Abstract
The effects of drought on chlorophyll fluorescence characteristics of PSII, photosynthetic pigments, thylakoid membrane protein (D1), and proline content in different varieties of mung bean plants were studied. Drought stress inhibits PSII activity and induces alterations in D1 protein. We observed a greater decline in the effective quantum yield of PSII, electron transport rate, and saturating photosynthetically active photon flux density (PPFDsat) under drought stress in var. Anand than var. K-851 and var. RMG 268. This may possibly be due to either downregulation of photosynthesis or photoinhibition process. Withholding irrigation resulted in gradual diminution in total Chl content at Day 4 of stress. HPLC analysis revealed that the quantity of β-carotene in stressed plants was always higher reaching maxima at Day 4. Photoinactivation of PSII in var. Anand includes the loss of the D1 protein, probably from greater photosynthetic damage caused by drought stress than the other two varieties.
1. Introduction
Osmotic stress induced by dehydration and salinity is among the major abiotic stresses that adversely impacts crop productivity (Garg et al. Citation2013), especially in arid and semiarid areas (Mudgal et al. Citation2010). In many regions of the world, anthropogenic climate change is resulting in extended periods of high temperatures and reduced water supply for crops. Often coupled to problems of groundwater pollution (Postel Citation2000), this decrease in water availability is increasing the potential for drought in many crop production systems. Understanding how plants respond to drought can play a major role in stabilizing crop performance under drought conditions and in the protection of natural vegetation.
Available reports have demonstrated that drought stress eventually results in a wide gamut of various biochemical and physiological modifications (Garg et al. Citation2012; Maksup et al. Citation2014). However, stressful environments considerably hamper the process of photosynthesis in most plants by altering the ultrastructure of the organelles and concentration of various pigments and metabolites involved in this process. Light energy absorbed by Chl is transformed into Chl fluorescence (Maxwell & Johnson Citation2000). Despite the fact that the extent of Chl fluorescence does not comprise more than 1–2% of total light absorbed by the Chl, its measurement is convenient and noninvasive (Ashraf & Harris Citation2013). It gives a valuable insight into exploitation of the excitation energy by PSII and indirectly by the other protein complexes of the thylakoid membranes (Roháček Citation2002). When drought stress is severe, decreases in the rate of utilization of ATP and NADPH in photosynthetic metabolism will not be compensated for by increases in water–water cycling and photorespiration, or other electron sinks and consequently, decreases in photosynthetic yield () will occur (Fracheboud & Leipner Citation2003).
A number of studies conducted in vivo have shown that drought stress causes considerable damage to the oxygen-evolving center (OEC) coupled with PSII (Kawakami et al. Citation2009) as well as degradation of D1 polypeptide, leading to the inactivation of the PSII reaction center (Liu et al. Citation2006; Zlatev Citation2009). The changes lead to the generation of reactive oxygen species (ROS), which ultimately causes the photoinhibition and oxidative damage (Ashraf Citation2009; Gill & Tuteja Citation2010). Plants have evolved a variety of protective mechanisms against the ROS-induced damage to cellular components, such as the dissipation of excess excitation energy and the synthesis of protective pigments, such as Carotenoids (Car) (Efeoglu et al. Citation2009).
It has been widely reported that plants accumulate a variety of compatible solutes such as proline, as an adaptive mechanism of tolerance to salinity and drought (Ashraf & Harris Citation2004). Compatible solutes, especially proline, are known to confer tolerance to plants against a variety of abiotic stresses by protecting membrane damage and protein, maintaining osmotic balance, scavenging free radicals, etc. (Tripathi et al. Citation2006; Tripathi Citation2010).
Bean plants are commonly exposed to high irradiance during the diurnal period (Ribeiro et al. Citation2004), which may cause additional disturbances in the photosynthetic apparatus under water deficit. Some experiments show that Vigna radiata var. radiata [L.] Wilczek, contrary to popular belief, cannot tolerate drought stress (Rafieishirvan & Asgharipur Citation2009), but there are little reports about negative effects of drought stress on yield and physiological characteristics of mungbean. However, in vitro drought-screening methods are facilitating progress in our understanding of drought-resistance traits and in our selection of drought-resistant genotypes (Muscolo et al. Citation2014). This work aimed to evaluate the responses of leaf chlorophyll fluorescence to water deficit in three bean varieties, and to discuss the possible differences between varieties namely RMG 268, K-851, and Anand in relation to changes in photosynthetic pigments, thylakoid membrane proteins, and proline content, as triggered by drought.
2. Materials and methods
2.1. Plant materials and growth conditions
Seeds of Vigna radiata namely var. RMG 268, var. K-851, and var. Anand were obtained from KVK (Krishi Vigyan Kendra, Banasthali University) and local seed market, Jaipur, Rajasthan, India. The seeds were washed in distilled water, surface-sterilized with 0.1% HgCl2 for 1 min, followed by repeated washing with sterile water, and then germinated on moist filter paper, in Petri dishes at 28°C, in the dark, for 3 days. Morphologically similar seedlings having well-developed roots were selected and cultivated in pots as soil culture in a plant growth chamber at day/night temperature 25 ± 2°C /17 ± 2°C, a photosynthetic photon flux density (PPFD) of 250 to 300 μmol m−2 s−1, day/night photoperiod of 14/10 h, and relative air humidity of 65–70%. The position of the plants in the growth room was changed weekly to reduce any effects of localized variation in light intensity and air temperature. The leaf samples from control and stressed plants from nearly the same position were harvested for the determination of various biochemical parameters.
2.2. Drought treatment
Drought treatment started two weeks after plant emergence. One set of plants kept as control were watered daily with half strength Hoagland's nutrient solution (Hoagland & Arnon Citation1950), whereas the other set of plants were subjected to progressive drought by withholding water.
2.3. Chlorophyll a fluorescence parameters
Chl a fluorescence was measured with a portable pulse-amplitude modulated photosynthesis yield analyzer (Mini-Pam, Heinz Walz, Effeltrich, Germany) equipped with a standard 2030-B leaf clip holder (Rascher et al. Citation2000) essentially according to the protocol described by Kumari et al. (Citation2005). Measurements of light intensity, close to the leaf surface, were taken by the micro-quantum sensor on the leaf clip calibrated against a Li-Cor quantum sensor (Li-Cor Inc, Nebraska, USA). The effective quantum yield of PSII was calculated as
, where F is the fluorescence yield of the light-adapted sample and
is the maximum light-adapted fluorescence yield when a saturating light pulse (3000 µmol m−2 s−1) of 800 ms duration is superimposed on the prevailing environmental light levels (Schreiber et al. Citation1995). During these measurements, special care was taken not to change the ambient conditions, e.g. the angle of the leaf or shading. Apparent rates of photosynthetic electron transport rate (ETR) were obtained as 0.5 × 0.84 ×
× PPFD. The factor 0.5 accounts for the excitation of both PSII and PSI and assumes equal distribution of the excitation energy to both photosystems. The factor 0.84 assumes that 16% of PAR is not absorbed by the photosystems. To maintain basic fluorescence (F) in the optimal range of detection, internal gain and measuring light intensity of Mini-PAM were adjusted according to the plant variety and time of day.
2.4. Determination of photosynthetic pigments
For pigment analysis, leaf disks were collected in early morning from fully expanded leaves. Photosynthetic pigments such as Chl a, Chl b, and total Chl were extracted and estimated according to Arnon (Citation1949). The optical density of the extracted Chl was measured at 645, 663, and 740 nm, using a double beam UV-VIS spectrophotometer (UV5704SS).
2.4.1. Pigment extraction
For β-carotene and lutein + zeaxanthin estimations, 0.1 g of leaf segments was punched from a leaf, frozen in liquid nitrogen, and stored at −80°C. For extraction, the leaf disks were first grounded in a mortar in liquid nitrogen, followed by grinding in cold 80% acetone. The acetone supernatants were measured, and filtered through a 0.45 µm syringe filter into amber HPLC vials.
2.4.2. HPLC
Chromatography was carried out on a 4.6 × 250 mm PARTISIL 5 OD S-3 C 8 column (Whatman Schleicher and Schuell). Samples were injected with a 25-µl syringe, and mobile phases were pumped at a flow rate of 1 ml/min. Peaks were detected at 440 nm by a Shimadzu, SCL-10, AV VP, System Controller. The method used was a modified version of Rivas et al. (Citation1989) and Pocock et al. (Citation2004). All solvents were of HPLC grade. Pure β-carotene was obtained from Sigma Chemical (St. Louis, MO, USA). For lutein and zeaxanthin, fractions were obtained by TLC.
2.5. Isolation of thylakoids
Thylakoid membranes were isolated as described by Chakraborty and Tripathy (Citation1992). The purity of the membrane fractions was verified by immunoblot analysis using antibodies against membrane-specific marker proteins. The sample preparations were protected from light and kept ice-cold during the isolation procedure. Isolated thylakoids were stored at −80°C.
2.6. Polyacrylamide gel electrophoresis (PAGE) and western blotting
Thylakoid membrane proteins were separated by SDS-PAGE according to Laemmli (Citation1970) in stacking and resolving gels 12.5% (2 M urea was added since protein was more sensitive to this reagent) and 5% respectively. For Western blotting, electrophoresed proteins were immediately electrotransferred onto nitrocellulose membrane. The immuno-staining of the blot was performed following the method of Knecht and Dimond (Citation1983). D1 primary antibody was kindly provided by Prof. E. M. Aro (University of Turku, Finland). Secondary antibody alkaline phosphatase–conjugated goat-anti-rabbit IgG was obtained from Sigma. Image was analyzed using the software package Kodak Digital Science 1D Image, analysis with profile 1 gel densitometer application.
2.7. Proline content
Free proline content was estimated using the acid ninhydrin method described by Bates et al. (Citation1973). Proline concentration was determined using a calibration curve prepared from L-proline (0–100 μg/ml) and expressed as μg proline g−1 FW.
2.8. Statistical analysis
The statistical analyses were done using the Indostat software (IndoStat Inc. Hyderabad, India). The data were analyzed with ANOVA, using stress regimes, varieties, and days of stress treatments as main effects for all the parameters. Interactions among the effects were also analyzed, and lower case letters a (p ≤ 0.001), b (p ≤ 0.01) and c (p ≤ 0.05) were considered as statistically significant. Graphs were drawn using Sigma Plot 8.0 software (SPSS Inc.). Results are presented as percent change for Chl a fluorescence and pigments after statistical analysis.
3. Results
3.1. Effects on photosynthetic performance
The results of various photosynthetic parameters, as measured by Mini-PAM in two weeks old plants of V. radiata exposed to incipient drought, are given (). Regression lines of and ETR of the light curves, each plotted versus PPFD, are depicted (). Experiments beyond fifth day of stress in all three varieties could not be continued as the leaves of the plants wilted perhaps due to excessive desiccation (–). This was corroborated by very low or negligible ETR and yield values in the stressed plants.
Table 1. Cardinal points of the regression lines of saturating photosynthetically active photon flux density, half saturating PPFD at half saturating ETR, effective quantum yield of PSII at saturating photosynthetically active photon flux density, and at half saturating photosynthetically active photon flux density and maximum electron transport rate for variety RMG 268, variety K-851, and variety Anand of Vigna radiata at day 1, day 2, day 3, and day 4 of drought stress.
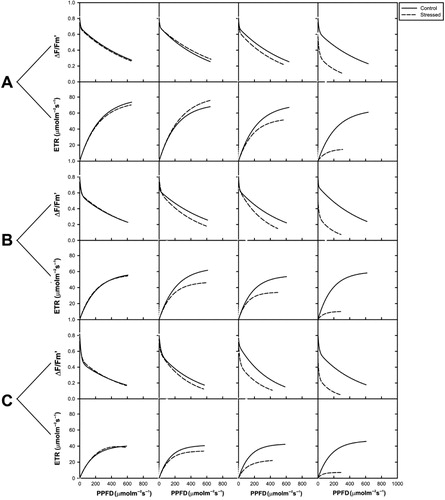
The cardinal points revealed that the ETRmax of stressed plants was significantly affected (p < 0.001), with a reduction of 76% (var. RMG 268), 81% (var. K-851), and 85% (var. Anand), as compared to control on fourth day of stress. For all V. radiata varieties studied, the yield of drought stressed plants was lower than the yield of control. It is noticed that yields of var. RMG, var. K-851, and var. Anand at Day 4 of stress were 56%, 67%, and 71% suppressed over control.
3.2. Effects on photosynthetic pigments
The net photosynthetic pigment content/concentration changes, on a fresh weight basis (Chl a, b, total Chl, and a:b content), were measured in control and stressed leaves of the three varieties of V. radiata, and the results are depicted (). Control plants of all varieties exhibited a higher total Chl content. Withholding irrigation resulted in gradual diminution in total Chl content up to 39% in var. RMG 268, 54% in var. K-851, and 57% in var. Anand, as compared to control at Day 4 of stress. However, concentrations of Chl a, Chl b, and total Chl increased first and then decreased with incipient drought stress in var. RMG 268. The concentration of var. RMG 268 had a distinctly faster increase and lower decrease in comparison with var. K-851 and retained significantly higher value at fourth day of drought stress. On fourth day of stress, Chl a content reduced to about 1.62-fold in var. RMG 268, 2.15-fold in var. K-851, and 2.30-fold in var. Anand, as compared to control plants. Chl b contents were 1.80-fold in var. RMG 268, 2.25-fold in var. K-851, and 2.30-fold lower in var. Anand as compared to control plants. Thus, it is obvious that a pronounced decrease was observed in var. Anand. An overall increase in the Chl a:b ratio was seen at Day 4 of stress and the differences were statistically significant for all the varieties. However in var. RMG 268, increase was observed in comparison to control at all days of drought stress.
Table 2. Photosynthetic pigment contents for variety RMG 268, variety K-851, and variety Anand of Vigna radiata at day 1, day 2, day 3, and day 4 of drought stress.
In contrast, the Car content was found to increase progressively with the increase of drought increments (). HPLC analysis revealed that compared to the amounts present in the respective control unstressed plants (Day 0), the quantity of β-carotene in stressed plants was always higher, reaching maxima (4.3-, 3-, 2-fold in var. RMG 268, K-851, and Anand, respectively) at Day 4. As compared to their respective controls (Day 0), lutein contents were 3.93-, 3.62-, and 1.80-folds higher in var. RMG 268, var. K-851, and var. Anand, respectively. Further, increase of Car content in var. RMG 268 and var. K-851 was higher than var. Anand.
Table 3. Relative concentrations of β-carotene and lutein + zeaxanthin obtained through HPLC in two-week-old plants of three varieties of Vigna radiata exposed to drought stress.
3.3. Effects on D1 core protein
We have primarily focused on the rate of D1 protein degradation in V. radiata plants under drought stress. D1 protein (32 kDa) was detected after western blotting on the nitrocellulose membrane (). The level of D1 protein decreased during photoinhibition in drought-stressed plants. Intensity of D1 protein declined by 25%, 17.8%, and 15.2% in stressed leaves of var. Anand, var. K-851, and var. RMG 268. Stressed plants of var. Anand were more susceptible to photoinhibition, as compared to var. K-851 and var. RMG 268.
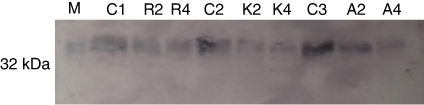
3.4. Effects on proline content
The free proline content significantly increased in stressed plants of all vars. () of V. radiata. Incipient drought increased proline levels by 36, 23, and 6 times at day 4 in var. RMG 268, var. K-851, and var. Anand, respectively. The control plants maintained lower concentrations at all days of stress.
4. Discussion
Photosynthesis is inhibited under drought stress (Liu et al. Citation2006; Zlatev Citation2009) due to the perturbation of the biochemical processes involving the PSII reaction centers and the concomitant uncoupling of noncyclic photophosphorylation (Yordanov et al. Citation2000), which results in an imbalance between the generation and utilization of electrons, thereby resulting in decrease of electron transport rate. In drought-tolerant Norway spruce seedlings, the photosynthetic quantum efficiency decreases several days later than in less tolerant seedlings in the progression of drought treatments (Blödner et al. Citation2005). Despite the decrease in the photochemical efficiency of PSII, var. RMG retained highest ETRmax, PPFDsat, and values, followed by var. K-851. Var. Anand showed strongest decrease in photosynthetic capacity under drought stress. These decreases may be due to a direct dehydration effect on Rubisco, reflecting an increase in Rubisco hydrolysis, since the amount of Rubisco largely determines photosynthesis (Lawlor Citation2002) and/or a decline in its catalytic ability.
A decrease of total Chl with drought stress in V. radiata varieties implies a lowered capacity for light harvesting. Such retardation in the content of photosynthetic pigments was attributed to the ultrastructural deformation of plastids including the protein membranes forming the thylakoids, which in turn causes untying of PSII that captures photons; so its efficiency declined (Yang et al. Citation2001), thus causing decline in electron transfer (Kannan & Kulandaivelu Citation2011). This reduction may occur due to stress-induced impairment in pigment biosynthetic pathways or in pigment degradation. The decrease in Chl content is a commonly observed phenomenon under drought stress (Bijanzadeh & Emam Citation2010; Din et al. Citation2011). More decrease in var. Anand indicates that it is more sensitive to water deficits than var. RMG 268 and var. K-851. Chl b is synthesized from Chl a (chlorophyllide a) by chlorophyllide a oxygenase (Tanaka & Tanaka Citation2005). When the availability of Chl b exceeds the amount needed for import and stabilization of light-harvesting antenna proteins, surplus Chl b molecules seem to trigger chlorophyllide a oxygenase protein degradation (Yamasato et al. Citation2005) and thereby suppress Chl b biosynthesis, resulting in an overall increase in the Chl a:b ratio (Jain et al. Citation2010).
Plants have evolved a variety of protective mechanisms against the ROS-induced damage to cellular components, such as the dissipation of excess excitation energy and the synthesis of protective pigments, such as Car and anthocyanins (Efeoglu et al. Citation2009). This protection against the ‘attack’ on the integrity of the chloroplast membrane as observed by a higher increase in var. RMG 268 and var. K-851 is very important for the maintenance of the photosynthetic activity as a whole (Dwivedi et al. Citation1995). Furthermore, a small fraction of non-protein-bound Car serves as antioxidant in the lipid phase of photosynthetic membranes (Havaux et al. Citation2004; Gruszecki & Strzaka Citation2005). Both lutein and β-carotene (Farooq et al. Citation2009) are capable of acting as energy acceptors, thereby quenching excited Chl molecules.
A number of studies conducted in vivo have shown that drought stress causes considerable damage to the OEC coupled with PSII (Kumari & Sharma Citation2010; Kawakami et al. Citation2009) as well as degradation of D1 polypeptide, leading to the inactivation of the PSII reaction center (Zlatev Citation2009). In an earlier study, we have also demonstrated that salt exposure in Brassica juncea varieties causes reduction in D1 protein (Mittal et al. Citation2012). Previous studies have shown that translation elongation of mRNA and membrane insertion of new D1 protein depend on the availability of assembly partners (Zhang et al. Citation1999) and ligation of Chl a (He & Vermaas Citation1998) and β-carotene (Trebst & Depka Citation1997).
Proline hyperaccumulation, a frequently reported phenomenon in the plant kingdom, is considered an adaptive strategy of plants to cope with a variety of stresses. (Singh et al. Citation2010; Tripathi et al. Citation2013). Proline influences protein solvation and preserves the quaternary structure of complex proteins, maintains membrane integrity under dehydration stress, and reduces photoinhibition (Demiral & Türkan Citation2004). Furthermore, it also contributes to stabilizing subcellular structures, scavenging free radicals (Tripathi & Gaur Citation2004), and buffering cellular redox potential under stress conditions (Ashraf & Foolad Citation2007). Progressive drought stress induced a higher accumulation of proline in var. RMG 268 and var. K-851.
5. Conclusions
Our results suggest that exposure of V. radiata to drought stress inhibits PSII activity and affects the energy transfer processes by inducing alterations in thylakoid membrane protein D1. Plants of var. RMG 268 and var. K-851 were tolerant to drought stress as judged by leaf Chl content, photosynthetic performance, thylakoid core protein, D1, and accumulation of osmoprotectants, compared to var. Anand. PSII activity in var. RMG 268 and var. K-851 was more efficiently protected than var. Anand, as indicated by fluorescence measurements. Progressive drought stress significantly reduced pigment content, increased Car contents, and accumulated free proline. Var. Anand can be considered as drought sensitive. The information obtained from current study may be used to breed drought-tolerant materials. Most importantly, our results emphasize the need to consider Car biosynthesis in the course of photoprotection in relation to pigment dynamics during the D1 repair cycle following PSII photoinactivation.
Acknowledgments
The authors gratefully acknowledge the assistance of Prof. E. M. Aro, University of Turku, Finland, for the kind donation of D1 primary antibody.
References
- Arnon DI. 1949. Copper enzymes in isolated chloroplasts. Polyphenoloxidase in Beta vulgaris. Plant Physiol. 24:1–15. 10.1104/pp.24.1.1
- Ashraf M. 2009. Biotechnological approach of improving plant salt tolerance using antioxidants as markers. Biotechnol Adv. 27:84–93. 10.1016/j.biotechadv.2008.09.003
- Ashraf M, Foolad MR. 2007. Roles of glycinebetaine and proline in improving plant abiotic stress resistance. Environ Exp Bot. 59:206–216. 10.1016/j.envexpbot.2005.12.006
- Ashraf M, Harris PJC. 2004. Potential biochemical indicators of salinity tolerance in plants. Plant Sci. 166:3–16. 10.1016/j.plantsci.2003.10.024
- Ashraf M, Harris PJC. 2013. Photosynthesis under stressful environments: an overview. Photosynthetica. 51:163–190. 10.1007/s11099-013-0021-6
- Bates LS, Waldren RP, Teare ID. 1973. Rapid determination of free proline for water-stress studies. Plant Soil. 39:205–207. 10.1007/BF00018060
- Bijanzadeh E, Emam Y. 2010. Effect of defoliation and drought stress on yield components and chlorophyll content of wheat. Pak J Biol Sci. 13:699–705. 10.3923/pjbs.2010.699.705
- Blödner C, Skroppa T, Johnson O, Polle A. 2005. Freezing tolerance in two Norway spruce (Picea abies [L.] Karst.) progenies is physiologically correlated with drought tolerance. Plant Physiol. 162:549–558. 10.1016/j.jplph.2004.09.005
- Chakraborty N, Tripathy BC. 1992. Involvement of singlet oxygen in 5-aminolevulinic acid induced photodynamic damage of cucumber (Cucumis sativus L.) chloroplasts. Plant Physiol. 98:7–11. 10.1104/pp.98.1.7
- Demiral T, Türkan I. 2004. Does exogenous glycinebetaine affect antioxidative system of rice seedlings under NaCl treatment? Plant Physiol. 161:1089–1100. 10.1016/j.jplph.2004.03.009
- Din J, Khan SU, Ali I, Gurmani AR. 2011. Physiological and agronomic response of canola varieties to drought stress. J Anim Plant Sci. 21:78–82.
- Dwivedi U, Sharma M, Bhardwaj R. 1995. Down regulation of photosynthesis in Artabotrys hexapetatus by high light. Photosyn Res. 46:393–397. 10.1007/BF00032293
- Efeoglu B, Ekmekçi Y, Çiçek N. 2009. Physiological responses of three maize cultivars to drought stress and recovery. S Afr J Bot. 75:34–42. 10.1016/j.sajb.2008.06.005
- Farooq M, Wahid A, Kobayashi N, Fujita D, Basra SMA. 2009. Plant drought stress: effects, mechanisms and management. Agron Sustain Dev. 29:185–212. 10.1051/agro:2008021
- Fracheboud Y, Leipner J. 2003. The application of chlorophyll fluorescence to study light, temperature and drought stress. In: DeEll JR, Tiovonen PMA, editors. Practical applications of chlorophyll fluorescence in plant biology. Boston: Kluwer Academic Publishers; p. 125–150.
- Garg B, Lata C, Prasad M. 2012. A study of the role of gene tamyb2 and an associated snp in dehydration tolerance in common wheat. Mol Biol Rep. 39:10865–10871. 10.1007/s11033-012-1983-3
- Garg B, Puranik S, Misra S, Tripathi BN, Prasad M. 2013. Transcript profiling identifies novel transcripts with unknown functions as primary response components to osmotic stress in wheat (Triticum aestivum L.). Plant Cell Tiss Organ Cult. 113:91–101. 10.1007/s11240-012-0254-2
- Gill SS, Tuteja N. 2010. Reactive oxygen species and antioxidant machinery in abiotic stress tolerance in crop plants. Plant Physiol Biochem. 48:909–930. 10.1016/j.plaphy.2010.08.016
- Gruszecki WI, Strzaka K. 2005. Carotenoids as modulators of membrane physical properties. Biochim Biophys Acta. 1740:108–111. 10.1016/j.bbadis.2004.11.015
- Havaux M, Dall'Osto L, Cuine S, Giuliano G, Bassi R. 2004. The effect of zeaxanthin as the only xanthophyll on the structure and function of the photosynthetic apparatus in Arabidopsis thaliana. J Biol Chem. 279:13878–13888. 10.1074/jbc.M311154200
- He Q, Vermaas W. 1998. Chlorophyll a availability affects psbA translation and D1 precursor processing in vivo in Synechocystis sp. PCC 6803. Proc Natl Acad Sci USA. 95:5830–5835. 10.1073/pnas.95.10.5830
- Hoagland DR, Arnon DI, editors. 1950. The water culture method for growing plants without soil. Circular No. 347: California Agriculture Experiment Station. Berkeley: The College of Agriculture, University of California; p. 1–32.
- Jain M, Tiwary S, Gadre R. 2010. Sorbitol-induced changes in various growth and biochemical parameters in maize. Plant Soil Environ. 56:263–267. 10.1111/j.1747-0765.2010.00448.x
- Kannan ND, Kulandaivelu G. 2011. Drought induced changes in physiological, biochemical and phytochemical properties of Withania somnifera Dun. J Med Plants Res. 5:3929–3935.
- Kawakami K, Umenab Y, Kamiyab N, Shen J. 2009. Location of chloride and its possible functions in oxygen-evolving photosystem II revealed by X-ray crystallography. Proc Natl Acad Sci USA. 106:8567–8572. 10.1073/pnas.0812797106
- Knecht DA, Dimond RL. 1983. Visualization of antigenic proteins on Western blots. Anal Biochem. 136:180–184. 10.1016/0003-2697(84)90321-X
- Kumari N, Sharma V. 2010. Stress-mediated alteration in V-ATPase and V-PPase of Butea monosperma. Protoplasma. 245:125. 10.1007/s00709-010-0153-5
- Kumari N, Sharma V, Mikosch M, Unfried C, Gessler A, Fischer-Schliebs E, Luettge U. 2005. Seasonal photosynthetic performance and nutrient relations of Butea monosperma TAUB in comparison to two other woody species of a seasonal deciduous forest in SE-Rajasthan and to planted trees in the area. Ind J For. 26:116–126.
- Laemmli UK. 1970. Cleavage of structural proteins during the assembly of the head of bacteriophage T4. Nature. 227:680–685. 10.1038/227680a0
- Lawlor DW. 2002. Limitation to photosynthesis in water-stressed leaves: stomata vs. metabolism and the role of ATP. Ann Bot. 89:871–885. 10.1093/aob/mcf110
- Liu N, Ko S, Yeh KC, Charng Y. 2006. Isolation and characterization of tomato Hsa32 encoding a novel heat-shock protein. Plant Sci. 170:976–985. 10.1016/j.plantsci.2006.01.008
- Maksup S, Roytrakul S, Supaibulwatana K. 2014. Physiological and comparative proteomic analyses of Thai jasmine rice and two chick cultivars in response to drought stress. J Plant Interact. 9:43–55.
- Maxwell K, Johnson GN. 2000. Chlorophyll fluorescence—a practical guide. J Exp Bot. 50:659–668. 10.1093/jexbot/51.345.659
- Mittal S, Kumari N, Sharma V. 2012. Differential response of salt stress on Brassica juncea: photosynthetic performance, pigment, proline, D1 and antioxidant enzymes. Plant Physiol Biochem. 54:17–26. 10.1016/j.plaphy.2012.02.003
- Mudgal V, Madaan N, Mudgal A. 2010. Biochemical mechanisms of salt tolerance in plants: a review. Int J Bot. 6:136–143. 10.3923/ijb.2010.136.143
- Muscolo A, Sidari M, Anastasi U, Santonoceto C, Maggio A. 2014. Effect of PEG-induced drought stress on seed germination of four lentil genotypes. J Plant Interact. 9:354–363.
- Pocock T, Krol M, Huner NPA. 2004. The determination and quantification of photosynthetic pigments by reverse phase high-performance liquid chromatography, thin-layer chromatography and spectrophotometry. In: Carpentier R, editor. Photosynthesis research protocols. Totowa: Humana Press; p. 137–148.
- Postel SL. 2000. Entering an era of water scarcity: the challenges ahead. Ecol Applicat. 100:941–948. 10.1890/1051-0761(2000)010[0941:EAEOWS]2.0.CO;2
- Rafieishirvan M, Asgharipur MR. 2009. Yield reaction and morphological characteristics of some mungbean genotypes to drought stress. J Modern Agric Knowl. 5:67–76.
- Rascher U, Liebig M, Lüttge U. 2000. Evaluation of instant light-response curves of chlorophyll fluorescence parameters obtained with a portable chlorophyll fluorometer on site in the field. Plant Cell Environ. 23:1397–1405. 10.1046/j.1365-3040.2000.00650.x
- Ribeiro RV, Santos MG, Souza GM, Machado EC, Oliveira RF, Angelocci LR, Pimentel C. 2004. Environmental effects on photosynthetic capacity of bean genotypes. Pesq Agropec Bras. 39:615–623. 10.1590/S0100-204X2004000700001
- Rivas JDI, Abadia A, Abadia J. 1989. A new reversed phase- HPLC method resolving all major higher plant photosynthetic pigments. J Plant Physiol. 91:190–192. 10.1104/pp.91.1.190
- Roháček K. 2002. Chlorophyll fluorescence parameters: the definitions, photosynthetic meaning, and mutual relationships. Photosynthetica. 40:13–29. 10.1023/A:1020125719386
- Schreiber U, Bilger W, Neubauer C. 1995. Chlorophyll fluorescence as a noninstructive indicator for rapid assessment of in vivo photosynthesis. In: Schulze ED, Caldwell MM, editors. Ecophysiology of photosynthesis. Berlin: Springer Verlag; p. 49–70.
- Singh V, Bhatt I, Aggarwal A, Tripathi B, Munjal A, Sharma V. 2010. Proline improves copper tolerance in chickpea (Cicer arientinum). Protoplasma. 245:173–181. 10.1007/s00709-010-0178-9
- Tanaka R, Tanaka A. 2005. Effects of chlorophyllide a oxygenase overexpression on light acclimation in Arabidopsis thaliana. Photosynth Res. 85:327–340. 10.1007/s11120-005-6807-z
- Trebst A, Depka B. 1997. Role of carotene in the rapid turnover and assembly of photosystem II in Chamydomonas reinhardtiis. FEBS Lett. 400:359–362. 10.1016/S0014-5793(96)01419-6
- Tripathi BN. 2010. Stress metabolism of plants. Protoplasma. 245:1. 10.1007/s00709-010-0196-7
- Tripathi BN, Gaur JP. 2004. Relationship between copper- and zinc-induced oxidative stress and proline accumulation in Scenedesmus sp. Planta. 219:397–404. 10.1007/s00425-004-1237-2
- Tripathi BN, Mehta SK, Amar A, Gaur JP. 2006. Oxidative stress in Scenedesmus sp. during short- and long-term exposure to Cu2+ and Zn2+. Chemosphere. 62:538–544. 10.1016/j.chemosphere.2005.06.031
- Tripathi BN, Singh V, Ezaki B, Sharma V, Gaur JP. 2013. Mechanism of Cu- and Cd-induced proline hyperaccumulation in Triticum aestivum (wheat). J Plant Growth Regul. 32:799–808. 10.1007/s00344-013-9343-7
- Yamasato A, Nagata N, Tanaka R, Tanaka A. 2005. The N-terminal domain of chlorophyllide a oxygenase confers protein instability in response to chlorophyll b accumulation in Arabidopsis. Plant Cell. 17:1585–l597. 10.1105/tpc.105.031518
- Yang J, Zhang J, Wang Z, Zhu Q, Liu L. 2001. Water deficit-induced senescence and its relationship to the remobilization of pre-stored carbon in wheat during grain filling. Agron J. 93:196–206. 10.2134/agronj2001.931196x
- Yordanov I, Velikova V, Tsonev T. 2000. Plant response to drought, acclimation, and stress tolerance. Photosynthetica. 38:171–186. 10.1023/A:1007201411474
- Zhang XW, Zhang YM, Chen F. 1999. Application of mathematical models to the determination optimal glucose concentration and light intensity for mixotrophic culture of Spirulina platensis. Process Biochem. 34:477–481. 10.1016/S0032-9592(98)00114-9
- Zlatev Z. 2009. Drought-induced changes in chlorophyll fluorescence of young wheat plant. Biotechnol. 23:437–441.