016-7061(93)90128-8
- Grobe S, Wingender J, Trüper HG. 1995. Characterization of mucoid Pseudomonas aeruginosa strains isolated from technical water systems. J Appl Bacteriol. 79:94–102. 10.1111/j.1365-2672.1995.tb03129.x
- Han HS, Lee KD. 2005. Physiological responses of soybean-Inoculation of Bradyrhizobium japonicum with PGPR in saline soil conditions. Res J Agric Biol Sci. 1:216–221.
- Hepper CM. 1975. Extracellular polysaccharides of soil bacteria. In: Walker N, editor. Soil microbiology, a critical review. New York: Wiley; p. 93–111.
- Hunter RC, Beveridge TJ. 2005. High resolution visualization of Pseudomonas aeruginosa PAO1 biofilms by freeze substitutions electron microscopy. J Bacteriol. 187:7619–7630. 10.1128/JB.187.22.7619-7630.2005
- Hullebusch VED, Zandvoort MH, Lens PNL. 2003. Metals immobilization in biofilms: mechanisms and analytical tools. Re/Views Environ. Sci. Bio/Technol. 2:9–33.
- Jamieson PD, Martin RJ, Francis GS. 1995. Drought influence on grain yield of Barley, wheat and maize. NZ J Crop Hortic Sci. 23:55–66. 10.1080/01140671.1995.9513868
- Jeffries P, Gianinazzi S, Perotto S, Turnau K, Barea JM. 2003. The contribution of arbuscular mycorrhizal fungi in sustainable maintenance of plant health and soil fertility. Biol Fértil Soils. 37:1–16.
- Junkins AD, Doyle MP. 1992. Demonstration of exopolysaccharide production by enterohemorrhagic Escherichia coli. Curr Microbiol. 25:9–17. 10.1007/BF01570076
- Karnwal A. 2009. Production of indole acetic acid by Fluorescent pseudomonas in the presence of L-tryptophan and rice root exudates. J Plant Pathol. 91:61–63.
- Khalid A, Arshad M, Zahir ZA. 2004. Screening plant growth-promoting rhizobacteria for improving growth and yield of wheat. J Appl Microbiol. 96:473–480. 10.1046/j.1365-2672.2003.02161.x
- Kovacs N. 1956. Identification of Pseudomonas pyocyanea by the oxidase reaction. Nature. 178:703.
- Kumon H, Tomoshika K, Matunaga T, Ogawa M, Ohmori HA. 1994. Sandwich cup method for the Pseudomonas exopolysaccharides. The IR spectrum of the polymer proved the presence. Microbiol Immunol. 38:615–619. 10.1111/j.1348-0421.1994.tb01831.x
- Kumar AM, Anandapandian KTK, Parthiban K. 2011. Production and characterization of exopolysaccharides (EPS) from biofilm forming marine bacterium. Braz Arch Biol Technol. 54:259–265. 10.1590/S1516-89132011000200006
- Liu Y, Fiskum G, Schubert D. 2002. Generation of reactive oxygen species by mitochondrial electron transport chain. J Neurochem. 80:780–787. 10.1046/j.0022-3042.2002.00744.x
- Lowry OH, Rosebrough NJ, Farr AL, Randall RJ. 1951. Protein measurement with the Folin phenol reagent. J Biol Chem. 193:265.
- Manca de Nadra, MC, Strasser AM, de Saad AA, de Ruiz Holgado P, Oliver G. 1985. Extracellular polysaccharide production by Lactobacillus bulgaricus CRL 420. Milchwissenschaft. 40:409–411.
- McFadden JF. 1980. Biochemical tests for identification of medical bacteria. Baltimore (MD): Williams and Wilkins; p. 51–54.
- McKeague JA. 1978. Manual on soil sampling and methods of analysis. Can Soc Soil Sci. 30:66–68.
- McKee GW. 1964. A coefficient for computing leaf area in hybrid corn. Agron J. 56:240–241. 10.2134/agronj1964.00021962005600020038x
- McLean EO. 1982. Soil pH and lime requirement methods of soil analysis, Part 2: chemical and microbiological properties. Am Soc Agron. 45:199–224.
- Murphy J, Riley JP. 1962. A modified single solution method for the determination of phosphate in natural waters. Analytica Chimica Acta. 27:31–36. 10.1016/S0003-2670(00)88444-5
- Nair S, Chandramohan D, Bharathi B. 1992. Differential sensitivity of pigmented and non-pigmented marine bacteria to metals and antibiotics. Water Res. 26:431–434. 10.1016/0043-1354(92)90042-3
- Nakbanpote W, Panitlurtumpai N, Sangdee A, Sakulpone N, Sirisom P, Pimthong A. 2013. Salt-tolerant and plant growth-promoting bacteria isolated from Zn/Cd contaminated soil: identification and effect on rice under saline conditions. J Plant Interact. 21:1–9. 10.1080/17429145.2013.842000
- Nemat M, Awad Azza Sh. Turky, Magdi TA, Magdy A. 2012. Ameliorate of environmental salt stress on the growth of Zea mays L. plants by exopolysaccharides producing bacteria. J Appl Sci Res. 8:2033–2044.
- Pal A, Paul AK. 2008. Microbial extracellular polymeric substances: central elements in heavy metal bioremediation. Ind J Microbiol. 48:49–64. 10.1007/s12088-008-0006-5
- Qureshi R. 2004. Floristic and ethnobotanical study of Desert Nara region, Sindh [Ph.D. thesis]. Khairpur, Sindh, Pakistan: Department of Botany, Shah Abdul Latif University. Vol. I–II: 1–454.
- Qureshi R, Bhatti GR. 2005. Nara desert, Pakistan: I: soils, climate and vegetation. Rangelands. 27:27–31. 10.2111/1551-501X(2005)27[27:NDP]2.0.CO;2
- Roberson EB, Firestone MK. 1992. Relationship between desiccation and exopolysaccharide production in soil Pseudomonassp. Appl Environ Microbiol. 58:1284–1291.
- Rosenberg E, Zuckerberg A, Rubinovitz C, Gutnick, DL. 1979. Emulsifier of Arthrobacter RAG-1: isolation and emulsifying properties. Appl Environ Microbiol. 37:402–408.
- Sandhya V, Ali SZ, Grover M, Kishore N, Venkateswarlu B. 2009a. Pseudomonas sp. strain P45 protects sunflowers seedlings from drought stress through improved soil structure. J Oilseed Res. 26:600–601.
- Sandhya V, Ali SKZ, Grover M, Reddy G, Venkateswarlu B. 2009b. Alleviation of drought stress effects in sunflower seedlings by exopolysaccharides producing Pseudomonas putida strain GAP-P45. Biol Fertil Soil. 46:17–26. 10.1007/s00374-009-0401-z
- Sandhya V, Ali SZ, Grover M, Reddy G, Venkateswaralu B. 2010. Effect of plant growth promoting Pseudomonas sp. On compatable solutes antioxidant status and plant growth of maize under drought stress. Plant Growth Regu. 10.1007/s10725-010-9479-4
- Schmitt J, Flemming HC. 1998. FTIR-spectroscopy in microbial and material analysis. Int Biodeteri or Biodegrad. 41:1–11 10.1016/S0964-8305(98)80002-4
- Sheng GP, Yu HQ, Wang CM. 2006. FTIR-spectral analysis of two photosynthetic H2-producing strains and their extracellular polymeric substances. Appl Microbiol Biotechnol. 73:204–210. 10.1007/s00253-006-0442-2
- Steel KJ. 1961. The oxidase reaction as a taxonomic tool. J Gen MicroMol. 25:297–806. 10.1099/00221287-25-2-297
- Sutherland IW. 2001. Biofilm exopolysaccharides: a strong and sticky framework. Microbiology. 147:3–9.
- Sutherland IW. 1996. Extracellular polysaccharides. Biotechnology. 200:615–657.
- Taylor KA, Buchanan-Smith JG. 2001. A colorimetric method for the quantitation of uronic acids and a specific assay for galacturonic acid. Anal Biochem. 110:190.
- Tisdall JM, Oadea JM. 1982. Organic matter and water stable aggregates in soils. J Soil Sci. 33:141–163. 10.1111/j.1365-2389.1982.tb01755.x
- Vanhooren P, Vandamme EJ. 1998. Biosynthesis, physiological role, use and fermentation process characteristics of bacterial exopolysaccharides. Rec Res Develop Ferment Bioeng. 1:253–300.
- Van-Hullebusch ED, Utomo S, Zandvoort MH, Lens PNL. 2004. Comparison of three sequential extraction procedures to describe metal fractionation in anaerobic granular sludges. Talanta. 65:549–558.
- Vardharajula S, Zulfikar Ali S, Grover M, Reddy G, Bandi V. 2011. Drought-tolerant plant growth promoting Bacillus spp.: effect on growth, osmolytes, and antioxidant status of maize under drought stress. J Plant Interact. 6:1–14. 10.1080/17429145.2010.535178
- Vetter JL, Steinberg MP, Nelson AI. 1958. Quantitative determination of peroxidase in sweet corn. J Agric Food Chem. 6:39–41. 10.1021/jf60083a006
- Vimala P, Lalithakumari D. 2003. Characterization of exopolysaccharides (EPS) produced by Leuconostocsp. V 41. Asian J Microbiol Biotechnol Environ Sci. 5:161–165.
- Vincent JM. 1970. A manual for the practical study of the root-nodule bacteria. Oxford: Blackwell Scientific [I.B.P Handbook, 15].
- Wahid A. 2007. Heat tolerance in plants: an overview. Environ Exp Bot. 61:199–223. 10.1016/j.envexpbot.2007.05.011
- Weatherley PE. 1950. Studies in the water relations of the cotton plant. The field measurement of water deficits in leaves. New Phytol. 49:81–87. 10.1111/j.1469-8137.1950.tb05146.x
- Weisburg WG, Dobson ME, Samuel JE, Dasch GA, Mallavia LP, Baca O, Mandelco L, Sechrest JE, Weiss E, Woese CR. 1991. 16S ribosomal DNA amplification for phylogenetic study. Phylogenetic diversity of the Rickettsiae. J Bacteriol. 171:4202–4206.
- Wingender J, Neu TR, Flemming HC. 1999. Microbial extracellular polymeric substances: characterization, structures and function. Berlin Heidelberg, NewYork: Springer.
- Yoshiba Y, Kiyosue T, Nakashima K, Yamaguchi-Shinozaki K, Shinozaki K. 1997. Regulation of levels of proline as an osmolyte in plants under water stress. Plant Cell Physiol. 38:1095–1102. 10.1093/oxfordjournals.pcp.a029093
- Zahir ZA, Munir A, Asghar HN, Shaharoona B, Arshad M. 2008. Effectiveness of rhizobacteria containing ACC deaminase for growth promotion of peas (Pisum sativum) under drought conditions. J Microbiol Biotechnol. 18:958–963.