Abstract
Potassium is actively involved in many functions such as enzyme activation, osmotic adjustment and uptake of deleterious ions like Na. Present report analyses the effectivity of different potassium salts on growth and certain components of nitrogen metabolism and antioxidant system in oat and their possible role in amelioration of water stress. Potassium induced enhancement in the activities of nitrate reductase and aminotransferases was evident indicating a positive role of potassium in nitrogen metabolism. Potassium supplementation enhanced activities of antioxidant enzymes (superoxide dismutase, catalase and ascorbate peroxidase) and contents of total phenols and tannins, probably strengthening both the enzymatic as well as non enzymatic antioxidant system. Free amino acids, proline and free sugars also exhibited the same trend in treated plants ensuring better plant growth.
Introduction
Inadequate soil moisture and unbalanced supply of mineral nutrients result in impaired soil fertility and decreased global food production especially in arid and semi-arid regions. Water stress is one of major environmental constraints limiting crop productivity the world over (see Agarwal et al. Citation1999; Araus et al. Citation2002). About 25% of the world's agricultural land is affected by water stress (Jajarmi Citation2009). Changes in global climate have worsened the situation even more (Cominelli & Tonelli Citation2010). Water stress affects yield by depressing both sink and source, depending on the timing and the severity of stress with respect to plant phenology. Plants respond differently to water deficiency during different growth stages. The generative phase and the beginning of flowering are most frequently the periods of the greatest sensitivity to water deficit (Blum Citation1996; Istanbulluoglu et al. Citation2009).
It is estimated that around 60% of cultivated soils have growth limiting problems associated with mineral nutrient deficiencies and toxicities (Cakmak Citation2002). Increasing evidence suggest that mineral nutrient status of plants plays a critical role in increasing plant resistance to environmental stress factors and is important in exploiting the genetic potential of crop plants.
Potassium (K) is the third important macronutrient (i.e. after nitrogen and phosphorus) required for plant growth and is involved in many biochemical and physiological processes such as osmoregulation, stomatal movement, activation of enzymes, protein synthesis, photosynthesis, phloem loading and transport and in reducing excess uptake of ions such as Na and Fe in saline soils (Wang et al. Citation2013; Zorb et al. Citation2014).
Greater concentrations of potassium are found in young developing tissues and reproductive organs indicating its requirements in cell metabolism and growth. Potassium fertilizers are not subjected to leaching or volatilization and therefore can be applied to a wide range of environmental conditions by different methods of application. Availability of potassium to plants is highly variable and is mostly affected by root soil interaction (Ashley et al. Citation2006). In long distance transport, potassium is the dominant cation within the xylem and phloem sap neutralizing inorganic and organic anions, conferring high potassium mobility throughout the entire plant (Jeschke et al. Citation1997). Uptake and accumulation of potassium by plant cells is the primary driving force for their osmotic expansion.
Reactive oxygen species (ROS) affect normal metabolic functioning of plants because of effects on lipids, proteins as well as nucleic acids (Ahmad et al. Citation2010). Several defence mechanisms help plants to avert ROS induced alterations. Enzymatic and non enzymatic antioxidants present within the plant system mediate scavenging of ROS thereby preventing their accumulation and protecting plant cells from deleterious effects.
Oat (Avena sativa L) is grown throughout the temperate zone as rabi crop and has a low summer heat requirement and greater tolerance of rain than other cereals, such as wheat, rye or barley, and therefore are particularly important in areas with cool, wet summers. Oat is an annual plant and can be planted either in autumn (for late summer harvest) or in the spring (for early autumn harvest). Its consumption is believed to lower low density lipoprotein (bad cholesterol) and it contains a wide range of functional ingredients having antioxidant property which are mostly concentrated in different parts of the kernel (Gray et al. Citation2000, Peterson Citation2001). Oat grain is a good source of quality protein, unsaturated fatty acids, minerals and vitamins, phenolic compounds as well as dietary fiber especially β-glucans (Drzikova et al. Citation2005; Sun et al. Citation2006). Present experiments evaluate the growth, biochemical parameters particularly associated with the antioxidant and nitrogen metabolism of A. sativa L. cultivar KENT as altered by treatment of different potassium salts.
Material and methods
Treatments and cultivation conditions
Seeds of A. sativa L. (oat) cultivar ‘KENT’ were obtained from Indian Grassland and Fodder Research Institute, Jhansi UP. Healthy seeds were screened for sowing and both laboratory and pot experiments were conducted as outlined below:
Laboratory experiments: Healthy seeds were selected and used for germination and growth under different treatments. Seeds were surface sterilized using 0.01% mercuric chloride and washed with distilled water. Each Petri plate was lined with Whatman's filter paper number 1 in two layers and ten seeds were placed in each Petri plate. Three salts of potassium, that is, K2O, KCl and KNO3 were used in varying concentrations [0.1, 0.01 and 0.001 M (w/v)] and Polyethylene glycol (PEG-6000) was employed to induce water stress and water potential of solution was maintained at −2.7 bars following Michel et al. (Citation1983). Potassium salts were also used in treatments subjected to polyethylene glycol-6000 – induced water stress to comprehend possible role of potassium salts in ameliorating PEG-6000 induced water stress, if any. Petriplates were kept on culture racks under constant light and dark periods of 10/14 h. Seedlings were put to analysis seven days after sowing (7 DAS).
Pot experiments: Separate bottom perforated pots were maintained for different treatments in the botanical garden of School of Studies in Botany, Jiwaji University, Gwalior MP, India under natural conditions with day and night temperatures of 25/8 ± 4°C and relative humidity of 85/55 ± 10%. Each of the pots used was 24ʺ × 12ʺ × 10ʺ in dimensions and were filled with well ploughed soil having the characteristics given in . Nitrogen (in the form of urea) and phosphorous (single super phosphate) fertilizers were applied as per the recommended requirement, that is, 120 and 30 kg ha−1 respectively. Two potassium salts K2O and KNO3 were applied at the rate of 0, 20, 40 and 60 kg ha−1. Replicates receiving only nitrogen and phosphorous but no potassium (0 kg ha−1) served as control. Equal number of seeds were sown at the depth of 3–4 cm. Growth of plants was monitored regularly. After 55 days leaves, roots and stem were separated for measurement of shoot/root length and fresh and dry weight.
Table 1. Characteristics of soil used for present study.
Relative water content: Relative water content (RWC) of leaf was measured in fully expanded leaves following Weatherley's (Citation1950) method. Twenty discs (0.5 cm in diameter) were excised from the leaves (excluding mid vein) by cork borer and their fresh weight (FW) was determined. These discs were floated on distilled water in petri dishes/cavity discs for an hour to gain turgidity and thereafter removed and soaked on filter paper and then reweighed (TW). The samples were dried at 60°C for 24 h and then their dry weight (DW) was determined. RWC was calculated using the following formula:
Estimation of Chlorophylls and Carotenoids: Chlorophyll contents in leaves were determined following Arnon's method (Citation1949). After extraction with 80% acetone final volume was made up to 5 ml with 80% acetone (v/v) and optical density was recorded at 480, 645 and 663 nm.
Lipid peroxidation: Lipid peroxidation was determined by measuring content of malondialdehyde (MDA) formation. For calculating MDA content an extinction coefficient (ε) of 155 mM−1 cm−1 was used (Heath & Packer Citation1968).
Proline: Free proline was estimated following Bates et al. (Citation1973). Dry plant material was extracted in sulphosalicylic acid [3% (w/v)] followed by centrifugation at 3000 g for 10 min. Two milliliter supernatant was mixed with glacial acetic acid and acid ninhydrin followed by incubation for 1 h at 100°C. The reaction was terminated in ice. Free proline was separated using toluene in a separating funnel and optical density was recorded at 520 nm.
Free amino acids: Free amino acids were estimated following the method outlined in Sadasivam and Manickam (Citation2004). Powdered plant sample was extracted in 80% ethanol (v/v) and centrifuged at 2000 g for 20 min. Hundred microliter supernatant was made up to 2 ml with distilled water and to this 1 ml ninhydrin [prepared in 0.2 M citrate buffer (pH 5.0) and 2-methoxyethanol] was added and after incubating for 30 min 5 ml diluent was added. After 20 min optical density was recorded at 570 nm. Computation was done using standards.
Free sugars: Total free sugars were worked out using anthrone method. One hundred milligram powdered sample was extracted in ethanol (80%) followed by centrifugation at 5000 g for 10 min. About 0.5 ml supernatant was taken and volume was made upto 1 ml followed by addition of 1 ml HCl (1N) and kept in water bath at boiling temperature. Thereafter, 4 ml anthrone (0.2%) was added and again kept in water bath for 10 min and optical density was recorded at 620 nm (Fong et al. Citation1953; Jain & Guruprasad Citation1989).
Phenols: 0.5 gm powdered plant material was extracted in 80% ethanol and centrifuged at 10,000 g for 20 min. The supernatant was collected and residue was re-extracted. The supernatant was evaporated to dryness and dissolved in 5 ml distilled water. Aliquot (0.1 ml) was taken and made up to 2 ml with distilled water. One normality Folin-Ciocalteu's reagent was added and incubated for 3 min. Twenty percent Na2CO3 (2 ml) was added and kept in boiling water bath for 1 min and subsequently cooled and read at 650 nm (Malick & Singh Citation1980).
Tannins: Dry powdered plant material was extracted in distilled water and heated for 30 min in boiling water bath followed by centrifugation at 2000 g for 20 min. The supernatant was collected and final volume was made up to 10 ml with distilled water. Hundred μL extract was taken and made up to 2 ml with distilled water followed by addition of Folin Denis reagent (1 ml) and 35% (w/v) Na2CO3 (2 ml) and thereafter kept in dark for 45 min at room temperature. Optical density was recorded at 700 nm and computation was done using standards and expressed as mg g−1 dry weight tannic acid (Swain & Hills Citation1959).
Nitrate reductase assay: Nitrate reductase (EC 1.6.6.1) assay was done following Srivastava (Citation1974) as adopted by Sharma and Agarwal (Citation2002). Three hundred microgram fresh plant sample was cut into small pieces in 5 ml tubes filled with incubation medium [0.1 M phosphate buffer maintained at pH 7.5 containing 200 mM KNO3 and 0.5% n-propanol] kept in dark for 3 h at 30°C. Thereafter, 1 ml aliquot was taken and 1ml sulfanilamide (prepared in 1 N HCL) and 1 ml 0.2% 1-naphthyl ethylene diamine dihydrochloride were added to it followed by thorough mixing and left for 25 min to develop color. Optical density was recorded at 540 nm.
Assay of Aminotransferases: Aspartate (EC 2.6.1.1) and alanine aminotransferase (EC 2.6.1.2) activity was determined following Reitman and Frankel's (Citation1957) method. One hundred milligram sample was homogenized in 1.5 ml chilled sodium potassium buffer (pH 7). After centrifugation for 15 min at 4000 g at 4°C the supernatant was used as enzyme source. 50 µl supernatant was added to 0.1 ml substrate buffer (100 µL phosphate buffer containing 2-oxoglutarate (2 mM) and 100 mM L-aspartate or 200 mM DL-alanine) followed by thorough mixing and incubation at 37°C in a water bath (incubation time for aspartate is 60 min and for alanine 30 min). Thereafter, 0.1 ml DNPH (2, 4-dinitrophenyl hydrazine prepared in 1 M HCl) was added and after 20 min l ml sodium hydroxide (0.4 M) was added and mixed. After 5 min optical density was recorded at 540 nm.
For superoxide dismutase (SOD) and catalase (CAT) fresh plant sample (0.1 gm) was homogenized in chilled potassium phosphate buffer (pH 7.5) containing 0.1% PVP and 0.5 mM EDTA followed by centrifugation at 12,000 g for 15 min and the supernatant was used as enzyme source. However, for ascorbate peroxidase (APX) crushing buffer contained 2 mM ascorbate. Protein content was estimated as per Lowry et al. (Citation1951).
Superoxide Dismutase: SOD (EC 1.15.1.1) activity was determined following the method of Dhindsa et al. (Citation1981). The assay mixture consisting of 1.5 ml potassium phosphate buffer (pH 7.8), 0.2 ml methionine, 0.1 ml enzyme extract with equal volume of 1 M Na2CO3, 2.25 mM nitroblue tetrazolium, 3 mM EDTA, riboflavin and 1 ml double distilled water was incubated under 15 W fluorescent lamp for 15 min. Blank A containing the same reaction mixture was placed in the dark. Blank B containing the same reaction mixture except the enzyme extract was placed in light along with the sample. The reaction was terminated by switching off the light and the tubes were covered with a black cloth. Absorbance of each sample along with blank B was read against blank A at 560 nm and the difference in percent color reduction between blank B and the sample was calculated. Fifty percent color reduction was considered as one unit of enzyme activity and activity was expressed as EU mg−1 protein.
Catalase: The activity of CAT (EC 1.11.1.6) was assayed in accordance with Aebi (Citation1984). Assay mixture contained 50 mM potassium phosphate buffer (pH 7), 100 mM H2O2 and 100 µL enzyme extract in a final volume of 2 ml. Change in optical density at 240 nm was recorded for 2 min. Activity was calculated using extinction coefficient (ε) 0.036 mM−1 cm−1 and was expressed as enzyme unit mg−1 protein.
Ascorbate peroxidase: APX (EC 1.11.1.1) was spectrophotometrically assayed following Nakano and Asada (Citation1981). 3 ml assay mixture contained 50 mM potassium phosphate buffer (pH 6), 0.1 mM EDTA, 0.5 mM ascorbate and 100 µL H2O2 and 100 µL enzyme extract. H2O2 dependent oxidation of ascorbate was followed by change in the absorbance at 290 nm. APX activity was calculated using extinction coefficient (ε) 2.8 mM−1 cm−1 and expressed as enzyme unit mg−1 protein.
Estimation of K, Na and Ca
Dry plant samples were digested in triacid (H2SO4 + HNO3 + HClO4 in 9:3:1 ratio) and the volume of white colorless digested material was made up to 100 ml with distilled water and was subsequently filtered. Filtrate was read directly on digital flame photometer using K, Na and Ca filters separately (Tomar & Agarwal Citation2013; Jatav et al. Citation2014).
Statistical analysis
Data presented are mean of four replicates with standard error (±SE) calculated. All the data were statistically analyzed by analysis of variance with the MSTAT C PROGRAM (Mich. University, East Lasing Mich. USA). Least significant difference (LSD) was calculated for the significant data at p < .05. Data followed by same letter are not significantly different by LSD test at p < .05.
Results and discussion
Increase in shoot/root length and fresh/dry weight was observed as a result of potassium application excepting for treatments using 0.1 M concentration of potassium salts in which a decline was observed, may be because of osmotic stress (). Singh et al. (Citation2000) and Jatav et al. (Citation2012) have also found better growth and yield of wheat crop with the addition of potassium. Increase in root and shoot length as a result of application of potassium has been earlier reported in Triticum aestivum L. by Shirazi et al. (Citation2005) also. KNO3 induced increase in fresh and dry weight has earlier been reported in sunflower (Helianthus annuus L.) and safflower (Carthamus tinctorius L.) by Jabeen and Ahmad (Citation2011) as well.
Table 2. Shoot/root length and fresh/dry weight of oat (A. sativa L. cultivar KENT) seedlings raised under different potassium treatments (7 DAS).
Water stress induced using polyethylene glycol (PEG 6000) caused reduction in shoot/root length and fresh / dry weight, however, this effect was ameliorated by application of potassium to some extent (). PEG-induced reduction in seedling growth has been reported in Zea mays L (Farsiani & Ghobadi Citation2009) and Triticum aestivum L (Jatav et al. Citation2012). This reduction in plant height is attributed to a decline in the cell enlargement and greater leaf senescence in the plants subjected to water stress (Manivannan et al. Citation2007b). Added potassium has been reported to alleviate the negative effects of water stress in Oryza sativa L (Tiwari et al. Citation1998), sorghum, mustard and groundnut (Umar Citation2006) and Triticum aestivum L (Jatav et al. Citation2012) as well.
Table 3. Shoot/root length and fresh/dry weight of oat (A. sativa L. cultivar KENT) seedlings raised under different potassium treatments and PEG-induced water stress (7 DAS).
Moreover, increase in number of tillers per pot as a result of potassium supplementation was obvious at the later stage of development with slight increase in RWC () indicating positive role of potassium in hydration and thereby in the maintainence of turgor and subsequently growth as well. Other reports of improved water use efficiency as a result of potassium application are also available such as in O. sativa L (Tiwari et al. Citation1998), Cicer arietinum L (Ali et al. Citation2005), sorghum, mustard and groundnut (Umar Citation2006), Brassica napus L (Fanaei et al. Citation2009) and Triticum aestivum L (Jatav et al. Citation2012).
Table 4. Plant height, Number of tillers and RWC in A. sativa L. cultivar KENT supplied with different salts of potassium (55 DAS).
An increase in Chlorophyll and carotenoid contents was also found as a result of potassium treatments excepting for higher concentration, that is, 0.1 M. Reduction in chlorophyll and carotenoid contents resulted due to polyethylene glycol 6000 induced water stress was overcome substantially as indicated in the treatments in which potassium salts were applied alongwith PEG-6000 (–). Loss of chlorophyll contents under water stress is considered to be the main cause of inactivation of photosynthesis. Water deficit induced reduction in chlorophyll contents is attributed to the loss of chloroplast membranes, excessive swelling, distortion of the lamellae vesiculation and the appearance of lipid droplets (Kaiser et al. Citation1981). Exposure to environmental stresses results in inhibition of chlorophyll biosynthesis (Khan Citation2003) as well as instability of pigment protein complex.
Table 5. Chlorophyll and Carotenoid (mg g−1 fresh wt) contents in oat (A. sativa L. cultivar KENT) seedlings raised under different potassium treatments (7 DAS).
Table 6. Chlorophyll and Carotenoid (mg g−1 fresh wt.) contents in oat (A. sativa L. cultivar KENT) seedlings raised under different potassium treatments and PEG-induced water stress (7 DAS).
Table 7. Chlorophyll a, b, Total Chlorophylls and Carotenoid (mg g−1 fr wt.) contents in flag leaf of A. sativa L. cultivar KENT supplied with different salts of potassium (55 DAS).
Nitrate reductase activity in both shoot and root showed an increase in seedlings raised with potassium supplementation and increase in activity was greater where potassium was used as potassium nitrate ((a) and ). Polyethylene glycol-6000 induced stress reduced nitrate reductase activity in oat seedlings, and the negative effect of stress was ameliorated to some extent by application of potassium ((b)). Reduction due to polyethylene glycol-6000 induced water stress in rice nitrate reductase activity has been reported by Pandey and Agarwal (Citation1998). An increase in nitrate reductase activity with the application of potassium has earlier been reported in Cicer arietinum L (Sharma & Agarwal Citation2002; Ali et al. Citation2005), Lepidium sativum L (Dhawan et al. Citation2011), sunflower and safflower (Jabeen & Ahmad Citation2011) as well.
Figure 1. Nitrate reductase activity (µ mole NO2 produced hr−1 g−1 fr wt) in oat (A. sativa L. cultivar KENT) seedlings raised under different potassium treatments (A) and PEG (B) induced water stress (7 DAS).
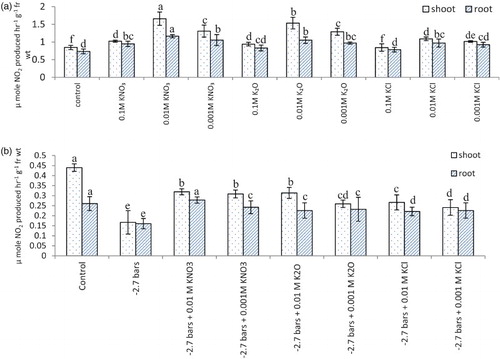
Figure 2. Nitrate reductase activity (µ mole NO2 produced hr−1 g−1 fr wt) in flag leaf of A. sativa L. cultivar KENT supplied with different salts of potassium (55 DAS).
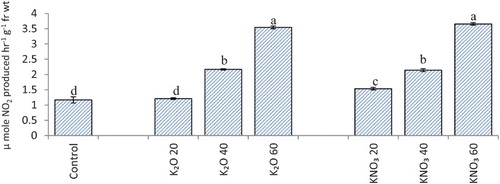
Potassium supplementation caused considerable increase in alanine and aspartate aminotransferase activity in the shoot as well as root ((a) and 3(b)). PEG-6000 induced water stress has resulted in reduction of alanine and aspartate aminotransferase activity. Treatment of potassium has however, alleviated this effect to some extent ((a) and 4(b)). Similar trend was obvious for aminotransferases under field conditions ((a) and 5(B)). Osmotic and water stress induced reduction in aspartate and alanine aminotransferase activity alongwith altered amino acid contents has been reported in rice (Pandey et al. Citation2004). Aminotransferases serve as a link between carbohydrate and amino acid metabolism. Increased activity of aminotransferases leads to greater synthesis of certain amino acids which may play some protective role under stress conditions (Rao & Ramamoorthy Citation1981). Besides, having key role in primary nitrogen assimilation, aminotransferases also help in transfer of reducing equivalents and bringing about interchanges of carbon and nitrogen pools within subcellular compartments (Torre et al. Citation2007).
Figure 3. Alanine (A) and Aspartate (B) aminotransferase activity (µ mol keto acid released mg−1 protein min−1) in oat (A. sativa L. cultivar KENT) seedlings raised under different potassium treatments (7 DAS).
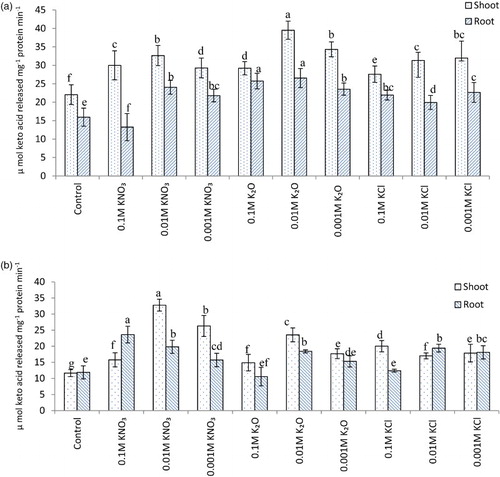
Figure 4. Alanine (A) and Aspartate (B) aminotransferase activity (µ mol keto acid released mg−1 protein min−1) in oat (A. sativa L. cultivar KENT) seedlings raised under different potassium treatments and PEG-induced water stress (7 DAS).
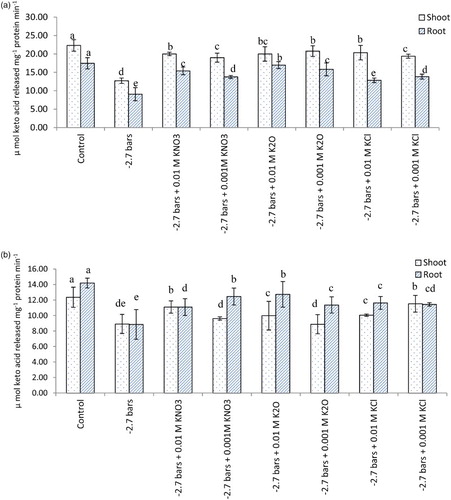
Figure 5. Alanine (A) and Aspartate Aminotransferase (B) (µ mol keto acid released mg−1 protein min−1) in flag leaf of A. sativa L. cultivar KENT supplied with different salts of potassium (55 DAS).
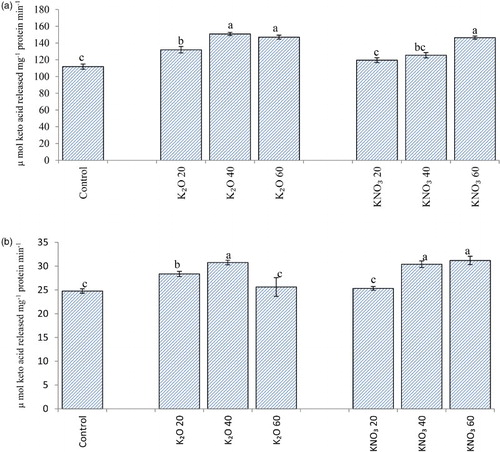
Total phenols and tannins exhibited an increase due to potassium application in all the parts, that is, flag leaf, stem and root of oat plants (). Potassium induced enhancement in phenolic content has earlier been reported in Ocimum basilicum L (Nguyen et al. Citation2010), Labisia pumila Benth (Ibrahim et al. Citation2012) and Triticum aestivum L (Tomar & Agarwal Citation2013).
Table 8. Phenols and Tannins (mg g−1 dry wt.) contents in A. sativa L. cultivar KENT supplied with different salts of potassium (55 DAS).
An increase in activities of SOD, CAT and APX ((a)–6(c)) was also found in plants receiving potassium supplementation which further indicates role of potassium in strengthening plant antioxidant potential. ROS are produced as a normal product of plant cellular metabolism and their overproduction on exposure to various stresses leads to oxidative damage and ultimately cell death. ROS accumulate in cells and lead to the oxidation of proteins, chlorophylls, lipids, nucleic acids and carbohydrates. Alleviation of oxidative damage and resistance of plants to changing environment is often correlated to efficient antioxidative system (Manivannan et al. Citation2007a). Activities of antioxidant enzymes as a result of potassium application have been reported to increase in O. sativa L. (Yu-Chuan et al. Citation2008) and Panax ginseng (Devi et al. Citation2012) also.
Figure 6. Superoxide dismutase (A), Catalase (B) and Ascorbate peroxidase (C) (EU mg−1 protein) activity in flag leaf of A. sativa L. cultivar KENT supplied with different salts of potassium (55 DAS).
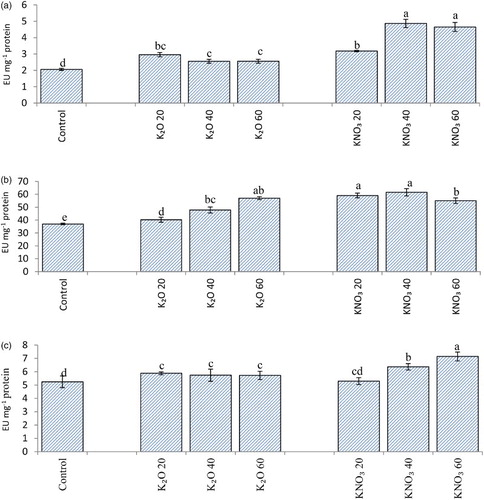
Osmotic constituents like free amino acids, free proline and free sugars also increased in plants receiving potassium supplementation which may improve their water/salinity stress tolerance by possible enhancement of osmotic adjustment (). Accumulation of compatible osmotic constituents is one of the important and basic adaptation strategies for better growth and adaptation under environmental stresses (Chen et al. Citation2007). Proline is one the important organic osmolytes utilized in osmotic adjustment leading to maintenance of turgor/osmotic balance, through better extraction of water from the soil (Kusaka et al. Citation2005; Gonzalez et al. Citation2010). Proline acts as metal chelator, antioxidative defense molecule bringing concentrations of ROS within normal ranges, thus preventing oxidative burst (Hayat et al. Citation2012).
Table 9. Free amino acids (mg g−1 dry wt.) Proline (µ mole proline g−1 dry wt.) and Free sugars (mg g−1 dry wt.) contents in A. sativa L. cultivar KENT supplied with different salts of potassium (55 DAS).
Malonaldehyde content, a product of membrane lipid peroxidation, decreased in plants supplied with potassium salts possibly indicating stability to membranes and hence reducing leakage (). Lipid peroxidation, the oxidative deterioration of unsaturated lipids, is an indicator of oxidative stress in cells and tissues. Lipid peroxides are unstable and decompose to form deleterious compounds including reactive carbonyl compounds. Decrease in MDA content as a result of potassium supplementation have been reported in O. sativa L. (Yu-Chuan et al. Citation2008) and Hordeum vulgare L. (Hafsi et al. Citation2010). Various kinds of antioxidants with different functions inhibit lipid peroxidation and the deleterious effects caused by the lipid peroxidation products (El-Beltagi & Mohamed Citation2013). Present experiments provide evidence that potassium treated plants are equipped with improved antioxidant system that includes increased antioxidant contents and enhancement in the activity of SOD, CAT and APX.
Table 10. Malondialdehyde content (µ mol g−1 fr wt.) in flag leaf of A. sativa L. cultivar KENT supplied with different salts of potassium (55 DAS).
Increased uptake of potassium and calcium was obvious in all potassium treatments and in addition potassium supplementation reduced the uptake and accumulation of sodium ions (). Sodium shows antagonistic behavior towards potassium. Higher potassium content observed in leaf indicates efficient uptake of potassium and selectivity of plants towards potassium uptake in comparison to sodium. Increased uptake of potassium has direct effect on the growth and productivity of crop plants (Tomar & Agarwal Citation2013; Jatav et al. Citation2014). Enhanced calcium and reduced sodium uptake due to potassium supplementation has been earlier reported in Cicer arietinum L. (Sharma et al. Citation2006) and wheat (Tomar & Agarwal Citation2013; Jatav et al. Citation2014).
Table 11. Sodium, potassium and Calcium (mg g−1 dry wt.) contents in A. sativa L. cultivar KENT supplied with different salts of potassium (55 DAS).
Increased nitrate reductase and aminotransferase activity and altered free proline and amino acid contents in potassium treated plants/seedlings in our experiments alongwith improved antioxidant system indicate positive role of potassium in the growth of oat under normal and stress conditions.
Conclusion
Potassium supplementation improved the growth of oat (A. sativa L.) which was evident from the morphological and physiological parameters studied. Increase in contents of phenols and tannins as well as the activities of SOD, CAT and APX in potassium treated plants ensure role of potassium in enhancing and strengthening the antioxidant potential. Increase in synthesis and accumulation of compatible osmolytes like free proline, free sugars and free amino acids and decrease in lipid peroxidation justifies the importance of potassium to crop plants even under stress conditions.
Acknowledgement
Thanks are due to Prof. Rekha Bhadhauria, the then Head, School of Studies in Botany for providing necessary laboratory facilities.
Disclosure statement
No potential conflict of interest was reported by the authors.
References
- Aebi H. 1984. Catalase in vitro. Meth Enzymol. 105:121–126.
- Agarwal RM, Pandey R, Gupta S. 1999. Certain aspects of water stress induced changes and tolerance mechanisms in plants. J Indian Bot Soc. 78:255–269.
- Ahmad P, Jaleel CA, Sharma S. 2010. Antioxidant defense system, lipid peroxidation, proline metabolizing enzymes and biochemical activities in two Morus alba genotypes subjected to NaCl stress. Russian J Plant Physiol. 57(4):509–517.
- Ali B, Hayat S, Ahmad A. 2005. Response of germinating seeds of Cicer arietinum to 28-hormobrassnolide and/or potassium. Gen Appl Plant Physiol. 31(1–2):55–63.
- Araus JI, Slafer GA, Reynolds MP, Toyo C. 2002. Plant breeding and drought in C3 cereals, what should we breed for? Annals Bot. 89:925–940.
- Arnon DI. 1949. Copper enzymes in isolated chloroplasts. polyphenoloxidase in Beta vulgaris. Plant Physiol. 24:1–15.
- Ashley MK, Grant M, Grabov A. 2006. Plant responses to potassium deficiencies. a role for potassium transport proteins. J Exp Bot. 57:425–436.
- Bates LS, Waldren RP, Teare ID. 1973. Rapid determination of free proline for water stress studied. Plant Soil. 39:205–207.
- Blum A. 1996. Crop response to drought and the interpretation of adaptation. J Plant Growth Reg. 20:135–148.
- Cakmak I. 2002. Plant nutrition research. Priorities to meet human needs for food in sustainable ways. Plant Soil. 247:3–24.
- Chen Z, Cuin TA, Zhou M, Twomey A, Naidu BP, Shabala S. 2007. Compatible solute accumulation and stress-mitigating effects in barley genotypes contrasting in their salt tolerance. J Exp Bot. 58(15–16):4245–4255.
- Cominelli E, Tonelli T. 2010. Transgenic crops coping with water scarcity. New Biotech. 27(5):473–477.
- Devi BSR, Kim YJ, Selvi SK, Gayathri S, Altanzul K, Parvin S, Yang DU, Lee OR, Lee S, Yang DC. 2012. Influence of potassium nitrate on antioxidant level and secondary metabolite genes under cold tress in Panax ginseng. Russian J Plant Physiol. 59(3):318–325.
- Dhawan NG, Umar S, Tariq SO, Iqbal M. 2011. Nitrogen assimilation and yield of Lipidium sativum L. as affected by potassium availability. J Funct Environ Bot. 1(1):1–10.
- Dhindsa RH, Plumb-Dhindsa R, Thorpe TA. 1981. Leaf senescence correlated with increased level of membrane permeability, lipid peroxidation & decreased level of SOD and CAT. J Exp Bot. 32:93–101.
- Drzikova B, Dongowski G, Gebhardt E, Habel A. 2005. The composition of dietary fibre-rich extrudates from oat affects bile acid binding & fermentation in vitro. Food Chem. 90(1–2):181–192.
- El-Beltagi HS, Mohamed HI. 2013. Reactive oxygen species, lipid peroxidation and antioxidative defense mechanism. Not Bot Hort Agrobot. 41(1):44–57.
- Fanaei HR, Galavi M, Kafi M, Ghanbari A, Bonjar G. 2009. Amelioration of water stress by potassium fertilizer in two oilseed species. Int J Plant Prod. 3(2):41–54.
- Farsiani A, Ghobadi ME. 2009. Effects of PEG and NaCl stress on two cultivars of corn (Zea mays L.) at germination and early seedling stages. World Aca Sci Eng Tech. 3(9): 362–365.
- Fong J, Schaffer FL, Kirk PL. 1953. The ultramicrodetermination of glycogen in liver. A comparison of the anthrone and reducing-sugar methods. Arch Biochem Biophy. 45(2):319–326.
- Gonzalez A, Bermejo V, Gimeno BS. 2010. Effect of different physiological traits on grain yield in barley grown under irrigated and terminal water deficit conditions. J Agri Sci. 148:319–328.
- Gray DA, Auerbach RH, Hill S, Wang R, Campbell GM, Webb C, South JB. 2000. Enrichment of oat antioxidant activity by dry milling and sieving. J Cereal Sci. 32:89–98.
- Hafsi C, Maria C, Romero-Puertas Luis A, del Rio Luisa M, Sandalio M, Abdelly C. 2010. Differential antioxidative response in barley leaves subjected to the interactive effects of salinity & potassium deprivation. Plant Soil. 334:449–460.
- Hayat S, Hayat Q, Alyemeni MN, Wani AS, Pichtel J, Ahmad A. 2012. Role of proline under changing environments. A review. Plant Sig Beh. 7(11):1456–1466.
- Heath RL, Packer L. 1968. Photoperoxidation in isolated chloroplasts. I. Kinetics and stoichiometry of fatty acid peroxidation. Arch Biochem Biophysic. 125(1):189–198.
- Ibrahim MH, Jaafar HZE, Karimi E, Ghasemzadeh A. 2012. Primary, secondary metabolites, photosynthetic capacity and antioxidant activity of the Malaysian herb Kacip Fatimah (Labisia pumila Benth) exposed to potassium fertilization under greenhouse conditions. Int J Mol Sci. 13:15321–15342.
- Istanbulluoglu A, Gocmen E, Gezer E, Pasa C, Konukcu F. 2009. Effects of water stress at different development stages on yield and water productivity of winter and summer safflower (Carthamus tinctorius L.). Agri Water Manag. 96:1429–1434.
- Jabeen N, Ahmad R. 2011. Foliar application of potassium nitrate affects the growth and nitrate reductase activity in sunflower and safflower leaves under salinity. Not Bot Hort Agro. 39(2):172–178.
- Jain VK, Guruprasad KN. 1989. Effect of chlorocholin chloride and gibberellic acid on the anthocyanin synthesis in radish seedlings. Plant Physiol. 75:233–236.
- Jajarmi V. 2009. Effect of water stress on germination indices in seven wheat cultivar. World Aca Sci Eng Tech. 49:105–106.
- Jatav KS, Agarwal RM, Singh RP, Shrivastava M. 2012. Growth and yield responses of wheat (Triticum aestivum L) to suboptimal water supply and different potassium doses. J Funct Environ Bot. 2(1):39–51.
- Jatav KS, Agarwal RM, Tomar NS, Tyagi SR. 2014. Nitrogen metabolism, growth and yield responses of wheat (Triticum aestivum L.) to restricted water supply and varying potassium treatments. J Indian Bot Soc. 93(3&4):177–189.
- Jeschke WD, Kirkby EA, Peuke AD, Pate JS, Hartung W. 1997. Effects of P deficiency on assimilation and transport of nitrate and phosphate in intact plants of castor oil bean (Ricinus communis L). J Exp Bot. 48:75–91.
- Kaiser WM, Kaiser G, Schoner S, Neimanis S. 1981. Photosynthesis under osmotic stress. Differential recovery of photosynthetic activities of stroma enzymes, intact chloroplasts and leaf slices after exposure to high solute concentrations. Planta. 153(5):430–435.
- Khan NA. 2003. NaCl inhibits chlorophyll synthesis and associated changes in ethylene evolution and antioxidative enzyme activities in wheat. Bio Plant. 47:437–440.
- Kusaka M, Antonio GL, Tatsuhito F. 2005. The maintenance of growth and turgor in pearl millet Pennisetum glaucum L. Leeke cultivars with different root structures and osmo-regulation under drought stress. Plant Sci. 168:1–14.
- Lowry O, Rosebrough A, Far A, Randall R. 1951. Protein measurement with folin phenol reagent. J Biol Chem. 193:680–685.
- Malick CP, Singh MB. 1980. Plant enzymology and histoenzymology. New Delhi: Kalyani Publishers, p. 286.
- Manivannan P, Jaleel CA, Sankar B, Kishorekumar A, Somasundaram R, Alagu Lakshmanan GM, Panneerselvam R. 2007a. Changes in antioxidant metabolism of Vigna unguiculata L. Walp. by propiconazole under water deficit stress. Coll Surf B Bioint. 57:69–74.
- Manivannan P, Jaleel CA, Sankar B, Kishorekumar A, Somasundaram R, Alagu Lakshmanan GM, Panneerselvam R. 2007b. Growth, biochemical modifications and proline metabolism in Helianthus annuus L. as induced by drought stress. Coll Surf B Bioint. 59:141–149.
- Michel BE, Wiggins OK, Outlaw Jr WH. 1983. A guide to establishing water potential of aqueous two phase solutions (polyethylene glycol plus dextran) by amendment with mannitol. Plant Physiol. 72:60–65.
- Nakano Y, Asada K. 1981. Hydrogen peroxide is scavenged by ascorbate specific peroxidase in spinach chloroplast. Plant Cell Physiol. 22:867–880.
- Nguyen PM, Kwee EM, Niemeyer ED. 2010. Potassium rate alters the antioxidant capacity and phenolic concentration of basil (Ocimum basilicum L.) leaves. Food Chem. 123:1235–1241.
- Pandey R, Agarwal RM. 1998. Water stress-induced changes in proline contents and nitrate reductase activity in rice under light and dark conditions. Physiol Mol Bio Plants. 4:53–57.
- Pandey R, Agarwal RM, Jeevaratnam K, Sharma GL. 2004. Osmotic stress-induced alterations in rice (Oryza sativa L.) and recovery on stress release. Plant Growth Reg. 42:79–87.
- Peterson DM. 2001. Oat Antioxidants. J Cereal Sci. 33:115–129.
- Rao ACS, Ramamoorthy B. 1981. Effect of moisture stress on yield, nutrient uptake and nutrient movement into grain in nine varieties of wheat. Indian J Plant Physiol. 24:269–282.
- Reitman S, Frankel S. 1957. A colorimetric method for the determination of serum glutamic oxalactic and glutamic pyruvic transaminases. Amer J Clin Path. 28(1):56–63.
- Sadasivam S, Manickam A. 2004. Biochemical methods. 2nd ed. New Delhi: New Age International Limited Publishers.
- Sharma GL, Agarwal RM. 2002. Potassium induced changes in nitrate reductase activity in Cicer arietinum L. Indian J Plant Physiol. 7(3):221–226.
- Sharma GL, Agarwal RM, Singh RP. 2006. Potassium induced changes in certain aspects of nitrogen metabolism in chick pea (Cicer arietinum L.). Physiol Mol Biol Plants. 12(2):157–162.
- Shirazi MU, Ashraf MY, Khan MA, Naqvi MH. 2005. Potassium induced salinity tolerance in wheat. Inter J Environ Sci Tech. 2:233–236.
- Singh J, Sharma HL, Singh CM. 2000. Effect of levels & phases of potassium application on growth & yield of rice and wheat. J Pot Res. 16(1–4):35–40.
- Srivastava HS. 1974. In vivo activity of nitrate reductase in maize seedlings. Indian J Biochem Biophys. 11:230–232.
- Sun T, Xu Z, Godber JS, Prinyawiwatkul W. 2006. Capabilities of oat extracts in inhibiting cholesterol & long chain fatty acid oxidation during heating. Cereal Chem. 83(4):451–454.
- Swain T, Hills WE. 1959. The phenolic constituents of Purnus domestica: the quantitative analysis of phenolic constituents. J Sci Food Agri. 10:63–68.
- Tiwari HS, Agarwal RM, Bhatt RK. 1998. Photosynthesis, stomatal resistance and related characteristics as influenced by-potassium under normal water supply and water stress conditions in rice (Oryza sativa L). Indian J Plant Physiol. 3:314–316.
- Tomar NS, Agarwal RM. 2013. Influence of treatment of Jatropha curcas L. leachates and potassium on growth and phytochemical constituents of wheat (Triticum aestivum L.). Amer J Plant Sci. 4:1134–1150.
- Torre FDL, Suarez MF, Santis LD, Canovas FM. 2007. The aspartate aminotransferase family in conifers. biochemical analysis of a prokaryotic-type enzyme from maritime pine. Tree Physiol. 27:1283–1291.
- Umar S. 2006. Alleviating adverse effects of water stress on yield of sorghum, mustard and groundnut by potassium application. Pak J Bot. 38(5):1373–1380.
- Wang M, Zheng Q, Shen Q, Guo S. 2013. The critical role of potassium in plant stress response. Int J Mol Sci. 14(4):7370–7390.
- Weatherley PE. 1950. Studies in water relations of cotton plant in the field measurements of water deficits in leaves. New Phytol. 49:81–97.
- Yu-Chuan D, Chun-Rong C, Wen L, Yan-Shou W, Xiao-Li R, Ping W, Guo-Hua X. 2008. High potassium aggravates the oxidative stress induced by magnesium deficiency in rice leaves. Pedosphere. 18(3):316–327.
- Zorb C, Senbayramb M, Peiter E. 2014. Potassium in agriculture – Status and perspectives. J Plant Physiol. 171:656–669.