Abstract
TaLr35PR5 gene was obtained from the gDNA and cDNA of TcLr35 wheat. It was induced by Puccinia triticina, ABA and SA, but TaLr35PR5 was induced earlier and its expression level was higher in the incompatible interaction than that in the compatible interaction. In addition, the accumulations of TaLr35PR5 increased stably and showed significant peak challenged by P. triticina at different growth and development periods of TcLr35 wheat while it maintained similar level and changed little in mock inoculated. Western blottings were conducted to confirm that TaLr35PR5 be induced by P. triticina infection at the protein expression level. Similar to the expression patterns of TaLr35PR5 at RNA levels, the accumulations of TaLr35PR5 protein were weak in the seedling stage, then increased to the peak and kept constant levels at the mature stage which is consistent with the expression feature of Lr35 gene as an adult plant resistance gene.
1. Introduction
Wheat (Triticum aestivum L.) leaf rust caused by Puccinia triticina is one of the most serious diseases worldwide, leading to considerable losses and causing pandemic in the short term in wheat production. Presently, wheat leaf rust disease has attracted worldwide attention, and various researches had been conducted on wheat symptoms, epidemiology and physiological races of the P. triticina fungus. Development of genetic resistance to rust has been approved as the most efficient, cost-effective and environment-friendly approach to prevent the losses caused by rust epidemics (Singh et al. Citation2004). However, single R gene tends to be quickly overcome by changes in the P. triticina population. More durable resistance can be achieved by gene pyramiding, the stacking of multiple leaf rust genes, such as the Canadian cultivar Pasqua(Liu and Kolmer Citation1998; Kolmer Citation2001). Therefore, the application of transgenic technology to realize directional improvement and molecular assisted breeding technology linked to target trait provide an alternative way to obtain resistant varieties, which can overcome the above-mentioned problems related to conventional breeding.
To survive and adapt to their native surroundings, plants develop the mechanism that detect and rapidly respond to invasions of phytopathogens and arthropods with coordinated and/or integrated gene expression. Resistance genes and defense response gene are two main types of genes mediating defense responses. In host-pathogen interactions, various novel proteins, referred to as pathogenesis-related proteins (PR), are induced systemically and accumulated locally both at the site of infection and in distal parts of the plants, leading to development of the hypersensitive reaction (HR) and systemic acquired resistance (SAR) (Hammond-Kosack and Jones Citation1996). PR proteins are defined as proteins coded by the host plant but induced specifically in pathological or related situations (Hamamouch et al. Citation2011). Induction of PRs has been found in many plant species belonging to various families, PR1–PR17 (Jan et al. Citation2008), playing important roles in the resistance of the host against the pathogen and biotic stress conditions. Meanwhile, an increasing body of research suggests that they exist as constitutive components in different plant organs, irrespective of stress conditions (Borad and Sriram Citation2008). Analysis of the expression patterns of PR genes would be useful to understand their possible functions and for use as characteristic markers in studies on plant defense reactions.
In different families of PRs, PR5 family is known as thaumatin-like proteins (TLPs) because the amino acid sequences of these proteins exhibit extensive homology with that of thaumatin, a sweet-tasting protein derived from Thaumatococcus daniellii (Eden et al. Citation1982). PR5 proteins of the high molecular weight group exhibit antifungal activities and are found in cell vacuoles. The smaller TLPs located in the extracellular are also involved in plant defense against fungal infection (Chan et al. Citation1998; Wang et al. Citation2013), their amino acid sequences share a certain degree of homology, and the research on the proteins encoded by PR5 is a ‘hot spot’ in the area of resistance of plant. PR5 genes have been identified from many plants, such as Prunus domestica (El-kereamy et al. Citation2011), Brassica rapa (Ahmed et al. Citation2013), corn (Liu et al. Citation2011), poplar (Lei et al. Citation2012), and so on, and proved that PR5 protein could obviously improve plant disease resistance. For example, over expression of thaumatin-like protein gene from Pyrus pyrifolia and in tobacco increased resistance to pathogenic fungito (Liu et al. Citation2012); rice TLP-D34 enhanced resistance to the sheath blight pathogen Rhizoctonia solani (Datta et al. Citation1999); rice TLP enhanced resistance to the head blight pathogen Fusarium graminearum (Mackintosh et al. Citation2007). Furthermore, expression changes in fungal-related genes between compatible and incompatible fungal strains (rust fungus Melampsora larici-populina) in P. trichocarpa 9P. deltoides ‘Beaupre’ indicated that the gene could strongly induced transcript coding PR-5 protein showed a 28.8-fold induction based on cDNA microarray (Rinaldi et al. Citation2007). Dharmendra Singh et al. (Citation2012) examined 5 target genes encoding for chitinase 3, β-1, 3/1, 4 glucanase, thaumatin-like protein, peroxidase 2 mitogen activated protein kinase 1 to unravel their coordinated action during compatible and incompatible interaction, and attributed the differences to the presence of seedling leaf rust resistance Lr28 gene, which facilitated prevention of leaf rust infection in resistant wheat plants.
Although many PR5 genes have been cloned and reported to be involved in plant disease resistance responses and SAR, the role of PR5 genes in resistance in wheat to leaf rust is not fully understood and so far there isn’t any report to prove that PR5 be related to the wheat growth and adult plant resistance (APR). Wheat leaf rust resistance gene, Lr35, provides an important source of partial resistance that is expressed in adult plants during the critical grain-filling stage and is the most effective in flag leaf. Understanding the molecular nature of this kind of resistance has important implications for long-term control of rust and other diseases. Previous studies showed that the gene was originally introgressed into chromosome 2B from Aegilops speltoides, a diploid relative of wheat (Kerber and Dyck Citation1990), and localized between the two markers BCD260 and UBC836 by RFLP and ISSR (Seyfarth et al. Citation1999). As this gene has not yet been used in wheat breeding programs, it is still effective against wheat leaf rust worldwide. Its resistance expression starts from the second leaf stage, fully expressed till six leaf stage, and it is a very effective APR gene, also known as ‘slow rusting’ confers a broad spectrum of durable resistance. In this study, we isolated a PR5 gene, designated TaLr35PR5, from wheat near-isogenic lines TcLr35 infected by P. triticina. To further identify the relationship between TaLr35PR5 and Lr35-mediated resistance response to leaf rust, the expression profiles of TaLr35PR5 induced by pathogen, SA and ABA were measured temporally and spatially in wheat tissues at the transcript and protein levels. These results will provide systematic dada for further study the function and the mechanism of TaLr35PR5 in defensing adversity stress.
2. Materials and methods
2.1. Plant material, inoculation system and ABA/SA elicitor pre-treatments
Wheat near-isogenic line TcLr35, Thatcher and leaf rust race 07-10-426-1(FHNT) were the biological materials used for gene cloning and expression analyses. Based on the gene-for-gene model, wheat TcLr35 was resistant to leaf rust race 07-10-426-1 and showed incompatible reaction (type 1). But, Thatcher was susceptible to leaf rust race 07-10-426-1 and showed compatible reaction (type 4) according to Roelfs standard (Citation1984). The plants were grown under ideal conditions of temperature (20°C), relative humidity (80%), and light periods (16 h day with 350 lux light, 8h dark periods) in the greenhouse. Wheat seedlings were grown in the greenhouse about 4 months when up to mature-plant stage. Fresh uredospores collected from the susceptible wheat cv. Zhengzhou5389 were applied to the surface of the primary leaves of TcLr35 and Thatcher with a brush. After inoculation, the plants were kept at 100% relative humidity environment in the dark for 24 hours at 20°C, and then moved to the greenhouse. Inoculated wheat leaves were excised at 0, 6, 24, 36, 48, 72, 96, and 120 hour post inoculation (hpi) for expression analyses in the compatible interaction and incompatible interaction. Inoculated wheat leaves, root and stem were excised at 0, 12, 24, 36, 48, 72, 96, and 120 hpi for temporal and spatial expression analyses when the adult stage. Wheat leaves at different growth periods including seedling stages [one-leaf stage (1 week), two-leaf stage (2 weeks), trefoil stage (3–4 weeks)], tillering stage (4–5 weeks), booting stage (10–11 weeks), flowering stage (13–15 weeks) and mature stage (16–18 weeks) were inoculated at expression peak (96 hpi). Leaves samples collected at 0, 12, 24, 48, 72, 96, 120, 144, and 168 hpi were used to analyze the expression profiles at protein levels while samples at expression peak (144 hpi) were used to analyze the accumulation of TaLr35PR5 at different growing stages of wheat, and noninoculation treatment (inoculation with water) as control.
According to the method of Zambounis et al. (Citation2012) and our pre-experiments, the adult wheat leaves were drenched with 50µmol/L SA and 50µmol/L ABA prior to leaf rust pathogen inoculation. Tween 20 (0.01%) was added as a surfactant (Fisher Scientific, Nepean, ONT, Canada). Afterward, the seedlings were placed on a greenhouse bench at 20°C for three days and then inoculated with Puccinia triticina. All samples collected at 0, 12, 24, 48, 72, and 120 hpi were immediately frozen in liquid nitrogen and stored at −80°C prior to the extraction of total RNA.
2.2. Cloning and expression of TaLr35PR5
Genomic DNA (gDNA) was extracted from leaves of wheat TcLr35 using a modified CTAB method (Saghai-Maroof et al. Citation1984). Total RNA was extracted from wheat leaves at different time points from 0 to 120 hpi according to the protocol of the Triozol reagent. Subsequently, they were treated with amplification grade DNaseI (TaKaRa, Japan) to remove any residual DNA and reverse-transcript PCR was performed using SuperScript II reverse transcriptase, oligo(dT)12–18, and RNAse OUT (Invitrogen), then gDNA and cDNA were used as the templates for PCR using primer pairs TaLr35PR5-F(5′-CCCAAGCTTTCAGAAGCTGATGGGGTAGAC-3′) and TaLr35PR5-R (5′-CGGGATCCTGGGTCAGGAGCAACATCCAG-3′) designed according to the conserved domains of PR5 gene (Gabriela et al. Citation1991). PCRs were done on a thermal cycler (Applied Biosystems 2720, ABI, Foster City CA) with the following systems: 2.5 μL buffer, 0.5 μL dNTP, 0.3 μL Taq, 1.0 μL cDNA, 1.0 μL of each primer (10 μmol/L) in a total volume of 25 μL. PCR was performed according to the following amplification procedure: an initial denaturation step at 95°C 1 min, followed by 35 cycles of denaturation at 94°C 1 s, annealing at 61.6°C 30 s and extension at 72°C 2 min, sample volume loaded is 5 μL. The PCR products were detected by an agarose gel electrophoresis and cloned into pGEM-T easy vector (Sambrook et al. Citation1998). Positive clones were sequenced by the Shanghai GeneCore BioTechnologies Co., Ltd. Then we use SignalP (http://www.cbs.dtu.dk/services/SignalP/) and TMHMM (http://www.hsls.pitt.edu/obrc/index.) on line tools to analyze the Schematic structure of TaLr35PR5 gene.
Total RNA extracted from wheat leaves, steams, and roots at different time points from 0 to 120 hpi or different growing periods of wheat was used to analyze the expression profiles of TaLr35PR5. Wheat glyceraldehyde 3-phosphate dehydrogenase gene (GAPDH, GenBank accession No. AF251217) amplified by primer pairs GAPDH-F (5′-AACTGCCTTGCTCCTCTTGC-3′) and GAPDH-R (5′-CTGTTGTCACCCTGGAAGTCA-3′) was selected as an endogenous control to normalize differences in input RNAs and check efficiencies of reverse transcription among the various samples. Genetools (SYNGENE, USA) and Statistical Package for the Social Sciences (SPSS) software (IBM, USA) were used to calculate the experiment data and analyze the average and standard deviation respectively in order to get the expression levels of TaLr35PR5 relative to noninoculated control. Three independent biological replicates were performed for each sample.
2.3. Expression of recombinant protein
The ORF of TaLr35PR5 without signal peptide was amplified by PCR using the primer pairs YTaLr35PR5-R (5′-CCCAAGCTTTCATGGACAGAAGGTGATCTGGTA-3′) and YTaLr35PR5-F (5′-CGCGGATCCATGGCGACCTCCGCGGTGCTC-3′). PCR products were cloned into pEASY-E1 vector according to the instructions of pEASY-E1 expression kit (TransGen). The positive recombinant plasmid pEASY-TaLr35PR5 was identified by PCR and transformed into E. coli strain BL21 (DE3). Single colony was cultured in liquid Luria-Bertani (LB) medium (with 50 mg/mL ampicillin) at 37°C overnight, and inoculated to a new LB liquid medium until the culture reached an optical density of 0.5–0.8 at a 600 nm wavelength spectrophotometer in order to identify condition of the optimal protein production, a series of concentrations of isopropyl-b-D-thiogalactopyranoside (IPTG: 0, 0.01, 0.1, 0.3, 0.5, 0.8, and 1.0 mmol/L) and induction times (2, 4, 6, 8, 10 h, and overnight were set. The expression of recombinant protein was induced at the optimal condition and pured with the Ni-Agarose HIS trap column (TransGen, China). The targeted protein were analyzed by SDS-PAGE with 15% poly-acrylamide gels and stained with coomassie brilliant blue R-250.
2.4. Antibody preparation and immunodetection
The preparation of antibody was entrusted to the Biology Institute of Academy of Sciences of Hebei Province. Purified recombinant protein (2 mg) was injected into rabbits to produce antibodies. The titer of antibody was monitored by indirect ELISA. The rabbit antiserum was collected when the antibody reached a high level. The proteins of wheat leaves with different treatments were extracted according to instruction of one step plant active protein extraction kit (Sangon). For western blotting analysis, approximately 10μg of protein per lane was separated using SDS-PAGE and electroblotted to a PVDF membrane (Sangon) for 45 min at 100 V. The nonspecific binding sites on the membrane were then blocked with Blotto. Incubation with the primary antibodies (1:200) was performed in 3% bovine serum albumin in TBST for 1 h at room temperature followed by three 10 min washes in TBST. Then the membrane was incubated with the secondary antibody (1:10,000) for 1 h at room temperature followed by three 10 min washes in TBST. The blot was developed using the SuperSignal Trial kit (Thermo). The heat shock proteins (HSPs) were used as reference to normalize the protein concentration in the samples. Gel-pro analyzer software was used to scan the signal intensity and calculated the average and standard deviation among three repeats of WB analysis.
3. Results
3.1. Rust resistance phenotypes
Wheat leaf rust resistance NILs TcLr35 and susceptible parent cultivar Thatcher were used for infectivity analysis after inoculation with P. triticina, and different degrees of infection (severity) were visualized on the surface of leaf blades on 10 dpi according to susceptible and resistant behavior of different plants at different growth periods. The mock inoculated plants did not show any infection whereas Thatcher started appearing infection courts within 4 dpi and scattered pustules surrounded by pale-halo region of chlorosis on the surface of leaf in whole wheat growth stage (, ). However, resistant NIL plants TcLr35 showed necrosis on leaf surface at the adult stage (), while it showed similar leaf rust symptoms to Thatcher at the seedling stage (). These results of infection development and resistance against pathogen at different wheat growth stage respectively warranted further investigation.
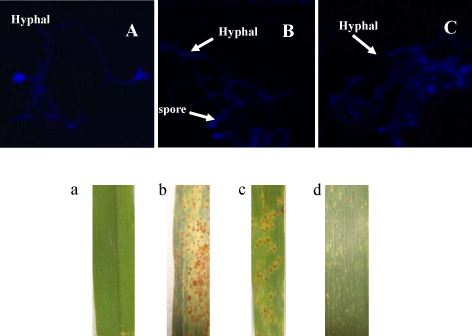
3.2. Cloning and characterization of TaLr35PR5
A wheat 516-bp homologue of thaumatin-like proteins was identified in wheat TcLr35 cDNA and gDNA and designated as TaLr35PR5 (GenBank accession number KJ764822, Supplementary Figure 1). The predicated opening reading frame (ORF) of TaLr35PR5 encoding 171 amino acid protein with a molecular weight of approximately 17.3 kDa. Analysis of the protein domain features indicated that TaLr35PR5 contains thaumatin-like proteins conserved domains. Multisequence alignment showed that TaLr35PR5 is 90%, 92%, 92%, 81%, 70%, 66%, 59%, and 53% identical to Secale cereale (AAC83824.1), Hordeum vulgare Linn. (P32937.1), Triticum aestivum Linn.(P27357.1), Avena sativa (P50695.1), Brachypodium distachyon (XP_003571622.1), Oryza (ACA50508.1), Setaria italica (XP_004963267.1) and Pinus monticola (S3ADB97933.1). ProtScale software predict (Kyte and Doolittle Citation1982) TaLr35PR5 encoded hydrophobic protein, and Spidey forecast analysis showed that the gene do not contain introns. SignalP 4.1 predict (Nielsen et al. Citation1997) the TaLr35PR5 amino acid sequence contain a signal peptide between 1~20 amino acid residues (Supplementary Figure 2).
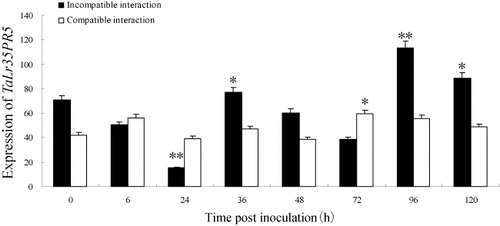
3.3. Pathogen-induced expression pattern of TaLr35PR5
To investigate the expression profiles of TaLr35PR5 in response to the infection by leaf rust pathogen, semi-quantitative RT-PCR was performed to analyze the accumulations of TaLr35PR5 at different treatments. The transcripts quantity of TaLr35PR5 in incompatible interactions (TcLr35) was detected at 0 hpi, then the transcript levels declined sharply to a relatively low level from 6 to 24 hpi, but increased at 36 hpi (about 1.1–fold more than 0 hpi). At 96 hpi, there was an expression peak that was 1.6-fold higher than 0 hpi. However, in compatible interaction (Thatcher), the transcripts of TaLr35PR5 maintained similar levels and changed little. Especially at 96 hpi, the accumulations of TaLr35PR5 transcripts in incompatible interaction was 2.0 times higher than that in compatible interaction ( Supplementary Figure 3). All in all, the expression levels of TaLr35PR5 were higher in incompatible interaction than compatible interaction at most time points.
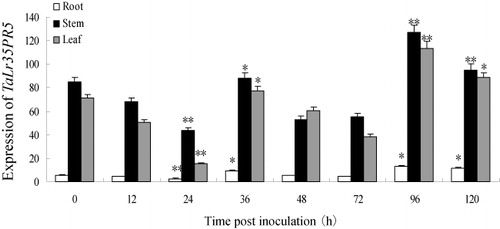
3.4. Tissue-specific expression of TaLr35PR5 transcripts
The roots, stems, and leaves of the mature TcLr35 wheat infected with leaf rust pathogen were used to detect the tissue-specific expression of TaLr35PR5. Gene expression pattern of TaLr35PR5 showed significant variation among different plant tissues and different time points after inoculation which indicated that the expression of TaLr35PR5 have tissue-specific and could be induced by leaf rust pathogen. More transcripts of TaLr35PR5 were accumulated in stems than that in leaves and roots from 0 to 120 hpi, however, the expression trend in different tissues is similar which increased or decreased at the same hpi and reached the peaks at 96 hpi in three different tissues ( Supplementary Figure 4).
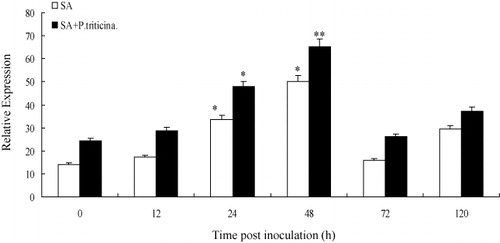
3.5. Responses of TaLr35PR5 gene in wheat induced by chemical reagents
The assessment of gene expression between treated and untreated samples of TcLr35 was investigated by semi-quantitative RT-PCR respectively. The transcripts of TaLr35PR5 treated with 50 μmol/L SA reached the peak at 48 hpi rapidly which was 1.7 times higher than 0 hpi, then declined. For those using 50 μmol/L SA treatment then inoculating with leaf rust pathogen, the expression trend was consistent with that by SA treatment and reached the peak at 48 hpi, and the accumulation of TaLr35PR5 transcripts was about 1.9 times higher than the control group (0 hpi). Moreover, the expression levels of TaLr35PR5 in wheat leaves pre-treated with SA chemical inducers prior to P. triticina inoculation were much higher than SA treatment ( Supplementary Figure 5). The TaLr35PR5 transcripts treated by ABA reached peak at 12 hpi rapidly (about 1.8 times higher than control), then declined. After 50μmol/L ABA stress treatment, and then inoculated with leaf rust pathogen, the expression level of TaLr35PR5 gene was significantly increased at 24hpi and reached the maximum which was about 2.0 times higher than that in the control group, then a slight downturn, but the expression level was higher than the overall treatment group without leaf rust pathogen (Supplementary Figure 6, ). In addition, there were obvious differences in expression time between ABA and SA infection reaction. The results showed that TaLr35PR5 gene could be induced by signaling molecules ABA and SA, and the gene expression levels were significantly increased especially pre-treatment with SA and ABA to infection with leaf rust pathogen.
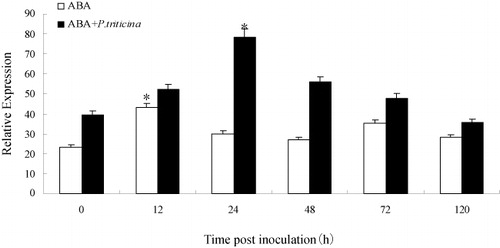
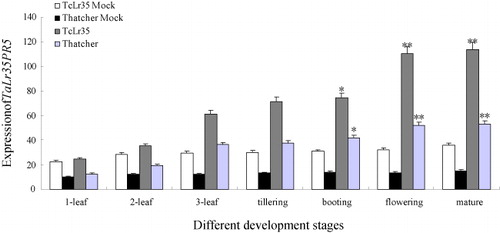
3.6. Accumulation of TaLr35PR5 is strongly increased at wheat different developmental stages upon leave rust pathogen infection
In order to define the relationship between TaLr35PR5 gene and wheat adult leaf rust disease resistance gene Lr35, we detected the expression levels of TaLr35PR5 at different growth periods based on the reported expression features of Lr35 gene (Seyfarth et al. Citation1999). As can be seen from , at different growth and development periods of wheat TcLr35 and Thatcher, TaLr35PR5 could be expressed at certain levels without inoculation (Mock) both in compatible and incompatible interaction, and the expression levels remained stable and showed the similar profiles at different periods, but the expression levels were much lower than the treatment with leaf rust pathogen. It showed that the expression level of TaLr35PR5 was much higher in incompatible interaction than compatible interaction, and then expressed stably at the mature stage. In short, the accumulations of TaLr35PR5 induced by leaf rust pathogen was significantly increased from the second-leaf stage and reached peak at the mature stage, and the expression levels at booting stage, flowering stage, and mature stage were 3.1 times, 3.9 times and 4.2 times higher than that at the second leaf stage, which is consistent with the expression profile of Lr35 gene (, Supplementary Figure 7).
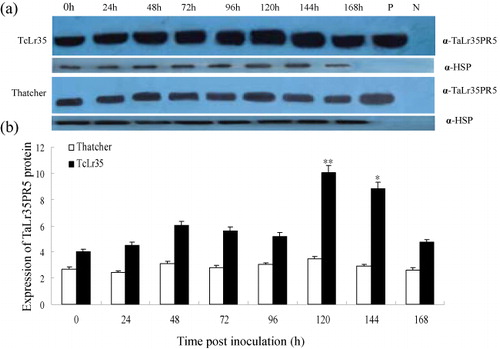
3.7. Expression profiles of TaLr35PR5 protein in wheat leaves infected by leaf rust
To detect the accumulation level of TaLr35PR5 protein in wheat leaves, polyclonal antibody was raised in rabbits against his-tagged TaLr35PR5 protein that was heterologously produced in E. coli. SDS-PAGE showed that the MW of the recombinant protein was about 28 kDa (Supplementary Figure 8), which is consistent with the deduced amino acids composition of TaLr35PR5 including the related sequence on pEASY plasmid. The ELISA result indicated that the antibody concentration of the antiserum reached 1: 768,000, which is sufficient for further experimentation. The generated antibody was then used to detect the accumulation level of TaLr35PR5 during incompatible (TcLr35) and compatible (Thatcher) interaction. As shown in ), a single or major band was detected in most of the WB results, which demonstrated the specificity of the antibodies and a 28 kDa signal band as expected was detected in TcLr35 and Thatcher infected by P. triticina from 0 hpi to 168 hpi. It was obvious that the signal intensity of TaLr35PR5 band in TcLr35 steadily increased from 0 to 120 hpi, then declined at 144 hpi. However, a much weaker signal was detected in Thatcher compared with TcLr35, in another words, the accumulation of TaLr35PR5 protein in TcLr35 was much higher than Thatcher (). These results strongly implied that accumulation of TaLr35PR5 protein was correlated with resistance against leaf rust pathogen infection.
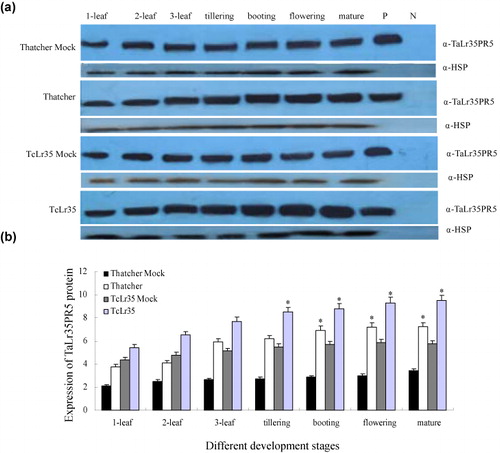
3.8. Expression of TaLr35PR5 protein in wheat leaves at different developmental stages
Western blotting was used to detect the expression of TaLr35PR5 in wheat leaves at different developmental stages. As shown in , the expression of TaLr35PR5 showed variable patterns in wheat leaves at different developmental stages. TaLr35PR5 could express at certain levels without inoculation (Mock) both in compatible (Thatcher) and incompatible (TcLr35) interaction and increased slowly from the second leaf stage to mature stage. Compared to Mock, TaLr35PR5 infected by P. triticina increased rapidly both in compatible and incompatible interaction. However, TaLr35PR5 expressed weakly at the first leaf stage and began to increase at the second leaf stage and contained constant levels at mature stage in TcLr35. The accumulation of TaLr35PR5 at the mature stage was 2.0 and 1.7 times higher than the first leaf stage and the second leaf stage. In addition, TaLr35PR5 increased more rapidly and its expression level was much higher in incompatible interaction than compatible interaction ().
4. Discussions
A number of reports have demonstrated that PR proteins play roles in the late periods of the resistance response (Datta and Muthukrishnan Citation1999). In Arabidopsis thaliana and tobacco, PR family number such as PR1, PR2, and PR5 have been proved to be involved in the SAR reaction. Datta et al. (Citation1999) found that over expressing of thaumatin protein gene in rice could enhance the resistance of transgenic rice to Thanatephorus cucul’neri (Frank) Donk; Chen et al. (Citation1999) translate thaumatin protein gene into wheat, transgenic plants have a certain resistance to Fusarium graminearum Schw; Jayasanka (Jayasankar et al. Citation2003) found that over expression of thaumatin protein gene could enhance the resistance of grape (Vitis vinifera Linn.) to Anthracnose. This study firstly detected that TaLr35PR5 was induced by leaf rust pathogen both at RNA and protein levels. It was interesting to find the expression profiles of TaLr35PR5 gene changes occurred in the incompatible and compatible reaction. The significant differences of TaLr35PR5 expression between mock inoculated, resistant infected and susceptible infected plants occurred can be attributed to the presence of adult leaf rust resistance Lr35 gene, which facilitated prevention of leaf rust infection in resistant wheat plants. This is the first example of study on the expression of TLPs gene in the interactions between TcLr35 wheat and P. triticina.
When plants face adverse natural environment, the accumulation of ABA can increase in plants and then promote the growth of plants. Plants have ABA and non-ABA two regulating system (Von Dahl and Baldwin Citation2007). In addition, plants in the process of long-term evolution formed two different signal transduction pathways including SA/JA (Jane Citation2001; Von Dahl and Baldwin Citation2007). In this study, we found that TaLr35PR5 gene was significantly induced by ABA and SA, especially the transcripts of TaLr35PR5 genes accumulated at higher levels inoculated with P. triticina following ABA or SA pre-treatment rather than only with ABA or SA treatment, but the expression trends of TaLr35PR5 were consistent in both inoculation and noninoculation treatment which are in accord with Zambounis et al. (Citation2012) suggest a correlation between increasing disease resistance and elevated PR transcript accumulation. So, we hypothesized that TaLr35PR5 may play a role in mediating plant responses to above chemical inducers and pathogen challenge in TcLr35 wheat and the expression of TaLr35PR5 might be regulated by SA and ABA signaling pathway.
Most of PR proteins expressed in normal leaf tissues, but with different patterns. Some of them expressed constitutively, while others expressed at low levels at the seeding stage and high levels at the adult stage (Hou et al. Citation2012). Different expression patterns might implicate different functions. These data added to our knowledge of PR proteins expressed in various tissues have illustrated that they are not only important components of the defense mechanism in wheat but also can sustain the function and regulate normal development. Studies have shown that the differences of different genes in different tissues and organs, such as hubei haitang MhPR8 gene expression in the root is the largest, followed by the stem, the leaf expression of minimum (Zhang et al. Citation2012); Zhang found that PR5 gene expressed differently in the different tissues and organs in strawberry (Zhang and Shih Citation2007). In our study, we found that TaLr35PR5 genes expressed in different tissues and organs of wheat including roots, stems, and leaf, but had obvious tissue specificity according to the significant differences in gene expression. The localization analysis of TLPs in poplar indicated that TLPs were present at the tissue level in the phloem, specifically in sieve elements, and were detected in cell walls and within cells associated with starch at the cellular level (Dafoe et al. Citation2010). So, we speculate that the expression in stem higher than leave and root may be associated with starch.
Moreover, In order to define the relationship between TaLr35PR5 genes and wheat leaf rust disease resistance gene Lr35, we detected the expression levels of TaLr35PR5 at different growth periods based on the reported expression features of Lr35 gene (Seyfarth et al. Citation1999). It was reported that Lr35 gene in wheat was not expressed at the first leaf stage, similar to that, a little accumulation of TaLr35PR5 was detected at the first leaf stage. The expression level of TaLr35PR5 began to increase in the different treatment since the second leaf stage which is consistent with that Lr35 gene begins express at certain degree since the second leaf stage. All in all, TaLr35PR5 gene expressed at lower levels at the seeding stage but higher levels at the adult stage, and the expression at the adult stage was stable. These results indicated that the expression profiles of TaLr35PR5 at different growth periods be accord with the expression features of Lr35 gene and showed that the gene might play an important role in wheat resistance during adult stage. In addition, there was a certain amount of background expression in different growth periods in TcLr35 wheat reflected from the expression profiles of TaLr35PR5 in noninoculated treatments including resistant and susceptible materials, which suggested that TaLr35PR5 play roles not only in plant disease resistance but also in the growth and development of wheat. On this basis, we should better to understand the expression profiles of TaLr35PR5 at protein level. Variation in expression level of TaLr35PR5 using western blot showed that the target protein was highly induced by leaf rust pathogen, and the induction peak time of TaLr35PR5 in the incompatible interaction occurred earlier and higher compared to the compatible one. Western blot showed that the expression trends of target protein were consistent with its expression at nucleic acid level. However, compared with the expression peak (at 96 hpi) at nucleotide level, the maximal expression of TaLr35PR5 protein (144 hpi) was delayed both in incompatible and compatible combinations. On the other hand, TaLr35PR5 transcript began to increase at 36 hpi, while TaLr35PR5 protein was detected to begin increasing at 48 hpi in incompatible interaction, which might because protein expressed after transcription and post-transcriptional modification.
In conclusion, TaLr35PR5 was initially cloned and identified the expression profiles infected by P. triticina which might take part in SA and ABA signaling pathways and be involved in the interactions between leaf rust pathogen and TcLr35 wheat. In order to get insight into the role of TaLr35PR5 in wheat growth and resistance to leaf rust pathogens attack, its function should be analyzed. In the future experiment, we have to obtain positive generation of the transgenic plant used to analysis the co-expression between TaLr35PR5 gene and Lr35 gene, and contributing to the defense against P. triticina infection.
Supplemental data
Supplemental data for this article can be accessed at http://dx.doi.org/10.1080/17429145.2015.1036140
Supplementary_material.doc
Download MS Word (1.8 MB)Disclosure statement
No potential conflict of interest was reported by the authors.
Additional information
Funding
References
- Ahmed NU, Park JJ, Jung HJ, Kang KK, Lim YP, Hur YK, Nou IS. 2013. Molecular characterization of thaumatin family genes related to stresses in Brassica rapa. Scientia Horticulturae. 152:26–34.10.1016/j.scienta.2013.01.007
- Borad V, Sriram S. 2008. Pathogenesis-related proteins for the plant protection. Asian J Exp Sci. 22:189–196.
- Chan YW, Tung WL, Griffith M, Chow KC. 1998. Cloning of a cDNA encoding the thaumatin-like proein of winter rye (Secale cereale L. Musketeer) and its functional characterization. J Exp Bot. 50:1627–1628.
- Chen WP, Chen PD, Liu DJ, Kynast R, Friebe B, Velazhahan R, Muthukrishnan S, Gill BS. 1999. Development of wheat scab symptoms is delayed in transgenic wheat plants that constitutively express a rice thaumatin-like protein gene. Theor Appl Genet. 99: 755–760.10.1007/s001220051294
- Dafoe NJ, Gowen BE, Constabel CP. 2010. Thaumatin-like proteins are differentially expressed and localized in phloem tissues of hybrid poplar. BMC Plant Biol. 10:191–201.10.1186/1471-2229-10-191
- Datta SK, Muthukrishnan S. 1999. Pathogenesis-related proteins in plants. Boca Raton (FL): CRC Press LLC.
- Datta K, Velazhahan R, Oliva N, Ona I, Mew T, Khush GS, Mew T, Khush GS, Muthukrishnan S, Datta SK. 1999. Over-expression of the cloned rice thaumatin-like protein (PR-5) gene in transgenic rice plants enhances environmental friendly resistance to Rhizoctonia solani causing sheath blight disease. Theor Appl Genet. 98: 1138–1145.10.1007/s001220051178
- Dharmendra S, Govindraj B, Rajib B, Kumble VP, Pushpendra KG, Kunal M. 2012. Targeted spatio-temporal expression based characterization of state of infection and time-point of maximum defense in wheat NILs during leaf rust infection. Mol Biol Rep. 39:9373–9382.10.1007/s11033-012-1801-y
- Eden L, Hesling L, Klock R, Ledeboer AM, Meat J, Toonen MY. 1982. Cloning of cDNA encoding the sweet tasting plant protein thaumatin and its expression in Escherichia coli. Gene. 18:1–12.10.1016/0378-1119(82)90050-6
- El-kereamy A, El-Sharkawy I, Ramamoorthy R, Taheri A, Errampalli D, Kumar P, et al. 2011. Prunus domestica pathogenesis-pelated protein-5 activates the defense response pathway and enhances the resistance to fungal infection. PLoS One. 6:17973.
- Gabriela R, Felix M, Robert D. 1991. Sequence of a wheat cDNA encoding a pathogen-induced thaumatin-like protein. Plant Mol Biol. 17:283–285.10.1007/BF00039506
- Hamamouch N, Li CY, Seo PJ, Park CM, Davis EL. 2011. Expression of Arabidopsis pathogenesis-related genes during nematode infection. Mol Plant Pathol. 12: 355–364.10.1111/j.1364-3703.2010.00675.x
- Hammond-Kosack KE, Jones JD. 1996. Resistance gene-dependent plant defense response. Plant Cell. 8:1773–1791.10.1105/tpc.8.10.1773
- Hou MM, Li WJ, Bai H, Liu YM, Li LY, Liu LJ, Liu B, Liu G. 2012. Characteristic expression of rice pathogenesis-related proteins in rice leaves during interactions with Xanthomonas oryzae pv. Oryzae. Plant Cell Rep. 31:895–904.10.1007/s00299-011-1210-z
- Jan S, Janick M, Barbara MA, De C, Bruno PA, Cammue. 2008. Plant pathogenesis-related (PR) proteins: A focus on PR peptides. Plant Physiol Bioch. 46:941–950.10.1016/j.plaphy.2008.06.011
- Jane G. 2001. Genes controlling expression of defense responses in Arabidopsis-2001 status. Curr Opin Plant Biol. 4:301–308.
- Jayasankar S, Li Z, Gray DJ. 2003. Constitutive expression of Vitis vinifera thaumatin-like protein after in vitro selection and its role in anthracnose resistance. Funct Plant Biol. 30:1105–1115.10.1071/FP03066
- Kerber ER, Dyck PL. 1990. Transfer to hexaploid wheat of linked genes for adult-plant leaf rust and seeding stem rust resistance from an amphiploid of Aegilops speltoidesx Triticum monococcum. Genome. 33:530–537.10.1139/g90-079
- Kolmer JA. 2001. Physiologic specialization of Puccinia triticina in Canada in 1998. Plant Dis. 85:155–158.
- Kyte J, Doolittle RF. 1982. A simple method for displaying the hydropathic character of a protein. J Mol Biol. 157: 105–132.10.1016/0022-2836(82)90515-0
- Lei Y, Zhang ZY, Liu WF, Wang X, An XM, Lin SZ, et al. 2012. Cloning and expression of three pathogenesis related protein genes from Populus tomeutosa. J Northeast Agricultural University. 40:67–75.
- Liu DQ, He X, Li WX, Chen CY, Ge F. 2012. Molecular cloning of a thaumatin-like protein gene from Pyrus pyrifoliaand overexpression of this gene in tobacco increased resistance to pathogenic fungi. Plant Cell Tiss Org10.1007/s11240-012-0167-0.
- Liu JQ, Kolmer JA. 1998. Genetics of stem rust resistance in wheat cus Pasqua and AC Taber. Phytopathology. 88:171–176.
- Liu L, Zhang ZM, Zhao MJ, Wang J, Lin HJ, Shen YO, et al. 2011. Molecular cloning and characterization of pathogenesis-related protein 5 in Zea mays and its antifungal activity against Rhizoctonia solani. Afr J Biotechnol. 10:19286–19293.
- Mackintosh CA, Lewis J, Radmer LE, Shin S, Heinen SJ, Smith LA. et al. 2007. Overexpression of defense response genes in transgenic wheat enhances resistance to Fusarium head blight. Plant Cell Rep. 26:479–488.10.1007/s00299-006-0265-8
- Nielsen H, Engelbrecht J, Brunak S, von Heijne G. 1997. Identification of prokaryotic and eukaryotic signal peptides and prediction of their cleavage sites. Protein Eng. 10:1–6.10.1093/protein/10.1.1
- Rinaldi C, Kohler A, Frey P, Duchaussoy F, Ningre N, Couloux A, Wincker P, Le Thiec D, Fluch S, Martin F, Duplessis S. 2007. Transcript profiling of poplar leaves upon infection with compatible and incompatible strains of the foliar rust melampsora larici-populina. Plant Physiol. 144:347–366.10.1104/pp.106.094987
- Roelfs AP. 1984. Race specificity and methods of study. In: Bushnell WR, Roelfs AP, editors. The Cereal rusts: origins, specificity, structure and physiology. Orlando: Academic; p. 131–164.
- Saghai-Maroof MA, Soliman KM, Jorgesen RA, Allard RW. 1984. Ribosomal DNA spacer-length polymorphisms in barley Mende-lian inheritance, chromosomal location and population dynamics. Proc Natl Acad Sci USA. 81:8014–8018.10.1073/pnas.81.24.8014
- Sambrook J, Fritsch EF, Maniatis T. 1998. Molecular cloning: a laboratory manual. 2nd edn. New York: Cold Spring Harbor Laboratory Press, Cold Spring Harbor.
- Seyfarth R, Feuillet C, Schachermayr G, Winzeler M, Keller B. 1999. Development of a molecular marker for the adult plant leaf rust resistance gene Lr35 in wheat. Theor Appl Genet. 99:554–560.
- Singh R, Datta D, Priyamvada, Singh S, Tiwari R. 2004. Marker-assisted selection for leaf rust resistance genes Lr19 and Lr24 in wheat (Triticum aestivum L.). J Appl Genet. 45: 399–403.
- Von Dahl CC, Baldwin IT. 2007. Deciphering the role of ethylene in plant herbivore interactions. J Plant Growth Regul. 26:201–209.10.1007/s00344-007-0014-4
- Wang L, Yang L, Zhang JX, Dong J, Yu J, Zhou J, et al. 2013. Cloning and characterization of a thaumatin-like protein gene PeTLPin Populus deltoides3P. euramericanacv. ‘Nanlin895’. Acta Physiol Plant. 35:2985–2998.
- Zambounis AG, Kalamaki MS, Tani EE, Paplomatas EJ, Tsaftaris AS. 2012. Expression analysis of defense-related genes in cotton (Gossypium hirsutum) after Fusarium oxysporum f. sp. vasinfectum infection and following chemical elicitation using a salicylic acid analog and methyl jasmonate. Plant Mol Biol Rep. 30:225–234.
- Zhang YH, Shih DS. 2007. Isolation of an osmotin-like protein gene from strawberry and analysis of the response of this gene to abiotic stresses. J Plant Physiol. 164:68–77.10.1016/j.jplph.2006.02.002
- Zhang JY, Qu SC, Xue HB, Gao ZH, Guo ZR, Zhang Z. 2012. Isolation and expression of pathogenesis-related protein gene MhPR8 from Malus hupehensis. Scientia Agricultura Sinica. 45:1568–1575.