Abstract
Allelopathy has been considered not only as an environmentally friendly approach for weed control but also a potential reason causing autotoxicity in crop production. In this study, the responses of seeds of lettuce, wheat, rice, clover broomrape (CB), and sunflower broomrape (SB) to the root exudates of rice cultivars were studied. Lettuce germination was promoted by root exudates of Yliangyou 3218 and I-Kung-Pao. Wheat seedling growth was inhibited by all nine rice species. I-Kung-Pao and Ganxin 203 exerted greater autotoxicity than other cultivars. Yongyou 15 and I-Kung-Pao induced the highest germination rate of CB, while Yongyou 13, Zhongzao 22, and I-Kung-Pao induced the highest germination rate of SB. A significantly correlation was noted between germination-inducing ability on broomrape seeds and allelopathic effects on target plants. It is suggested that using broomrape seeds germination is a better receptor for the identification of rice allelopathic potential.
Introduction
Allelopathy refers to the effect of one plant species on another through the release of chemical compounds into the environment (Weih et al. Citation2008), which may be either growth promoting (i.e. synergistic) or inhibiting (i.e. antagonistic), depending on the compounds released and target plants. Allelopathy can play a beneficial role in various cropping systems, including mixed cropping, multiple cropping, cover cropping, crop rotation, and minimum or no-till systems (Ben et al. Citation2001). The potential use of allelopathy as a natural means for weed suppression in agroecosystems has attracted the interest of researchers for a long time (Putnam Citation1986).
About 3.5% of rice germplasm tested in laboratory (Fujii Citation1992; Navarez & Olofsdotter Citation1996) and field experiments (Dilday et al. Citation1998; Olofsdotter et al. Citation1999) has allelopathic potential against weeds. A number of compounds, such as phenolic acids, fatty acids, phenylalkanoic acids, hydroxamic acids, terpenes, indoles, and the labdane-related diterpenoid momilactones, have been identified as potential rice allelochemicals (Rimando & Duke Citation2003; Khanh et al. Citation2007; Kato-Noguchi & Peters Citation2013). Allelopathic potential against a broad spectrum of target weeds would be a desirable trait in a rice cultivar. Preliminary genetic studies indicate that allelopathy in rice is a quantitatively inherited feature (Courtois & Olofsdotter Citation1998; Dilday et al. Citation1998; Jebsen et al. Citation2001; Olofsdotter Citation2001). Therefore, allelopathic potential must be selected at the early stages of plant-breeding programs, as a large amount of allelopathic traits could be lost during artificial selection for increased yield. However, most of the allelopathy studies on rice were mainly focused on the relationship between rice and barnyardgrass (Echinochloa crus-galli (L.) Beauv.), and the allelochemicals identified were also based on suppression rate of barnyardgrass growth. The allelopathic effects of rice varied depending on target plants (Xuan et al. Citation2005; Kato-Noguchi et al. Citation2011; Kato-Noguchi et al. Citation2013). Thus, it is essential to analysis the allelopathic interactions between rice and other plant species.
Broomrape (Orobanche spp.) is an obligate holo-parasite that infests many broadleaf crops and weeds, causing severe yield loss in crop products (Parker & Riches Citation1993). The germination of broomrape seeds requires chemical compounds secreted by potential host roots. It was reported that rice was widely used as a trap crop in broomrape control in Africa (Parker & Riches Citation1993), indicating signal communications existed between rice and broomrape seeds. As mentioned above, some of rice cultivars exhibited significant allelopathy in field. Thus, there is a question that whether the rice cultivars which induced high germination rate of broomrape seeds are with high allelopathic potential inhibiting associated plant species growth.
Lettuce (Lactuca sativa L.) and wheat (Triticum aestivum L.) are widely used in allelopathy studies because the germination of the seeds are high, sensitive, and stable (Chou & Chung Citation1974; Olofsdotter Citation2001). By using seeds of lettuce and wheat as targets, the allelopathic potentials of varied plant species were evaluated (Kato-Noguchi Citation1999; Abdelgaleil & Hashinaga Citation2007; Kato-Noguchi et al. Citation2009; Zeng et al. Citation2009). In this article, we conducted an allelopathy study on root exudates of nine rice cultivars using lettuce, wheat and rice seeds as receptors and followed with germination test of seeds of sunflower broomrape (SB; Orobanche cumana Wallr.) and clover broomrape (CB; Orobanche minor Sm.) treated with the exudates. The purpose of this study is to determine if there is a relationship between allelopathy and broomrape germination-inducing ability of rice root exudates.
Materials and methods
Plant materials and chemicals
Nine rice cultivars were used in the experiment: Xiushui 417 (traditional cultivar, nonallelopathy), Yongyou 13 (hybrid rice), Yongyou 15 (hybrid rice), Zhongzao 22 (traditional cultivar), Zhongzu 14 (traditional cultivar), Zhunliangyou 527 (hybrid rice), Yliangyou 3218 (hybrid rice), Ganxin 203 (hybrid rice), and I-Kung-Pao (native cultivar from Taiwan, high-allelopathy). Seeds for these cultivars were provided by Dr Yongjun Zhou of the China National Rice Research Institute. CB (O. minor) seeds were collected from the fields of Utsunomiya University of Japan, and SB (O. cumana) seeds were collected under the infested sunflower fields in the Xinjiang Uygur Autonomous Region of China, respectively. Lettuce (L. sativa L.) and wheat (T. aestivum L. var. No. 22 Xiaoyan) seeds were purchased from local seed company. Synthetic strigolactone, GR24, was supplied by Professor B. Zwanenburg, Radboud University, The Netherlands.
Collection of rice root exudates
Seeds used in the experiment were uniformly surface-sterilized by immersing in 1% NaClO for 3 min, in 75% (V/V) ethanol for 3 min, and then rinsed with autoclaved distilled water five times and air-dried on a clean bench. Before use, the rice seeds were germinated on moistened filter paper in Petri dishes for 72 h at 25°C in the dark. After germination, 224 rice seedlings were transferred to a strainer (36 cm × 26 cm × 8 cm) lined with a sheet of gauze that had been moistened by placing it in a slightly larger container (42 cm × 30 cm × 11 cm) containing 4 L of deionized water. The seedlings were placed in a growth chamber with a 12 h photoperiod at 120 µmol photons m−2 s−1 at 25°C. The deionized water medium was replaced every two days. On day 7, the deionized water was replaced with half strength Tadano and Tanaka medium (Tadano & Tanaka Citation1980) containing 2.43 mmol L−1 N, 0.16 mmol L−1 P, 1 mmol L−1, 1 mmol L−1 Ca, 2.43 mmol L−1 Mg, and 1 mmol L−1 4-morpholine ethanesulfonic acid (MES). The pH of the culture media was adjusted to 6.0 with NaOH. The Tadano and Tanaka medium was replaced every two days. On day 15, the Tadano and Tanaka medium was replaced with deionized water containing one mmol L−1 CaCl2. The deionized water plus CaCl2 medium was circulated through an activated charcoal filter with an aquarium pump. The rice was grown on this medium for one week. At the same time, the root exudates trapping system without rice seedling was used as control. The medium and the charcoal filters were replaced every two days.
Root exudates adsorbed onto the charcoal filters were eluted with acetone. The acetone was removed by vacuum evaporation in a rotary evaporator at 40°C. The residue was dissolved in 50 mL distilled water, and then filtered three times with 50 mL ethyl acetate (EtOAc). The EtOAc extracts were combined, dried over anhydrous Na2SO4, and then evaporated to dryness under vacuum at 40°C using a rotary evaporator. The residues were dissolved in 5 mL acetone and then stored in sealed glass vials at 4°C. The stored residues were diluted with distilled water to final concentration of 100, 10 and 1 mg L−1 before use. By using a pH meter (Mettler-Toledo, Swizerland) and a vapor pressure osmometer (Model 5600, Wescor, Logan, UT, USA), the pH and the osmolality of the aliquots of root exudates of nine rice varieties were characterized at 100, 10, and 1 mg L−1, respectively. However, the values of pH were between 6.00 and 6.38 and osmolality were between 8 and 11 mmol kg−1 of these aliquots among rice varieties. So it was believed that the allelopathic effects were the major factor influencing the germination of target plants in this experiment.
CB and SB germination assays
CB and SB seeds must be conditioned before becoming receptive to a germination stimulant. To condition the seeds, 5 mL aliquots of gibberellin (GA3, 10−4 mol L−1) were applied into Petri dishes (9 cm diameter) lined with double-filter papers. Glass fiber filter disks (8 mm diameter, Whatman GF/A) were laid on the filter paper and then about 30–50 broomrape seeds were sown on each glass fiber disk. The Petri dishes with CB seeds were sealed with parafilm and incubated at 25°C in the dark for six days as described by Parker et al. (Citation1977), whereas the Petri dishes with SB seeds were conditioned for three days under the same condition.
Aliquots (20 µL) of rice root exudates were applied to the glass fiber disks with conditioned broomrape seeds in Petri dishes. Individual treatments were replicated three times. The Petri dishes were sealed and incubated at 25°C. Germination rates were determined with a microscope after 10 days incubation. Broomrapes seeds treated with GR24 and distilled water were used as positive and negative controls (Mangnus et al. Citation1992).
Lettuce, wheat, and rice germination assays
Wheat (T. aestivum L.), lettuce (L. sativa L.) and rice (Oryza sativa L.) seeds were surface-sterilized by immersion for 2 min in 1% (v/v) sodium hypochlorite followed by immersion for 2 min in 70% (v/v) ethanol, then the seeds were washed with sterile distilled water. Lettuce seeds directly placed in Petri dishes (12.5 cm in diameter) on two layers of Whatman No. 1 filter paper, while seeds of wheat and rice were presoaked with water for about 72 h until the radicle was just visible, and then placed in Petri dishes on two layers of Whatman No. 1 filter paper. The Petri dishes and filter paper had been sterilized in a high-pressure steam sterilizer for 30 min. Each dish contained 20 wheat, lettuce, or rice seeds. A 3-mL aliquot of the test solution (100 mg L−1, 10 mg L−1, or 1 mg L−1 dilution of rice root exudates and system control) was added to the filter paper. Each treatment was replicated three times. The seeds were incubated at 25°C. Germination rates of lettuce were determined after 24 h, by counting the number of lettuce seeds with radicles > 1 mm long. Radicle and hypocotyl lengths of lettuce seedlings were measured after 48 h (Leather & Einhellig Citation1986). Coleoptile length of wheat seedling and rice seedling were measured. Total radicle length of wheat seedling or rice seedling was determined by counting up the length of each radicle within a seedling, whereas the max radicle length was the length of the longest radicle of the seedling.
The allelopathic response index (RI) was calculated using Equation (1) (Williamson & Richardson Citation1988):
The average response index (ARI) of test species to rice root exudates was calculated using Equation (2):
Data analysis
Data were processed using Excel 2010 and SPSS 18.0 software (SPSS Inc., Chicago, IL, USA). Tukey honestly significant difference (HSD) tests were used to separate the means. The association among RIs of three target plants to rice root exudates (100 and 10 mg L−1) and germination rates of both CB and SB induced by rice root exudates (100 mg L−1) were carried out using Pearson's correlation coefficients.
Results
Response of lettuce germination to rice root exudates
The effect of rice root exudates on the germination and growth of lettuce seedlings varied significantly depending on the concentration of the root exudates and cultivar (). At 100 mg L−1, exudates of five rice cultivars (Zhongzu 14, Zhunliangyou 527, Ganxin 203, Zhongzao 22, Xiushui 417) significantly reduced lettuce germination. The inhibitory effects decreased when the root exudates were diluted to 10 and 1 mg L−1. Root exudates of three cultivars (Yliangyou 3218, Yongyou 13, I-Kung-Pao) tended to increase lettuce germination. However, the increases were not statistically significant.
Table 1. Response of lettuce seeds to rice root exudates.
Among rice cultivars on-test, lettuce radicle growth was strongly reduced by Zhongzu 14 (67% of control) at 100 mg L−1, Zhunliangyou 527 (31% of control) at 10 mg L−1, while Zhongzu 14, Ganxin 203, and Zhongzao 22 significantly reduced radicle growth at 1 mg L−1. However, root exudates of I-Kung-Pao and Yliangyou 3218 tended to increase lettuce radicle growth.
The effect of root exudates on lettuce hypocotyl growth was similar to that on radicle growth. All rice cultivars showed inhibitory effects at 100 mg L−1, especially for Zhongzu 14, Zhunliangyou 527, Ganxin 203, Zhongzao 22, and Xiushui 417. The root exudates of most cultivars still inhibited lettuce hypocotyl growth at 10 mg L−1, but the effect declined dramatically with concentration further decreased. However, the root exudates of I-Kung-Pao promoted lettuce hypocotyl growth by 56% at 1 mg L−1.
The ARIs, based on the data of , varied significantly with respect to cultivars (). At 1–100 mg L−1, the indexes of Zhongzu 14, Ganxin 203, Zhunliangyou 527, Zhongzao 22, Xiushui 417 were negative, indicating that the root exudates of these cultivars inhibited lettuce growth, whereas Yliangyou 3218, I-Kung-Pao were positive, showing these two cultivars promoted lettuce growth. The ARI of Yongyou 13 and Yongyou 15 were negative at 100 mg L−1, but positive at 1 and 10 mg L−1, informing that the concentration influenced the response of lettuce to rice root exudates.
Response of wheat germination to rice root exudates
The root exudates of the rice cultivars inhibited both wheat radicle and coleoptile growth (). The inhibition on max radicle elongation ranged between 9.7% and 26.1% of control, and the inhibitory effect decreased as concentration declined. At 100 mg L−1, the inhibitory effects varied significantly among the cultivars, with Xiushui 417 having the greatest inhibitory effect on wheat max radicle growth. Diluted to 10 mg L−1, the exudates still exhibited an inhibitory effect on wheat max radicle elongation, but the differences between cultivars vanished. However, root exudates of Zhongzao 22 at 1 mg L−1 still had significant inhibitory effect on wheat max radicle growth.
Table 2. Response of wheat seeds to rice root exudates.
Same as the response of wheat max radicle, total radicle length of wheat seedlings was also affected by rice root exudates. At 100 mg L−1, root exudates of Xiushui 417 reduced wheat radicle growth by 53.7%, whereas Yongyou 13 showed little inhibitory effect. At one mg L−1, root exudates of Zhongzu 14 and Zhunliangyou 527 reduced wheat radicle elongation much more than the other cultivars did. Compared with the influence on radicle length, the inhibitory effect of rice root exudates on wheat coleoptile growth was less. Some rice cultivars even showed promoting effects, especially I-Kung-Pao, which promoted wheat coleoptile growth at 1 and 10 mg L−1.
The ARI of all rice cultivars on wheat were negative because of the great inhibitory effect of root exudates on wheat radicle growth. It indicated that the effect of rice exudates was predominantly inhibitory to wheat. The ARI ranged between –0.39 and –0.03, with Xiushui 417 the lowest at 100 mg L−1 and Zhunliangyou 527 the lowest at one and 10 mg L−1. As the concentration of root exudates declined, the inhibitory effects of Yongyou 15 and I-Kung-Pao decreased, but Yongyou 13 and Zhunliangyou 527 were constant ().
Response of rice germination to rice root exudates
Effects of root exudates of nine rice cultivars on rice seeding growth varied significantly (). At 100 mg L−1, root exudates of Zhongzu 14 increased rice radicle length by 28%, whereas Zhunliangyou 527 and Yliangyou 3218 reduced rice coleoptile elongation by 23.3% and 30.0%, respectively. As the root exudates diluted to 1 mg L−1, Zhongzu 14 and Yongyou 13 significantly increased rice radicle length. The ARI of Zhongzao 22, Yongyou 13, and Yongyou 15 on rice was positive, while Ganxin 203 was negative at 1–100 mg L−1. The ARI of all cultivars were positive at 1 and 10 mg L−1, except I-Kung-Pao and Ganxin 203 (), indicating that these two cultivars should be with higher allelopathic potential on rice itself than other cultivars.
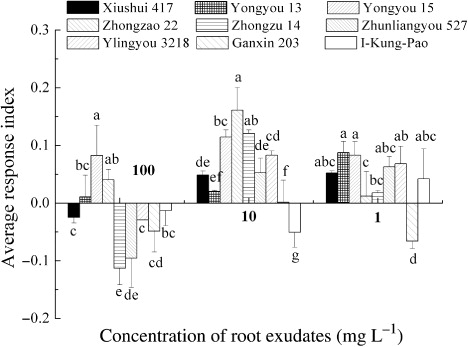
Table 3. Response of rice seeds to rice root exudates.
Effect of rice root exudates on broomrape germination
Distilled water and the residues from the control of root exudates trapping system did not stimulate CB or SB germination (data not shown), whereas GR24 induced the highest germination rate of CB (89.1%) and SB (68.3%). Germination of CB treated with rice root exudates varied between 0 and 79.7%, depending on the rice cultivar and root exudates concentration ( top). At 100 mg L−1, exudates of five cultivars induced CB germination greater than 60%, with exudates of Yongyou 15 and I-Kung-Pao inducing the highest germination rate. Germination declined when CB seeds were treated with 10 mg L−1 root exudates. At the concentration of 10 mg L−1, root exudates of Xishui 417 induced the highest CB germination (45.0%). The root exudates at 1 mg L−1 did not induce CB germination (data not shown).
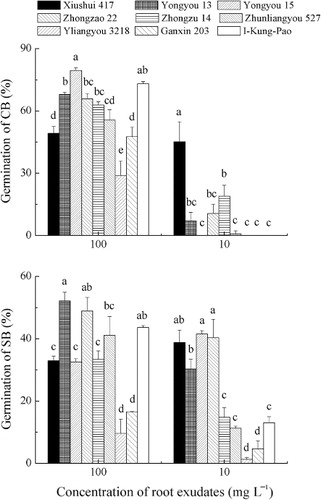
Germination of SB treated with rice root exudates ranged between 0 and 52.0% ( bottom). Exudates of four cultivars at concentration of 100 mg L−1 induced SB germination greater than 40%, with exudates of Yongyou 13, Zhongzao 22, and I-Kung-Pao, inducing the highest germination rate. Compared to the germination of CB, germination of SB was lower at 100 mg L−1, but higher at 10 mg L−1 root exudates. Yongyou 15 and Zhongzao 22 induced the highest SB germination rates among the cultivars at 10 mg L−1. The root exudates at 1 mg L−1 did not induce SB germination (data not shown).
Relationship between ARIs of lettuce, wheat, rice, and broomrape germination
There was no significant liner relation among ARIs of lettuce, wheat, and rice. But ARI of rice (100 mg L−1) and ARI of wheat (10 mg L−1) was significantly positive correlated with the germination rate of CB (). Between germination rates of CB and that of SB, there was also a significant correlation (r = 0.749, p < 0.05).
Table 4. Pearson's correlation coefficients for ARI of lettuce, wheat, rice, and germination rates of seeds of CB and SB.
Discussion
Lettuce germination test is widely used in allelopathy studies. By using lettuce as a target, Uddin et al. (Citation2013) screened the potent allelochemicals of Phargmites australis, Mahmood et al. (Citation2013) investigated the effects of UV exposure on rice allelopathic potentials. Our results showed that high concentrations of rice root exudates inhibited lettuce growth, whereas low concentrations promoted, indicating that root exudates of on-test rice cultivars contained allelochemicals affecting lettuce germination. And a significant correlation between ARIs of lettuce to rice root exudates at given concentrations () evinced that lettuce used as target plant in allelopathy study on rice could lead to stable results, this agreed with previous studies (Fujii Citation1992; Maneechote & Krasaesindhu Citation1996). Among the rice cultivars on-test, lettuce germination was promoted by Yliangyou 3218 and I-Kung-Pao, inhibited by Zhongzu 14, Ganxin 203, Zhunliangyou 527, Zhongzao 22, and Xiushui 417 at concentrations of 10–100 mg root exudates per L (). However, Li et al. (Citation2004) identified I-Kung-Pao as a rice germplasm with allelopathic potential according to its strong inhibitory effect on barnyardgrass in paddy filed and the inhibitory effect of leaf water extracts on lettuce in lab. In Ebana et al.'s (Citation2001) and Kanesawa et al.'s (Citation2009) studies, allelopathic potential of rice varied among tissues extracts. It is speculated that I-Kung-Pao is an allelopathic rice germplasm whose allelopathic effect is mainly from leaf leachate (Zhou et al. Citation2005).
In wheat germination test, root exudates of all rice varieties on-test inhibited the early growth of wheat seedlings (). Zhunliangyou 527 and Yliangyou 3218 showed stable inhibitory effects at concentration of 1–100 mg rice root exudates per L, whereas the inhibitory effects of other cultivars declined with the concentration decreased. Nowadays, rice–wheat rotation is popular around world, especially in China and India (Dawe et al. Citation2004; Hobbs et al. Citation2008). But most studies focused on the yield, resource use efficiency, and even greenhouse gas emissions within this rotation system (Bhandari et al. Citation2002; Ladha et al. Citation2005; Zhao et al. Citation2009). Fewer considered the allelopathic effects between rice and wheat. In our study, it is found that rice root exudates inhibited wheat seedling growth, even at the concentration of one mg L−1, which indicated that strong allelopathic effects existed between rice and wheat. Thus, whether or not the cultivation of allelopathic rice in the rice–wheat rotation system will inhibit wheat growth and reduce the output need further investment in field.
Autotoxicity is an essential problem that hampered the intensified/continuous cropping (Huang et al. Citation2013). Yu et al. (Citation1993), Yu and Matsui (Citation1994) reported the growth of tomato or cucumber in hydroponic culture was inhibited by organic substance from root exudates. Chou (Citation1980) reported that decomposed rice residues left on the paddy field soil and persisted into the next crop season could reduce the rice yield by up to 25% compared with that of the first crop. It seems both the release of phytotoxic compounds from root exudates and crop residues could be the reasons leading to intensified/continuous cropping problem. In this paper, the autotoxicitic effects of the root exudates from each on-test rice cultivars were screened. Zhunliangyou 527 and Zhongzu 14 inhibited rice seed germination at 100 mg root exudates per L, whereas Yongyou 15 and Zhongzao 22 prompted. However, the inhibitory effects vanished while the concentration of root exudates declined (), only I-Kung-Pao and Ganxin 203 have inhibitory effects at 10 and one mg L−1, respectively. In Chou and Lin's study (Citation1976), decomposed rice residues significantly suppressed rice radicle growth, and the ARI ranged from –0.13 to –0.36 for 25–100 g rice straw mixed with 3 kg soil decomposing for 2 weeks in aerobic condition and ranged from –0.09 to –0.32 for anaerobic condition, so rice straw was likely one of the autotoxitic source resulting in rice cropping problem. Kong et al. (Citation2006) noted allelochemicals released by rice roots and residues were different, and the living rice plant could detect the presence of interspecific neighbors and responded by increased allelochemicals. ARI of rice seedlings to their root exudates in our study were between –0.13 and +0.16, it seemed rice root exudates can either suppress or promote the growth of rice depending on the cultivar and the concentration of root exudates. This phenomenon is possibly resulting from the agronomic traits of rice cultivars or certain allelochemicals released (Bhadoria Citation2011; Gealy et al. Citation2013).
Broomrape seedlings can only survive for a few days after germination before connecting to a host root (Parker & Riches Citation1993). Thus, the germination of broomrape seeds requires chemical compounds secreted by potential host roots. Our results showed the root exudates of Yongyou 13, Yongyou 15, Zhongzao 22, and I-Kung-Pao induced highest germination of CB/SB seeds, whereas Yliangyou 3218 induced the lowest germination rate of both CB and SB seeds. These informed that Yongyou 13, Yongyou 15, Zhongzao 22, and I-Kung-Pao could be potential rice cultivars for the use as trap crop in broomrape infestation areas.
Broomrape becomes popular in the studies of plant physiology as its seeds are sensitive responders to strigolactones, which is a phytohormone-inhibiting plant shoot branching (Gomez-Roldan et al. Citation2008), an allelochemical inducing both AMF hyphal branching (Akiyama et al. Citation2005) and seeds of the some parasitic plants germination (Yoneyama et al. Citation2008), and also a signal in the adaptive responses of plants to a number of environmental stimuli (Liu et al. Citation2013). Xie et al. (Citation2013) screened the rice root exudates and found that the germination stimulation activities of the root exudates fractions on broomrape seeds were accompanied by the peaks of strigolactones identified by liquid chromatography tandem mass spectrometry. In our study, there was a significant correlation between the germination rates of the two broomrapes induced by rice root exudates (). Based on the germination induced by rice root exudates, it is speculated that the content of active strigolactones (and/or the analogs) in root exudates of Yliangyou 3218 is much less than that of Yongyou 13, Yongyou 15, Zhongzao 22, and I-Kung-Pao. Furthermore, germination rate of CB induced by rice root exudates was significantly positive correlated with ARIs of rice and wheat (), showing ARI decreased with germination rate of CB declined. ARI is a parameter showing the response of target plant to the substance with allelopathic potential. Thus, there probably is a relationship between the allelopathic potential of rice and the quantity of strigolactones it released. Gomez-Roldan et al. (Citation2008) revealed strigolactones inhibited shoot branching of pea. Kapulnik et al. (Citation2011) found exogenous GR24 (a strigolactone analog) reduced number of lateral roots of Arabidopsis. These researches indicate strigolactones reduce the interlacing space between plants. It is supposed that strigolactones (and/or the analogs) are likely taking part in secondary metabolites of plants and with the function altering the allelopathic effects between plants. Furthermore, it informed that the application of allelopathy in weed control should be more cautious before determining the subsequent effects on associated crops.
To our knowledge, I-Kung-Pao is an allelopathic cultivar of rice, which displayed greater allelopathic effect than PI3127777, the generally accepted allelopathic rice (Li et al. Citation2004; Zhou et al. Citation2005). However, lettuce misevaluated the allelopathic potential of rice cultivars if only in consideration of effects of root exudates. But broomrape seeds bioassays showed good consistency with the allelopathic potential of on-test rice cultivars (; ). Otherwise, it is possible to perform mass screening of rice varieties or lines at the early growth stage by using broomrape germination bioassays. Because the size of CB and SB seeds are very small and could be tested on 8 mm of glass fiber filter paper. One treatment needs only 20 µL of the test solution. An experiment with three replications would require only 60 µL of the test solution. This procedure will merely require a 20 m2 area, constant temperature (20°C–25°C), a clean bench, and a binocular dissecting microscopes at 20× magnification. A normal tissue culture laboratory is enough for this purpose. Thus, we believe broomrape seeds bioassays is a cheap, reliable, simple, and fast technique and suggest these assays be used in mass screening on the allelopathic potential of rice.
Acknowledgments
The authors thank the National Science and Technology Support Program [2011BAD31B05] for the financial support of this work. We thank Prof. A. Egrinya Eneji of the University of Calabar, Nigeria, and visiting professor at the State Key Laboratory of Soil Erosion and Dry Land Farming on Loess Plateau and also an American scientist Dr. Jeff Gale, present working at Shihezi University of China for critical reading and editing of the manuscript. The authors also thank the anonymous reviewers of this paper for their useful suggestions.
References
- Abdelgaleil S, Hashinaga F. 2007. Allelopathic potential of two sesquiterpene lactones from Magnolia grandiflora L. Biochem Syst Ecol. 35:737–742. 10.1016/j.bse.2007.06.009
- Akiyama K, Matsuzaki K, Hayashi H. 2005. Plant sesquiterpenes induce hyphal branching in arbuscular mycorrhizal fungi. Nature. 435:824–827. 10.1038/nature03608
- Ben HM, Ghorbal H, Kremer RJ, Oussama O. 2001. Allelopathic effects of barley extracts on germination and seedlings growth of bread and durum wheats. Agronomie. 21:65–71. 10.1051/agro:2001109
- Bhadoria PBS. 2011. Allelopathy: a natural way towards weed management. Am J Exp Agric. 1:7–20.
- Bhandari AL, Ladha JK, Pathak H, Padre AT, Dawe D, Gupta RK. 2002. Yield and soil nutrient changes in a long-term rice-wheat rotation in India. Soil Sci Soc Am J. 66:162–170. 10.2136/sssaj2002.0162
- Chou CH. 1980. Allelopathic researches in the subtropical vegetation in Taiwan. Comp Physiol Ecol. 5:222–234.
- Chou CH, Chung YT. 1974. The allelopathic potential of Miscanthus floridulus. Bot Bull Acad Sinica. 15:12–27.
- Chou CH, Lin HJ. 1976. Autointoxication mechanism of Oryza sativa I. Phytotoxic effects of decomposing rice residues in soil. J Chem Ecol. 2:353–367. 10.1007/BF00988282
- Courtois B, Olofsdotter M. 1998. Incorporating the allelopathy trait in upland rice breeding programs. In: Olofsdotter M, editor. Allelopathy in rice. Manila: International Rice Research Institute; p. 57–68.
- Dawe D, Frolking S, Li C. 2004. Trends in rice–wheat area in China. Field Crop Res. 87:89–95. 10.1016/j.fcr.2003.08.008
- Dilday RH, Yan WG, Moldenhauer KAK, Gravois KA. 1998. Allelopathic activity in rice for controlling major aquatic weeds. In: Olofsdotter M, editor. Allelopathy in rice. Manila: International Rice Research Institute; p. 7–26.
- Ebana K, Yan W, Dilday RH, Namai H, Okuno K. 2001. Variation in the allelopathic effect of rice with water soluble extracts. Agron J. 93:12–16. 10.2134/agronj2001.93112x
- Fujii Y. 1992. The potential biological control of paddy weeds with allelopathy: allelopathic effect of some rice varieties. In: Proceedings of the International Symposium on Biological Control and Integrated Management of Paddy and Aquatic Weeds in Asia; 1992 Oct 19–25; Tsukuba: National Agricultural Research Center; p. 305–320.
- Gealy D, Moldenhauer K, Duke S. 2013. Root distribution and potential interactions between allelopathic rice, sprangletop (Leptochloa spp.), and barnyardgrass (Echinochloa crus-galli) based on 13C isotope discrimination analysis. J Chem Ecol. 39:1–18. 10.1007/s10886-013-0246-7
- Gomez-Roldan V, Fermas S, Brewer PB, Puech-Pagès V, Dun EA, Pillot JP, Rochange SF. 2008. Strigolactone inhibition of shoot branching. Nature. 455:189–194.
- Hobbs PR, Sayre K, Gupta R. 2008. The role of conservation agriculture in sustainable agriculture. Philos Trans R Soc B. 363:543–555. 10.1098/rstb.2007.2169
- Huang LF, Song LX, Xia XJ, Mao WH, Shi K, Zhou YH, Yu JQ. 2013. Plant-soil feedbacks and soil sickness: from mechanisms to application in agriculture. J Chem Ecol. 39:1–11. 10.1007/s10886-013-0244-9
- Jebsen LB, Courtois B, Shen LS, Li ZK, Olofsdotter, M, Mauleon, RP. 2001. Locating genes controlling allelopathic effects against barnyardgrass in upland rice. Agron J. 93:21–26. 10.2134/agronj2001.93121x
- Kanesawa T, Itani T, Kato-Noguchi H. 2009. Inhibitory effects of rice seedling extracts and root exudates of Japanese traditional rice varieties. Allelopathy J. 24:397–403.
- Kapulnik Y, Delaux PM, Resnick N, Mayzlish-Gati E, Wininger S, Bhattacharya C, Séjalon-Delmas N, Combier JP, Bécard G, Belausov E, et al. 2011. Strigolactones affect lateral root formation and root-hair elongation in Arabidopsis. Planta. 233:209–216. 10.1007/s00425-010-1310-y
- Kato-Noguchi H. 1999. Effect of light-irradiation on allelopathic potential of germinating maize. Phytochemistry. 52:1023–1027. 10.1016/S0031-9422(99)00365-9
- Kato-Noguchi H, Kobayashi K, Shigemori H. 2009. Allelopathy of the moss Hypnum plumaeforme by the production of momilactone A and B. Weed Res. 49:621–627. 10.1111/j.1365-3180.2009.00731.x
- Kato-Noguchi H, Nitta K, Itani T. 2013. Allelopathic potential of white, red and black rice cultivars. Plant Prod Sci. 16:305–308. 10.1626/pps.16.305
- Kato-Noguchi H, Peters RJ. 2013. The role of momilactones in rice allelopathy. J Chem Ecol. 39:175–185. 10.1007/s10886-013-0236-9
- Kato-Noguchi H, Salam MA, Suenaga K. 2011. Isolation and identification of potent allelopathic substances in a traditional Bangladeshi rice cultivar Kartikshail. Plant Prod Sci. 14:128–134. 10.1626/pps.14.128
- Khanh T, Xuan T, Chung I. 2007. Rice allelopathy and the possibility for weed management. Ann Appl Biol. 151:325–339. 10.1111/j.1744-7348.2007.00183.x
- Kong CH, Li HB, Hu F, Xu XH, Wang P. 2006. Allelochemicals released by rice roots and residues in soil. Plant Soil. 288:47–56. 10.1007/s11104-006-9033-3
- Ladha JK, Pathak H, Krupnik TJ, Six J, van Kessel C. 2005. Efficiency of fertilizer nitrogen in cereal production: retrospects and prospects. Adv Agron. 87:85–156.
- Leather GR, Einhellig FA. 1986. Bioassays in the study of allelopathy. In: Putnam AR, Tang CS, editors. The science of allelopathy. New York: John Wiley and Sons; p. 133–145.
- Li D, Zhou YJ, Liu XC, Yu LQ, Tang FB, Wei XH, Guo LB. 2004. Evaluation of allelopathic potential of some Chinese rice against weeds. Chin J Rice Sci. 16:309–314. Chinese with English abstract.
- Liu J, Lovisolo C, Schubert A, Cardinale F. 2013. Signaling role of strigolactones at the interface between plants, (micro)organisms, and a changing environment. J Plant Interact. 8:17–33. 10.1080/17429145.2012.750692
- Mahmood K, Khan MB, Song YY, Ljaz M, Luo SM, Zeng RS. 2013. UV-irradiation enhances rice allelopathic potential in rhizosphere soil. J Plant Growth Regul. 71:21–29. 10.1007/s10725-013-9804-9
- Maneechote C, Krasaesindhu P. 1996. Allelopathic effects of some upland and wild rice genotypes in Thailand. In: Macias FA, Fujii Y, editors. Recent advances in allelopathy, Vol. 1, A science for the future. Cadiz: University of Cadiz Press; p. 81.
- Mangnus EM, Stommen PLA, Zwanenburg B. 1992. A standardized bioassay for evaluation of potential germination stimulants for seeds of parasitic weeds. J Plant Growth Regul. 11:91–98. 10.1007/BF00198020
- Navarez D, Olofsdotter M. 1996. Relay seeding technique for screening allelopathic rice (Oryza sativa). In: Brown H, Cussans GW, Devine MD, Duke SO, Fernandez-Quantilla C, Helweg A, Labrada RE, Landes M, Kudsk P, Streigbig JC, editors. Proceedings of the 2nd International Weed Control Conference; 1996 Jun 25–28; Copenhagen: Department of Weed Control and Pesticide Ecology; p. 1285–1290.
- Olofsdotter M. 2001. Rice-a step toward use of allelopathy. Agron J. 93:3–8. 10.2134/agronj2001.9313
- Olofsdotter M, Navarez D, Rebulanan M, Streibig JC. 1999. Weed suppressing rice cultivars-does allelopathy play a role? Weed Res. 39:441–454. 10.1046/j.1365-3180.1999.00159.x
- Parker C, Hitchcock A, Ramaiah M. 1977. The germination of Striga species by crop root exudates: techniques for selecting resistant crop cultivars. In: Proceedings of Asian-Pacific Weed Science Society 6th Conference; 1977 July 11–17; Jakarta: Asian-Pacific Weed Science Society; p. 67–74.
- Parker C, Riches CR. 1993. Parasitic weeds of the world: biology and control. Wallingford: CAB international.
- Putnam AR. 1986. Adverse impacts of allelopathy in agricultural system. In: Putnam AR, Tang CS, editors. The science of allelopathy. New York: John Wiley and Sons; p. 43–56.
- Rimando AM, Duke SO. 2003. Studies on rice allelochemicals. In: Smith CW, Dilday RH, editors. Rice: origin, history, technology and production. Hoboken: Wiley; p. 221–244.
- Tadano T, Tanaka A. 1980. The effect of low phosphate concentrations in culture medium on early growth of several crop plants. Jap J Soil Sci Plant Nutr. 51:399–404.
- Uddin MN, Robinson RW, Caridi D. 2013. Phytotoxicity induced by Phragmites australis: an assessment of phenotypic and physiological parameters involved in germination process and growth of receptor plant. J Plant Interact. 9:338–353.
- Weih M, Didon UME, Ronnberg-Wastljung AC, Bjorkman C. 2008. Integrated agricultural research and crop breeding: allelopathic weed control in cereals and long-term productivity in perennial biomass crops. Agr Syst. 97:99–107. 10.1016/j.agsy.2008.02.009
- Williamson GB, Richardson D. 1988. Bioassays for allelopathy: measuring treatment responses with independent controls. J Chem Ecol. 14:181–187. 10.1007/BF01022540
- Xie XN, Yoneyama K, Kisugi T, Uchida K, Ito S, Akiyama K, Hayashi H, Yokota T, Nomura T, Yoneyama K. 2013. Confirming stereochemical structures of strigolactones produced by rice and tobacco. Mol Plant. 6:153–163. 10.1093/mp/sss139
- Xuan TD, Shinkichi T, Khanh TD, Min CI. 2005. Biological control of weeds and plant pathogens in paddy rice by exploiting plant allelopathy: an overview. Crop Prot. 24:197–206. 10.1016/j.cropro.2004.08.004
- Yoneyama K, Xie XN, Sekimoto H, Takeuchi Y, Ogasawara S, Akiyama K, Hayashi H, Yoneyama K. 2008. Strigolactones, host recognition signals for root parasitic plants and arbuscular mycorrhizal fungi, from Fabaceae plants. New Phytol. 179:484–494. 10.1111/j.1469-8137.2008.02462.x
- Yu JQ, Lee KS, Matsui Y. 1993. Effect of the addition of activated charcoal to the nutrient solution on the growth of tomato in hydroponic culture. Soil Sci Plant Nutr. 39:13–22. 10.1080/00380768.1993.10416970
- Yu JQ, Matsui Y. 1994. Phytotoxic substances in root exudates of cucumber (Cucumis sativus L.). J Chem Ecol. 20:21–31. 10.1007/BF02065988
- Zeng HY, Alan AR, Saxena PK. 2009. Evaluation of in vitro shoots of Artemisia judaica for allelopathic potential. Acta Physiol Plant. 31:1237–1248. 10.1007/s11738-009-0360-x
- Zhao X, Xie YX, Xiong ZQ, Yan XY, Xing GX, Zhu ZL. 2009. Nitrogen fate and environmental consequence in paddy soil under rice-wheat rotation in the Taihu lake region, China. Plant Soil. 319:225–234. 10.1007/s11104-008-9865-0
- Zhou YJ, Li D, Lu YL, Yu LQ, Chen MX. 2005. Evaluation of allelopathic potential in rice germplasm. Acta Ecol Sin. 25:1599–1603. Chinese with English abstract.