Abstract
In this study, we investigated the physiological effects of three synthetic 1,2,3-thiadiazole compounds (compound I: 4-[1,2,3]-Thiadiazole-4-yl-phenol, compound II: 1,3-Bis[4-(1,2,3-thiadiazol-4-yl)phenoxy]propane, compound III: 4-(4-{2,3,4,5,6-Penta[4-(1,2,3-thiadiazole-4-yl)phenoxymethyl]benzyloxy}phenyl)-1,2,3-thiadiazole) treatments on plants via the characterization of γ-aminobutyric acid metabolite level, the accumulation of reactive oxygen species (ROS), total protein contents, total carbohydrate contents, fresh weight and dry mass in two lentil (Lens culinaris Medik) cultivars (Jordan 1 and Jordan 2). In response to the three synthetic 1,2,3-thiadiazole compounds (I, II and III) treatments. A significant increase in γ- aminobutyric acid (GABA) and malondialdehyde (MDA) levels and a significant reduction in carbohydrates and protein levels and fresh weight and dry mass were obtained in both lentil cultivars. Jordan 2 cultivar showed the highest GABA and MDA accumulation and significant reduction in the carbohydrate and protein levels under the various thiadiazole compounds treatments. This suggests that GABA molecule may act as a defense mechanism and signaling molecule in carbohydrate metabolism and other physiological systems in lentil. In conclusion, our results revealed that the synthetic 1,2,3-thiadiazole compound ІІ (1,3-Bis[4-(1,2,3-thiadiazol-4-yl)phenoxy]propane) had the strongest physiological inhibitory effect on lentil.
1. Introduction
Plants interact with many surrounding environmental conditions such as: water, soil content, climate, other organisms and external chemicals. Thiadiazole compounds are chemicals that interact with plants, cause physiological responses and affect their growth (Khalil Citation2007). Thiadiazole compounds have both sulfur and nitrogen atoms and many properties that allow plants to grow rapidly in research field (Wang et al. Citation2011). Many thiadiazole derivatives such as buthidiazole and tebuthiuron have herbicidal activity and are used for selective weed control in corn (Zea mays L.) and sugarcane (Sacchan officiaum L.) (Kubo et al. Citation1970; Hatzios et al. Citation1980; Nomura & Hilton Citation1982). The herbicidal effects of these thiadiazole derivatives occurs through inhibition of photosynthesis, prevention of starch accumulation in bundle sheath, ultra-structural disruption of mesophyll chloroplast and inhibition of electron transport and photophosphorylation (Hatzios et al. Citation1980). Another derivative of thiadiazole compounds is 3-Arylimino-5,6-dihydro-3H-thiazolo[2,3-c][1,2,4]thiadiazole which also has herbicidal activities (Hagiwara et al. Citation1993). During the treatments of foliage Amaranthus retroflexus with this derivative, plants showed fast acting and light dependent herbicidal action, bleaching symptoms, wilting, desiccation and finally plant death (Hagiwara et al. Citation1993). Similarly; a new series of 2-cyanoacrylates containing 1,2,3-thiadiazole ring moiety have herbicidal activity against amaranth pigweed, rape, alfalfa and hair crabgrass (Wang et al. Citation2010). In addition to the herbicidal activity, [1,2,4 ]triazolo[3,4-b][1,3,4] thiadiazole derivatives showed growth regulatory effects on different plants due to the presence of toxophoric N-C-S moiety (Khalil Citation2007). 2-Aminothiazoles were known to be intermediates in the synthesis of some antibiotics and synthetic dyes (Gholivand et al. Citation2010). Some of the 5-substituted-l,3,4-thiadiazol-2-yl) urea and pyrimidine derivatives especially some fused heterocyclic-2H-1,2,4-thiadiazolo[2,3-a] pyrimidine have also herbicidal activity (Kubo et al. Citation1970). Thiadiazuron and its derivatives mimick the adenine-type cytokinin resulting in the stimulation of ethylene production and growth of callus of Phaseolus lunatus cv kington (Yip & Yang Citation1986). Tiadinil, which has 1,2,3-thiadiazole group, is a fungicide for rice blast and has antibacterial effect for rice leaf blight disease (Tsubata et al. Citation2006). Benzo (1,2,3)-thiadiazole-7-carbothioic acid-S-methyl ester (BTH) is a synthetic thiadiazole which induces disease resistance by activating the systemic resistance signal transduction pathway in many plants such as bean, cauliflower, cucumber, tobacco, apple and pear (Oostendorp et al. Citation2001; Soylu et al. Citation2003). In addition, BTH decreases disease severity against powdery mildew in wheat and cucumber (Gorlach et al. Citation1996). Because Thiadiazole compounds cause many physiological, developmental and biochemical changes, they are expected to play an important role in the accumulation of γ-aminobutyric acid (GABA), which is likely mediated via increased synthesis from glutamate and possibly by decreased catabolism or changes in intracellular or intercellular transport (Kuznetsov and Shevyakova, Citation1999). Various abiotic stresses lead to the overproduction of reactive oxygen species (ROS) in plants which are highly reactive, toxic and cause damage to proteins, lipids and carbohydrates (Gill & Tuteja Citation2010). Under such stress conditions, plants may make specific changes in protein synthesis that enable them to cope with stresses (Salama et al. Citation2011). Synthesis, accumulation or depletion of different families of proteins are known to be associated with plants response to stresses (Timperio et al. Citation2008).
To the authors’ knowledge; the physiological effects of three synthetic 1,2,3-thiadiazole derivatives; (compound I: 4-[1,2,3]-thiadiazole-4-yl-phenol , compound II: 1,3-Bis[4-(1,2,3-thiadiazol-4-yl)phenoxy] propane and compound III: 4-(4-{2,3,4,5,6-Penta[4-(1,2,3-thiadiazole-4-yl)phenoxymethyl]benzyloxy}phenyl) 1,2,3-thiadiazole) on plants including lentil were not previously determined. Therefore; the objective of this study was to determine the physiological effects of these three thiadiazole derivatives on two lentil (Lens culinaris Medik) cultivars (Jordan 1 and Jordan 2) in terms of seed germination, plant growth, GABA accumulation, oxidative damage, total protein and total carbohydrates contents.
2. Materials and methods
2.1. Synthetic 1,2,3-thiadiazole compounds (I, II and III):
Three synthetic 1,2,3-thiadiazole compounds (compound I: 4-[1,2,3]-Thiadiazole-4-yl-phenol, compound II: 1,3-Bis[4-(1,2,3-thiadiazol-4-yl)phenoxy]propane and compound III: 4-(4-{2,3,4,5,6-Penta[4-(1,2,3-thiadiazole-4-yl)phenoxymethyl]benzyloxy}phenyl)1,2,3-thiadiazole) () were obtained from Dr. Mousa Al-Smadi Laboratory, Department of Chemistry, Faculty of Science and Arts, Jordan University of Science and Technology. Compounds I & III were prepared according to AL-Smadi and Meier (Citation1997) and compound II was prepared according to Al-Smadi et al. (Citation1997). The three compounds were used to prepare stock solutions by dissolving each one in Dimethyl Sulfoxide (DMSO, 78.13 g/mol) which act as surfactant (Galaz-Montoya & Maldonado Citation2006). Then four different concentrations (0, 5, 25 and 50 µg/L) from each stock solution were prepared in distilled water and used for plant treatments.
2.2. Plant materials and growth conditions:
Two Jordanian lentil cultivars (Lens culinaris Medik); Jordan 1 and Jordan 2 used in this study were obtained from Jordan National Center for Agricultural Research and Extension (NCARE). Jordan 1 cultivar seeds were selected from the land race accession number UJC176, with yellow cotyledon, which grows well in medium to high rainfall areas (Snobar & Haddad Citation1993). Jordan 2 cultivar seeds were selected from cross between UJL195XILL4400, the selection number was 82S15 with red cotyledon and large seed, which grows well in medium rainfall areas (Snobar & Haddad Citation1993). The lentil seed lot that used in this study for both cultivars was produced in 2013 (NCARE). All experiments were conducted in a growth chamber using synthetic soil (promix). Seedlings were grown for 14 days under continuous illumination (40µmol m−2 s −1) provided by cool white fluorescent lamps at 25°C. 14 days old seedlings were sprinkled completely to saturation level with 100 ml of four concentrations (0, 5, 25 and 50 µg/L) of the three synthetic 1,2,3-thiadiazole compounds (I, II and III) separately for three days. After one week, treated plants shoots were collected for the experimental analysis
2.3. Seed sensitivity to synthetic 1,2,3-thiadiazole compounds (I, II and III).
Fifteen surface sterilized seeds of each lentil cultivar were placed on one layer of cotton as artificial growth surface supplemented with 2 ml of 1,2,3-thiadiazole compounds (I, II andIII) at concentrations of 0, 5, 25 and 50 µg/L separately for three days. Seeds were allowed to germinate under continuous light at 25 oC for 7 days. Emergences of radicles from seeds were scored 7 days post germinating. The effect of 1,2,3-thiadiazole compounds (I, II and III) on seed germination were calculated as germination percentage and compared to the non-treated plants. The average of three replicates was used for each treatment.
2.4. Plants growth determination
For determination of the plant growth, two parameters were applied for each treated seedlings from both lentil cultivars compared to the control; shoot fresh weight and shoot dry mass. Shoot fresh weight (gm) was determined by collecting the shoot of each seedlings sample after treatments with four 1,2,3-thiadiazole concentrations (0, 5, 25 and 50 µg/L) of each compound, separately and weighing them directly, while the shoot dry mass was determined by air drying the shoot of each seedlings sample after the treatment with the three different thiadiazole compounds using different concentrations (0, 5, 25 and 50 µg/L) separately.
2.5. Oxidative damage assay (TBARS assay)
Three separate sets of two-week old seedlings of each lentil cultivar were grown on synthetic soil and sprayed completely to saturation level with three 1,2,3-thiadiazole compounds (I, II and III) for three different concentrations (5, 25, 50 µg/L) to saturation level for 3 days. The level of malonaldehyde (MDA) as a reference for reactive oxygen species in shoot tissues of seedlings sprayed with 1,2,3-thiadiazole compounds (I, II and III); separately was determined using the TBARS assay (Heath & Packer Citation1968). Three plates with 50 seeds each were used in each replicate for each treatment. Shoot tissues were separated and frozen in liquid nitrogen. Tissue (0.5 gm) was grounded using pestle and mortar, and 0.5 mL of 0.5% (w/v) thiobarbituric acid in 20% (w/v) trichloroacetic acid and 0.5 mL 175 mM NaCl in 50 mM Tris-HCl, pH 8 was added to the grounded tissues. Tubes were heated to 90°C for 25 min. The supernatant was collected after the samples were centrifuged for 20 min at 13000 rpm. The absorbance of the supernatant was measured at 532 nm. The level of malonaldehyde (MDA) was determined as nmol/mgFW from a standard curve of MDA.
2.6. GABA-shunt metabolites extraction
GABA was extracted according to Zhang and Bown (Citation1997) with the following modifications: harvested shoot tissues of two-week old seedlings of each of the two lentil cultivars grown on synthetic soil and sprayed with 0, 5, 25 and 50 µg/L thiadiazole for three days were grounded separately with pestle and mortar then collected in 1.5 ml microcentrifuge tubes. 400 µL Methanol was added and the samples were mixed for 10 min. Liquid from samples was removed by regular evaporation overnight. 500 µL of 70 mM lanthanum chloride was added to each tube. The tubes were mixed for 15 min, and subsequently centrifuged at 15000 rpm for 5 min. Supernatants were removed to new tubes and mixed with 160 µL of 1 M KOH. After 10 min mixing, tubes were centrifuged at 13000 rpm for 5 min. The supernatant containing metabolites was transferred to a new tube and used to determine the quantity of GABA metabolite.
2.7. GABA (γ-aminobutyric acid) Level Determination
GABA (γ-aminobutyric acid) was measured according to Zhang and Bown (Citation1997) with the following modifications: The reaction mixture contained 50 µL of sample extract, 14 µL of 4 mM NADP+, 19 µL of 0.5 M potassium pyrophosphate, pH 8.6, 10 µL of (2 u/µL) GABASE enzyme (GABASE enzyme was suspended in 0.1 M potassium pyrophosphate, pH 7.2 containing 12.5% Glycerol and 5 mM β-marcaptoethanol), and 10 µL of α-ketoglutarate. The change in absorbance at 340 nm after addition of α-ketoglutarate was recorded after 90 min incubation at 25°C using the nanodrop-spectorphotometry (ND-1000). The level of GABA, nmol/mg FW, was determined using an NADPH standard curve. The average of three replicates was used for each treatment.
2.8. Total carbohydrates content determination
Total carbohydrates content was determined by using total carbohydrate protocol in which total saccharides were estimated by the anthron method (Gerhardt et al. Citation1994) with the following modification: 50 µL from all treated shoot samples were obtained and added to COD tubes and placed on ice to chill, 0.1 ml of already chilled 75% H2SO4 was added and all tubes were mixed for 5 minutes; then 0.2 ml of already chilled anthron solution was added to each tube. All COD tubes placed in water bath at 100°C and removed after 15 minutes and cooled down to room temperature. The amount of total carbohydrates in samples was estimated via reading absorbance at 578 nm against glucose standard curve.
2.9. Total proteins content determination
Total proteins content was determined by using SMART BCA Protein Assay Kit (Intron Biotechnology, Korea) according to manufacturer instructions with the following modification: 50 µL of each treated sample was obtained. 1 ml of working solution was added to each tube and mixed well. Each sample was incubated at 37ᵒC for 30 min and cooled at room temperature. The absorbance was measured for each sample at 562 nm and protein concentration was determined by using BSA standards curves.
3. Experimental design and data analysis
Randomized complete block design was the experimental design for all studies. Treatments were replicated three times for the three synthetic thiadiazole compounds. There were no interactions among assays for all treatments. The mean and standard deviation (SD) values were determined for all assays parameters. The results were expressed as the mean ± SD from triplicate assays. The data from each study were analyzed by one-way analysis of variance (ANOVA) using the Least Significant Difference (LSD) multiple comparison tests on the means. All statistical analyses were performed using the statistical product and service solution (SPSS Inc.) version 16.0. p ≤ .05 were considered significant.
4. Results and discussion
4.1. Plant growth determination of Lentil (Lens culinaris Medik) in response to synthetic 1,2,3-thiadiazole compounds treatments
There was no seed germination detected in both lentil cultivars under all treatments of 1,2,3-thiadiazole compounds compared to 73 and 77 germination percentage in Jordan 1 and Jordan 2 seeds, respectively at no treatment. Our data indicate that 1,2,3-thiadiazole stress has a powerful destructive effect on seed germination in both cultivars. Fresh weight and dry mass for shoot under all treatments for both lentil cultivras were determined. Data showed the same effects of the three 1,2,3-thiadiazole compounds on lentil seedlings. Shoot fresh weight and dry mass values reduced significantly with increasing concentrations of compound I, II and III treatments. Under the treatment of compound І, the fresh weight of Jordan 1 and Jordan 2 seedlings shoot tissues were reduced significantly (p = .004, .001). The same results were observed under compound II and compound III treatment for Jordan 1 (p = .003, .02) and for Jordan 2 (p = .002, .026) seedlings, respectively (). Similarly, shoot dry mass values reduced significantly with increasing concentrations of compound I, II and III treatments, separately. Under the treatment of compound І, the dry mass of Jordan 1 and Jordan 2 seedlings shoot were reduced significantly (p = .002, .008). The same results were observed under compound II and III treatment for Jordan 1 (p = .001, .036) and for Jordan 2 (p = .004, .04) seedlings, respectively (). Data showed that Jordan 2 seedlings were more sensitive than Jordan 1 under all treatment of the three synthetic 1,2,3-thiadiazole compounds used in this study.
Table 1. Lentil shoot fresh weight (gm) in response to the three 1,2,3-thiadiazole compounds treatment.
Table 2. Lentil shoot dry mass (gm) in response to the three 1,2,3-thiadiazole compounds treatment.
In agreement with our data; it has been found that propanil and diuron herbicides inhibited the photosynthetic electron transport of photosystem II (PSII) in chloroplasts, also inhibition of growth of L. minor during treatment with propanil was observed (Mitsou et al. Citation2006). Kozlowski and Torrie (Citation1965) reported the effect of simazine herbicide on red pine in which the plant seedlings were killed when exposed to simazine treatments. Other finding showed that treatment of maize with the recommended field dose of rimsulfuron, imazethapyr, alachlor, atrazine and fluometuron significantly reduced the magnitudes of increase in shoot fresh and dry weight of 10-day-old maize seedlings during the following 12 days (Martinetti et al. Citation1995; Ketal et al. Citation1996). In addition, chlorimuron-ethyl had toxic effects on wheat (Triticum aestivum) by inhibiting the germination rate, root, and shoot elongation (Wang & Zhou Citation2006). Furthermore, AL-Quraan et al. (Citation2014) study indicated a direct effect of cold, heat, salt and osmotic abiotic stresses on seed viability and photosynthetic pigments in two lentil (Lens culinaris Medik) cultivars (Jordan 1 and Jordan 2) that are grown in Jordan. Moreover, a marked seed germination reduction under salt and osmotic stress was reported by AL-Quraan et al. (Citation2013) in five wheat cultivars (Hurani 75, Sham I, Acsad 65, Um Qayes, and Nodsieh) in response to salt and osmoic stress treatments. Adam and Duncan (Citation2002) revealed a reduction in seed emergence and reduction in percentage of germination in many plant species under the diesel fuel contamination stress.
4.2. Oxidative Damage in Lentil (Lens culinaris Medik) in response to treatment with synthetic 1,2,3-thiadiazole compounds
Malonaldehyde (MDA) concentration increased in Jordan 1 and Jordan 2 lentil seedlings with increasing 1,2,3-thiadiazole compounds (I, II and III) concentration, independently ().
Figure 2. The levels of malondialdehyde (MDA) in 10 days old seedlings of Jordan 1 and Jordan 2 lentil cultivars exposed to 1,2,3-thiadiazole compounds (I, II and III) independently determined by TBARS assay as described in materials and methods. MDA level was determined as nmol/mg fresh weight. (a) MDA levels in response to 1,2,3-thiadiazole compound I. (b) MDA levels in response to 1,2,3-thiadiazole compound II. (c) MDA levels in response to 1,2,3-thiadiazole compound III.
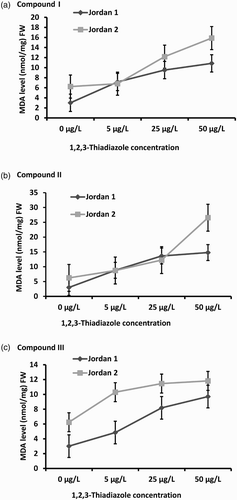
The effect of compound I on the level of oxidative damage was increased about 137.5% at 5 µg/L concentration, while it increased about 216% and 260% at 25 µg/L and 50 µg/L, respectively, in Jordan 1 seedlings (p = .001). Jordan 2 seedlings were more sensitive to 1,2,3-thiadiazole compound I treatment. Our results showed that MDA concentration increased coordinately (p = .008), it was increased about 9%, 95% and 154% at 5, 25, and 50 µg/L concentrations, respectively, ((a)). The data showed significant increase in MDA concentration for compound II at 5 µg/L where it reached 192% in Jordan 1 and 40% in Jordan 2. Under 25 µg/L treatment, MDA level in Jordan 1 was about 3 folds higher than the control compared to 96% increase in Jordan 2. At 50 µg/L, MDA accumulated in Jordan 1 up to 391% (p = .005) and 325% (p = .035) in Jordan 2 compared to the control. The oxidative damage was approximately the same in Jordan 2 in contrast to Jordan 1 at 50 µg/L ((b)).
The effect of compound III was the lowest compared to compound I and II. At 5 µg/L treatment, the MDA level increased up to 61% in Jordan 1 and about 65% in Jordan 2, while at 25 µg/L concentration, the MDA level reached 172% in Jordan 1 and 84% in Jordan 2 compared to the control. At 50 µg/L, it was about 222% in Jordan 1 (p = .005) and 89% in Jordan 2 (p = .00) compared to the control ((c)).
Many abiotic stresses lead to the overproduction of reactive oxygen species (ROS) in plants. ROS are highly reactive, toxic and cause damage to lipids and result in oxidative stress (Gill & Tuteja Citation2010). Our results come in agreement with Jiang et al. (Citation2009) who proved that MDA content was elevated with the increase of salinity concentration. Hua and Wang (Citation2003) reported that a marked increase of MDA content was observed during leaf senescence in hybrid rice. Various types of herbicides cause oxidative damage and result in MDA formation. Quinclorac is herbicide that can induce oxidative damage in Echinochloa oryzicola Vasing and a high-level of O2– production in maize roots and results in lipid peroxidation (Sunohara et al. Citation2010). Cummins et al. (Citation2006) reported that when plants were exposed to synthetic compounds such as diphenyl ether herbicides, notably lactofen, they elicit isoflavone accumulation in soybean leaves through the generation of ROS. Luo et al. (Citation2004) found that fluazifop-P-butyl increased the MDA content in a concentration-dependent manner in bristly starbur (Acanthospermum hispidum DC.). ROS formation has been described after several abiotic stresses, such as herbicides (Shaner Citation1991) including triazines, ureas, diphenyl ethers, bipyridyls and phenoxycarboxylic acids (Bowler et al. Citation1992; Romero-Puertas et al. Citation2004). MDA content was increased significantly in leaves of imazethapyr (IM)-treated plants after 10 days of treatment (Zabalza et al. Citation2007). MDA level increased significantly in response to cadmium treatment (Hegedu et al. Citation2001). The herbicidal damage of the antioxidant defense system of plants treated with high concentration of chlorimuron-ethyl herbicide in which the increased MDA level was detected in leaves and roots after one day of exposure (Wang & Zhou Citation2006). Treatment of white lupin plants with cadmium results in membrane lipid peroxidation which was observed through the significant increase of MDA levels in nodules (Carpena et al. Citation2003).
4.3. GABA level in Lentil (Lens culinaris Medik) seedlings under the treatment with 1,2,3-thiadiazole compounds.
GABA accumulated in both lentil cultivars in response to the three concentrations of the three 1,2,3-thiadiazole compounds (I, II and III) independently. Also, it was observed that GABA level in Jordan 2 was higher than GABA level in Jordan 1 under the three 1,2,3-thiadiazole compounds concentrations used in this study ().
Figure 3. Gamma-aminobutyric acid (GABA) levels in Jordan 1 and Jordan 2 lentil cultivars sprayed with different concentrations of 1,2,3-thiadiazole compounds (I, II, and III) independently. GABA was extracted and determined according to the materials and methods. The level of GABA was determined as nmol/mg FW. (a) GABA levels in response to 1,2,3-thiadiazole compound I. (b) GABA levels in response to 1,2,3-thiadiazole compound II. (c) GABA levels in response to 1,2,3-thiadiazole compound III.
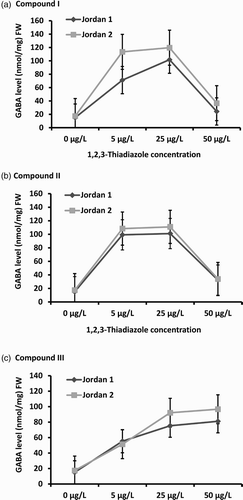
Under compound I treatments, GABA level increased in response to 5 µg/L treatment. GABA increased up to 4 folds in Jordan 1 and to 5 folds in Jordan 2 compared to the control. Furthermore; in response to 50 µg/L treatment, GABA level in Jordan 1 and Jordan 2 declined sharply up to 61% (p = .025) in Jordan 1 and 99% (p = .018) in Jordan 2 seedlings ((a)).
GABA level under compound II treatment was also increased coordinately with concentrations. At 5 µg/L, GABA level was increased about 5 folds in Jordan 1 and Jordan 2 seedlings while at 25 µg/L, the GABA level increased up to 6 folds in Jordan 1 and about 5 folds in Jordan 2 compared to the control. At 50 µg/L, GABA level was sharply reduced up to 86% (p = .016) in Jordan 1 and about 95% (p = .017) in Jordan 2 compared to the control ((b)). This observation might be explained by the lentil sensitivity and inability to tolerate the high thiadiazole compound concentration on GAD expression machinery, which might inhibit GABA production and accumulation in response to stress treatments.
The effect of the treatment with compound III was the lowest on GABA level accumulation under the three concentrations in both cultivars seedlings (Jordan 1 (p = .003) and Jordan 2 (p = .004)). At 5 µg/L, GABA level was increased up to 3 folds in Jordan 1and 2 folds in Jordan 2 and it increased coordinately to reach 4 folds in Jordan 1 and Jordan 2 compared to the control under the 25 µg/L concentration. At 50 µg/L, GABA increased in both cultivar and reached 4 folds in Jordan 1 and 5 folds in Jordan 2 seedlings compared to the control ((c)).
The 4 carbon non-protein amino acid (GABA) prevents the accumulation of reactive oxygen species under different environmental stresses and the sudden increase in GABA accumulation after exposure to abiotic stresses such as treatment with chemical compounds is an indication of its role in metabolism adjustment under stress., In agreement with our results, Renault et al. (Citation2010) reported that GABA readily accumulated during NaCl treatment in A.thialina at the whole metabolic level. AL-Quraan et al. (Citation2013) reported that high accumulation of GABA metabolite occurred under salt and osmotic stress; where authors found that GABA was the only non-amino acid out of three intermediates of GABA-shunt pathway that increased dramatically in response to osmotic stress in wheat (Triticum aestivum L.). Furthermore; the exposure of wheat seedlings to 20% polyethylene glycol for 28 h caused significant GABA accumulation (Bouche et al. Citation2003). Glyphosate herbicide caused GABA accumulation in treated plant tissues (Ulanov et al. Citation2009).
4.4. Carbohydrate contents reduction under 1,2,3-thiadiazole compounds treatments
Our study showed that the carbohydrate content decreased coordinately with the increase of the three 1,2,3-thiadiazole compounds concentration treatments (). Under the treatment with compound I, the carbohydrate content decreased under the three concentrations treatments in both cultivars (Jordan 1 (p = .003) and Jordan 2 (p = .012)) compared to the control. Carbohydrates contents were reduced up to 19% and 38% at 25 µg/L and 50 µg/L concentrations in Jordan 1 and 5%, 42%, 55% in Jordan 2 at 5, 25 and 50 µg/L treatments, respectively ((a)).
Figure 4. Total Carbohydrates levels in Jordan 1 and Jordan 2 lentil cultivars sprayed with different concentrations of 1,2,3-thiadiazole compounds (I, II,and III) independently. Carbohydrate levels were determined by using Anthron method as described in the materials and methods. The level of carbohydrate was determined as (mg/L)/FW. (a) Carbohydrates levels in response to1,2,3-thiadiazole compound I. (b) Carbohydrates levels in response to 1,2,3-thiadiazole compound II. (c) Carbohydrates levels in response to 1,2,3-thiadiazole compound III.
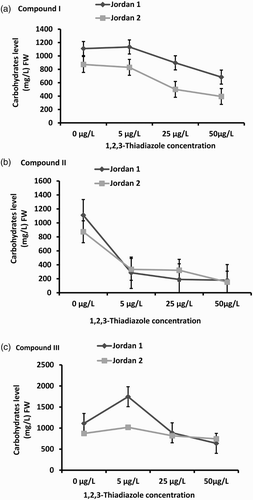
Similar results were obtained under compound II treatment. The carbohydrate content decreased significantly at the three concentrations of both Jordan 1 (p = .025) and Jordan 2 (p = .035) seedlings. At 5 µg/L, the carbohydrate level was reduced up to 74% in Jordan 1 and 62% in Jordan 2 compared to the control. At 25 µg/L, reduction level reached 83% in Jordan 1 and 63% in Jordan 2. At 50 µg/L, the reduction reached 84% in Jordan 1 and 83% in Jordan 2 compared to the control ((b)). The effect of 1,2,3-thiadiazole compound III on the carbohydrate content reduction was the lowest. It decreased at 50 µg/L to reach 43% in Jordan 1 (p = .019) and 15% in Jordan 2 (p = .001) under all concentration treatments compared to the control ((c)).
Carbohydrates are a major category of compatible solutes which accumulate in response to stress (Mustafa et al. Citation2007). It has been found that carbohydrates (starch and sucrose) level increased significantly in the two kinds of leaves (first and second leaves) in cucumber plants (Cucumis sativus) under copper stress (Alaoui-Sosse et al. Citation2004). Other results suggested that drought induced changes in carbohydrate status and metabolism in crop reproductive structures (Liu et al. Citation2004). Lower carbohydrate concentrations in roots of flooded plants were observed (Chen et al. Citation2005). In addition, it has been demonstrated that heavy metal stress reduced the rate of glycolysis and pyruvate content accompanied by an increase in the contribution of oxidative pentose phosphate pathway and residual respiration (Rakhmankulova et al. Citation2003). In these conditions, plants can switch biochemical carbohydrate pathway from primary to secondary metabolism (Pradoa et al. Citation2010). On the other hand, ozone might impair phloem loading and therefore alter carbon partitioning, causing a decrease in root carbohydrate concentration (Thomas et al. Citation2005).
In agreement with our results, under the increasing of biomass power plant effluent concentrations of carbohydrates in leaves and roots of Arachis hypogaea L. showed dramatic declining trend (Nagajyoti et al. Citation2009). Propanil and Diuron are herbicides which inhibited the photosynthetic electron transport of Photosystem II (PSII) in chloroplasts and could prevent carbohydrates production (Ahemad & Khan Citation2010). Conte and Lloyd (Citation2011) found that Diuron which is a phenylurea herbicide -used for pre and postemergence control of weeds in agriculture- inhibited photosynthesis and lead to reduce carbohydrate content and lipid synthesis in treated plant tissues.
4.5. Proteins contents reduction under synthetic 1,2,3-thiadiazole compounds treatments.
The protein content decreased coordinately with the increasing concentrations of the used 1,2,3-thiadiazole compounds (). The level of proteins content was reduced even at lowest concentration (5 µg/L) of compound I.; proteins content decreased up to 19% (p = .007) and 31% (p = .01) in Jordan 1 and Jordan 2 seedlings, respectively, in contrast with the control. Furthermore; protein level reduced up to 48% in Jordan 1 and about 53% in Jordan 2 at 50 µg/L treatment ((a)).
Figure 5. Total Protein levels in Jordan 1 and Jordan 2 lentil cultivars sprayed with different concentrations of 1,2,3-thiadiazole compounds (I, II,and III) independently. Protein levels were determined by using SMART BCA Protein Assay Kit according to the materials and methods. The level of Protein was determined as (μg/L)/FW. A) Proteins levels in response to 1,2,3-thiadiazole compound I. B) Proteins levels in response to 1,2,3-thiadiazole compound II. C) Proteins levels in response to 1,2,3-thiadiazole compound III.
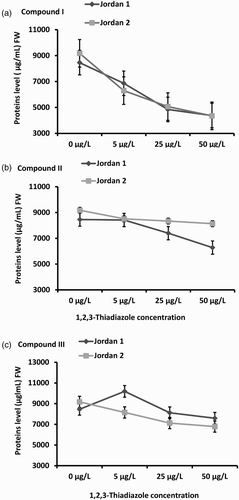
Under compound II treatment , the proteins content decreased in response to the 5, 25 and 50 µg/L concentration in both lentil cultivars (Jordan 1 (p = .001) and Jordan 2 (p = .001)). Proteins content showed 1%, 13% and 26% reduction in Jordan 1 and 7%, 9%, 11% reduction in Jordan 2 at the three concentration treatments, respectively ((b)).
The data showed that treatment with compound III reduced the proteins content in response to the three concentrations in both cultivars seedlings. At 25 µg/L, protein level was reduced up to 4% in Jordan 1 and to 22% in Jordan 2, while at 50 µg/L the reduction reached about 10% in Jordan 1and about 26% in Jordan 2 ((c)).
Under such stress conditions, plants may induce specific changes in protein synthesis to enable them to cope with stress (Salama et al. Citation2011). It has been found that exposing Vigna unguiculata (L.) plants at the age of 14 days to 75 mM sodium chloride resulted in a significant reduction of soluble protein content (Qados Citation2011). In agreement with our study, Jiang et al. (Citation2006) showed that soluble protein concentrations of seedling roots of two cotton cultivars were decreased in response to 50 and 100 mM NaCl treatments. Also it has been suggested that exposure to ultraviolet radiation reduces plant proteins, total sugars and starch contents (Salama et al. Citation2011). Under water stress, Avena coleoptile cells showed a quantitative reduction in the rate of incorporation of leucine into proteins resulting in reduction of protein contents in stressed plant tissues (Dhindsa & Cleland Citation1975).
Glyphosate herbicide inhibits the synthesis of essential aromatic amino acid which causes a decline in the synthesis of proteins. It inhibited synthesis of proteins in mainly perennial plants (Nielsen & Dahllof Citation2007; Ulanov et al. Citation2009). Nemat Alla et al. (Citation2007) reported that chloroacetanilide herbicide, affected some cellular metabolic processes like reducing the rate of protein synthesis in treated plants.
Treatments of maize with the recommended dose of rimsulfuron, imazethapyr, alachlor, atrazine and fluometuron resulted in significant decrease in leaf protein content (Scarponi et al. Citation1996; Hassan & Nemat Alla Citation2005). All these herbicides induced a general reduction in maize growth and protein content. Fluometuron and atrazine inhibit photosynthesis, therefore they indirectly retard protein skeleton formation and subsequently enzyme activity (Nemat Alla et al. Citation2007). Endothall herbicide is a strong inhibitor of plant serine/threonine protein phosphatases and causes membrane dysfunction and reduction in protein synthesis (Dayan & Watson Citation2011).
Zabalza et al. (Citation2007) reported that after 7 days of imazethapyr (IM)-treatment; pea plants showed a modified metabolism, including a decrease in soluble protein levels. In addition; Deng et al. (Citation2012) found that thiobencarb at concentrations ranging from 2 to 6 mg/L led to significant decrease in growth, DNA, RNA and total protein content of Nostoc muscorum and the nodule concentrations of soluble protein decreased by 23% compared with the control treatment values. In agreements with our study; Carpena et al. (Citation2003) showed that the sulfonylurea herbicides-chlorsulfuron and sulfometuron methyl- act on plant tissue by blocking the biosynthesis of the amino acids valine and isoleucine which reduce the protein content in treated plants (Ray Citation1984).
In summary, our study showed that there was an inhibition effect on germination and a remarkable decrease in the fresh weight and the dry mass of both lentil cultivars (Jordan 1 and Jordan 2) under the three 1,2,3-thiadiazole compounds stress compared to the control . Also there was a significant increase in GABA metabolite and MDA level and a significant decrease in proteins and carbohydrates contents in Jordan 1 and Jordan 2 cultivars under treatment with the three 1,2,3-thiadiazole compounds.
From this study, we revealed that compound ІІ had the strongest inhibition effect so we recommend using it as herbicide. Also it has been found that Jordan 2 cultivar was more sensitive than Jordan 1, so Jordan 1 cultivars showed higher adaptation to herbicidal stress. Further investigation on the GABA-shunt roles in different plants in response to changing environmental and genetics perturbations is recommended.
Additional information
Funding
References
- Adam G, Duncan H. 2002. Influence of diesel fuel on seed germination. Environ Pollut. 120:363–370.
- Ahemad M, Khan MS. 2010. Ameliorative effects of Mesorhizobium sp. MRC4 on chickpea yield and yield components under different doses of herbicide stress. Pestic Biochem Physiol. 98:183–190.
- Alaoui-Sosse B, Genet P, Vinit-Dunand F, Toussaint M, Epron D, Badot P. 2004. Effect of copper on growth in cucumber plants (Cucumis sativus) and its relationships with carbohydrate accumulation and changes in ion contents. Plant Sci. 166:1213–1218.
- AL-Quraan NA, AL-Sharbati M, Dababneh Y, AL-Olabi M. 2014. Effect of temperature, salt and osmotic stresses on seed germination and chlorophyll contents in lentil (Lens culinaris Medik). Acta Hort. (ISHS) 1045:47–54. http://www.actahort.org/books/1054/1054_4.htm
- AL-Quraan NA, Sartawe FA, Qaryouti MM. 2013. Characterization of -aminobutyric acid metabolism and oxidative damage in wheat (Triticum aestivum L.) seedlings under salt and osmotic stress. J Plant Physiol. 170:1003–1009.
- AL-Smadi M, Hanold N, Meier H. 1997. Multiple 1,2,3-thiadiazoles. J Heterocycl Chem. 34:605–611.
- AL-Smadi M, Meier H. 1997. Multi –Arm 1,2,3-thiadiazole systems. Liebigs Ann./Recl. 1997:2357–2361.
- Bouche N, Lacombe B, Fromm H. 2003. GABA signaling: a conserved and ubiquitous mechanism. Trends Cell Biol. 13:607–610.
- Bowler C, Montagu MV, Inze D. 1992. Superoxide dismutase and stress tolerance. Annu Rev Plant Physiol Plant Mol Biol. 43:83–116.
- Carpena RO, Vázquez S, Esteban E, Fernández-Pascual M, Rosario de Felipe M, Zornoza P. 2003. Cadmium-stress in white lupin: effects on nodule structure and functioning. Plant Physiol Biochem. 41:911–919.
- Chen H, Qualls RG, Blank RR. 2005. Effect of soil flooding on photosynthesis, carbohydrate partitioning and nutrient uptake in the invasive exotic Lepidium latifolium. Aquat Bot. 82:250–268.
- Conte SS, Lloyd AM. 2011. Exploring multiple drug and herbicide resistance in plants—Spotlight on transporter proteins. Plant Sci. 180:196–203.
- Cummins I, Brazier-Hicks M, Stobiecki M, Franski R, Edwards R. 2006. Selective disruption of wheat secondary metabolism by herbicide safeners. Phytochemistry. 67:1722–1730.
- Dayan FE, Watson SB. 2011. Plant cell membrane as a marker for light-dependent and light-independent herbicide mechanisms of action. Pestic Biochem Physiol. 101:182–190.
- Deng L, Senseman SA, Gentry TJ, Zuberer DA, Weiss TL, Devarenne TP, Camargo ER. 2012. Effect of selected herbicides on growth and hydrocarbon content of Botryococcus braunii (race B). Ind Crops Prod. 39:154–161.
- Dhindsa RS, Cleland RE. 1975. Water stress and protein synthesis. I. differential inhibition of protein synthesis. Plant Physiol. 55:778–781.
- Galaz-Montoya ML, Maldonado AD. 2006. Effect of DMSO on bilayers made from a neutral surfactant (C14DMAO). AIP Conf Proc. 854, 182; http://dx.doi.org/10.1063/1.2356441.
- Gerhardt P, Murray RGE, Wood WA, Kreig NR. 1994. Methods for general and molecular bacteriology. American Society for Microbiology, Washington, DC. ISBN, 1-55581-048-9, p518.
- Gholivand K, Farshadian S, Erben MF, Védova COD. 2010. Synthesis and characterization of the first phosphonic diamide containing thiazolyl groups: Structural properties and tautomeric equilibrium. J Mol Struct. 978:67–73.
- Gill SS, Tuteja N. 2010. Reactive oxygen species and antioxidant machinery in abiotic stress tolerance in crop plants. Plant Physiol Biochem. 48:909–930.
- Gorlach J, Volrath S, Knauf-Beiter G, Hengy G., Beckhove U, Kogel KH, Oostendorp M, Staub T, Ward E, Kessmann H, Ryals J. 1996. Benzothiadiazole, a novel class of inducers of systemic acquired resistance, activates gene expression and disease resistance in wheat. Plant Cell. 8:629–643.
- Hagiwara K, Saitoh K, Iihama T, Kawana T, Hosaka H. 1993. Synthesis and herbicidal activity of fused Δ2–1,2,4-thiadiazolines: synthesis and biological activity of 1, 2, 4-thiadiazolines (Part 2). J Pesticide Sci. 18:309–318.
- Hassan NM, Nemat Alla MM. 2005. Oxidative stress in herbicide-treated broad bean and maize plants. Acta Physiol Plant. 27:429–438.
- Hatzios KK, Penner D, Bell D. 1980. Inhibition of photosynthetic electron transport in isolated spinach chloroplasts by two 1, 3, 4-thiadiazolyl derivatives. Plant Physiol. 65:319–321.
- Heath RL, Packer L. 1968. Photoperoxidation in isolated chloroplasts: I. kinetics and stoichiometry of fatty acid peroxidation. Arch Biochem Biophys. 125:189–198.
- Hegedu A, Erdei S, Horvath G. 2001. Comparative studies of H2O2 detoxifying enzymes in green and greening barley seedling under cadmium stress. Plant Sci. 160:1085–1093.
- Hua C, Wang R. 2003. Changes of SOD and CAT activities and MDA content during senescence of hybrid rice and three lines leaves. Acta Bot Bor-Occident Sin. 23:406–409.
- Jiang J, Zhuang JY, Fan YY, Shen B. 2009. Mapping of QTLs for leaf malondialdehyde content associated with stress tolerance in rice. Rice Science. 16:72–74.
- Jiang L, Duan L, Tian X, Wang B, Zhang H, Zhang M, Li Z. 2006. NaCl salinity stress decreased Bacillus thuringiensis (Bt) protein content of transgenic Bt cotton (Gossypium hirsutum L.) seedlings. Environ Exp Bot. 55:315–320.
- Ketal DH, Wielen MJW, Lotz LAP. 1996. Prediction of a low dose herbicide effect from studies on binding of metribuzin to the chloroplasts of Chenopodium album L. Ann. Appl. Biol. 128:519–531.
- Khalil NS. 2007. N- and S-α-L-Arabinopyranosyl [1,2,4] triazolo [3,4-b][1,3,4 ]thiadiazoles. First synthesis and biological evaluation. Eur J Med Chem. 42:1193–1199.
- Kozlowski TT, Torrie JH. 1965. Effect of soil incorporation of herbicides on seed germination and growth of pine seedlings. Soil Sci. 100:139–146.
- Kubo H, Sato R, Hamura I, Ohi T. 1970. Herbicidal activity of 1,3,4-thiadiazole derivatives, J. Agr Food Chem. 18:60–65.
- Kuznetsov VV, Shevyakova NI. 1999. Proline under stress: biological role, metabolism and regulation. Russ J Plant Physiol. 46:274–287.
- Liu F, Jensen CR, Andersen MN. 2004. Drought stress effect on carbohydrate concentration in soybean leaves and pods during early reproductive development: its implication in altering pod set. Field Crops Res. 86:1–13.
- Luo XY, Sunohara Y, Matsumoto H. 2004. Fluazifop-butyl causes membrane peroxidation in the herbicide-susceptible broad leaf weed bristly starbur (Acanthospermum hispidum). Pestic Biochem Physiol. 78:93–102.
- Martinetti L, Scarponi L, Nemat Alla MM. 1995. Effect of rimsulfuron and its major product on ALS activity and on protein and starch formation in maize. Brighton Crop Prot Conf-Weeds. 1:405–410.
- Mitsou K, Koulianou A, Lambropoulou D, Pappas P, Albanis T, Lekka M. 2006. Growth rate effects, responses of antioxidant enzymes and metabolic fate of the herbicide Propanil in the aquatic plant Lemna minor. Chemosphere. 62:275–284.
- Mustafa RM, Jouve L, Hausman J-F, Hoffmann L, Stewart JMcD. 2007. Alteration of oxidative and carbohydrate metabolism under abiotic stress in two rice (Oryza sativa L.) genotypes contrasting in chilling tolerance. J Plant Physiol. 164:157–167.
- Nagajyoti PC, Dinakar N, Suresh S, Udaykiran Y, Suresh C, Prasad TNVKV, Damodharam T. 2009. Biomass industrial effluent effect on carbohydrates, amino acids, nitrite and nitrite enzyme activities of Arachis hypogaea L. Agr Sci China. 8:203–215.
- Nemat Alla MM, Hassan NM, El-Bastawisy ZM. 2007. Differential influence of herbicide treatments on activity and kinetic parameters of C4 photosynthetic enzymes from Zea mays. Pestic Biochem Physiol. 89:198–205.
- Nielsen LW, Dahllof I. 2007. Direct and indirect effects of the herbicides Glyphosate, Bentazone and MCPA on eelgrass (Zostera marina). Aquat Toxicol. 82:47–54.
- Nomura NS, Hilton HW. 1982. Distribution of residues and metabolism of the herbicide buthidazole in sugarcane from root and foliar treatments. J Agric Food Chem. 30:232–238.
- Oostendorp M, Kunz W, Dietrich B, Staub T. 2001. Induced disease resistance in plants by chemicals. Eur J Plant Pathol. 107:19–28.
- Pradoa C, Rodríguez-Montelongo L, Gonzalez JA, Pagano EA, Hilal M, Prado FE. 2010. Uptake of chromium by Salvinia minima: Effect on plant growth, leaf respiration and carbohydrate metabolism. J Hazard Mater. 177:546–553.
- Qados AMSA. 2011. Effect of salt stress on plant growth and metabolism of bean plant Vicia faba (L.). JSSAS. 10:7–15.
- Rakhmankulova ZF, Fedyaev VV, Podashevka OA, Usmanov IYu. 2003. Alternative respiration pathways and secondary metabolism in plants with different adaptive strategies under mineral deficiency. Russ J Plant Physiol. 50:206–212.
- Ray TB. 1984. Site of action of chlorsulfuron inhibition of valine and isoleucine biosynthesis in plants. Plant Physiol. 75:827–831.
- Renault H, Roussel V, El Amrani A, Arzel M, Renault D, Bouchereau A, Deleu C. 2010. The Arabidopsis pop2-1 mutant reveals the involvement of GABA transaminase in salt stress tolerance. BMC Plant Biol. 10:20. doi:10.1186/1471-2229-10-20
- Romero-Puertas MC, McCarthy I, Gomez M, Sandalio LM, Corpas FJ, Del Rio LA, Palma JM. 2004. Reactive oxygen species-mediated enzymatic systems involved in the oxidative action of 2,4-dichlorophenoxyacetic acid. Plant Cell Environ. 27: 1135–1148.
- Salama HMH, AL Watban AA, Al-Fughom AT. 2011. Effect of ultraviolet radiation on chlorophyll, carotenoid, protein and proline contents of some annual desert plants. Saudi J Biol Sci. 18:79–86.
- Scarponi L, Martinetti L, Nemat Alla MM. 1996. Growth responses and changes in starch formation as a result of imazethapyr treatment to soybean (Glycine max L.). J Agric Food Chem. 44:1572–1577.
- Shaner DL. 1991. Physiological effects of the imidazolinone herbicides. In: Shaner DL, O'Connor SL. The Imidazolinone Herbicides. Boca Raton, FL: CRC Press Inc; p. 129–137.
- Snobar B, Haddad N. 1993. Food legumes improvement project. 1980–1992. General knowledge Series. No 1095. p. 7. University of Jordan Publication. Faculty of Agriculture. September 1993.
- Soylu EM, Soylu S, Baysa O. 2003. Induction of disease resistance and antioxidant enzymes by acibenzolar-s-methyl against bacterial canker (Clavibacter michiganensis subsp. Michiganensis) in tomato. J Plant Pathol. 85:175–181.
- Sunohara Y, Shirai S, Wongkantrakorn N, Matsumoto H. 2010. Sensitivity and physiological responses of Eleusine indica and Digitaria adscendens to herbicide quinclorac and 2,4-D. Environ Exp Bot. 68:157–164.
- Thomas VFD, Braun S, Fluckiger W. 2005. Effects of simultaneous ozone exposure and nitrogen loads on carbohydrate concentrations, biomass, and growth of young spruce trees (Picea abies), Environ Pollut. 137:507–516.
- Timperio AM, Egidi MG, Zolla L. 2008. Proteomics applied on plant abiotic stresses: role of heat shock proteins (HSP). J Proteomics. 71:391–411.
- Tsubata K, Kuroda K, Yamamoto Y, Yasokawa N. 2006. Development of a novel plant activator for rice diseases, tiadinil. J. Pestic Sci. 31:161–162.
- Ulanov A, Lygin A, Duncan D, Widholm J, Lozovaya V. 2009. Metabolic effects of glyphosate change the capacity of maize culture to regenerate plants. J Plant Physiol. 166: 978–987.
- Wang H, Yang Z, Fan Z, Wu Q, Zhang Y, Mi N, Wang S, Zhang Z, Song H, Liu F. 2011. Synthesis and insecticidal activity of N-tert-Butyl-N, N-diacylhydrazines containing 1,2,3-Thiadiazoles. J Agric Food Chem. 59:628–634.
- Wang M, Zhou Q. 2006. Effects of herbicide chlorimuron-ethyl on physiological mechanisms in wheat (Triticum aestivum L.). Ecotoxicol Environ Saf. 64:190–197.
- Wang TT, Bing GF, Zhang X, Qin ZF, Yu HB, Qin X, Dai H, Fang J. 2010. Synthesis, crystal structure and herbicidal activity of novel 1,2,3-thiadiazole substituted 2-cyanoacrylates. Arkivoc. ii:330–339.
- Yip WK, Yang SF. 1986. Effect of thidiazuron, a cytokinin-active urea derivative, in cytokinin-dependent ethylene production systems. Plant Physiol. 80:515–519.
- Zabalza A, Gaston S, Sandalio LM, del Rio LA, Royuela M. 2007. Oxidative stress is not related to the mode of action of herbicides that inhibit acetolactate synthase. Environ Exp Bot. 59:150–159.
- Zhang G, Bown AW. 1997. The rapid determination of γ-aminobutyric acid. Phytochemistry. 44:1007–1009.