Abstract
Juglone (5-hydroxy-1,4-naphthoquinone) is an important allelochemical in walnut trees (Juglans nigra L.). Its allelopathic potential has been reported in different plant species. We investigated the phytotoxic effects of the allelochemical juglone and the protective role of proline in tobacco seedlings. Juglone inhibited the growth of tobacco seedlings and increased reactive oxygen species content in tobacco roots. Moreover, juglone stress increased proline concentration. The expression of two proline synthesis genes, pyrroline-5-carboxylate synthetase and ornithine aminotransferase, was upregulated and that of a proline catabolism gene, proline dehydrogenase, was downregulated with juglone treatment in tobacco roots. Furthermore, plants pretreated with proline and then exposed to juglone showed attenuated toxic effects in roots. Proline was able to modulate allelochemical juglone-induced stress in tobacco. In summary, this study suggested that increased proline content in the tobacco seedlings treated with juglone may mitigate the deleterious effect of allelochemical stress in plants by inhibiting reactive oxygen species accumulation.
Introduction
Plant growth in the field is often affected by a number of environmental stresses. Allelochemical toxicity is one of the important factors limiting crop production. Allelopathy is defined as the effect of one plant species on another through the release of chemical components into the environment (Rice Citation1979). Plants introduce allelochemicals into the environment through foliar leaching, root exudation, residue decomposition, volatilization, and debris incorporation into soil (Inderjit & Keating Citation1999). The action of allelochemicals in target plant is diverse and affects a large number of biochemical reactions resulting in modifications of different physiological functions (Inderjit & Duke Citation2003). It has long been understood that plant allelochemical compounds belong to natural xenobiotics (Laue et al. Citation2014). In mammals, the signaling mechanisms associated with the response to xenobiotic compounds have been extensively studied. An array of xenobiotic receptors is involved in different aspects of xenobiotic responses (Ghose et al. Citation2011). However, in plant systems, allelochemical-sensing system seems to be difficult to characterize.
Hoy and Stickney (Citation1881) reported a deleterious effect by black walnut on the growth of plants nearby. Juglone is a quinone and an allelopathic growth regulator released by walnut (Juglans nigra L.) (Rice Citation1984). The toxic effects of juglone have been studied in maize (Hejl & Koster Citation2004), soybean (Bohm et al. Citation2006), and Arabidopsis (Reigosa & Pazos-Malvido Citation2007). One of the most important mechanisms underlying the toxic effect of juglone is involved in the pro-oxidation action within tissues of targeted plants (El Hadrami et al. Citation2005; Murakami et al. Citation2010). In order to survive when exposed to juglone, plants must defend themselves. Edwards et al. (Citation2011) reported that plant responses to various xenobiotics shows the induction of similar classes of genes involved in metabolization and detoxification, conjugation, transport, antioxidant defense and cell protection, and repair. Recently, Mylona et al. (Citation2007) demonstrated that juglone can induce enzymatic activities of antioxidant enzymes in maize. In our previous study, the microarray assay of the mechanism of action of juglone revealed the involvement of detoxification enzymes and reactive oxygen species (ROS) scavengers in protection against juglone toxicity (Chi et al. Citation2011).
In response to different environmental stresses plants accumulate compatible solutes (Serraj & Sinclair Citation2002). These different types of compatible solutes, such as proline, sucrose, and glycine betaine, protection to plants from stress. Proline accumulation occurs in plants under environmental stresses such as salinity, low temperature, and heavy-metal exposure and is considered involved in the stress defense mechanism. Proline is synthesized from glutamic acid by Δ1-pyrroline-5-carboxylate synthetase (P5CS) (Delauney & Verma Citation1993; Yoshiba et al. Citation1997). Plants also synthesize proline from ornithine by ornithine-δ-aminotransferase (OAT, EC 2.6.1.13). However, the content of proline also depends on its degradation, which is catalyzed by the enzyme proline dehydrogenase (PDH, EC 11.5.99.8) (Yoshiba et al. Citation1997). Salt and water stress increase proline accumulation along with increased P5CS level (Hu et al. Citation1992; Savouré et al. Citation1995; Verbruggen et al. Citation1996; Yoshiba et al. Citation1997), and salt stress downregulated PDH accumulation (Kiyosue et al. Citation1996; Peng et al. Citation1996; Verbruggen et al. Citation1996).
Tobacco plants have been used as one of the most common models for investigating defense pathways in response to environmental stress (Nakasugi et al. Citation2013). Ability of juglone as a model allelochemical to induce cellular damage and its connection with oxidative stress in tobacco BY-2 cells has been reported (Babula et al. Citation2009). Here, we used juglone to study its effect on tobacco plant growth, ROS generation, proline content, and proline metabolic gene expression to better understand the mode of action of allelochemical juglone-induced toxicity and defense responses in tobacco.
Material and methods
Growth bioassay
Nicotiana benthamiana plants were grown in a growth chamber at 27°C with cool white fluorescent light (100 μmol s−1 m−2 light intensity) under long-day conditions (16-h white light/8-h dark). Five-day-old tobacco seedlings were transferred to Murashige and Skoog (MS) medium supplemented with different concentrations of juglone (0, 5, 10, 25, 50 μM). Primary roots were measured by manual recording on the plate. Data were obtained from three biological replicates.
Detection of ROS levels in tobacco roots
The increased level of cellular ROS was most likely an early response to environmental stresses. In most studies, ROS production is observed in minutes or even hours (Wong & Shimamoto Citation2009). To determine whether juglone treatment induced ROS production in tobacco roots, we labeled roots with the ROS-sensitive dye 5-(and-6)-chlormethyl-2′, 7′-dichlordihydrofluorescein diacetate, acetyl ester (CM-H2DCF-DA, Molecular Probes). Untreated tobacco seedlings were preincubated with 10 μM CM-H2DCFDA for 30 min, then with concentrations of juglone (0, 5, 10, 25, 50 μM) for 15 min. A Leica MPS60 fluorescent microscope equipped with a green-fluorescent-protein filter (excitation 450–490 nm, emission 500–530 nm) was used to detect fluorescence. Images were captured with use of a CoolSNAP Cooled CCD Camera (CoolSNAP 5.0, North Reading, MA, USA). All experiments were repeated at least three times.
Determination of proline
Five-day old tobacco seedlings were transferred to Murashige and Skoog medium supplemented with or without 10 μM juglone. Accumulation of proline was determined after 5 days. The free proline concentration was determined according to the method of Bates et al. (Citation1973). Approximately one gram of tobacco shoots or roots were frozen by immersion in liquid nitrogen and ground using the TissueLyser LT (QIAGEN, Hilden, Germany). The free proline was extracted from plant materials by homogenizing shoot and root tissues in 3% sulfosalicylic acid followed by centrifugation at 5000 g for 20 min at 25°C. To 1 ml of the extract was added 1 ml of the reagent mixture (consisting of 6 ml glacial acetic acid, 4 ml phosphoric acid water and 0.25 g ninhydrin). The samples were boiled for 1 h, cooled and extracted with 4 ml toluene. The absorbance of the toluene phase was determined at 520 nm and proline concentration was calculated from a standard curve and expressed as µmol g−1 fresh weigh. Three replicates were performed for each experiment.
Semi-quantitative RT-PCR
Five-day-old tobacco seedlings were transferred to MS medium with concentrations of juglone (0, 5, 10, 25, 50 μM) for 24 h before RNA extraction. Total RNA was isolated from root and shoot tissues by use of the RNeasy Plant Mini kit (QIAGEN, Hilden, Germany) and with DNase by use of the RNase-Free DNase Set (QIAGEN, Hilden, Germany). Total RNA was reversed transcribed into cDNA by use of the ImProm-II Reverse Transcription System with a mix of oligo (dT)18 and (dT)20 primers according to the manufacturer's manual (Promega). The sequence-specific primer pairs and recommended annealing temperatures (Ta) corresponding to each gene are in Table A.1 (Supplemental Table). cDNA was added to the PCR mixture containing 1 U Taq DNA polymerase (Promega), 25 mM MgCl2, 10 mM dNTPs and 1 μM of each primer pair. Amplicons were analyzed by agarose gel electrophoresis (1%), and PCR products were sequenced. Experiments were repeated at least twice and reproducibility was confirmed. The accession numbers of tobacco (N. benthamiana) P5CS, OAT, PDH, and EF1-α genes were JF903807, JF903808, AY639145, and AY206004, respectively.
Statistical analysis
Data are presented as mean ± SE of at least three separate experiments. The normal distribution of data was analyzed by Kolmogorov-Smirnov test and the homogeneity of error variance by the F-test. Comparisons involved paired t-test. p < 0.05 was considered statistically significant.
Results
ROS production in tobacco roots under juglone stress
Toxicity of juglone to tobacco roots was evaluated by dose dependent analysis. Compared with control treatment, root growth was significantly reduced with juglone treatment, beginning with 10 μM juglone inhibited root growth (). To determine whether juglone treatment induced ROS production, tobacco roots of 3-day-old seedlings were pretreated with ROS-sensitive dye (CM-H2DCFDA), then juglone for 15 min. CM-H2DCFDA is nonfluorescent but is oxidized to the highly fluorescent CM-H2DCFDA by intracellular ROS. The levels of CM-H2DCFDA fluorescence was significantly increased with juglone between 10 and 50 μM in tobacco roots ( and Supplemental Figure 1).
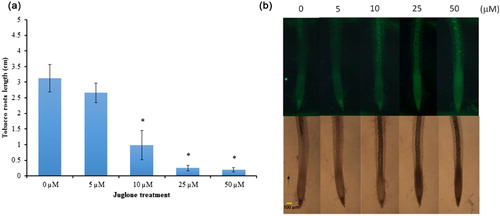
Effect of juglone on proline accumulation and proline metabolism gene expression
Proline content in tobacco seedlings exposed to juglone (10 μM) increased significantly by almost twofold that of control levels in roots, and slightly increased about 1.2-fold in shoots (). To determine whether the expression of proline metabolism genes was regulated by juglone, we examined the content of proline synthesis genes P5CS and OAT and the proline catabolism gene PDH in tobacco roots and shoots treated with juglone for 24 h. The mRNA level of P5CS and OAT was increased with increasing dose of juglone, whereas that of PDH was decreased ().
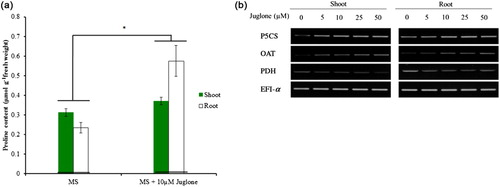
Effect of exogenous application of proline on juglone stress-induced changes in tobacco roots
To elucidate the possible relation between the accumulation of proline and resistance to juglone, we tested the role of proline in juglone-inhibited root growth. Tobacco roots were pretreated with or without proline, and root growth was assayed after exposure to juglone stress. Treatment with juglone inhibited root growth (). However, pretreatment with proline and then exposure to juglone improved the root growth.
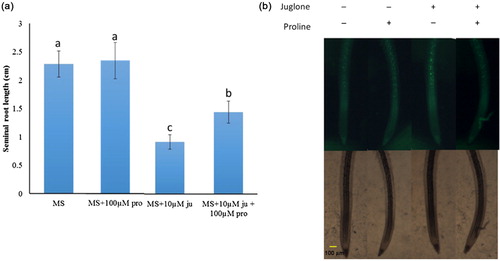
In order to examine the influence of proline on juglone-induced ROS accumulation, tobacco roots were preincubated for 3 h with 100 μM proline and subsequently exposed to 10 μM juglone for another 15 min. As shown in and Supplemental Figure 1, proline pretreatment mitigated juglone-induced ROS accumulation.
Discussion
It is well known that allelochemical toxicity can inhibit plant growth. Allelopathy stress typically results from a combination of allelochemicals which interfere with several biochemical reaction and physiological processes in the receiving plant (Gniazdowska & Bogatek Citation2005). Increased ROS levels are an important component of environmental stress signaling (Apel & Hirt Citation2004). Like other stress factors, the effect of allelopathy stress on target plant may be uncontrolled production and accumulation of ROS (Bais et al. Citation2003). Juglone was found an ROS-generating xenobiotic in plants (Mylona et al. Citation2007). ROS play a vital role in the plant defense against stresses. Low ROS content, as a signal, can lead to repair of cellular damage, but high levels can lead to programmed cell death (Neill et al. Citation2002; Gao et al. Citation2008). We found that juglone rapidly induced ROS production. ROS production may contribute to juglone-inhibited root growth.
Stress-induced proline accumulation has been studied in plants such as rice, Arabidopsis, and tobacco. Accumulation of proline could be due to de novo synthesis or decreased degradation or both. It is clear from many studies that proline accumulation in plants exposed to environmental stress was found associated with increased expression of P5CS and OAT and decreased expression of PDH. Here, we found that proline concentration increased the mRNA expression of P5CS and OAT and decreased that of PDH with juglone treatment, indicated that juglone participate in the regulation of proline synthesis and degradation in tobacco roots. Yang et al. (Citation2009) found that exogenous ROS (H2O2) treatment lead to a rapid accumulation of proline and regulate gene expression of P5CS. Here, we had observed that juglone treatment induced ROS production. Taken together, our results may link the ROS accumulation with perception of juglone stress and activation of a signaling pathway leading to alteration of proline metabolism-related gene expression in plants.
Proline is known to protect plants against environmental stresses such as drought and salinity. The functional role of proline in different environmental stresses has been explored by overexpressing or suppressing a number of synthesis and catabolism pathway genes. Several works reported that overproduction of proline in plants led to increased tolerance against osmotic stress (Kishor et al. Citation1995; Molinari et al. Citation2007) and knockout seedlings of Arabidopsis mutant AtP5CS1 and rice mutant OsP5CS2 were sensitive to salt stress (Hur et al. Citation2004; Székely et al. Citation2008). Thus, proline is thought to contribute to osmotic adjustment. Recently, proline was proposed to quench singlet oxygen (Alia et al. Citation2001). Chen and Dickman (Citation2005) demonstrated that proline can be an intracellular ROS scavenger. Duran-Servantes et al. (Citation2002) reported that three allelochemicals, 2-benzoxazolinone (BOA), p-hydroxybenzoic, and ferulic acid, induced accumulation of proline. Oxidative stress induction has been found to be the mode of action of numerous allelochemicals (Weir et al. Citation2004; Cruz-Ortega et al. Citation2007). However, the role of proline in aspects of antioxidant defense has not been reported during allelochemical stress in plants. In this study, proline pretreatment improved root growth under juglone stress in tobacco seeding and mitigated juglone-induced ROS accumulation (). It is tempting to speculate that proline may be an antioxidant compound, involved in the molecular physiology of allelochemical stress protection.
Therefore, increased proline content in the tobacco seedlings treated with juglone may mitigate the deleterious effect of allelochemical stress in plants by inhibiting ROS accumulation. The present work extends current knowledge of early transcriptional regulation by juglone stress in tobacco roots and provides valuable insights into aspects of juglone detoxification and acquired tolerance.
Supplemental data
Supplemental data for this article can be accessed at http://dx.doi.org/10.1080/17429145.2015.1045946
Supplementary_table.docx
Download MS Word (15.4 KB)Supplementary_figure.pdf
Download PDF (68 KB)Disclosure statement
No potential conflict of interest was reported by the authors.
Additional information
Funding
References
- Alia Mohanty P, Matysik J. 2001. Effect of proline on the production of singlet oxygen. Amino Acids. 21:195–200. 10.1007/s007260170026
- Apel K, Hirt H. 2004. Reactive oxygen species: metabolism, oxidative stress, and signal transduction. Annu Rev Plant Biol. 55:373–399.
- Babula P, Adam V, Kizekb R, Sladký Z, Havel L. 2009. Naphthoquinones as allelochemical triggers of programmed cell death. Environ Exp Bot. 65:330–337.
- Bais HP, Vepachedu R, Gilroy S, Callaway RM, Vivanco JM. 2003. Allelopathy and exotic plant invasion: from molecules and genes to species interactions. Science. 301:1377–1380. 10.1126/science.1083245
- Bates LS, Waldren RP, Teare ID. 1973. Rapid determination of free proline for water stress studies. Plant Soil. 39: 205–207. 10.1007/BF00018060
- Bohm PAF, Zanardo FML, Ferrarese MLL, Ferrarese-Filho O. 2006. Peroxidase activity and lignification in soybean root growth-inhibition by juglone. Biol Plant. 50:315–317.
- Chen C, Dickman MB. 2005. Proline suppresses apoptosis in the fungal pathogen Colletotrichum trifolii. Proc Natl Acad Sci USA. 102:3459–3464.
- Chi WC, Fu SF, Huang TL, Chen YA, Chen CC, Huang HJ. 2011. Identification of transcriptome profiles and signaling pathways for the allelochemical juglone in rice roots. Plant Mol Biol. 77:591–607. 10.1007/s11103-011-9841-6
- Cruz-Ortega R, Lara-Núñez A, Anaya AL. 2007. Allelochemical stress can trigger oxidative damage in receptor plants mode of action of phytotoxicity. Plant Signal Behav. 2:269–270.
- Delauney AJ, Verma DPS. 1993. Proline biosynthesis and osmoregulation in plants. Plant J. 4:215–223.
- Duran-Servantes B, Gonzalez L, Reigosa MJ. 2002. Comparative physiological effects of three allelochemicals and two herbicides on Dactylis glomerata. Acta Physiol Plantarum. 24:385–392. 10.1007/s11738-002-0034-4
- Edwards R, Dixon DP, Cummins I, Brazier-Hicks M, Skipsey M. 2011. New perspectives on the metabolism and detoxification of synthetic compounds in plants. In: Schro¨ der P, Collins CD, editor. Organic xenobiotics and plants. Berlin: Springer; p. 125–148.
- El Hadrami A, Kone D, Lepoivre P. 2005. Effect of juglone on active oxygen species and antioxidant enzymes in susceptible and partially resistant banana cultivars to black leaf streak disease. Eur J Plant Pathol. 113:241–254.
- Gao S, Yan R., Cao M, Yang W, Wang S, Chen F. 2008. Effects of copper on growth, antioxidant enzymes and phenylalanine ammonialyase activities in Jatropha curcas L. seedling. Plant Soil Environ. 54:117–122.
- Ghose R, Guo T, Vallejo JG, Gandhi A. 2011. Differential role of Toll-interleukin 1 receptor domain-containing adaptor protein in Toll-like receptor 2-mediated regulation of gene expression of hepatic cytokines and drug-metabolizing enzymes. Drug Metab Dispos. 39:874–881.
- Gniazdowska A, Bogatek R. 2005. Allelopathic interaction between plants: multi site action of allelochemicals. Acta Physiol Plant. 27:395–407.
- Hejl AM, Koster KL. 2004. Juglone disrupts root plasma membrane H+-ATPase activity and impairs water uptake, root respiration, and growth in soybean (Glycine max) and corn (Zea mays). J Chem Ecol. 30:453–471.
- Hoy PR, Stickney JS. 1881. Toxic action of black walnut. Trans Wisc State Horti Soc. 11:166–167.
- Hu CA, Delauney AJ, Verma DP. 1992. A bifunctional enzyme (delta 1-pyrroline-5-carboxylate synthetase) catalyzes the first two steps in proline biosynthesis in plants. Proc Natl Acad Sci USA. 89:9354–9358. 10.1073/pnas.89.19.9354
- Hur J, Jung KH, Lee CH, An G. 2004. Stress-inducible OsP5CS2 gene is essential for salt and cold tolerance in rice. Plant Sci. 167:417–426.
- Inderjit Duke SO. 2003. Ecophysiological aspects of allelopathy. Planta. 217:529–539.
- Inderjit Keating KI. 1999. Allelopathy: principles, procedures, processes, and promises for biological control. Adv Agron. 67:141–231.
- Kishor P, Hong Z, Miao GH, Hu C, Verma D. 1995. Overexpression of [delta]-pyrroline-5-carboxylate synthetase increases proline production and confers osmotolerance in transgenic plants. Plant Physiol. 108:1387–1394.
- Kiyosue T, Yoshiba Y, Yamaguchi-Shinozaki K, Shinozaki K. 1996. A nuclear gene encoding mitochondrial proline dehydrogenase, an enzyme involved in proline metabolism, is upregulated by proline but downregulated by dehydration in Arabidopsis. Plant Cell. 8:1323–1335.
- Laue P, Bährs H, Chakrabarti S, Steinberg CE. 2014. Natural xenobiotics to prevent cyanobacterial and algal growth in freshwater: contrasting efficacy of tannic acid, gallic acid, and gramine. Chemosphere. 104:212–220.
- Molinari HBC, Marur CJ, Daros E, de Campos MKF, de Carvalho JFRP, Bespalhok JC, Pereira LFP, Vieira LGE. 2007. Evaluation of the stressinducible production of proline in transgenic sugarcane (Saccharum spp.): osmotic adjustment, chlorophyll fluorescence and oxidative stress. Physiol Plant. 130:218–229. 10.1111/j.1399-3054.2007.00909.x
- Murakami K, Haneda M, Iwata S, Yoshino M. 2010. Effect of hydroxy substituent on the prooxidant action of naphthoquinone compounds. Toxicol In Vitro. 24:905–909. 10.1016/j.tiv.2009.11.018
- Mylona PV, Polidoros AN, Scandalios JG. 2007. Antioxidant gene responses to ROS-generating xenobiotics in developing and germinated scutella of maize. J Exp Bot. 58:1301–1312.
- Nakasugi K, Crowhurst RN, Bally J, Wood CC, Hellens RP, Waterhouse PM. 2013. De novo transcriptome sequence assembly and analysis of RNA silencing genes of Nicotiana benthamiana. PLoS One. 8:e59534.
- Neill SJ, Desikan R, Clarke A, Hurst RD, Hancock JT. 2002. Hydrogen peroxide and nitric oxide as signalling molecules in plants. J Exp Bot. 53:1237–1247.
- Peng Z, Lu Q, Verma DP. 1996. Reciprocal regulation of delta 1-pyrroline-5-carboxylate synthetase and proline dehydrogenase genes controls proline levels during and after osmotic stress in plants. Mol Gen Genet. 253:334–341.
- Reigosa MJ, Pazos-Malvido E. 2007. Phytotoxic effects of 21 plant secondary metabolites on Arabidopsis thaliana germination and root growth. J Chem Ecol. 33:1456–1466.
- Rice EL. 1979. Allelopathy: an update. Bot Rev. 45:15–109. 10.1007/BF02869951
- Rice EL. 1984. Allelopathy. 2nd ed. Orlando (FL): Academic Press.
- Savouré A, Jaoua S, Hua XJ, Ardiles W, Van Montagu M, Verbruggen N. 1995. Isolation, characterization, and chromosomal location of a gene encoding the delta 1-pyrroline-5-carboxylate synthetase in Arabidopsis thaliana. FEBS Lett. 372:13–19.
- Serraj R, Sinclair TR. 2002. Osmolyte accumulation: can it really help increase crop yield under drought conditions? Plant Cell Environ. 25:333–341.
- Székely G, Abrahám E, Cséplo A, Rigó G, Zsigmond L, Csiszár J, Ayaydin F, Strizhov N, Jásik J, Schmelzer E, et al. 2008. Duplicated P5CS genes of Arabidopsis play distinct roles in stress regulation and developmental control of proline biosynthesis. Plant J. 53:11–28.
- Verbruggen N, Hua XJ, May M, Van Montagu M. 1996. Environmental and developmental signals modulate proline homeostasis: evidence for a negative transcriptional regulator. Proc Natl Acad Sci USA. 193:8787–8791.
- Weir TL, Park SW, Vivanco JM. 2004. Biochemical and physiological mechanisms mediated by allelochemicals. Curr Opin Plant Biol. 7:472–479.
- Wong HL, Shimamoto K. 2009. Sending ROS on a bullet train. Sci Signal. 2:e60.
- Yang SL, Lan SS, Gong M. 2009. Hydrogen peroxide-induced proline and metabolic pathway of its accumulation in maize seedlings. J Plant Physiol. 166:1694–1699.
- Yoshiba Y, Kiyosue T, Nakashima K, Yamaguchi-Shinozaki K, Shinozaki K. 1997. Regulation of levels of proline as an osmolyte in plants under water stress. Plant Cell Physiol. 38:1095–1102.