Abstract
Artemisinin is frequently used in the artemisinin-based combination therapy to cure drug-resistant malaria in Asian subcontinent and large swath of Africa. The hairy root system, using the Agrobacterium rhizogenes LBA 9402 strain to enhance the production of artemisinin in Artemisia annua L., is developed in our laboratory. The transgenic nature of hairy root lines and the copy number of transgene (rol B) were confirmed using polymerase chain reaction and Southern Blot analyses, respectively. The effect of different concentrations of methyl jasmonate (MeJA), fungal elicitors (Alternaria alternate, Curvularia limata, Fusarium solani, and Piriformospora indica), farnesyl pyrophosphate, and miconazole on artemisinin production in hairy root cultures were evaluated. Among all the factors used individually for their effect on artemisinin production in hairy root culture system, the maximum enhancement was achieved with P. indica (1.97 times). Increment of 2.44 times in artemisinin concentration by this system was, however, obtained by combined addition of MeJA and cell homogenate of P. indica in the culture medium. The effects of these factors on artemisinin production were positively correlated with regulatory genes of MVA, MEP, and artemisinin biosynthetic pathways, viz. hmgr, ads, cyp71av1, aldh1, dxs, dxr, and dbr2 in hairy root cultures of A. annua L.
Abbreviations | ||
ACT | = | artemisinin-based combination therapy |
ADS | = | amorpha-4,11-diene synthase |
ALDH1 | = | aldehyde dehydrogenase 1 |
B5 | = | Gamborg's medium |
CYP71AV1 | = | cytochrome P450 monooxygenase |
DBR2 | = | artemisinic aldehyde double bond reductase |
DW | = | dry weight |
DXS | = | 1-deoxy-d-xylulose 5-phosphate synthase |
DXR | = | 1-deoxy-d-xylulose 5-phosphate reductoisomerase |
HMGR | = | 3-hydroxy-3-methylglutaryl coenzyme A reductase |
HPLC | = | high-performance liquid chromatography |
MS | = | Murashige and Skoog |
qPCR | = | quantitative polymerase chain reaction |
Introduction
Artemisinin is currently used for the production of artemisinin-based combination therapy (ACT) to treat drug-resistant and cerebral malaria (White Citation2008). It has also been found effective against various infectious diseases such as schistosomiasis, HIV, hepatitis-B, and leishmaniasis (Borrmann et al. Citation2001; Romero et al. Citation2005; Sen et al. Citation2007). Artemisinin has shown effectiveness against a variety of cancer cell lines including breast cancer, human leukemia, colon cancer, and small cell lung carcinomas (Efferth et al. Citation2001). The current production of artemisinin is 232–262 metric tons (MT) and global demand is 289 MT (Source: A2S2 market intelligence, 2012) for its use in the manufacturing of ACT. The diversification of its use in other diseases, however, may increase its demand further in near future. At present, the sole source of commercial artemisinin is Artemisia annua L. plants, where the artemisinin production is subjected to seasonal and environmental changes (Luo & Shen Citation1987). Moreover, the artemisinin concentration in the plant is very low (0.01–1.1% dry weight [DW]). Due to these limitations, the commercialization of drug in the regions of the world with prevalence of drug-resistant malaria is a serious problem (Liu et al. Citation2006). To overcome these problems, efforts are being made worldwide to enhance its biosynthesis employing biochemical and molecular approaches (MaujiRam et al. Citation2010; Aftab et al. Citation2010, Citation2011; Alam & Abdin Citation2011; Alam et al. Citation2014; Tang et al. Citation2014). The chemical synthesis of artemisinin is complicated and economically unviable due to the poor yields (Haynes Citation2006). The biotechnological approaches such as metabolic engineering of microbes and plants are alternative methods to produce artemisinin (Ro et al. Citation2006). These strategies are, however, still at developmental phase and economically not viable. In vitro culture of A. annua L. is used as an alternative source for the production of artemisinin throughout the year, as the production of artemisinin employing this system is independent of geographical, seasonal, and environmental factors (Rao & Ravishankar Citation2002). Among in vitro culture methods, hairy roots provide better advantage over cell suspension such as high growth rate, genetic stability, and growth in hormone-free media (Guillon et al. Citation2006). They can also be used as an experimental system to study secondary metabolism (Bais et al. Citation2001). The efforts have been made in past on large-scale production of artemisinin through optimization of hairy root system (Giri et al. Citation2001; Xie et al. Citation2001; Liu et al. Citation2002; Putalun et al. Citation2007), but the yield was very poor. There have been reports, where variations in secondary metabolite contents among the hairy root lines, induced from same plant or different parts of the same plant, were also observed (Kim et al. Citation2002).
Enhancement of secondary metabolites by elicitation is also now used in commercial production. The use of biotic or abiotic stress on tissue cultures has been shown to have an effect on the secondary metabolite accumulation. Elicitors are mainly of microbial origin or non-biological origin, which upon contact with higher plant cells trigger the increased production of pigments, flavones, phytoalexins, and other defense-related compounds (Jaleel et al. Citation2009). Methyl jasmonate (MeJA) is a well-known signal molecule that induces reprogramming of gene expression and elicits the pathway of secondary metabolism in plant cells (Pauwels et al. Citation2008). Effect of various treatments like MeJA on hairy root culture (Patra et al. Citation2013) and miconazole on cell suspension culture (Caretto et al. Citation2011) of A. annua L. has been reported earlier. Effect of an oligosaccharide elicitor (OE) induced nitric oxide (NO) from Fusarium oxysporum mycelium was observed on A. annua L. roots (Zheng et al. Citation2008). Stimulation of artemisinin production in hairy roots was also done by the OE from an endophytic fungus Colletotrichum gloeosporioides of A. annua L. (Wang et al. Citation2002, Citation2006). A significant enhancement in artemisinin content has been achieved in A. annua L. shoots by co-cultivating with Piriformospora indica (Sharma & Agrawal Citation2013). Effect of Aspergillus oryza and Penicillium chysogenum 3446 strain (Liu et al. Citation1999) on artemisinin production was also observed on hairy root cultures of A. annua L. Liu et al. (Citation2009) reported that jasmonic acid increases the density of glandular trichomes on A. annua leaves and enhances artemisinin production. P. indica, a root endophytic fungus functions as a plant growth promoter and biofertilizer in nutrient-depleted soils. It also acts as a bioprotector against a variety of pathogens and insect invaders (Oelmuller et al. Citation2009). The fungus has shown growth-promoting and yield enhancement activities on a variety of host plants such as maize, tobacco, and Bacopa monnieri (Varma et al. Citation1999, Citation2001). Precursors and inhibitors of secondary plant metabolic pathways have also proved to be an effective way to enhance secondary metabolites in hairy root cultures. Miconazole is an antifungal compound, known to inhibit sterol biosynthesis and to redirect carbon flux toward the terpenoid pathway (Kudakasseril et al. Citation1987; Zarn et al. Citation2003). Effect of farnesyl pyrophosphate (FPP) was observed on Centella asiatica and Ginkgo biloba cell cultures (Kiong et al. Citation2005; Kang et al. Citation2006).
Though a lot of work has been done on elicitation in hairy root cultures, the effect of FPP and miconazole has not been reported earlier. Synergistic effect of MeJA and P. indica has not yielded very much enhancement in artemisinin content in hairy roots of A. annua L. till now. Similarly, no correlation has been established between the effect of these treatments and gene expression of mevalonate (MVA), methylerythritol phosphate (MEP) pathway and artemisinin biosynthetic genes in case of hairy roots yet. In the present study, we, however, report higher biomass and artemisinin production in hairy root lines of A. annua L. with the combined application of both abiotic and biotic elicitors (MeJA and cell homogenate [CH] of P. indica) with the effect of FPP as precursor and miconazole as inhibitor. Simultaneously, we have also established correlation between the effect of these treatments on artemisinin production and expression of MVA, MEP, and artemisinin biosynthetic genes (hmgr, ads, cyp71av1, aldh1, dxs, dxr, and dbr2) using real-time polymerase chain reaction (PCR).
Materials and methods
Plant material
The seeds of A. annua L. (0.6–0.8% artemisinin), obtained from Centre for Transgenic Plant Development, Jamia Hamdard, New Delhi 110062, India, were washed in 1% Savlon, surface sterilized with 70% ethanol for 1 min and rinsed thrice using sterile water. This was followed by treatment with 0.01% w/v mercuric chloride for 2 min and washed again with sterile water for 4–5 times. For germination, sterilized seeds were then placed on Murashige and Skoog (Citation1962) medium containing 3% sucrose and 1% agar at 25 ± 1°C under 16/8 hours light/dark regime. After seed germination, the seedlings were grown in culture room for a period of 45 days prior to harvesting for explants.
Hairy root culture development and maintenance
Hairy roots of A. annua L. were initiated by infecting leaf discs with Agrobacterium rhizogenes (LBA9402) (Ahlawat et al. Citation2012) and maintained by transferring 1.8 g of fresh root every 20th day in 250 ml Erlenmeyer flask filled with 40 ml liquid B5 (Gamborg et al. Citation1968) medium supplemented with 30 g l−1 sucrose for 3 weeks on a gyratory shaker at 80 rpm and 25 ± 1°C under 16/8 hours light/dark photoperiod. The fresh weight used for inoculation was calculated from the ratio of the fresh weight to DW, which was equal to 12.
PCR and Southern blot analyses of transformed hairy root lines
Integration of the TL-DNA (rol B gene) region of the pRi plasmid was confirmed by PCR analysis in transformed hairy root lines of A. annua L., where plasmid DNA was used as positive control and wild-type root as negative control. The rol B-specific primers used to amplify gene were: forward primer, 5′ CAGAATGGAATTAGCCGGACTA 3′ and the reverse primer, 5′ CGTATTAATCCCGTAGGTTTGTTT 3′. PCR conditions for amplification of rol B gene included denaturation at 94°C for 1 min, 2 min annealing at 55°C followed by a 3 min extension at 72°C, and a final extension at 72°C for 7 min. The PCR products were analyzed through agarose gel electrophoresis.
After PCR analysis, Southern blot was performed to check the copy number of rol B gene. Genomic DNA samples (conc. 10 µg), isolated from hairy root lines and wild-type root, were digested with EcoRI, resolved on 0.7% agarose gel and blotted on a nylon membrane (Roche Diagnostic). For hybridization, rol B gene (750 bp)-specific probe was prepared. The probe was labeled with digoxigenin using the DIG High Prime DNA labeling and detection starter kit (Roche Diagnostics, USA). The blot was probed with the labeled rol B gene at 42°C. After 16 hours of hybridization, the membrane was checked for hybridization signals to confirm the stable integration and copy number of transgene by following the standard protocol (Hamill & Lidgett Citation1997).
Artemisinin production enhancement strategies in transformed hairy root cultures
The following experiments were carried out in a 250 ml Erlenmeyer flask on an orbital shaker running at 80 rpm and at 25 ± 1°C in 16/8 hours light/dark regime.
Elicitation by addition of elicitors
Effect of MeJA was evaluated on biomass (g l−1) and artemisinin production (mg l−1) in hairy root cultures of A. annua L. MeJA was dissolved in 95% v/v ethanol. MeJA and equivalent volumes of 95% ethanol were aseptically added to the cultivation medium at 0, 50, 100, and 250 µM concentrations on 15th day (day of addition optimized, data not shown) of hairy root cultivation.
The fungal cultures of Alternaria alternate, Curvularia limata, and Fusarium solani (AA, CL, and FS) were obtained from the Institute of Microbial Technology, Chandigarh, India and P. indica (PI) culture was taken from Dr. Ajit Varma, Amity University, UP, India. All fungal cultures except P. indica were grown on a liquid potato dextrose growth medium for 2 weeks under static conditions at 25 ± 1°C in dark by transferring 1 cm2 piece of grown fungi on the solid potato dextrose medium.
P. indica cultures were maintained on Kaefer agar medium (Kaefer Citation1977) supplemented with 15 g l−1 agar at pH 6.5 and 30 ± 1°C. From these Petri plates, 8-mm agar discs were punched out with a sterilized cork-borer and used as such with hairy root culture grown in B5 medium supplemented with 7 g l−1 agar (Singh et al. Citation2013). Discs were kept on 5th, 10th, 15th, and 18th day to the growing hairy root cultures of A. annua L.
For liquid culture, these discs were inoculated in 500 ml Erlenmeyer flasks containing 100 ml Kaefer medium without agar at 30 ± 1°C on a gyratory shaker at 200 rpm. The 5-day-old cultures were used as inoculum to grow the fungi. After growth, the fungal suspension cultures were autoclaved at 15 lb in−2 and 121°C for 15 min. The autoclaved cultures were filtered, and the filtrate was denoted as culture filtrate (CF). The cell residues were washed thrice with sterile water and homogenized in a mortar and pestle with 20 ml sterile water under aseptic conditions. The volume was then made up to 50 ml with sterile water. This preparation was denoted as the CH. These CFs and CHs of different fungi were kept in refrigerator at 4–6°C until further use. All the biotic elicitors (CH and CF of AA, CL, FS, and PI) were added at different concentrations (0.5, 1.0, 2.0, 3.0, and 5.0% v/v) on 5th, 10th, 15th, and 18th day to the growing hairy root cultures of A. annua L.
MeJA and optimized fungal elicitor were integrated in order to develop a bioprocess for synergistic enhancement of artemisinin productivity in hairy root cultures of A. annua L.
Feeding of FPP
FPP was procured from Sigma, USA and filtered. It was added to the B5 medium at different concentrations (0.0, 0.1, 0.5, and 1.0 mg l−1) on 10th day of cultivation (day of addition optimized, data not shown). Methanol:NH4OH (7:3) solution was prepared for control. Control samples were treated with equivalent volumes of this solvent.
Addition of miconazole
Miconazole was procured from Sigma, USA and dissolved in dimethyl sulfoxide (DMSO, Sigma). After filter sterilization, it was added to the B5 medium at varying concentrations (0, 4, 20, and 40 mg l−1) on 10th day of inoculation (day of addition optimized, data not shown). Control samples were treated with equivalent volumes of DMSO.
Cultures were harvested on 20th day and experiments were performed in triplicate. The hairy root samples were analyzed for biomass and artemisinin content.
Estimation of artemisinin
One gram of dried hairy roots was used for the estimation of artemisinin content using the high-performance liquid chromatography (HPLC) method (Zhao & Zeng Citation1986). The derivatized artemisinin was analyzed and quantified using reverse phase column (C18, 5 µm, 4.6 × 250 mm) with premix methanol: 100 mM K-phosphate buffer (pH 6.5) in the ratio of 60:40 as mobile phase at a constant flow rate of 1 ml min−1 with the UV detector set at 260 nm. The artemisinin was quantified with the help of standard curve prepared by HPLC from pure artemisinin standard solutions with varying concentrations of artemisinin (10–100 µg ml−1).
Isolation of total RNA and quantitative PCR analysis
Tissue preparation
Five treatments (1) hairy root culture with MeJA and P. indica together, (2) hairy root culture with P. indica, (3) hairy root culture with MeJA, (4) hairy root culture with miconazole, and (5) hairy root culture with FPP and C (hairy root without any treatment) were taken for gene expression studies.
RNA extraction
Total RNA was extracted from 100 mg of hairy roots and normal roots using RNeasy plant mini kit (Qiagen, USA) according to the manufacturer's instructions. The RNA was treated with DNase I (Fermentas, St Leo-Roth, Germany) to get rid of the genomic DNA. The genomic DNA contamination was checked by performing qualitative PCR using β-actin as housekeeping gene taking RNA as a template.
cDNA synthesis
Two micrograms of isolated RNA were reverse transcribed using Long-range reverse transcriptase (Qiagen) primed with 0.5 µg oligo (dT) 18 primer. The RNA was removed from the first strand cDNA by RNase treatment using RNase H (Qiagen) according to the manufacturer's instructions. Isolated cDNA was used for further experiments.
Again endpoint PCR was done using primers for housekeeping gene (β-actin) to check the cDNA quality in terms of amplification.
Quantitative PCR
Real-time PCR was performed to check the quantitative expression of seven genes (hmgr, ads, cyp71av1, aldh1, dxs, dxr, and dbr2) of artemisinin biosynthetic pathway using gene-specific primers () and β-actin as the reference gene on a Mx 5000P quantitative PCR (qPCR) equipment (Stratagene, India). Dye-based chemistry was used for the study. cDNA was used as template (1 µl) in 25 µl reactions taking 12.5 µl Power SYBR®Green 2X PCR Master Mix (Roche), 0.3 µmol of each primer (Sigma), and 9.5 µl of nuclease-free water. The qPCR cycling was performed at 95°C (10 min) for 1 cycle, 40 cycles at 95°C (15 s), and annealing/extension at 60°C (1 min). A melt curve analysis was done to check the primer dimer and other contamination at 95°C (15 s), 60°C (20 s), and 95°C (15 s) for 40 cycles. Samples were run in triplicate using a no template control in each of the assays. MxPro analysis software was used for the cycle threshold (CT) values. Relative expression analysis was done using ▵▵CT method.
Table 1. Nucleotide sequences of primers used in qPCR.
Statistical analysis
All experiments were conducted with three replicates. Statistical analysis was performed using one-way analysis of variance followed by Duncan's multiple range test. The values are mean ± SE for three samples in each group. P values equal to 0.05 were considered significant.
Results and discussion
Total 380 hairy root lines were studied and screened for specific growth rate (day−1) and artemisinin content (mg g−1). Nine hairy root lines (A1, A2, A3, A4, A5, A6, A7, A8, and A9) were selected on the basis of higher specific growth rate (day−1) and artemisinin content (mg g−1) among all the induced hairy root lines. There was a clear-cut difference from one clone to another in terms of growth rate as well as artemisinin content (). These differences may be attributed to the variation in the location of T-DNA insertion and its copy number into the plant genome (Tenea et al. Citation2008).
Table 2. Specific growth rate and artemisinin content of hairy root lines of A. annua L.
Confirmation of transformation of hairy roots lines of A. annua L.
Integration of TL-DNA into plant genome was confirmed by the presence of rol B gene employing PCR and Southern blot analyses of hairy root lines as shown in and , respectively. These hairy root lines were found to contain either single (A1, A4, A8, and A9) or multiple copies (A2, A3, A5, A6, and A7) of the rol B confirming their transgenic nature (). It is interesting to note that all the selected hairy root lines with higher artemisinin content as compared to normal roots evidenced rol B gene integration (). The hairy root line A1 with single copy of rol B was, however, found to have higher artemisinin content (0.66 ± 0.01 mg g−1) followed by other hairy root lines (0.10 ± 0.02 − 0.35 ± 0.03 mg g−1) of A. annua L. (). Higher the number of T-DNA copies means harboring more number of transgenes involved in the synthesis of indole acetic acid (IAA; Camilleri & Jouanin Citation1991). Under such conditions, one can expect altered growth and metabolic pattern in root clones. In the present study, Southern analysis of different clones showed at least one copy of the T-DNA insert in A1, A4, A8, and A9 and two T-DNA inserts in A2, A3, A5, A6, and A7 lines; however, there was no correlation established between the T-DNA copy number and artemisinin content. This observation clearly indicates that mere presence of higher number of T-DNA copy inserts would not cause any increase in growth and production of secondary metabolites. It was reported earlier that copy number of T-DNA insertions is not an important factor of the manifestation of transformed phenotypes. A single copy transformation is the best choice to produce stable transgene effect (Lacroix et al. Citation2006).
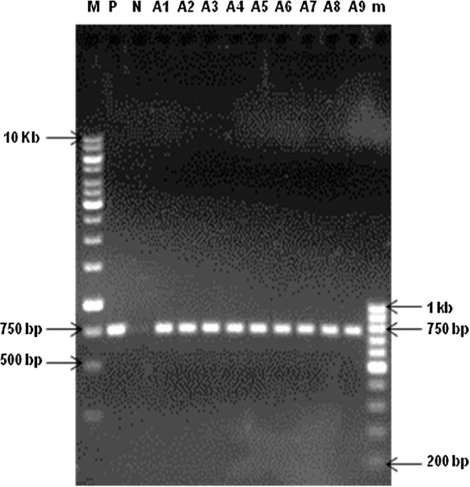
Southern blot analysis of genomic DNA digested with EcoRI and hybridization with rol B-specific gene probe of non-transformed and transformed hairy root lines from A. annua L. plant. Lanes: P, positive control (Agrobacterium rhizogenes plasmid); N, negative control (wild-type roots); A1–A9, hairy root lines of A. annua L. plants.
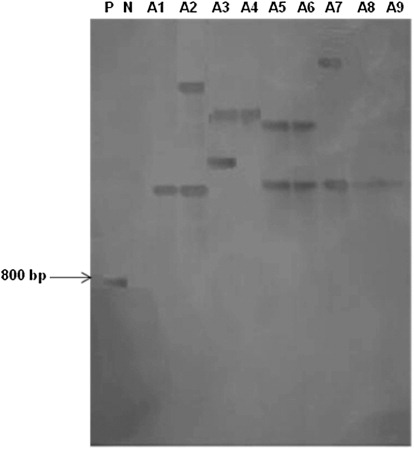
Out of these hairy root lines (A1–A9), A1 root line was selected for the following experiments on the basis of its maximum specific growth rate (0.07 ± 0.001 days−1) and artemisinin content (0.66 ± 0.01 mg g−1) for further experiments.
Effect of elicitors on artemisinin production
Exponentially growing A. annua L. hairy root cultures were subjected to different concentrations of MeJA before sample collection. Hairy root growth was maximally increased by 1.14 times (13.4 ± 0.56 g l−1) in comparison to ethanol-treated control hairy root cultures (11.7 ± 0.49 g l−1) upon addition of 100 µM MeJA (), while 1.64 times artemisinin production (13.3 ± 0.52 mg l−1) in comparison to ethanol-treated control hairy root cultures (8.1 ± 0.31 mg l−1) was recorded in the present study (). No significant differences in artemisinin content were observed between untreated and ethanol-treated cells (). MeJA is thought to be the precursor of the jasmonic acid which is one of the intermediates involved in the signal transduction. Jasmonic acid and its derivatives have been shown to play an integral role in the cascade of events that occur in the elicitation process leading to activation of genes coding for secondary metabolism (Gundlach et al. Citation1992). Application of jasmonates to the plant cell culture or intact plant is known to stimulate the biosynthesis of secondary metabolites (Suresh et al. Citation2004). Wang et al. (Citation2009) indicated that besides artemisinin, artemisinic acid, dihydroartemisinic acid, and other sesquiterpenoids as well as triterpenoids in A. annua L. were also increased by exogenous application of MeJA. In the present study, the observed significant increase in artemisinin levels after the addition of MeJA indicated a rapid response of these cells to MeJA, as well as the ability of MeJA to stimulate artemisinin biosynthesis. The effect of dose of an elicitor on secondary metabolites biosynthesis was also observed in MeJA-induced taxol biosynthesis (Ketchum et al. Citation1999).
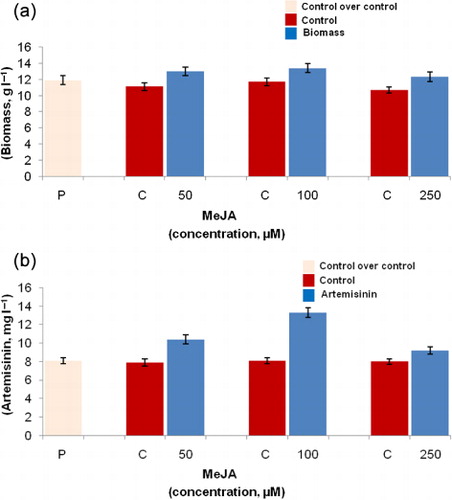
The effect of addition of CH and CF of the fungal elicitors on artemisinin production is summarized in . Addition of CH at 3% v/v F. solani, C. limata, and A. alternate resulted in 1.01 (13.1 ± 0.54 g l−1), 1.07 (12.4 ± 0.52 g l−1), and 1.07 times (12.9 ± 0.52 g l−1) increase in biomass and 1.44 (12.4 ± 0.52 mg l−1), 1.13 (8.2 ± 0.33 mg l−1), and 1.27 times (9.8 ± 0.45 mg l−1) increase in artemisinin production, respectively, in comparison to their respective control cultures. Addition of CF of fungal elicitors has not shown any increase in biomass or artemisinin production. 9.3 ± 0.32, 8.9 ± 0.39, and 8.8 ± 0.33 g l−1 biomass and 5.2 ± 0.25, 5.4 ± 0.26, and 6.2 ± 0.38 mg l−1 artemisinin production was achieved by addition of CF at 3% v/v C. limata, F. solani, and A. alternate, respectively. The possible hypothesis for enhanced production by CH of fungal elicitors might be due to the fact that the extracellular enzymes from A. annua L. hairy root cultures hydrolyze the walls of autoclaved fungi to generate the real elicitor moieties. The variation in response due to the addition of different fungal preparations might be the difference of elicitor moieties generated in quantity and quality, depending on the fungal species employed. The addition of elicitor preparations of Verticillium species had also found to increase the accumulation of tessaric acid production in Tessaria absinthioides cell suspension cultures (Sanz et al. Citation2000). The significant enhancement in various production of plant secondary metabolites by the addition of fungal preparations as biotic elicitors had been already reported by Savitha et al. (Citation2006). Ajmalicine accumulation in Catharanthus roseus suspension cultures was evaluated on exposure to different concentrations of fungal extracts of Trichoderma viride, Aspergillus niger, and Fusarium moniliforme. It was found that the ajmalicine content in cells treated with an optimum concentration (5.0%) of elicitor extracts was higher as compared to lower or higher concentrations (Namdeo et al. Citation2002). High dosage of elicitor has been reported to induce hypersensitive response leading to cell death, whereas an optimum level was required for induction (Roewer et al. Citation1992). Similarly, when the hairy roots of A. annua L. were exposed to fungal extracts of Sclerotium rolfsii, F. solani, and C. limata at various concentrations, artemisinin production in hairy roots treated with an optimum concentration (3.0%) of elicitor extracts was higher as compared to their lower or higher concentrations ().
Table 3. Effect of CH and CF of fungal elicitors on growth and artemisinin content of hairy roots.
P. indica was reported to be involved in high salt tolerance, disease resistance, and strong growth-promoting activities leading to enhancement of host plant yield (Waller et al. Citation2005). P. indica discs were kept on 5th, 10th, 15th, and 18th day to the growing hairy root cultures of A. annua L (). 1.57 times increase in artemisinin production (13.2 ± 0.41 mg l−1) was achieved in comparison to the control cultures (8.4 ± 0.41 mg l−1), when disc was kept on 10th day of cultivation. No effect of P. indica disc was observed on 18th day of inoculation (8.1 ± 0.43 mg l−1), while 1.05 and 1.13 times increase in artemisinin content (8.9 ± 0.38 and 9.5 ± 0.39 mg l−1) was achieved in comparison to the control cultures on 5th and 15th day of inoculation. The concentration and the exposure time of an elicitor to the plant cell/tissue cultures are important factors in maximizing its elicitation potential (Rijhwani & Shanks Citation1998; Ketchum et al. Citation1999).
Table 4. Effect of P. indica disc on artemisinin production in hairy root cultures of A. annua L.
The effect of addition of CH and CF on biomass and artemisinin production is summarized in and , respectively. The addition of CH of P. indica resulted in plant growth-promoting effect on hairy roots in liquid cultures. Hairy root growth was maximally increased by 1.27 times (15.6 ± 0.66 g l−1) in comparison to control cultures (11.9 ± 0.49 g l−1), when CH of P. indica was added at a concentration of 3.0% (v/v) on 15th day of cultivation. Similarly, the maximum enhancement of 1.09 times (13.4 ± 0.53 g l−1) was obtained in comparison to control cultures (12.2 ± 0.59 g l−1) on addition of CF under the same conditions. This plant growth-promoting effect of P. indica, in the present study, might be due to the presence of some metabolite released in the medium during the growth of fungal cells (Kumar et al. Citation2012). Optimized exposure time and the CH concentration were 5 days and 3.0% (v/v), respectively, for maximum biomass and artemisinin production. Comparatively, lesser increment in growth of the hairy roots was observed upon addition of CH for a shorter period, i.e. 2 days. This might be due to non-availability of sufficient time to the growth-promoting compounds present in the CH to exert their effect on hairy roots. In most of the studies, the growth-promoting effects of P. indica have been reported on pot-/field-grown or tissue culture-raised plants (Varma et al. Citation1999, Citation2001). Effect of P. indica on cell suspension cultures of Linum album was also observed (Baldi et al. Citation2008), where an increment in podophyllotoxin and 6-methoxypodophyllotoxin production by about four- and eight-fold, respectively, along with a 20% increase in biomass compared to the control cultures was recorded.
Table 5. Effect of CH of P. indica on growth and artemisinin production in hairy root cultures of A. annua L.
Table 6. Effect of CF of P. indica on growth and artemisinin production in hairy root cultures of A. annua L.
Addition of CH of P. indica at 3.0% (v/v) for an exposure time of 5 days resulted in maximum artemisinin production (15.6 ± 0.75 mg l−1), while 11.6 ± 0.45 mg l−1 artemisinin was achieved by addition of CF under same conditions. However, the CH exhibited greater elicitation potential for artemisinin (1.97 times; ) in comparison to CF (1.41 times; ) in comparison to their control cultures, respectively.
The increase in the artemisinin content could be due to the extracellular products of the fungal cells, which activated defense pathway of the hairy roots. It has been reported that the activation of plant defense pathways may be due to the secretion of some hydrolytic enzymes which are believed to have a role in defense (Pozo et al. Citation2002), or may be due to altered levels of plant growth hormones known to act as long-distance signals (Murphy et al. Citation2000). The addition of CF of Phytopthora parasitica at 0.1% (v/v) to the hairy root cultures of Cichorium intybus resulted in a 4.1-fold and 3.7-fold increase in the esculin and esculetin content, respectively (Bais et al. Citation2000).
In the present study, the enhancement of artemisinin was also found to be strongly dependent on the concentration of CH and the time of its addition. Irrespective of the biotic elicitor preparation added, an exposure time of 5 days and concentration of 3.0% (v/v) were found to be optimum for significant enhancement of artemisinin production.
MeJA and CH of P. indica gave optimum response in terms of artemisinin production from hairy root cultures of A. annua L. Based on these results, an integrated bioprocess for synergistic enhancement of artemisinin production was developed and experimentally implemented. This yield enhancement strategy resulted in artemisinin production of 19.8 ± 0.11 mg l−1, which was 2.44 times higher upon addition of selected abiotic (MeJA at 100 µM) and biotic (CH of P. indica at 3% v/v) elicitors together in comparison to the control cultures (8.1 ± 0.31 mg l−1) on 20th day of hairy root cultivation. Similarly, Sharan et al. (Citation1998) have demonstrated that MeJA along with the fungal elicitor activated the enzyme phenylalanine ammonia lyase leading to higher productions of scopoletin and scopoline in tobacco cell cultures.
Effect of FPP
The feeding of FPP to the hairy root cultures increased the growth of hairy roots of A. annua L. Hairy root growth was maximally increased by 1.06 times (13.2 ± 0.64 g l−1) in comparison to Methanol:NH4OH (7:3) solution treated control hairy root cultures (12.4 ± 0.59 g l−1) upon addition of 0.5 mg l−1 FPP (). Maximum enhancement in artemisinin production (1.25 times, 10.3 ± 0.16 mg l−1) was observed with 0.5 mg l−1 FPP () in comparison to control cultures (8.2 ± 0.43 mg l−1), while its lower and higher concentrations (0.1 and 1.0 mg l−1) resulted in slight increase in artemisinin production (7.9 ± 0.23 and 8.2 ± 0.29 mg l−1), respectively in comparison to their respective controls. As reported in , slight decrease in artemisinin content was observed with Methanol:NH4OH (7:3) solution treated hairy roots in comparison to untreated hairy roots.
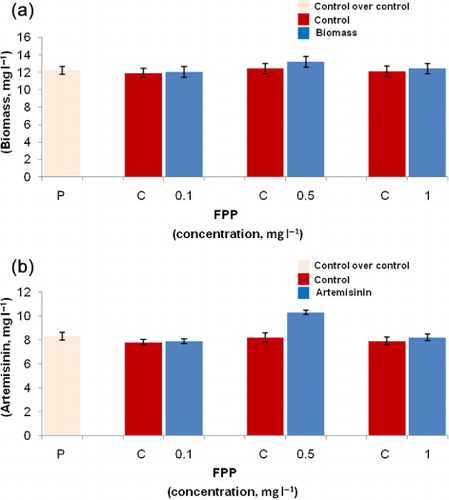
The precursor resemblance to endogenous compounds produced by the cells may have resulted in increased biomass. The artemisinin is biosynthesized through acetate-MVA pathway (Schramek et al. Citation2010). The FPP is one of the key intermediates in acetate-MVA pathway. Hence, its enhanced effect on bioproduction may be due to increased availability of precursor for the biogenesis of artemisinin. This study was also supported by G. biloba cell cultures where terpenoid biosynthesis-related precursors greatly influenced the productivity of bilobalide and ginkgolides (Kang et al. Citation2006). Bohm and Mack (Citation2004) have indicated that precursor feeding is not negative on growth as endogenous precursors are being continuously formed during metabolism in plants.
Effect of miconazole
A. annua L. hairy root cultures were also subjected to treatment with different concentrations of miconazole. Hairy root growth was maximally increased by 1.1 times (13.4 ± 0.51 g l−1) in comparison to DMSO-treated control hairy root cultures (12.1 ± 0.59 g l−1) upon addition of 20 mg l−1 miconazole (). Same concentration of miconazole resulted in 1.32 times (11.4 ± 0.41 mg l−1) increase in artemisinin concentration in comparison to control cultures (8.6 ± 0.29 mg l−1), while its lower and higher concentrations (4 and 40 mg l−1) resulted 1.07 and 1.22 times (9.0 ± 0.45 and 9.9 ± 0.42 mg l−1) increase in artemisinin production, respectively, in comparison to their respective controls (). A slight increase in artemisinin levels was also observed in hairy root cultures exposed to 4 and 20 mg l−1 DMSO (as a control).
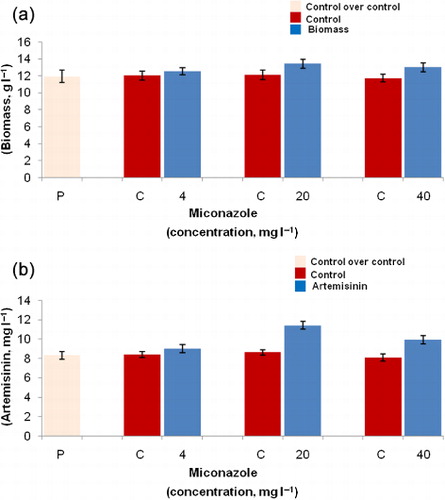
Miconazole is known to inhibit sterol biosynthesis by inhibiting sterol 14-a-demethylases and thus channeling carbon flux toward the terpenoid pathway (Kudakasseril et al. Citation1987; Zarn et al. Citation2003; Towler & Weathers Citation2007). Despite the known inhibitory effects of miconazole on specific enzymes, its effects on plant gene expression have not yet been elucidated. In A. annua L. hairy root cultures treated with 20 mg l−1 miconazole, an increase in artemisinin production was observed, indicating that miconazole could be useful to enhance artemisinin production. An increase in artemisinin level induced by DMSO was reported by Towler and Weathers (Citation2007). This group has also reported a positive effect on artemisinin production of miconazole treatment in A. annua L. seedlings, with slight chlorosis as an adverse effect. Loss of cell viability with increase in artemisinin production was recently reported in cell suspension culture of A. annua L. 200 µm miconazole induced a 2.5 times increase in artemisinin production from cell suspension culture of A. annua L. (Caretto et al. Citation2011).
Relative expression of MVA, MEP, and artmisinin biosynthetic genes influenced by stressors, precursor, and inhibitor of MVA and sterol biosynthetic pathways
The treatments selected to study the relative expression of MVA, MEP, and artemisinin biosynthetic genes were chosen on the basis of their effect on growth (biomass) and artemisinin production in hairy root culture of A. annua L. This study was done to find the correlation between the effect of the treatments and expression of MVA, MEP, and artemisinin biosynthetic genes.
The expression profiles of seven genes encoded enzymes such as (HMGR, ADS, CYP71AV1, ALDH1, DXS, DXR, and DBR2) of MVA, MEP, and artemisinin biosyntheses and one reference transcript by qPCR were studied using primer pairs listed in . The expression of these genes is summarized in and . Transcripts of all the genes studied could be detected in all treatments in different amounts using qPCR. In , expression levels in all the treatments were compared separately taking the cDNA from control hairy roots (without any treatment) as the reference sample.
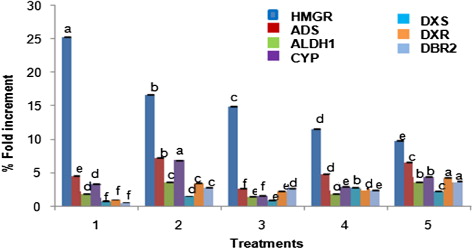
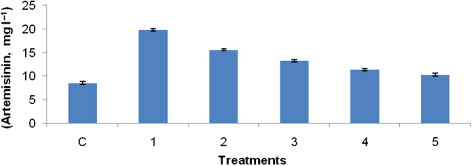
In our study, we have found that all treatments (1) hairy root culture with MeJA and P. indica together, (2) hairy root culture with P. indica, (3) hairy root culture with MeJA, (4) hairy root culture with miconazole, and (5) hairy root culture with FPP have led to increased expression of almost all the studied genes and consequently, increased production of artemisinin. Our results show that hmgr was upregulated in hairy roots with all treatments in comparison to control hairy root cultures. 4.62 and 4.79 times increment in the relative expression of ads gene was observed with treatment 1 (P. indica with MeJA) and treatment 4 (miconazole), respectively. Treatment 5 (FPP), treatment 2 (P. indica), and treatment 3 (MeJA) had shown 6.59, 7.31, and 2.74 times increase in the ads expression levels, respectively. The expression of dxs was 2.88 and 2.28 times in treatment 4 and treatment 5, respectively as compared to control. In treatments 1 and 3, dxs was downregulated, while 1.58 times upregulation was observed in treatment 2. All the analyzed genes except dxs and dbr2 were upregulated by treatment 1, whereas all the observed genes were upregulated by the treatment 2.
It has been demonstrated that A. annua L. has two independent biosynthetic pathways leading to the formation of isopentenyl diphosphate (IPP) for artemisinin production: MVA and MEP pathways (), MVA occurring in the cytosol and the MEP in the plastid (Srivastava & Akhila Citation2011). In the MVA pathway, acetyl-coenzyme A is converted to IPP through MVA, and the enzymes and genes are well studied (Miziorko Citation2011). HMGR is a rate-limiting enzyme of the mevalonate pathway (Schaller et al. Citation1995; MaujiRam et al. Citation2010) that supplies carbon for artemisinin biosynthesis, while the first committed enzyme of artemisinin biosynthesis is ADS (Bouwmeester et al. Citation1999). Different abiotic and biotic factors, precursor, inhibitor of sterol biosynthetic pathways led to the enhanced production of artemisinin and increased expression profile of the genes of MVA, MEP, and artemisinin biosynthetic pathways. The increased expression of these genes may, therefore, lead to more expression of enzymes related to artemisinin biosynthesis ultimately leading to more carbon supply and its utilization in artemisinin biosynthesis in hairy root cultures and account for higher artemisinin production. Our observations are also supported by previous studies where bioengineered A. annua L., overexpressing these genes, had higher level of artemisinin as compared to the wild-type plant (Alam & Abdin Citation2011).
CMK, 4-(Cytidine 5′-diphospho)-2-C-methyl-D-erythritol kinase; CMS, 2-C-methyl-Derythritol 4-phosphate cytidyl transferase; DXR, 1-Deoxy-D-xylulose 5-phosphate reductoisomerase; DXS, 1-Deoxy-D-xylulose 5-phosphate; HDDS, 1-Hydroxy-2-methyl-2-(E)-butenyl 4-diphosphate synthase; HMGR, 3-hydroxy-3-methylglutaryl-CoA reductase; IDS, isopentenyl diphosphate/Dimethylallyl diphospahte synthase; IPPi, isopentenyl diphosphate isomerase; MCS, 2-C-Methyl-D-erythritol 2, 4-cyclodiphosphate synthase; MDD, mevalonate diphosphate decarboxylase; MK, mevalonate kinase; MPK, mevalonate 5-phosphate kinase; SQS, Squalene synthase.
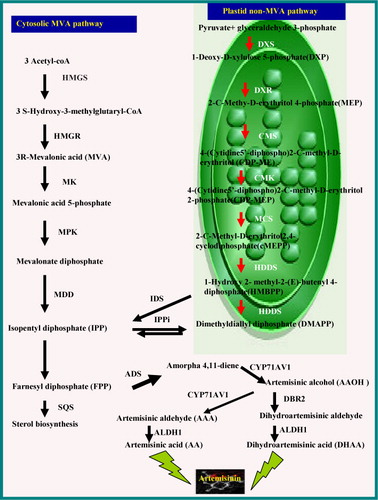
Real-time PCR results suggest that the artemisinin biosynthesis pathway is preferentially favored, indicated by the significant accumulation of enzyme transcripts related to this pathway in P. indica-treated samples compared with the control samples, which results in the upregulation of MVA, MEP, and artemisinin biosynthetic pathways genes, eventually leading to the enhanced biosynthesis of artemisinin, as evidenced by HPLC analysis. Perhaps, the induction of HMGR activity by pathogen infection and elicitor treatment mainly occurs in plant species, which resort to the MVA pathway for the biosynthesis of phytoalexins such as sesquiterpenes. For example, fungal elicitor-induced HMGR activity has been observed in potato tuber tissues (Bianchini et al. Citation1996).
MeJA is considered a key compound in the signal transduction pathway, involved in the induction of low-molecular weight compounds that take part in several plant responses (Creelman & Mullet Citation1997). Several reports have shown that exogenous application of MeJA can induce expression of plant genes for various biosynthetic pathways (Gundlach et al. Citation1992; Pauwels et al. Citation2008). Similar stimulatory effects of MeJA were also reported by Putalun et al. (Citation2007) in A. annua L. hairy root cultures, Baldi and Dixit (Citation2008) in cell cultures of A. annua L., by Liu et al. (Citation2009) in A. annua L. plants treated with jasmonic acid, and recently by Caretto et al. (Citation2011) in cell suspension culture of A. annua L. In our hairy root cultures, along with the artemisinin increase, expression of the cyp71av1 gene was also significantly enhanced in response to MeJA treatment. This gene encodes a monooxygenase enzyme that catalyses the three-step oxidation of amorpha-4,11-diene to artemisinic acid (Teoh et al. Citation2006). However, expression of dxs gene was not enhanced in the MeJA-treated samples. This could indicate that the cell culture mRNA levels were not rate limiting for artemisinin biosynthesis, but further experiments are needed to draw definitive conclusions. On the basis of these results, cyp71av1, being regulated at the transcriptional level by MeJA, could be a suitable candidate for metabolic engineering strategies to improve artemisinin production.
Conclusions
Hairy root cultures of A. annua L. offer continuous production of artemisinin with high growth potential. MeJA and P. indica, when applied together, show high efficiency at low concentrations in terms of artemisinin production by hairy root cultures. The use of MeJA and other signaling compounds for elicitation of transformed root cultures is opening new ways for a possibly profitable in vitro secondary metabolite production. To the best of our knowledge, this is the first report on the effect of endophytic fungus for the enhanced production of artemisinin in hairy root cultures of A. annua L. Large-scale culture feasibility and long-term stability make this biotechnological approach not only a reliable source of secondary metabolites (Peebles et al. Citation2007), but also an effective tool to study the biosynthetic pathways (Robins Citation1998). However, scaling up hairy roots to industrial levels poses a great challenge at the moment. The efficiency of the scaling up systems still needs optimization before industrial exploitation becomes viable.
Acknowledgments
This study was supported by a research grant to Prof. M. Z. Abdin from the Life Sciences Research Board, DRDO, Government of India and UGC-SAP. Seema Ahlawat is thankful to Department of Science and Technology, Government of India, for providing WoS-A Fellowship.
References
- Aftab T, Khan MMA, Idrees M, Naeem M, Moinuddin, Hashmi N, Varshney L. 2011. Enhancing the growth, photosynthetic capacity and artemisinin content in Artemisia annua L. by irradiated sodium alginate. Radiat Phys Chem. 80:833–836.
- Aftab T, Khan MMA, Idrees M, Naeem M, Singh M, MaujiRam. 2010. Stimulation of crop productivity, photosynthesis and artemisinin production in Artemisia annua L. by triacontanol and gibberellic acid application, J Plant Interact. 5:273–281. 10.1080/17429141003647137
- Ahlawat S, Saxena P, MaujiRam, Alam P, Nafis T, Mohd A, Abdin MZ. 2012. Influence of Agrobacterium rhizogenes on induction of hairy roots for enhanced production of artemisinin in Artemisia annua L. plants. Afr J Biotechnol. 11:8684–8691.
- Alam P, Abdin MZ. 2011. Over-expression of HMG-CoA reductase and amorpha-4,11-diene synthase genes in Artemisia annua L. and its influence on artemisinin content. Plant Cell Rep. 30:1919–1928. 10.1007/s00299-011-1099-6
- Alam P, Kamaluddin, Khan MA, Mohammad A, Khan R, Abdin MZ. 2014. Enhanced artemisinin accumulation and metabolic profiling of transgenic Artemisia annua L. plants over-expressing by rate-limiting enzymes from isoprenoid pathway. J Plant Interact. 9:655–665. 10.1080/17429145.2014.893030
- Bais HP, Govindaswamy S, Ravishankar GA. 2000. Enhancement of growth and coumarin production in hairy root cultures of Witloof chicory (Cichorium intybus L. cv Lucknow local) under the influence of fungal elicitors. J Biosci Bioeng. 90:648–653.
- Bais HP, Sudha G, George J, Ravishankar GA. 2001. Influence of exogenous hormones on growth and secondary metabolite production in hairy root cultures of Cichorium intybus L. cv. Lucknow Local. In Vitro Cell Dev Biol Plant. 37:293–299. 10.1007/s11627-001-0052-8
- Baldi A, Dixit VK. 2008. Yield enhancement strategies for artemisinin production by suspension cultures of Artemisia annua. Bioresource Technol. 99:4609–4614. 10.1016/j.biortech.2007.06.061
- Baldi A, Jain A, Gupta N, Srivastava AK, Bisaria VS. 2008. Co-culture of mycorrhiza-like fungi (Piriformospora indica and Sebacina vermifera) with plant cells of Linum album for enhanced production of podophyllotoxins: a first report. Biotechnol Lett. 30:1671–1677. 10.1007/s10529-008-9736-z
- Bertea CM, Freije JR, Van der WH, Verstappen FW, Perk L, Marwquez V, Kraker JW, Posthumus MA, Jansen B. 2005. Identification of intermediates and enzymes involved in the early steps of artemisinin biosynthesis in Artemisia annua. Planta Med. 71:40–47.
- Bianchini GM, Stermer BA, Paiva NL. 1996. Induction of early mevalonate pathway enzymes and biosynthesis of end products in potato (Solanum tuberosum) tubers by wounding and elicitation. Phytochemistry. 42:1563–1571. 10.1016/0031-9422(96)00139-2
- Bohm H, Mack G. 2004. Betaxanthin formation and free amino acids in hairy roots of Beta vulgaris var. lutea depending on nutrient medium and blutamate or glutamine feeding. Phytochemistry. 65:1361–1368. 10.1016/j.phytochem.2004.03.008
- Borrmann S, Szlezák N, Faucher JF, Matsiegui PB, Neubauer R, Binder R, Lell B, Kremsner PG. 2001. Artesunate and praziquantel for the treatment of Schistosoma haematobium infections: a double-blind, randomized, placebo-controlled study. J Infect Dis. 184:1363–1366. 10.1086/324004
- Bouwmeester HJ, Wallaart TE, Janssen MH, van Loo B, Jansen BJ, Posthumus MA, Schmidt CO, de Kraker JW, Knig WA, Franssen MC, et al. 1999. Amorpha-4,11-diene synthase catalyses the first probable step in artemisinin biosynthesis. Phytochemistry. 52:843–854. 10.1016/S0031-9422(99)00206-X
- Camilleri C, Jouanin L. 1991. The Tr-DNA region carrying the auxin synthesis genes of the Agrobacterium rhizogenes agropine-type plasmid pRiA4: nucleotide sequence analysis and introduction in to tobacco plants. Mol Plant Microbe In. 4:155–162.
- Caretto S, Quarta A, Durante M, Nisi R, de Paolis A, Blando F, Mita G. 2011. Methyl jasmonate and miconazole differently affect arteminisin production and gene expression in Artemisia annua suspension cultures. Plant Biol. 13:51–58. 10.1111/j.1438-8677.2009.00306.x
- Creelman RA, Mullet J. 1997. Biosynthesis and action of jasmonates in plants. Annu Rev Plant Physiol Plant Mol Biol. 48:355–381.
- Efferth T, Dunstan H, Sauerbrey A, Miyachi H, Chitambar CR. 2001. The anti-malarial artesunate is also active against cancer. Int J Oncol. 18:767–773.
- Gamborg OL, Miller RA, Ojima K. 1968. Nutrient requirements of suspension cultures of soybean root cells. Exp Cell Res. 50:151–158. 10.1016/0014-4827(68)90403-5
- Giri A, Ravindra ST, Dhingra V, Narasu ML. 2001. Influence of different strains of Agrobacterium rhizogenes on induction of hairy roots and artemisinin production in Artemisia annua. Curr Sci. 81:378–382.
- Guillon S, Tremouillaux GJ, Pati PK, Rideau M, Gantet P. 2006. Hairy root research: recent scenario and exciting prospects - Commentary. Curr Opin Plant Biol. 9:341–346. 10.1016/j.pbi.2006.03.008
- Gundlach H, Muller MJ, Kutchan TM, Zenk MH. 1992. Jasmonic acid is a signal transducer in elicitor-induced plant cell cultures. Proc Natl Acad Sci. 89:2389–2393. 10.1073/pnas.89.6.2389
- Hamill JD, Lidgett JA. 1997. Hairy root cultures – Opportunities and key protocols for studies in metabolic engineering. In: Doran PM, editor. Hairy roots-culture and applications. Amsterdam (The Netherlands): Harwood Academic Publishers; p. 1–31.
- Haynes RK. 2006. From artemisinin to new artemisinin antimalarials: biosynthesis, extraction, old and new derivatives, stereochemistry and medicinal chemistry requirements. Curr Top Med Chem. 6:509–537.
- Jaleel CA, Manivannan P, Wahid A, Farooq M, Somasundaram R, Panneerselvam R. 2009. Drought stress in plants: A review on morphological characteristics and pigments composition. Int J Agr Biol. 11:100–105.
- Kaefer E. 1977. Meiotic and mitotic recombination in Aspergillus and its chromosomal aberrations. Adv Genet. 19:33–131.
- Kang SM, Min JY, Kim YD, Park DJ, Jung HN, Karigar CS, Ha YL, Kim SW, Choi MS. 2006. Effect of supplementing terpenoid biosynthetic precursors on the accumulation of bilobalide and ginkgolides in Ginkgo biloba cell cultures. J Biotechnol. 123:85–92. 10.1016/j.jbiotec.2005.10.021
- Ketchum REB, Gibson DM, Croteau RB, Shuler ML. 1999. The kinetics of taxoid accumulation in cell suspension culture of taxus following elicitation with methyl jasmonate. Biotechnol Bioeng. 62:97–105. 10.1002/(SICI)1097-0290(19990105)62:1%3C97::AID-BIT11%3E3.0.CO;2-C
- Kim Y, Wyslouzil BE, Weathers PJ. 2002. Secondary metabolism of hairy root cultures in bioreactors. In Vitro Cell Dev Biol Plant. 38:1–10. 10.1079/IVP2001243
- Kiong ALP, Mahmood M, Fadzillah NM, Daud SK. 2005. Effects of precursor supplementation on the production of triterpenes by Centella asiatica Callus Cultures. Pakistan J Biol Sci. 8:1160–1169. 10.3923/pjbs.2005.1160.1169
- Kudakasseril GJ, Lam L, Staba EJ. 1987. Effect of sterol inhibitors on the incorporation of 14C-isopentenyl pyrophosphate into artemisinin by a cell-free system from Artemisia annua tissue cultures and plants. Planta Med. 53:280–284. 10.1055/s-2006-962706
- Kumar V, Rajauria G, Sahai V, Bisaria VS. 2012. Culture filtrate of root endophytic fungus Piriformospora indica promotes the growth and lignan production of Linum album hairy root cultures. Process Biochem. 47:901–907. 10.1016/j.procbio.2011.06.012
- Lacroix B, Tzfira T, Vainstein A, Citovsky V. 2006. A case of promiscuity: Agrobacterium's endless hunt for new partners. Trends Genet. 22:29–37. 10.1016/j.tig.2005.10.004
- Liu C, Wang Y, Xu X, Ouyang F, Ye H, Li G. 1999. Improvement of artemisinin accumulation in hairy root cultures of Artemisia annua L by fungal elicitor. Bioproc Biosyst Eng. 20:161–164.
- Liu C, Zhao Y, Wang Y. 2006. Artemisinin: current state and perspectives for biotechnological production of an antimalarial drug. Appl Microbiol Biotechnol. 72:11–20. 10.1007/s00253-006-0452-0
- Liu CZ, Guo C, Wang YC, Ouyang F. 2002. Effect of light irradiation on hairy root growth and artemisinin biosynthesis of Artemisia annua L. Process Biochem. 38:581–585. 10.1016/S0032-9592(02)00165-6
- Liu CZ, Wang YC, Ouyang F, Ye HC, Li GF. 1997. Production of artemisinin by hairy root cultures of Artemisia annua L. Biotechnol Lett. 19:927–930. 10.1023/A:1018362309677
- Liu S, Tian N, Li J, Huang J, Liu Z. 2009. Isolation and identification of novel genes involved in artemisinin production from flowers of Artemisia annua using suppression subtractive hybridization and metabolite analysis. Planta Med. 14:1542–1547. 10.1055/s-0029-1185809
- Luo XD, Shen CC. 1987. The chemistry, pharmacology, and clinical applications of qinghaosu (Artemisinin) and its derivatives. Med Res Rev. 7:29–52. 10.1002/med.2610070103
- MaujiRam, Khan MA, Jha P, Khan S, Kiran U, Ahmad MM, Javed S, Abdin MZ. 2010. HMG-CoA reductase limits artemisinin biosynthesis and accumulation in Artemisia annua L. plants. Acta Physiol Plant. 32:859–866.
- Miziorko HM. 2011. Enzymes of the mevalonate pathway of isoprenoid biosynthesis. Arch Biochem Biophys. 505:131–143. 10.1016/j.abb.2010.09.028
- Murashige T, Skoog F. 1962. A revised medium for rapid growth and bio assays with tobacco tissue cultures. Plant Physiol. 15:473–497. 10.1111/j.1399-3054.1962.tb08052.x
- Murphy JG, Rafferty SM, Cassells AC. 2000. Stimulation of wild strawberry (Fragaria vesca) arbuscular mycorrhizas by addition of shellfish waste to the growth substrate: interaction between mycorrhization, substrate amendment and susceptibility to red core (Phytophthora fragariae). Appl Soil Ecol. 15:153–158. 10.1016/S0929-1393(00)00091-3
- Namdeo A, Patil S, Fulzele DP. 2002. Influence of fungal elicitors on production of ajmalicine by cell cultures of Catharanthus roseus. Biotechnol Prog. 18:159–162. 10.1021/bp0101280
- Oelmuller R, Sherameti I, Tripathi S, Varma A. 2009. Piriformospora indica, a cultivable root endophyte with multiple biotechnological applications. Symbiosis. 49:1–17. 10.1007/s13199-009-0009-y
- Patra N, Srivastava AK, Sharma S. 2013. Study of various factors for enhancement of artemisinin in Artemisia annua hairy roots. Int J Chem Eng App. 4:157–160.
- Pauwels L, Morreel K, De Witte E, Lammertyn F, Van Montagu M, Boerjan W, Inze D, Goossens A. 2008. Mapping methyl jasmonate-mediated transcriptional reprogramming of metabolism and cell cycle progression in cultured Arabidopsis cells. Proc Natl Acad Sci. 105:1380–1385. 10.1073/pnas.0711203105
- Peebles CA, Gibson SI, Shanks JV, San KY. 2007. Long-term maintenance of a transgenic Catharanthus roseus hairy root line. Biotechnol Prog. 23:1517–1518. 10.1021/bp0702166
- Pozo M, Cordier C, Dumas-Gautod E, Gianinazzi SJ, Barea JM, Azcón-Aguilar C. 2002. Localized versus systemic effect of arbuscular mycorrhizal fungi on defence responses to Phytophthora infection in tomato plants. J Exp Bot. 53:525–534. 10.1093/jexbot/53.368.525
- Putalun W, Luealon W, De-Eknamkul W, Tanaka H, Shoyama Y. 2007. Improvement of artemisinin production by chitosan in hairy root cultures of Artemisia annua L. Biotech Lett. 29:1143–1146. 10.1007/s10529-007-9368-8
- Rao SR, Ravishankar GA. 2002. Plant cell cultures: chemical factories of secondary metabolites. Biotechnol Adv. 20:101–153. 10.1016/S0734-9750(02)00007-1
- Rijhwani S, Shanks JV. 1998. Effect of elicitor dosage and exposure time on biosynthesis of indole alkaloids by Catharanthus roseus hairy root cultures. Biotechnol Prog. 14:442–449. 10.1021/bp980029v
- Ro DK, Paradise EM, Ouellet M, Fisher KJ, Newman KL., Ndungu JM, Ho KA, Eachus RA, Ham TS, Kirby J, et al. 2006. Production of the antimalarial drug precursor artemisinic acid in engineered yeast. Nature. 440:940–943. 10.1038/nature04640
- Robins RJ. 1998. The application of root cultures to problems of biological chemistry. Nat Prod Rep. 15:549–570. 10.1039/a815549y
- Roewer IA, Cloutier N, Nessler CL, Luca V. 1992. Transient induction of tryptophan decarboxylase (TDC) and strictosidine synthase (SS) genes in cell suspension cultures of Catharanthus roseus. Plant Cell Rep. 11:86–89. 10.1007/BF00235259
- Romero MR, Efferth T, Serrano MA, Castaño B, Macias RL, Briz O, Marin JJ. 2005. Effect of artemisinin/artesunate as inhibitors of hepatitis B virus production in an “in vitro” system. Antiviral Res. 68:75–83. 10.1016/j.antiviral.2005.07.005
- Sanz MK, Hernandez XE, Tonn CE, Guerreio E. 2000. Enhancement of tessaric acid production in Tessaria absinthioides cell suspension cultures. Plant Cell Rep. 19:821–824. 10.1007/s002990000192
- Savitha BC, Thimmaraju R, Bhagyalakshmi N, Ravishankar GA. 2006. Different biotic and abiotic elicitors influence betalain production in hairy root cultures of Beta vulgaris in shake-flask and bioreactor. Process Biochem. 41:50–60. 10.1016/j.procbio.2005.03.071
- Schaller H, Grausem B, Benveniste P, Chye ML, Tan CT, Song YH, Chua NH. 1995. Expression of the Hevea brasiliensis (H.B.K.) müll. Arg. 3-hydroxy-3-methylglutaryl coenzyme A reductase 1 in tobacco results in sterol overproduction. Plant Physiol. 109:761–770.
- Schramek N, Wang H, Romisch-Margl W, Keil B, Radykewicz T, Winzenhörlein B, Beerhues L, Bacher A, Rohdich F, Gershenzon J, et al. 2010. Artemisinin biosynthesis in growing plants of Artemisia annua. A 13CO2 study. Phytochemistry. 71:179–187. 10.1016/j.phytochem.2009.10.015
- Sen R, Bandyopadhyay S, Dutta A, Mandal G, Ganguly S, Saha P, Chatterjee M. 2007. Artemisinin triggers induction of cell-cycle arrest and apoptosis in Leishmania dovani promastigotes. J Med Microbiol. 56:1213–1218. 10.1099/jmm.0.47364-0
- Sharan M, Taguchi G, Gonda K, Jouke T, Shimosaka M, Hayashida N. 1998. Effects of methyl jasmonate and elicitor on the activation of phenylalanine ammonia-lyase and the accumulation of scopoletin and scopolin in tobacco cell cultures. Plant Sci. 132:13–19. 10.1016/S0168-9452(97)00260-4
- Sharma G, Agrawal V. 2013. Marked enhancement in the artemisinin content and biomass productivity in Artemisia annua L. shoots co-cultivated with Piriformospora indica. World J Microbiol Biotechnol. 29:1133–1138. 10.1007/s11274-013-1263-y
- Singh A, Rajpal K, Singh M, Kharkwal AC, Arora M, Varma A. 2013. Mass cultivation of Piriformospora indica and Sebacina species. In: Varma A, Kost G, Oelmüller R, editors, Piriformospora indica, Sebacinales and their biotechnological applications. Germany: Springer-Verlag; p. 377–392.
- Srivastava N, Akhila A. 2011. Biosynthesis of artemisinin––revisited. J Plant Interact. 6:265–273. 10.1080/17429145.2011.570869
- Suresh B, Thimmaraju R, Bhagyalakshmi N, Ravishankar GA. 2004. Polyamines and methyl jasmonate-influenced enhancement of betalain production in hairy root cultures of Beta vulgaris grown in a bubble column reactor and studies on efflux of pigments. Process Biochem. 39:2091–2096. 10.1016/j.procbio.2003.10.009
- Tang K, Shen Q, Yan T, Fu X. 2014. Transgenic approach to increase artemisinin content in Artemisia annua L. Plant Cell Rep. 33:605–615. 10.1007/s00299-014-1566-y
- Tenea GN, Calin A, Gavrila L, Cucu N. 2008. Manipulation of root biomass and biosynthetic potential of Glycyrrhiza glabra L. plants by Agrobacterium rhizogenes mediated transformation. Rom Biotechnol Lett. 13:3922–3932.
- Teoh KH, Polichuk DR, Reed DW, Nowak G, Covello PS. 2006. Artemisia annua L. (Asteraceae) trichome-specific cDNAs reveal CYP71AV1, a cytochrome P450 with a key role in the biosynthesis of the antimalarial sesquiterpene lactone artemisinin. FEBS Lett. 580:1411–1416. 10.1016/j.febslet.2006.01.065
- Towler MJ, Weathers PJ. 2007. Evidence of artemisinin production from IPP stemming from both the mevalonate and the nonmevalonate pathways. Plant Cell Rep. 26:2129–2136. 10.1007/s00299-007-0420-x
- Varma A, Singh A, Sudha, Sahay NS, Sharma J, Roy A, Kumari M, Rana D, Thakran S, Deka D, et al. 2001. Piriformospora indica: an axenically culturable mycorrhiza-like endosymbiotic fungus. In: Hock B, editor. Fungal associations. Berlin, Heidelberg, New York: Springer; p. 123–150.
- Varma A, Verma S, Sudha Sahay N, Bütehorn B, Franken P. 1999. Piriformospora indica, a cultivable plant-growth-promoting root endophyte. Appl Environ Microbiol. 65:2741–2744.
- Waller F, Achatz B, Baltruschat H, Fodor J, Becker K, Fischer M, Heier T, Hückelhoven R, Neumann C, von Wettstein D, et al. 2005. The endophytic fungus Piriformospora indica reprograms barley to salt-stress tolerance, disease resistance and higher yield. Proc Natl Acad Sci USA 102:13386–13391. 10.1073/pnas.0504423102
- Wang H, Ma C, Li Z, Ma L, Wang H, Ye H, Xu G, Liu B. 2009. Effects of exogenous methyl jasmonate on artemisinin biosynthesis and secondary metabolites in Artemisia annua L. Ind Crop Prod. 31:214–218. 10.1016/j.indcrop.2009.10.008
- Wang JW, Xia ZH, Tan RX. 2002. Elicitation on artemisinin biosynthesis in Artemisia annua hairy roots by the oligosaccharide extract from the endophytic Colletotrichum sp. B501. Acta Bot Sinica. 44:1233–1238.
- Wang JW, Zheng LP, Tan RX. 2006. The preparation of an elicitor from a fungal endophyte to enhance artemisinin production in hairy root cultures of Artemisia annua L. Sheng Wu Gong Cheng Xue Bao. 22:829–834.
- White NJ. 2008. Qinghaosu (artemisinin): the price of success. Science. 320:330–334. 10.1126/science.1155165
- Xie D, Ye H, Li G, Guo Z, Zou Z, Guo Z. 2001. Selection of hairy root clones of Artemisia annua L. for artemisinin production. Isr J Plant Sci. 49:129–134. 10.1560/N11N-6BLG-ER7C-XKCT
- Zarn JA, Bru schweiler BJ, Schlatter JR. 2003. Azole fungicides affect mammalian steroidogenesis by inhibiting sterol 14-ademethylase and aromatase. Environ Health Persp. 111:255–261. 10.1289/ehp.5785
- Zhao SS, Zeng MY. 1986. Determination of Qinghaosu in Artemisia annua L. by high performance liquid chromatography. Chin J Pharm Ana. 6:3–5.
- Zheng LP, Guo YT, Wang JW, Tan RX. 2008. Nitric oxide potentiates oligosaccharide-induced artemisinin production in Artemisia annua hairy roots. J Intg Plant Biol. 50:49–55.