Abstract
The objective of this study was to understand and characterize the physiological and biochemical tolerance mechanisms of Argania spinosa under drought stress for selection tolerant ecotypes. Significant differences were observed among ecotypes in indices of leaf water status studied: stomatal conductance (gs), predawn leaf water potential (Ψpd) and leaf relative water content. There was a significant decrease in these physiological traits with increasing degree of drought stress in all ecotypes. Drought stress significantly increased endogenous H2O2 and lipid peroxidation. Moderate and severe drought stress increased significantly the catalase, superoxide dismutase, peroxidase, polyphenoloxidase and lipoxygenase activities, depending on time. Their constitutive activities were higher in inland ecotypes than in coastal ecotypes. According to canonical discriminant analysis, the inland ecotypes were essentially distinguished from the coastal ecotypes by the following physiological and biochemical traits: Ψpd, gs, polyphenol oxidase, superoxide dismutase and malonyldialdehyde. Inland ecotypes seem to be more tolerant to drought stress than coastal ecotypes.
Introduction
In Mediterranean ecosystem, the natural vegetation is often subjected to a continuous and severe drought stress (Nogués & Baker Citation2000). Some endemic species are well adapted to these conditions like the argan tree in Morocco (Msanda et al. Citation2005; Díaz-Barradas et al. Citation2010). The argan tree [(Argania spinosa (L.) Skeels] is endemic to the Southwestern part of Morocco, where it grows in over 800,000 hectares (Msanda et al. Citation2005). This tree has important socioeconomical and ecological roles in this area, in which it also plays a great role in the biodiversity of the forest's ecosystem (Msanda et al. Citation2005). About 1.3 million people are living in rural areas where traditional sylvo-pastoral systems are based on the argan tree (Chaussod et al. Citation2005). Argan oil is thought to be one of the highest quality vegetable oils, has a high nutritional and dietetic value due to its chemical composition.
Plant responses to drought stress have species- and genotype-dependent characteristics. Certain species have developed adaptive mechanisms to tolerate drought (Pita et al. Citation2005). Drought tolerance stimulates subtle variations in the biochemical and physiological processes. In fact, the physiological mechanisms implicated in the stomatal response to environmental conditions are diverse and have various quantitative effects on stomatal conductance (Tardieu & Simonneau Citation1998). Stomata close during the period of drought stress to respond to variations in soil water status and/or leaf water potential (Pita et al. Citation2005). Drought stress causes also an increase in the cellular level of reactive oxygen species (ROS), reflecting an oxidative stress (Møller et al. Citation2007; Pyngrope et al. Citation2013). To maintain homeostasis and prevent oxidative stress, the plants have evolved a scavenging system composed of certain enzymes such as catalase (CAT, EC 1.11.1.6), superoxide dismutase (SOD, EC 1.15.1.1) and peroxidase (POD, EC 1.11.1.7), which can minimize the cellular damage (Gill &Tuteja Citation2010). The ability to increase antioxidant enzyme activities has been correlated with a degree of drought tolerance in A. spinosa (Chakhchar et al. Citation2015a) and in multiple species such as Olea europea (Ben Ahmed et al. Citation2009; Boughalleb & Mhamdi Citation2011). Thus, polyphenol oxidase (PPO, EC1.14.18.1) can involve in the regulation of the redox state of phenolic compounds under drought stress (Rivero et al. Citation2001). When the generation of ROS is excessive under drought conditions and exceeds the scavenger capacity of the antioxidant system, they cause important oxidative damage to membrane lipids (Smirnoff Citation1993). This damage is quantified by variation in the malonyldialdehyde (MDA) level which essentially reflects lipid peroxidation (Chandra &Dubey Citation2008; Farouk et al. Citation2013). Lipoxygenases (LOX, EC 1.13.11.12) catalyse the dioxygenation of polyunsaturated fatty acids, generating hydroperoxy fatty acids which are highly reactive to initiate cell damage (Feussner & Kindl Citation1994; Ye et al. Citation2000).
In this study, our aims are to (i) understand the physiological and biochemical tolerance mechanisms and to test in A. spinosa the hypothesis that oxidative stress can cause disruptions in normal cellular mechanisms involved in drought tolerance, (ii) characterize the impact of drought stress on the activity of antioxidant enzymes and leaf water status and (iii) assess differences in physiological and biochemical traits studied between four contrasting A. spinosa ecotypes.
Materials and methods
Plant material, growth conditions and experimental layout
Sampling of seeds of A. spinosa was conducted in four regions of the argan tree forest in South-West Morocco. Climatic, geographical and hydrological conditions of these four regions are markedly different (Chakhchar et al. Citation2015a, Citation2015b). We chose two contrasting coastal ecotypes (site: Rabia (Rab) and Admine (Adm)) and two contrasting inland ecotypes (site: Aoulouz (Alz) and Lakhssas (Lks)) for a better interpretation of the mechanisms regulating biochemical and physiological processes. Uniform young A. spinosa plants of similar height, aged 14 months, were selected for the experiment for each ecotype. The protocol of cultivation and experimental layout was the same used previously (Chakhchar et al. Citation2015a, Citation2015b). The environmental conditions in chamber during the experiment were maintained at 28 ± 1°C temperatures during day and 25 ± 1°C during night in a 16:8 photoperiod and the rate of relative humidity was varied between 65% and 70%. The average maximum photosynthetically active radiation was approximately 400 µmol m−2 s−1 ensured by a combination of incandescent and fluorescent lamps.
The plants were randomly exposed to three levels of stress included the control treatment (100% of field capacity (FC)) and two stress treatments (50% and 25% of FC) which correspond to medium and to severe drought stress, respectively. The treatments were applied for two months in order to elucidate the effect of prolongation of drought stress levels on A. spinosa.
Physiological traits
Predawn water potential (Ψpd)
At predawn (05:00 to 06:00 h), Ψpd was determined with a pressure chamber (Skye Instruments, Powys, UK). Measurements were taken in the upper part of the stem (3 cm) with five leaves per plant (five plants/treatment).
Stomatal conductance (gs)
gs was determined using a leaf porometer (Decagon Devices, Pullman, WA, USA). Measurements were made between 10.00 and 12.00 h on leaves. The data were collected from two leaf samples per plant (five plants/treatment).
Leaf relative water content
Relative water content (RWC) was measured on five whole leaves per plant (five plants/treatment). RWC was calculated as RWC = (FW−DW)/(SW−DW) × 100, where FW is the fresh weight, SW is the turgid weight after leaves were soaked in distilled water for 24 h at 4°C in the dark and DW is the dry weight after the leaves were dried for 48 h at 70°C.
Biochemical traits
Enzymes extraction
The fresh leaf samples from control and treated plants were immediately grounded into a fine powder with liquid nitrogen. Using a potassium phosphate (K2HPO4/KH2PO4) buffer, the antioxidant enzymes were extracted by homogenizing on ice the powder (0.1 g for each antioxidant enzyme) in 50 mM K2HPO4/KH2PO4 (pH 7.8) containing 1% (w/v) polyvinyl pyrrolidone (PVP), ethylenediaminetetraacetic acid (EDTA), 5 mM 2-mercaptoethanol, 0.2% (v/v) Triton X100 and 0.1 mM, 0.1 mM phenylmethanesulfonyl fluoride solution (PMSF) for the determination of CAT, POD and SOD. A K2HPO4/KH2PO4 buffer (pH 5.5) was used for PPO. After centrifugation at 15,000 × g at 4°C for 15 min, the supernatant was used to determine the enzymatic activities and protein content. We opted to five replicates per treatment. According to Bradford (Citation1976), total soluble protein content for the determination of the specific activities of the enzymes was determined using bovine serum albumin as a standard.
All spectrophotometric measurements were taken in a Jenway (6305 UV/VIS, England) spectrophotometer.
Catalase
CAT activity was determined according to the method of Aebi (Citation1984). The assay mixture consisted of 50 mM K2HPO4/KH2PO4 (pH 7.0), 10 mM H2O2 and 0.1 mL enzyme extract. An extinction coefficient (E) = 39.4 mM−1 cm−1 at 240 nm was adopted to express the CAT activity as units: nmol of H2O2 decomposed/min/mg of protein.
Superoxide dismutase
Total SOD activity was evaluated by monitoring its ability to inhibit the photochemical reduction of p-nitrobluetetrazolium chloride (NBT) at 560 nm according to the method of Beauchamp and Fridovich (Citation1971). The reaction mixture contained 50 mM K2HPO4/KH2PO4 buffer (pH 7.8), 0.1 mM EDTA, 33 µM NBT, 10 mM l-Methionine, 4 µM riboflavin and enzyme extract. Reactions were carried out for 8 min at a light intensity of 50 mmol photons m−2 s−1. One unit of SOD activity was defined as the amount of enzyme, which causes 50% inhibition of NBT reduction under the assay condition, and the results were expressed as enzyme units (nmol/min) per mg of protein.
Peroxidase
Total POD activity was measured according to the method of Zhang et al. (Citation2009). The assay mixture consisted of 100 mM K2HPO4/KH2PO4 buffer (pH 6.5), 40 mM guaiacol, 10 mM H2O2 and enzyme extract (0.1 mL). The absorbance was recorded at 436 nm and the results were expressed in nmol/min/mg of protein using an extinction coefficient of 25.5 mM−1 cm−1.
Polyphenoloxidase
Activity of PPO was estimated at 410 nm by monitoring the oxidation of catechol according to Moore and FIurkey (Citation1990). The assay mixture included 100 mM K2HPO4/KH2PO4 buffer (pH 6), 50 mM catechol and the enzymatic extract. Because of the uncertainty in the molar extinction coefficient of the oxidized product of catechol, PPO activity is calculated as enzyme units (nmol/min) per mg of protein.
Lipoxygenase
LOX activity was estimated according to the method of Axelrod et al. (Citation1981). LOX enzyme was extracted by homogenizing on ice an aliquot of frozen powder in 50 mM K2HPO4/KH2PO4 buffer (pH 7) containing 5 mM MgCl, 0.1 mM EDTA, 0.2% (v/v) Triton X100, 1% (w/v) PVP, 1 mM dithiothreitol and 0.1 mM PMSF. LOX activity was evaluated in a mixture assay consisting of 50 mM K2HPO4/KH2PO4 buffer (pH 6.5), 0.1% Tween 80, 0.5 mM linoleate and enzyme extract. The absorbance was recorded at 234 nm and the results were expressed in nmol/min/mg of protein using an extinction coefficient of 25 mM−1 cm−1.
Malonyldialdehyde
Lipid peroxidation was evaluated by measuring MDA content according to the method of Hernandez and Almansa (Citation2002). The frozen powder (100 mg) was homogenized in 0.1% (w/v) trichloroacetic acid (TCA) (2 mL). After centrifugation of the homogenate at 15,000 × g for 10 min, the supernatant (0.5 mL) was homogenized with 1.5 mL of 0.5% (w/v) thiobarbituric acid (TBA) in TCA 20% (w/v). The mixture was heated at 90°C for 20 min and then cooled quickly on an ice bath. Afterwards, the mixture was centrifuged at 10,000 × g for 5 min and the absorbance of the supernatant was measured at 532 nm and at 600 nm for the correction of nonspecific turbidity. The MDA content was calculated according to its extinction coefficient of 155 mM−1 cm−1and expressed as nmol/g.
Hydrogen peroxide
H2O2 was measured spectrophotometrically according to Nag et al. (Citation2000) based on the hydrogen peroxide–titanium (Ti) complex formation. Leaf material was ground with liquid nitrogen and the fine powdered material (0.1 g) was mixed with 3 mL 0.5% (w/v) TCA in an ice bath. The extracted solution (0.8 mL) was reacted by 0.1 mL of 15% (w/v) titanium sulfate (TiSO4) in 25% (v/v) H2SO4. The reaction mixture was centrifuged for 10 min at 10,000 × g and then the absorbance was recorded at 410 nm. The results of H2O2 content were expressed in nmol H2O2/g.
Statistical analysis
Each data was pointed the mean of five separate replicates and mean values and standard deviations were calculated. Results were examined by the three-way analysis of variance (ANOVA) in order to test the effect of ecotype, time, watering regime and their interactions in each of the physiological and biochemical study variables. Means were compared using Tukey's post hoc test. A Pearson correlation analysis was done for some variables for each ecotype. A canonical discriminant analysis (CDA), by entering all independent variables into the equation at once, was performed on the four contrasting A. spinosa to determine which variables discriminated between them. Statistical analyses were conducted using SPSS 10.0 for Microsoft Windows.
Results
Physiological traits
The results showed that effects of drought stress varied depending especially on stress duration and intensity. We noticed that the severe drought created more serious effects on the four ecotypes of the A. spinosa. The primary physiological effects of drought stress are a reduction in leaf water status, as measured by gs, Ψpd and leaf RWC ( and ). Highly significant differences (P < .001) between watering regimes (control: C, medium stress: MS and stress severe: SS) were noted for these traits. We found that both drought stress and the Argania ecotype had significant effects (P < .001) on these physiological traits. Ecotypes that exhibit low values of leaf RWC and low levels of gs are those that showed Ψpd negative values. The gs values for Rabia were the highest among ecotypes, whereas Ψpd was lowest in Lakhssas ( and ) than in the other ecotypes. A. spinosa plants subjected to drought stress showed decreases in gs and more negative Ψpd values. Positive and significant correlations at P < .01 were recorded between gs values and negative Ψpd values during experiment (r = .86, r = .90, r = .93 and r = .90 in Aoulouz, Lakhssas, Rabia and Admine, respectively). Also, positive and significant correlations at P < .01 were recorded between gs and RWC (r = .83, r = .85, r = .92 and r = .90 in Aoulouz, Lakhssas, Rabia and Admine, respectively). We observed significant differences among ecotypes in leaf water status indices studied (P < .001): Ψpd, gs and RWC. According to three-way ANOVA analysis ( and ), there were significant differences between ecotypes, watering regime, time and the interactions between them. A significant watering regime × time interaction was observed for all physiological traits studied (P < .001). Ecotype × watering regime, ecotype × time and ecotype × watering regime × time interactions were only significant for both traits: Ψpd and gs (P < .001). These statistical results illustrate intraspecific differences in water conservative strategy in A. spinosa plants under different levels of drought stress in a time-dependent manner.
Table 1. Three-way ANOVA results on the effects of ecotype, watering regime, time and the interaction among them for physiological traits: gs (mmol m−2 s−1), Ψpd (MPa) and RWC (%); and biochemical traits: SOD (UE mg−1 protein), CAT (nmol min−1 mg−1 protein), PPO (UE mg−1 protein), POD (nmol min−1 mg−1 protein), H2O2 (nmol g−1), LOX activity (nmol min−1 mg−1 protein) and MDA (nmol g−1) in leaves of four A. spinosa ecotypes exposed to three water regimes.
Table 2. Values of gs (mmol m−2 s−1), Ψpd (MPa) and RWC (%) in leaves of four A. spinosa ecotypes exposed to three water regimes (100, 50 and 25 of FC corresponding to control: C, medium stress: MS and stress severe: SS, respectively). T0: time 0 and T8: time after 8 weeks.
Biochemical traits
The effect of drought stress on antioxidant enzymes activities (SOD, CAT, PPO and POD) preventing ROS damage by scavenging free radicals is shown in . Drought stress significantly enhanced the activities of these four ROS-scavenging enzymes when compared with control. Moderate and severe stress increased significantly the activities of these enzymes, depending on time () (P < .001). SOD enzyme is responsible for the transformation of the aggressive superoxide radical to hydrogen peroxide. SOD activity was influenced by drought (MS and SS). A higher SOD activity was observed in the inland ecotypes ( and ). Constitutive and induced CAT activity values for Aoulouz and Rabia were the higher (P < .001) among ecotypes (). We recorded high values of PPO and POD activities in both ecotypes: Aoulouz and Lakhssas (P < .001) ( and ). However, we found that the constitutive and induced activities of PPO, POD and SOD in the inland ecotypes (Lakhssas and Aoulouz) were higher compared to coastal ecotypes ( and ). Only watering regime × time interaction was highly significant for all antioxidant enzymes studied (P < .001) ().
Table 3. Values of SOD (UE mg−1 protein), CAT (nmol min−1 mg−1 protein), PPO (UE mg−1 protein) and POD (nmol min−1 mg−1 protein) activities in leaves of four A. spinosa ecotypes exposed to three water regimes (100, 50 and 25 of FC corresponding to control: C, medium stress: MS and stress severe: SS, respectively). T0: Time 0 and T8: time after 8 weeks.
The MDA and H2O2 content was evaluated in A. spinosa leaves as a metabolic indicator of their status of oxidative stress. The MDA accumulation was significantly greater (P < .001) under MS and SS treatments than the control. This serious accumulation was also significantly influenced by ecotype (P < .001) and was more pronounced in Admine ecotype, whereas Aoulouz ecotype is characterized by a low MDA accumulation compared with other ecotypes ( and ). Endogenous H2O2 levels showed almost the same pattern than evolution of MDA (). Compared to control, we found a significant increase (P < .001) in MS and SS and an ecotype effect was noted at P < .001 (). As in the case of MDA, Aoulouz also shows the lowest endogenous H2O2 concentration among the four ecotypes ( and ). During the period of drought stress, the correlation between H2O2 and MDA was positive and significant at P < .01 (r = .97, r = .94, r = .85 and r = .64 in Admine, Rabia, Lakhssas and Aoulouz, respectively).
Table 4. Endogenous H2O2 (nmol g−1), LOX activity (nmol min−1 mg−1 protein) and MDA (nmol g−1) in leaves of four A. spinosa ecotypes exposed to three water regimes (100, 50 and 25 of FC corresponding to control: C, medium stress: MS and stress severe: SS, respectively). T0: time 0 and T8: time after 8 weeks.
Progressive watering regime caused marked significant increase in LOX activity in all ecotypes (P < .001) ( and ). The values of LOX activity in the four ecotypes during watering regime (MS and SS) were higher than those of the control. A higher LOX activity was observed in Lakhssas, nonetheless, Admine ecotype showed the lowest LOX activity and also a low constitutive activity compared to other ecotypes (). Positive and significant correlations at P < .01 were recorded between LOX activity and MDA content during experiment under watering regime (r = .87, r = .83, r = .82 and r = .47 in Admine, Lakhssas, Rabia and Aoulouz, respectively). According to three-way ANOVA analysis (), watering regime × time interaction was highly significant for these three traits (P < .001). Nevertheless, ecotype × watering regime, ecotype × time and ecotype × watering regime × time interactions were only significant for H2O2 and MDA (P < .001).
Canonical discriminant analysis
We tested our data by CDA using the physiological and biochemical traits (10 variables) as predictors of membership in a diagnostic group. This group corresponded to the four contrasting ecotypes of argan tree studied in our experiment. The results of CDA showed differences in physiological and biochemical characteristics of ecotypes. Wilk's lambda denoted a high significance of the model (Wilks's λ = 0.018) and calculated F-value also indicates significance (P < .001) for this factorial analysis. The three discriminant functions (DF) were calculated, accounting for 66.3%, 22.2% and 11.5% of the total variance. The χ2-test showed a significant discriminatory power for the three functions (P < .001). The eigenvalues of the first two functions (6.75 and 2.26) showed them to explain most of variance (88.5%) and their canonical correlations were r1 = 0.93 and r2 = 0.83.
The 2D scatterplot of discriminant space () displays the distribution of the samples spanned by the first two functions. Based on the standardized coefficients of the canonical DFs (), Ψpd, gs and H2O2 were highly weighted in the positive part of first DF, while PPO, SOD and LOX in the negative part. Ψpd, gs and MDA showed the highest standardized coefficients on the second DF in the positive part, whereas CAT and H2O2 were strongly weighted in the negative part. Indeed, the first DF contributed mostly to distinguish between inland and coastal ecotypes. Lakhssas was clearly separated from the other ecotypes by the second DF. Equal numbers of plants were compared in each ecotype.
Figure 1. 2D scatterplot showing the distribution of the four ecotypes studied according to the two DF gradients obtained by CDA for physiological and biochemical traits.
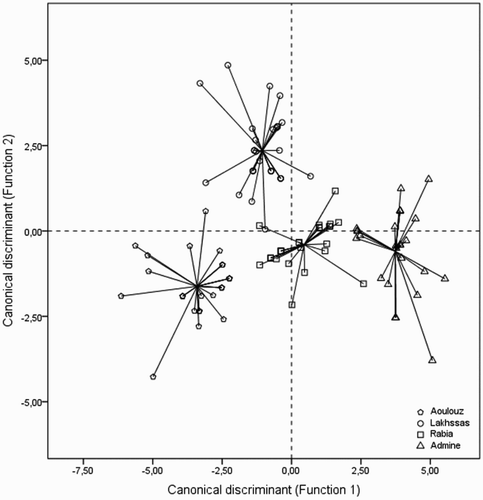
Table 5. Standardized canonical DF coefficients relative to physiological and biochemical traits according to CDA.
Discussion
A. spinosa is considered as drought-tolerant species of the Sapotaceae family (Díaz-Barradas et al. Citation2010). The reduction in Ψpd, gs and leaf RWC () was observed, reflecting a net response to drought as in several studies. Our scientific work differentiated these leaf water traits at MS and SS for all ecotypes of A. spinosa studied. Thus, we found a marked relationship between Ψpd and gs. A. spinosa plants subjected to drought stress showed decreases in gs and more negative Ψpd values. Ecotypes exhibited differences in Ψpd, with Lakhssas exhibiting the most negative values. As the stress becomes more severe, the Ψpd decreased in stressed A. spinosa plants and more stomatal closure was recorded. This agrees with the results of some studies in which qualitative relationships were described between stomatal closure and Ψpd (Gomes et al. Citation2004; Wahbi et al. Citation2005; Chakhchar et al. Citation2015b). Nonetheless, certain studies reported that gs was usually correlated with leaf water potential for numerous species, suggesting that the existence of chemical signals between the shoot and the root does not include and not explain all factors involved in the stomatal conductance mechanism (Tardieu et al. Citation1996; Tardieu & Simonneau Citation1998). Under drought stress, a positive and significant correlation is often observed between gs and leaf RWC. We noticed clear intraspecific differences in gs sensitivity, suggesting distinct tolerances to drought in relation to the ecotype effect. Rabia ecotype had high gs, whereas Lakhssas and Aoulouz were having a lower maximum stomatal conductance under severe stress ( and ).
Based on our findings, moderate and severe stress induced considerable differences between ecotypes in the leaf water status. There are some interesting differences in leaf RWC between ecotypes with different watering regimes. Leaf RWC is an important indicator that enables to evaluate physiologically the plant water status (Teulat et al. Citation1997; Farouk & Abdul Qados Citation2013). It can control the plant response to drought stress considering as an indicator of plant resource use (Garnier et al. Citation2001). Leaf RWC results of our pot-study showed that Rabia and Admine ecotypes maintained higher RWC in leaves than Lakhssas and Aoulouz ( and ). Nonetheless, the statistical results of significant interactions between fixed factors, especially ecotype × watering regime, ecotype × time and ecotype × watering regime × time for Ψpd and gs, revealed that the A. spinosa ecotypes studied use different strategies for water status control, under drought stress conditions in a time-dependent manner.
ROS leads to changes in cellular structure and function causing potential damage to nucleic acids, to proteins and to lipids inhibiting their normal function (Foyer & Noctor Citation2005). ROS are well recognized for playing a dual role as both deleterious and beneficial species (protective or signaling factors). Their concentrations are dependent on delicate balance between their rates of production and their rates of removal by various antioxidants at the proper time and site (Gill & Tuteja Citation2010). The overproduction of ROS can be counteracted by a variety of defense mechanisms, including enzymatic antioxidant mechanisms, such as CAT, SOD, PPO and POD. The tolerance of plants to ROS in response to drought stress requires the adaptation of many complex and multifaceted processes as the enzymatic antioxidant system (Patade et al. Citation2011; Pyngrope et al. Citation2013; Chakhchar et al. Citation2015a). The significant increase in these enzymes activities has been shown in the argan tree under drought stress conditions, reflecting their role as an effective line of antioxidant defense against oxidative damage (Chakhchar et al. Citation2011). The activation of these enzymes seems correlated with the degree and intensity of stress. SOD and CAT activities were greater in Aoulouz and Aoulouz and Rabia, respectively, when compared with other ecotypes under drought stress (). Liu et al. (Citation2009) reported that drought stress increased the activities of antioxidants such as SOD and CAT. These both enzymes are the important components of protective systems. SOD has been considered to be the most potent enzymatic antioxidant involved in the tolerance process, providing the first line of defense against ROS (Sayfzadeh et al. Citation2011). SOD removes superoxide and subsequently decreases the potential risk posed by the hydroxyl radical formation via the metal-catalyzed Habere–Weiss-type reaction (Gill & Tuteja Citation2010). In the present study, the ecotypes differed in their SOD activity induced by drought stress. Thus, in terms of comparison between ecotypes, the high SOD activity was observed in inland ecotypes, suggesting intraspecific differences in protection levels against drought stress. Similar to our results, a significant increase has been noted in the SOD activity in O. europea under drought stress conditions (Ben Ahmed et al. Citation2009; Boughalleb & Mhamdi Citation2011). CAT is an extremely affective enzyme that speeds up the breakdown of hydrogen peroxide into water and oxygen (nearly six million molecules of H2O2 per minute). It decomposes H2O2 and presumably prevents accumulation of this toxic compound which was generated as a byproduct in the process of fatty acid degradation by peroxisomes (Gill & Tuteja Citation2010). At moderate and severe drought stress, we recorded in all ecotypes an increase in the CAT activity and a high constitutive activity of CAT was noted in Aoulouz and Rabia. Also, some authors have reported that the CAT activity significantly increased in olive plants under drought stress (Aganchich et al. Citation2007; Ben Ahmed et al. Citation2009; Boughalleb & Mhamdi Citation2011). Thus, higher SOD, CAT and POD activities were reported in two contrasting poplar species in response to drought stress (Yang et al. Citation2009).
According to our results, the activities of PPO and POD significantly increased in the leaves of water-stressed plants of A. spinosa during the experimental period () (Chakhchar et al. Citation2011). The variation of constitutive activities of POD and PPO is related to genotype differences. Plant PPOs catalyze the reduction of H2O2 to H2O and O2 and thus help plants minimize the negative effects of oxidative damage (Chaparzadeh et al. Citation2004). Indeed, POD enzyme plays a key role to prevent oxidative damage and to maintain cell membrane integrity in decreasing H2O2 content and eliminating MDA. Recent study has indicated that under drought stress, the enhanced activation of POD was also implied in the lignification process (Lee et al. Citation2007). Plant PPOs catalyze the o-hydroxylation of monophenols to o-diphenols as well as the oxidation of o-diphenols to quinones in the presence of oxygen (Rivero et al. Citation2001). Both the enzymes play a possible protective role with the other components in the defensive mechanisms and the changes in their activities could be an indicator when important endogenous changes. Similar results showed an increase in POD and PPO activities in olive plants under drought stress (Boughalleb & Mhamdi Citation2011).
ROS are chemically active molecules containing one or more unpaired electrons in atomic or molecular orbitals. This unpaired electron(s) generally gives a considerable degree of reactivity to the free radical. ROS can easily damage cellular components and alter membrane (Møller et al. Citation2007). Stress treatments caused a significant increase in MDA and H2O2 content, suggesting that water-stressed plants have confronted lipid peroxidation and cellular damage, which were higher in coastal ecotypes (). An increase in H2O2 accumulation and lipid peroxidation during drought stress was shown in our previous study on A. spinosa (Chakhchar et al. Citation2011) and has also been reported by Al-Ghamdi (Citation2009) and by Hameed et al. (Citation2013) in Triticum aestivum, by Boughalleb and Mhamdi (Citation2011) in Olea europaea and by Fini et al. (Citation2012) in Fraxinus ornus leaves. Its accumulation seemed to depend on the intensity of stress water treatments (Fini et al. Citation2012). The increase in MDA content clearly reflects membrane damage due to the lipid peroxidation of polyunsaturated fatty acids in the presence of ROS (Montillet et al. Citation2005; Farouk et al. Citation2013). The positive and significant correlation between H2O2 generation and MDA amount confirmed the hypothesis that H2O2 brings about lipid peroxidation, leading to membrane damage. Similar results have been obtained by Boughalleb and Mhamdi (Citation2011) in some olive cultivars. The lower values of MDA and H2O2 content were observed in the inland ecotypes, indicating that at a cellular level these ecotypes are better equipped with an efficient antioxidative defense system that offers more protection against oxidative damage. Most studies reported differential responses of antioxidant system and antioxidant metabolites in some plant species to drought stress (Gao et al. Citation2008; Basu et al. Citation2009; Pyngrope et al. Citation2013; Farouk & Abdul Qados Citation2013). Jiang et al. (Citation2013) studied the correlation of drought resistance in grass pea (Lathyrus sativus) with ROS scavenging. Those authors reported that the antioxidant enzymes showed obvious up-regulation at the transcriptional level in grass pea leaves. According to the significant interactions between watering regime, time and ecotype factors for H2O2 and MDA in A. spinosa, the occurrence of oxidative stress reflects a genetic variation of the antioxidant defense system efficiency, taking into account the period and the intensity of drought stress.
The stress treatments caused a significant increase in the activity of LOX in leaves of stressed plants compared to control (). The enhanced generation of ROS in response to drought stress might activate some specific isoenzymes of LOX in water-stressed plants (Ye et al. Citation2000). Sofo et al. (Citation2004) have suggested the existence of a strict relationship between the gradual increase of LOX activity and the progression of drought-stress conditions. Knowing that polyunsaturated fatty acids, the major component of cell membranes phospholipids is considered as the substrate of LOX, the enhancement of LOX activity has been noticed to damage cell membranes and destroy other cell components. LOX enzyme therefore plays a key role in cell membrane lipids composition and cell membrane integrity. Referring to our results, we observed the high activity of LOX in Lakhssas ecotype; this suggests that there is a quantitative difference in polyunsaturated fatty acids of cell membrane between the four ecotypes. Hypothetically, the existence of a relationship among LOX activity, lipid content and drought stress, according to our results, was supported by the study of Maalekuu et al. (Citation2006). These authors have suggested a close relationship between these traits.
The 2D scatterplot of discriminant space relative to two DFs of physiological and biochemical traits is presented in . This scatterplot shows a good separation among ecotypes of A. spinosa. The horizontal separation was characterized in the first DF. Thus, the first DF quantifies the degree to which all ecotypes differ in traits studied, which we suggest to be the result of differences in their tolerance under drought stress. Inland ecotypes (Lakhssas and Aoulouz) were mainly separated from the coastal ecotypes (Rabai and Admine) by both physiological traits: and gs which showed the most discriminating power, and higher PPO and SOD activity and lower H2O2 accumulation in terms of biochemical traits. Considering the second DF, Lakhssas was mainly distinguished from the other ecotypes by a lower Ψpd; and lower MDA content compared, especially, to coastal ecotypes. This suggests that the inland ecotypes have a highly effective antioxidant defense system.
In conclusion, we examined and compared the tolerance mechanisms of four contrasting ecotypes of A. spinosa in response to drought stress by adopting a multifactorial approach. A significant ecotype effect was observed for leaf water status, oxidative damage and antioxidative enzyme activity. The recorded changes in physiological and biochemical responses might be related to the effect of ecotypes, time, watering regime and their interactions. According to the discriminant factor analysis, the four ecotypes have been distinguished and separated mainly by the traits having the most discriminating power: Ψpd, gs, PPO, SOD and MDA. Both inland ecotypes seem to be more droughts tolerant and very promising for the regeneration of the Moroccan Arganeraie in arid and semi-arid areas. Future research on the drought adaptation degree of A. spinosa will be necessary to fully appreciate the agronomic performance of inland and coastal ecotypes.
Disclosure statement
No potential conflict of interest was reported by the authors.
Funding
This research was funded by the Academy Hassan II des Sciences et Techniques and the Moroccan Ministry (Ministère de l'Enseignement Supérieur, de la Recherche Scientifique et de la Formation des Cadres) (Morocco).
References
- Aebi H. 1984. Catalase in vitro. Meth Enzymol. 105:121–126.
- Al-Ghamdi AA. 2009. Evaluation of oxidative stress tolerance in two wheat (Triticum aestivum) cultivars in response to drought. Int J Agric Biol. 11:7–12.
- Aganchich B, Tahi H, Wahbi S, El Modafar C, Serraj R. 2007. Growth, water relations and antioxidant defence mechanisms of olive (Olea europaea L.) subjected to Partial Root Drying (PRD) and Regulated Deficit Irrigation (RDI). Plant Biosyst. 141:252–264.
- Axelrod B, Cheesbrough TM, Laakso S. 1981. Lipoxygenase from soybeans: EC 1.13.11.12 linoleate: oxygenoxidoreductase. Meth Enzymol. 71:441–451.
- Basu S, Roychoudhury A, Saha P, Sengupta DN. 2009. Differential antioxidative responses of Indica rice cultivars to drought stress. Plant Growth Regul. 60:51–59.
- Beauchamp C, Fridovich I. 1971. Superoxide dismutase: improved assays and applicable to acrylamide gels. Anal Biochem. 44:276–287.
- Ben Ahmed C, Ben Rouina B, Sensoy B, Boukhris M, Ben Abdallah F. 2009. Changes in gas exchange, proline accumulation and antioxidative enzyme activities in three olive cultivars under contrasting water availability regimes. Environ Exp Bot. 67:345–352.
- Boughalleb F, Mhamdi M. 2011. Possible involvement of proline and the antioxidant defense systems in the drought tolerance of three olive cultivars grown under increasing water deficit regimes. Agric J. 6:378–391.
- Bradford MM. 1976. A rapid and sensitive method for the quantitation of microgram quantities of protein utilising the principle of protein-dye binding. Anal Biochem. 72:248–254.
- Chakhchar A, Ferradous A, Lamaoui M, Wahbi S, El Modafar C. 2011. Changes in antioxidant enzymes activity and oxidative damage in four Argania spinosa ecotypes under water stress conditions. Nature Precedings. doi: 10.1038/npre.2011.6189.1.
- Chakhchar A, Lamaoui M, Wahbi S, Ferradous A, El Mousadik A, Ibnsouda-Koraichi S, Filali-Maltouf A, El Modafar C. 2015a. Differential drought tolerance of four contrasting Argania spinosa ecotypes assessed by enzymatic and non-enzymatic antioxidant. Int J Recent Sci Res. 6:3002–3009.
- Chakhchar A, Lamaoui M, Wahbi S, Ferradous A, El Mousadik A, Ibnsouda-Koraichi S, Filali-Maltouf A, El Modafar C. 2015b. Leaf water status, osmoregulation and secondary metabolism as a model for depicting drought tolerance in Argania spinosa. Acta Physiol Plant. 37:1–16.
- Chandra A, Dubey A. 2008. Evaluation of genus Cenchrus based on malondialdehyde, proline content, specific leaf area and carbon isotope discrimination for drought tolerance and divergence of species at DNA level. Acta Physiol Plant 30:53–61.
- Chaparzadeh N, D'Amico ML, Khavari-Nejad RA, Izzo R, Navari-Izzo F. 2004. Antioxidative responses of Calendula officinalis under salinity conditions. Plant Physiol Biochem. 42:695–701.
- Chaussod R, Adlouni A, Christon R. 2005. The argan tree and argan oil in Morocco: towards a deep change in a traditional agroforestry system. Economic and scientific challenges. Cah Agric. 14:351–356.
- Díaz-Barradas MC, Zunzunegui M, Ain-Lhout F, Jáuregui J, Boutaleb S, Álvarez-Cansino L, Esquivias MP. 2010. Seasonal physiological responses of Argania spinosa tree from Mediterranean to semi-arid climate. Plant Soil. 337:217–231.
- Farouk S, Abdul Qados AMS. 2013. Osmotic adjustment and yield of cowpea in response to drought stress and chitosan. Indian J Appl Res. 3(10) doi:10.15373/2249555X.
- Farouk S, Ramadan AA, Showler AT. 2013. Chitosan effects on physiochemical indicators of drought-induced leaf stress in cowpea. Plant Knowl J. 2:135–144.
- Feussner I, Kindl H. 1994. Particulate and soluble lipoxygenase isozymes. Comparison of molecular and enzymatic properties. Planta. 194:22–28.
- Fini A, Guidi L, Ferrini F, Brunetti C, Di Ferdinando M, Biricolti S, Pollastri S, Calamai L, Tattini M. 2012. Drought stress has contrasting effects on antioxidant enzymes activity and phenylpropanoid biosynthesis in Fraxinus ornus leaves: an excess light stress affair?. J Plant Physiol. 169:929–939.
- Foyer CH, Noctor G. 2005. Redox homeostasis and antioxidant signaling: a metabolic interface between stress perception and physiological responses. Plant Cell. 17:1866–1875.
- Gao J, Xiao Q, Ding L, Chen M, Yin L, Li J, Zhou S, He G. 2008. Differential responses of lipid peroxidation and antioxidants in Alternanthera philoxeroides and Oryza sativa subjected to drought stress. Plant Growth Regul. 56:89–95.
- Garnier E, Shipley B, Roumet C, Laurent G. 2001. A standardized protocol for the determination of specific leaf area and leaf dry matter content. Funct Ecol. 15:688–695.
- Gill SS, Tuteja N. 2010. Reactive oxygen species and antioxidant machinery in abiotic stress tolerance in crop plants. Plant Physiol Biochem. 48:909–930.
- Gomes MMA, Lagôa AMMA, Medina CL, Machado EC, Machado MA. 2004. Interactions between leaf water potential, stomatal conductance and abscisic acid content of orange trees submitted to drought stress. Braz J Plant Physiol. 16:155–161.
- Hameed A, Goher M, Iqbal N. 2013. Drought induced programmed cell death and associated changes in antioxidants, proteases, and lipid peroxidation in wheat leaves. Biol Plant. 57:370–374.
- Hernandez JA, Almansa MS. 2002. Short-term effects of salt stress on antioxidant systems and leaf water relations of pea leaves. Physiol Plant. 115:251–257.
- Jiang J, Su M, Chen Y, Gao N, Jiao C, Sun Z, Li F, Wang C. 2013. Correlation of drought resistance in grass pea (Lathyrus sativus) with reactive oxygen species scavenging and osmotic adjustment. Biologia. 68:231–240.
- Lee BR, Kim KY, Jung WJ, Avice JC, Ourry A, Kim TH. 2007. Peroxidases and lignification in relation to the intensity of water-deficit stress in white clover (Trifolium repens L.). J Exp Bot. 58:1271–1279.
- Liu ZJ, Zhang XL, Bai JG, Suo BX, Xuand PL, Wang L. 2009. Exogenous paraquat changes antioxidant enzyme activities and lipid peroxidation in drought-stressed cucumber leaves. Sci Hort. 121:138–143.
- Maalekuu K, Elkind Y, Leikin-Frenkel A, Lurie S, Fallik E. 2006. The relationship between water loss, lipid content, membrane integrity and LOX activity in ripe pepper fruit after storage. Postharvest Biol Technol. 42:248–255.
- Møller IM, Jensen PE, Hansson A. 2007. Oxidative Modifications to Cellular Components in Plants. Annu Rev Plant Biol. 58:459–481.
- Montillet JL, Chamnongpol S, Rusterucci C, Dat J, Van de Cotte B, Agnel JP, Battesti C, Inzé D, Van Breusegem F, Triantaphylidès C. 2005. Fatty acid hydroperoxides and H2O2 in the execution of hypersensitive cell death in tobacco leaves. Plant Physiol. 138:1516–1526.
- Moore BM, FIurkey WH. 1990. Sodium dodecyl sulfate activation of a plant polyphenol oxidase. J Biol Chem. 265:4982–4988.
- Msanda F, El Aboudi A, Peltier JP. 2005. Biodiversity and biogeography of Moroccan argan tree communities. Cah Agri. 14:357–364.
- Nag S, Saha K, Choudhuri A. 2000. A rapid and sensitive assay method for measuring amine oxidase based on hydrogen peroxide-titanium complex formation. Plant Sci. 157:157–163.
- Nogués S, Baker NR. 2000. Effects of drought on photosynthesis in Mediterranean plants grown under enhanced UV-B radiation. J Exp Bot. 51:1309–1317.
- Patade VY, Bhargava S, Suprasanna P. 2011. Salt and drought tolerance of sugarcane under iso-osmotic salt and water stress: growth, osmolytes accumulation, and antioxidant defense. J Plant Interact. 6:275–282.
- Pita P, Cañas I, Soria F, Ruiz F, Toval G. 2005. Use of physiological traits in tree breeding for improved yield in drought-prone environments. The case of Eucalyptus globulus. The case of Eucalyptus globulus. Invest Agrar: Sist Recur For. 14:383–393.
- Pyngrope S, Bhoomika K, Dubey RS. 2013. Oxidative stress, protein carbonylation, proteolysis and antioxidative defense system as a model for depicting water deficit tolerance in Indica rice seedlings. Plant Growth Regul. 69:149–165.
- Rivero RM, Ruiz JM, García PC, López-Lefebre LR, Sánchez E, Romero L. 2001. Resistance to cold and heat stress: accumulation of phenolic compounds in tomato and watermelon plants. Plant Sci. 160:315–321.
- Sayfzadeh S, Habibi D, Taleghani DF, Kashani A, Vazan S, Qaen SHS, Khodae AH, Boojar MMA, Rashedi M. 2011. Response of antioxidant enzyme activities and root yield in sugar beet to drought stress. Int J Agric Biol. 13:357–362.
- Smirnoff N. 1993. The role of active oxygen in the response of plants to water deficit and desiccation. New Phytol. 125:27–58.
- Sofo A, Dichio B, Xiloyannis C, Masia A. 2004. Lipoxygenase activity and proline accumulation in leaves and roots of olive trees in response to drought stress. Physiol Plant. 121:58–65.
- Tardieu F, Lafarge T, Simonneau T. 1996. Stomatal control by fed or endogenous xylem ABA in sunflower: interpretation of correlations between leaf water potential and stomatal conductance in anisohydric species. Plant Cell Environ. 19:75–84.
- Tardieu F, Simonneau T. 1998. Variability among species of stomatal control under fluctuating soil water status and evaporative demand: modelling isohydric and anisohydric behaviours. J Exp Bot. 49:419–432.
- Teulat B, Monneveux P, Wery J, Borries C, Sourys I, Charrier A, This D. 1997. Relationships between relative water content and growth parameters under water stress in barley: a QTL study. New Phytol. 137:99–107.
- Wahbi S, Wakrim R, Aganchich B, Tahi H, Serraj R. 2005. Effects of partial root zone drying (PRD) on adult olive tree (Olea europaea) in field conditions under arid climate I. Physiological and agronomic responses. Agric Ecosyst Environ. 106:289–301.
- Yang F, Xu X, Xiao X, Li C. 2009. Responses to drought stress in two poplar species originating from different altitudes. Biol Plant. 53:511–516.
- Ye Z, Rodriguez R,Tran A, Hoang H, de los Santos D, Brown S, Vellanoweth L. 2000. The developmental transition to flowering repress ascorbate peroxidase activity and induces enzymatic lipid peroxidation in leaf tissue in Arabidopsis thaliana. Plant Sci. 158:115–127.
- Zhang S, Lu S, Xu X, Korpelainen H, Li C. 2009. Changes in antioxidant enzyme activities and isozyme profiles in leaves of male and female Populus cathayana infected with Melampsora larici-populina. Tree Physiol. 30:116–128.