Abstract
The allelopathic effect of A. altissima and the nitrogen fixing ability of R. pseudoacacia make the interaction between these two species important to community dynamics. A replacement series greenhouse experiment was used to investigate the type of interaction between seedlings of A. altissima and R. pseudoacacia at high and low soil nutrition states. Also, seeds of A. altissima from its native (China) and invasive ranges (USA) were used to compare the effect of the different seed sources on the interaction with R. pseudoacacia. Robinia pseudoacacia was the better competitor. The presence of A. altissima significantly inhibited nodulation of R. pseudoacacia roots. In summary, in low nutrient early succession sites, seedlings of R. pseudoacacia and A. altissima will coexist and R. pseudoacacia will be the better competitor. However, A. altissima can increase its competitive ability by inhibiting nitrogen fixation by R. pseudoacacia. Differences between the competitive ability of the Chinese and US seed lots of A. altissima did not support the rapid evolution theory for invasive species success.
1. Introduction
Many mechanisms can contribute to the impact of invasive species on native flora, but competition is often central (Wyckoff & Webb Citation1996; Gentle & Duggin Citation1997; Levine et al. Citation2003; Besaw et al. Citation2011; Vilà et al. Citation2011; Dostál et al. Citation2013; Ricciardi et al. Citation2013). Moreover, invasive and native species that have similar habitat preferences are most likely to interact during early succession because of their similar likelihood to germinate early following disturbance. Early-successional, native species may compete with the invader for the same available resources, facilitate the invader by creating a favorable environment, both compete and facilitate an invader under different environmental conditions, or neither compete with or facilitate an invader. Invasive species are a focus of research because they may have negative impacts on ecosystem processes compared with native species (Barney et al. Citation2013). The interaction between exotic invasive species and early succession fast-growing native species may regulate the success of the invader and such interactions first occur at the seedling level. Therefore, understanding the competitive interaction between exotic invasive and native species seedlings that have similar functional traits, yet influence communities and ecosystems differently, is an important aspect of invasive ecology research.
The overall research program reported here concerns the mechanisms of interaction between one important exotic invasive species Ailanthus altissima (Mill.) Swingle (Simaroubaceae) and a functionally similar species, Robinia pseudoacacia L. (Fabaceae), native to the mid-latitude Appalachian mountains. These two species were selected because they have comparable ecological traits. Both species are abundant in early-successional temperate forests, and both can survive and thrive in relatively nutrient-poor soils (Hu Citation1979; Hoshovsky Citation1988; Vlachodimos et al. Citation2013). Both species grow rapidly and reproduce by vegetative cloning and prolific seed production. However, the two species have an important difference that causes divergent impacts on forest succession and ecosystem processes. Ailanthus altissima is recognized as an invasive species with a novel weapon (allelochemicals) because of its negative effect on native species germination and growth, which can reduce plant diversity and forest succession after disturbance (Mergen Citation1959; Heisey Citation1990; Heisey Citation1996; Castro-Díez et al. Citation2008; Gómez-Aparicio & Canham Citation2008). Robinia pseudoacacia is one of the most important nitrogen-fixing trees in the eastern forests of the USA, which facilitates forest succession (Boring & Swank Citation1984b). Also, R. pseudoacacia is used for restoring vegetation on post-disturbance sites due to its ability to increase soil nitrogen content (Von Holle et al. Citation2006; Vlachodimos et al. Citation2013). Thus, the result of competition between these two species may have a significant effect on native community diversity and succession. One possibility is that allelochemicals produced by A. altissima may reduce the ability of root formation and nodulation of R. pseudoacacia (Halsall et al. Citation1995), hence changing nitrogen cycling, succession and community structure in eastern forests of the USA. Another possibility is that R. pseudoacacia facilitates the growth of A. altissima by increasing soil nitrogen levels around the A. altissima roots through leakage from nearby nitrogen fixation. Therefore, understanding the result of competition or facilitation between these two species is important for understanding the relationship between invasive–native species interactions and its influence on community dynamics.
There have been many theories proposed about why an invasive species is more successful in its invaded range than its native range, such as being a superior competitor (Tilman Citation1982; Shea & Chesson Citation2002), escaping from natural enemies (Keane & Crawley Citation2002), having novel weapons (Callaway & Ridenour Citation2004) and inducing invasive meltdown (Simberloff & Von Holle Citation1999). One aspect of that theoretical discussion is the effect of lower genetic diversity for the invasive species in the invaded habitat compared with its native habitat. This can result from establishment of only a small number of genotypes introduced in the new habitat that initiated the invasion (Lee Citation2000; Tsutsui et al. Citation2000). Particularly vigorous genotypes are usually selected for use in horticultural introductions. Thus, genotypes with high fitness dominate the introduced population more so than the native populations. Also, rapid evolution of highly adaptive traits could have occurred early in the introduction of A. altissima to the USA (Prentis et al. Citation2008). Therefore, it is logical to posit that the invasive species populations in the invaded ecosystem may be selected to be a more competitive group than the populations of invasive species in their native ecosystem.
The overall goal of this study was to investigate competition and facilitation between R. pseudoacacia and A. altissima seedlings in relatively low nutrient environments, and to assess if a difference between the competitive ability of A. altissima genotypes growing in the USA vs. those from its native China could be determined. We focused on both belowground and aboveground processes in a greenhouse setting, which facilitated harvesting the entire root system, counting and collecting nodules from R. pseudoacacia seedlings, and manipulating nutrient conditions.
A full-factorial substitutive design greenhouse experiment was used to address the following questions: (1) Does A. altissima produce the most belowground and aboveground biomass at the seedling stage? (2) Does A. altissima whole plant biomass and allocation to shoots increase significantly more from low to high nutrient levels than that for R. pseudoacacia seedlings? (3) Does the presence of A. altissima change the whole plant biomass and allocation patterns of R. pseudoacacia? (4) Does the presence of A. altissima inhibit nodule number per root mass of R. pseudoacacia? (5) Is the competitive ability of A. altissima seedlings from the invaded range (USA) greater than that of A. altissima seedlings from its native region (China)? Answers to these questions will provide substantial insight to the possible mechanisms of interactions between these species in the early-successional forest.
2. Materials and methods
2.1. Seed germination
The A. altissima seeds from the invaded ecosystems were collected from Mineral, Hampshire, Hardy, and Grant Counties of West Virginia, USA from multiple trees along highways in 2009 and 2010. The A. altissima seeds from its native range were bought from Shuang Ye Seeds Wholesale Co., Ltd. in 2011, and were collected in 2009 from multiple trees in JiangSu province, China. Both seed lots were stored at 4°C until being used in January 2013. The samaras were cut off, and about 500 seeds of A. altissima from each seed lot were soaked in 100 mL 500 ppm gibberellic acid (GA) solution in a dark incubator at 40°C for 24 h to break dormancy. We used the GA method to break dormancy instead of the traditional warm water incubation because we found very low germination rate in preliminary attempts with the traditional technique. Robinia pseudoacacia seeds purchased from Sheffield's Seed Co. Inc. (Locke, New York, USA) were collected from multiple trees in Kentucky, USA. The R. pseudoacacia seeds were stratified at 4°C for 60 days, soaked in water and put in a dark incubator at 40°C for 12 h. Following incubations, seeds of both species were sown in germination flats with Metro Mix® in the BIOL/VBI plant growth service center at Virginia Tech. Air temperature in the BIOL/VBI starting room used for establishing seedlings ranged from 22°C to 24°C during the experiment, light intensity (photosynthetic photon flux density) peaked at 1200–1500 μmol m−2 s−1 and relative humidity ranged from 75% to 80% on a daily basis.
2.2. Experimental design
After 3 weeks growing in flats in the greenhouse, six seedlings were transplanted into each 8 L pot (approximately 25 cm in diameter, 35 cm in height), which contained washed masonry sand mixed with 10 g of soil collected from under planted R. pseudoacacia stands at Blandy Experimental Farm, Boyce, Virginia, USA to stimulate nodulation. There were 2 levels of nutrient (defined as ‘nutrient’ treatments hereafter), 5 different species combinations (defined as ‘proportion’ treatments hereafter) and 2 sources of A. altissima seed (defined as ‘seed source’ treatments hereafter), with 8 replicates per treatment, for a total of 160 pots in the experiment. The planting density was held constant at six plants per pot. Mixtures consisted of the following species proportions: (1) monoculture of A. altissima (6A), (2) monoculture of R. pseudoacacia (6R), (3) two A. altissima and four R. pseudoacacia (2A4R), (4) three A. altissima and three R. pseudoacacia (3A3R) and (5) four A. altissima and two R. pseudoacacia (4A2R). Randomly selected similar sized seedlings from each species were planted into pots based on our experimental design. Air temperature in the BIOL/VBI plant growth bay used for this study ranged from 22°C to 28°C during the experiment, light intensity (photosynthetic photon flux density) peaked at 1600–1800 μmol m−2 s−1 and relative humidity ranged from 65% to 80% on a daily basis.
After transplantation, nutrient treatments were randomly assigned to each pot. Either 0.936 or 3.744 g (i.e. 39.5 and 158 kg N ha−1 yr−1) of Osmocote® 14-14-14 slow-release fertilizer was added to each pot to create the low and high nutrient treatments, respectively. These two nutrient treatments were selected to represent low soil nutrient conditions in a natural temperate forest (Finzi et al. Citation1998) and the upper limit of fertilizer added to agriculture land (158 kg N ha−1 yr−1). Fertilizer was reapplied at the same rates after 4 months because the slow-release fertilizer has a predicted lifespan of 5–6 months at 21°C. After all treatments were established, pots were completely randomized on two greenhouse benches and were rotated along the benches every month to minimize the effect of spatial variation in greenhouse microclimate.
Due to the difference in germination and growth rate, the replicates using Chinese A. altissima seedlings were transplanted about 40 days earlier than the replicates using US A. altissima seedlings. Hence, pots with the two different seed sources were harvested at different times, to keep the same number of growing days. Day length varied between 13 and 14.74 h during the entire length of both experimental growth periods. The plants were harvested after about 5 months, when the seedlings reached the maximum capacity of the pots without significant root binding. The aboveground parts were harvested first on the same day. Height, from ground level to top of the main axis, and basal diameter of each plant were measured. Little to no branching occurred during the growth period. Stems and leaves were separated and oven-dried at 60°C for approximately 4 days to insure constant dry weight. Roots were harvested during a 30-day period after shoot collection, and the pots with roots waiting to be harvested were kept unwatered in the greenhouse to make sure no additional growth occurred. Roots from each of the six plants in one pot were separated carefully, and all sand was washed off the roots. The extra roots, which were broken during the separation process and left in sand were extracted and the sand was carefully washed off the root biomass for each pot. The number of nodules on R. pseudoacacia root systems was determined. All nodules greater than 1 mm in size were picked off and dried to constant weight. All other root tissues were also oven-dried to constant weight. We used the number of nodules (number of infection sites) as a proxy for the amount of nitrogen fixed by the plant based on a positive correlation between nodule number and the isotopic signature of nitrogen (Δ15N) for R. pseudoacacia growing in sand fertilized with Hoaglands solution – N (Supplementary ).
2.3. Data analysis
During the harvest, it was unavoidable to have broken fine roots left in each pot. In this experiment, the total amount of extra roots left in the sand after separating the root systems of the six plants averaged 28% of the total root biomass in each pot. In a previous study, we found it was impossible to distinguish these two species' fine roots based on their morphological characteristics (unpublished data). Since these extra roots could not be separated between the six original plants, we assigned a proportion of the extra root biomass to each of the six plants based on the relative harvested root biomass of each plant in the pot. For example, if a plant had 33% of the total extracted root mass in a pot, then 33% of the extra roots sieved and washed from the sand was added to that plants’ root mass.
Aboveground biomass (leaf plus shoot), leaf biomass, belowground biomass, total biomass and root/shoot (i.e. belowground biomass/aboveground biomass) ratio were calculated, and the correlations among aboveground, belowground and total biomass were evaluated. Aboveground biomass and belowground biomass were found to be autocorrelated (r = .76), and they were both highly correlated with total biomass (r = .95 and .92). Therefore, three-way analysis of variance (ANOVA) was only presented for total biomass, which reflected plant growth over the experimental period, leaf biomass, which reflects investment into carbon gain and photosynthesis, and root/shoot ratio, which reflects the relative investment in nutrient accumulation. Three-way ANOVAs for each species were performed separately, to evaluate the main effects of nutrient level, species proportion, seed source, the interactions between each pair of these three factors and the three-way interaction. Subsample error was considered into this ANOVA, because there was more than one plant (subsample) in each pot (replicate). All the factors were treated as fixed effects. If a significant ANOVA result was identified, multiple-comparisons between all possible pairs of treatments in the particular effect group were evaluated using Tukey's honest significant difference.
Regressions were used to test the relationships between height or basal diameter and aboveground or total biomass at the end of the experimental growth period. Differences in the regression of total biomass vs. height for R. pseudoacacia competing with A. altissima seedlings from the two seed sources was evaluated with analysis of covariance.
To compare our results with a prior study (Call & Nilsen Citation2005), the relative yield (RY) was calculated to determine if the species were sharing resources or competing with each other for resources (Fowler Citation1982; Williams & McCarthy Citation2001). These indices were calculated separately based on total biomass of each species. The equations of calculating RY for the two species are
where YA = yield (dry biomass) of A. altissima in monoculture, YR = yield of R. pseudoacacia in monoculture, YAR = yield of A. altissima in the presence of R. pseudoacacia, YRA = yield of R. pseudoacacia in the presence of A. altissima, pA = proportion of A. altissima sown in one pot, pR = proportion of R. pseudoacacia sown in same pot, and pA + pR = 1.
A RY less than 1 indicates that the species is experiencing competition from the other species. A RY more than 1 indicates that the species is experiencing more competition from itself than from the other species.
A three-way ANOVA was applied to the calculated RYA and RYR using total biomass to compare the difference of competition intensity under different treatment combinations. There were only three levels in the proportion treatment for RYA and RYR, because both indices could only be calculated for mixtures. There was no subsample error in the RYA and RYR ANOVA, because both indices can only be calculated once for each pot.
To determine whether A. altissima affected nodulation of R. pseudoacacia, the relationship between nodule number and nodule weight under different nutrient levels was assessed by general linear regression. Poisson regressions of the numbers of nodules per root mass were fitted for R. pseudoacacia plants under high and low nutrient levels. Also, the average nodule number per R. pseudoacacia root mass was used as the response variable, and the relationships with number of neighboring A. altissima and A. altissima seed source were evaluated to explore the possibility of a negative effect of A. altissima roots on R. pseudoacacia nodulation. A three-way ANOVA was used to detect the effect of seed source, nutrient level and species proportion on nodule number per root weight. Also, a three-way ANOVA was used for nodule weight, but we suggest that nodule number (number of infection points) is a better representation of the amount of nitrogen fixation by R. pseudoacacia, because a previous study showed nodule nitrogen-fixing activity is the highest for mid-size nodules (1–2 mm), which are the most frequent size of nodules. Moreover, the nitrogen fixation activity does not increase as size of the nodules increases for nodules that are larger than the mid-size category (Tajima et al. Citation2007). Thus, increased size of the nodule does not represent effectively the amount of nitrogen fixed by the nodule.
3. Results
In general, R. pseudoacacia seedlings had a significantly higher total biomass (p < .0001) than A. altissima seedlings. The average total dry biomass per plant for R. pseudoacacia in all the treatments combined was almost seven times the total dry biomass per plant of A. altissima (). Ailanthus altissima seedlings produced more root biomass compared with shoot biomass because, in most treatment groups, root/shoot ratio >1 ( and ). In contrast, seedlings of R. pseudoacacia produced similar amounts of aboveground and belowground biomass because their root/shoot ratios were approximately equal to 1.
The regressions of basal area with biomass (dependent variable) had a very low R2 and are not presented for either species because they were insignificant. When grown with A. altissima seedlings from the Chinese seed lot the regression of height with aboveground (R2 = .37) and total (R2 = .35) for R. pseudoacacia were both significant at the p < .0001 level. Similarly, when grown with A. altissima seedlings from the US seed lot the regression of height with aboveground (R2 = .77) and total (R2 = .76) for R. pseudoacacia were both significant at the p < .0001 level. Although the slopes were steeper for the regressions calculated for R. pseudoacacia when growing with A. altissima seedlings from the Chinese seed source, those differences were not significant.
3.1. Effect of nutrient level
The effect of nutrient level on average total biomass per plant at high nutrient level was significantly greater than that at low nutrient level for both species (–). Total biomass of A. altissima increased by 1.23 times from low to high nutrients while that of R. pseudoacacia increased by 1.141 times. Leaf biomass increased significantly with increase of nutrient level for both species (–) and A. altissima leaf biomass increased by 1.38 times while that of R. pseudoacacia only increased by 0.92 times. Root/shoot ratio did not decrease significantly for either species from low to high nutrients (–). The only significant interaction for A. altissima between the nutrient effect and other effects was the interaction of nutrient with source for leaf biomass (). This interaction means that the effect of nutrient on leaf biomass was different for seedlings grown from the two seed sources. There was a significant interaction between the nutrient and proportion effects on total biomass and leaf biomass for R. pseudoacacia (). This interaction indicates that the increase in total biomass and leaf biomass induced by high nutrition varied among mixtures with A. altissima. Also, there was a significant interaction between nutrient level and A. altissima seed source on leaf biomass (). This interaction means that the increase in leaf biomass in high nutrient conditions is different when different sources of A. altissima seedlings are in the experiment. Although there was no significant effect of nutrients on root/shoot ratio, there was a significant interaction between nutrient and A. altissima seed source for root/shoot ratio (Section 3.3).
Table 1. Mean ± 1 standard error of total biomass, leaf biomass and root/shoot ratio per plant of Ailanthus altissima and Robinia pseudoacacia seedlings with different seed source, nutrient and proportion treatments.
Table 2. Results from three-way ANOVAs of seedling growth metrics for Ailanthus altissima growing in a full-factorial substitutive design competition experiment with Robinia pseudoacacia in a greenhouse environment.
Table 3. Results from three-way ANOVAs of seedling growth metrics for Robinia pseudoacacia growing in a full-factorial substitutive design competition experiment with Ailanthus altissima in a greenhouse environment.
3.2. Effect of proportional mixtures
Ailanthus altissima seedlings in monoculture had a significantly greater total biomass than those grown in mixed pots (). However, the R. pseudoacacia seedlings in monoculture had a significantly lower average individual biomass than those in mixed culture. The effect of proportion was significant for both species ( and ). In general, the bigger the proportion of R. pseudoacacia seedlings in a pot was, the lower the average total biomass of A. altissima seedlings was in the pot. Although, the effect of proportion was significant (), only the A. altissima seedlings in monoculture had a significantly greater leaf biomass than those grown in 3A3R treatment (). In contrast, the total average biomass per plant of R. pseudoacacia increased significantly as the proportion of A. altissima increased in the pot ( and ). In the high nutrient treatment, average biomass of R. pseudoacacia seedlings decreased more rapidly as the proportion of A. altissima decreased, which resulted in a significant interaction between nutrient and proportion (). The results for leaf biomass were similar among proportions to the results for total biomass for both species (). The monoculture group of A. altissima had significantly higher root/shoot ratio than the 4A2R and 3A3R groups ( and ), which resulted in a significant effect of proportion (). There was no significant effect of proportion on R. pseudoacacia root/shoot ratio ( and ).
Figure 1. Aboveground, belowground and total biomass of Ailanthus altissima in a full-factorial substitutive design experiment with Robinia pseudoacacia at constant density (six plants per 8 L pot). The solid bars and error bars represent the average biomass per A. altissima plant and the standard error within each treatment group. The upper graph represents the results from an experiment using A. altissima seedlings from a Chinese seed lot, and the lower graph represents A. altissima seedlings grown from a West Virginia, US seed lot. Two nutrient levels (low and high) were used for the experiment, along with the four different proportions of A. altissima and R. pseudoacacia. (e.g. 2A4R = two A. altissima and four R. pseudoacacia seedlings; 3A3R = three A. altissima and three R. pseudoacacia seedlings).
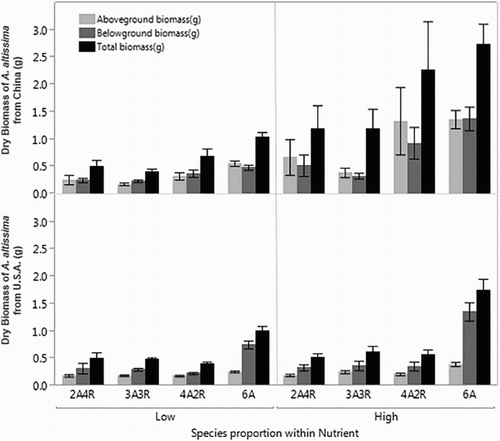
3.3. Effect of A. altissima seed source
The average total biomass and leaf biomass of Chinese A. altissima seedlings was significantly greater than that of seedlings from the US A. altissima seed lot ( and ). Also, in the germination stage, the Chinese seed lot of A. altissima had a much faster germination and higher germination rate than those from US seed lot, indicating a faster growth rate of Chinese A. altissima (personal observation). There was no significant difference in total biomass of R. pseudoacacia seedlings when grown with either of the two seed sources of A. altissima ( and ). However, R. pseudoacacia seedlings produced significantly less leaf biomass when mixed with the A. altissima seedlings from the US seed lot (), which resulted in a significant interaction between source and proportion (). The significant interaction between seed source and nutrient on leaf biomass was likely due to R. pseudoacacia producing greater leaf biomass in the high nutrient level than in the low nutrient level when grown with Chinese A. altissima, but less leaf biomass in the high nutrient level than in the low nutrient level when grown with US A. altissima.
The root/shoot ratio of A. altissima seedlings from the US seed lot was significantly higher than that of the seedlings from the Chinese seed lot ( and ). Moreover, there was a significant interaction with the proportion of R. pseudoacacia in the mixture ( and ). The interaction was significant because seedlings of A. altissima from the US seed lot had the highest root/shoot ratio in monoculture compared with those grown in mixtures with R. pseudoacacia (). The root/shoot ratio of R. pseudoacacia seedlings was significantly greater when R. pseudoacacia seedlings were grown with A. altissima seedlings from the US seed lot than when grown with A. altissima seedlings from the Chinese seed lot ( and ). When grown with Chinese A. altissima seedlings, R. pseudoacacia produced more root biomass in the high nutrient soil than in the low nutrient treatment; but this pattern was reversed for R. pseudoacacia grown with A. altissima seedlings from the US seed lot ( and ).
3.4. Intraspecific and interspecific competition
RY results for A. altissima (RYA) were mostly less than 1 (11 out of 12 values), indicating interspecific competitive interference from neighboring R. pseudoacacia seedlings was stronger than the intraspecific competition (). RYA was significantly different between nutrient levels (), and A. altissima seedlings in the lower nutrient treatment had lower RYA, indicating relatively stronger competition from R. pseudoacacia than from itself (). Also, the RYA was significantly different between the two seed sources (), and neighboring R. pseudoacacia seedlings had stronger negative impact on A. altissima seedlings from the US seed lot than those from the Chinese seed lot ( and ). There was a significant two-way interaction between seed source and nutrient (), because the increase in RYA from the low nutrient level to the high nutrient level for Chinese A. altissima was greater than that of US A. altissima ().
Figure 2. Aboveground, belowground and total biomass of Robinia pseudoacacia seedlings in a full-factorial substitutive design experiment with Ailanthus altissima at a constant density (six plants per 8 L pot). The solid bars and error bars represent the average biomass per R. pseudoacacia plant and the standard error within each treatment group. The upper graph represents the results from an experiment using A. altissima seedlings from a Chinese seed lot, and the lower graph represents using A. altissima seedlings grown from a West Virginia, US seed lot. Two nutrient levels were used for the experiment, along with four different proportions of R. pseudoacacia and A. altissima seedlings. (e.g. 2R4A = two R. pseudoacacia and four A. altissima seedlings; 3R3A = three R. pseudoacacia and three A. altissima seedlings).
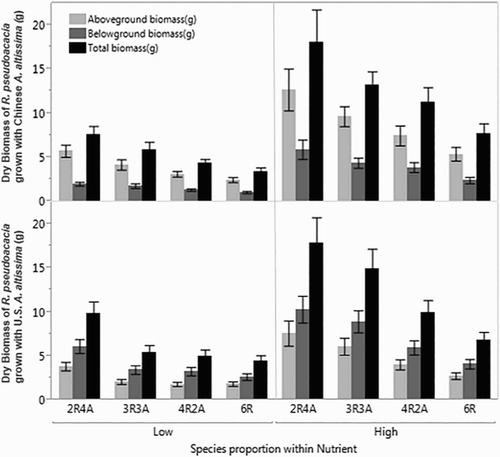
Table 4. RY for Ailanthus altissima (RYA) and Robinia pseudoacacia (RYR) in a full-factorial substitutive design experiment at a fixed density of six seedlings per 8 L pot.
Table 5. Results of a three-way ANOVAs on RY for Ailanthus altissima (RYA) and Robinia pseudoacacia (RYR) based on the final, total plant dry weight in a full-factorial substitutive design experiment at a fixed density of six seedlings per 8 L pot.
RY results for R. pseudoacacia seedlings (RYR) were mostly larger than 1 (9 out of 12 values), indicating more intraspecific competition than the negative effect from neighboring A. altissima seedlings (). Both nutrient and proportion treatments had a strongly significant effect, but not the seed source (). The high nutrient level group had a larger RYR than the low nutrient level (). The greater the number of A. altissima seedlings in the pot, the larger was the RYR and the stronger was intraspecific competition ( and ).
3.5. Nodulation of Robinia pseudoacacia
There was a positive linear relationship between nodule number and nodule weight, and this relationship was not statistically different for the two nutrient levels (p = .124). Therefore, in the following analysis of nodulation among different treatments, only the results using nodule number will be shown.
We found that nodule number was strongly positively related to root biomass (R2 = .42, p < .0001). There was no significant difference for this regression relationship between the two nutrient treatments (p = .19).
The average total numbers of nodules per plant in the high and low nutrient treatments were 81.6 ± 3.6 and 50.6 ± 3.6, respectively, and these values were significantly different (p = .03). Robinia pseudoacacia produced significantly more nodules per unit root mass at the low nutrient level (34.36 ± 2.01 g−1) than that at the high nutrient level (26.35 ± 2.04 g−1, p = .05). When grown with A. altissima, the number of nodules per root mass of R. pseudoacacia seedling was significantly lower (27.12 ± 1.80 g−1) than that of R. pseudoacacia seedlings in monoculture (35.31 ± 2.36 g−1, p = .005). Robinia pseudoacacia seedlings grown with A. altissima seedlings from the US seed lot had significantly fewer nodules per root weight (18.38 ± 0.87 g−1), compared with R. pseudoacacia seedlings grown with A. altissima seedlings from the Chinese seed lot (42.51 ± 2.53 g−1, p < .0001). When total nodule number was evaluated instead of nodule number per unit root mass, the effect of A. altissima seed lot became insignificant, because R. pseudoacacia seedlings grown with A. altissima seedlings from the US seed lot had larger root systems.
4. Discussion
4.1. Ailanthus altissima did not influence growth patterns of R. pseudoacacia
In response to our first question, we found that A. altissima seedlings did not inhibit the growth and allocation patterns of R. pseudoacacia seedlings. Robinia pseudoacacia grew much faster and produced more above and belowground biomass than A. altissima. As a result, intraspecific competition was more important for R. pseudoacacia growth than interspecific competition with A. altissima. Thus, in this study, R. pseudoacacia was the clear winner in all the environmental conditions used. This result contradicts previous research (Call & Nilsen Citation2005), in which the invasive species A. altissima was the winner in competition with R. pseudoacacia when planted in a higher nutrient level than is typically found under natural conditions. In both nutrient levels of our study, R. pseudoacacia formed nodules. In contrast, R. pseudoacacia seedlings in the experiment of Call and Nilsen (Citation2005) did not form nodules because nutrients were so high. The presence of nodules in this study suggests that these results are more applicable to early succession sites in the Mid-Appalachian regions.
4.2. Soil nutrition affected A. altissima growth more than R. pseudoacacia
Biomass indices for both species were very responsive to the difference in nutrient levels used in this study. Moreover, the relative effects of increasing nutrient availability caused a greater increase in biomass for A. altissima. However, the significant interactions between nutrient and proportion suggest that the interaction of species is altered in response to nutrient levels. Our second question related to whether R. pseudoacacia would be more competitive when soil nutrients are limiting due to its ability to fix nitrogen, while A. altissima would have a competitive edge when the nutrient levels are relatively high, as in Call and Nilsen (Citation2005). We found that in lower nutrient conditions, R. pseudoacacia was the stronger competitor (smaller RYA in low nutrient treatment). In addition, RYR was lower under the lowest nutrient condition, which indicated weaker intraspecific competition. Thus, when nutrients are relatively scarce, R. pseudoacacia is an effective competitor with A. altissima and also competes less with itself. In fact, the ability to tolerate a low nitrogen environment is essential to the strong competitive ability of R. pseudoacacia (Boring & Swank Citation1984a; Gioria & Osborne Citation2014), and is a main reason why R. pseudoacacia is considered to be a good invasive species itself outside of its native range in the eastern USA (Castro-Díez et al. Citation2008).
The comparatively small size of A. altissima seedlings in this study suggested that the nutrient levels used may have been too low for A. altissima to thrive in a sandy environment. For both species, the two nutrient levels used in this experiment were designed to be close to a natural system, but not high enough to let the plants achieve optimal growth at the seedling stage. Nutrients were limiting because total, leaf and root biomass of both species increased from low nutrient level to high nutrient level. Therefore, our conclusion that R. pseudoacacia has a competitive advantage over A. altissima should be limited to relatively low and limiting soil nutrient conditions such as those used in this study. The results of Call and Nilsen (Citation2005) indicated that under unlimited nutrients, A. altissima is a better competitor than R. pseudoacacia.
4.3. A. altissima did change the growth patterns of R. pseudoacacia
Contrary to our prediction, the presence of A. altissima seedlings was associated with increased biomass of R. pseudoacacia seedlings rather than the expected negative competitive effect. However, we think that the ‘facilitation’ observed in this experiment from A. altissima on R. pseudoacacia (RYR > 1) was mainly due to the comparatively small size of A. altissima under these experimental conditions, which minimized its competitive effectiveness. The R. pseudoacacia seedlings had more resources available for growth when there were more A. altissima (and fewer R. pseudoacacia) in the pot because the R. pseudoacacia seedlings were released from intraspecific competition. Ailanthus altissima is known to have a substantial tap-root, therefore the constraint of the pot size may have differentially affected A. altissima compared with R. pseudoacacia. However, in a previous study (Call & Nilsen Citation2005) A. altissima seedlings produced much more root biomass in pots of similar dimension to this study.
Although A. altissima did not alter biomass and allocation to roots of R. pseudoacacia, the difference in A. altissima root biomass between high and low nutrient treatments became smaller as the proportion of R. pseudoacacia in the pot increased. This might suggest that, in low nutrient conditions, greater R. pseudoacacia density in the pot led to more of a facilitation effect on A. altissima through nitrogen fixation. However, because RYA did not increase as the proportion of R. pseudoacacia increased, this explanation seems unlikely. Therefore, this result may simply relate to the dominance of R. pseudoacacia in the belowground biomass under the higher nutrient condition, which led to stronger competition with A. altissima as the proportion of R. pseudoacacia increased.
4.4. The presence of A. altissima inhibited nodulation of R. pseudoacacia
The presence of A. altissima seedlings in the pot reduced the number of nodules per root mass for R. pseudoacacia. In addition, our study found R. pseudoacacia seedlings grown with A. altissima seedlings from the US seed lot had significantly fewer nodules per root weight compared with R. pseudoacacia seedlings grown with A. altissima seedlings from the Chinese seed lot.
Ailanthus altissima is well known to have an allelopathic effect on many species (Mergen Citation1959), and this could be one mechanism that determines the successful invasion of A. altissima (novel weapons; Rice Citation1984; Callaway & Ridenour Citation2004). Allelochemicals from some invasive species have been found to have negative impacts on microbial mutualists of native species and on nodulation of nitrogen-fixing plants (Weston & Putman Citation1985, Citation1986; Batish et al. Citation2007; Callaway et al. Citation2008; Wurst & Beersum Citation2009), including A. altissima (Lincoln Citation2012). In another bioassay study (Binninger and Bao, unpublished result), we found that a water extraction of A. altissima leaves significantly decreased both the germination rate and radicle growth of R. pseudoacacia seeds. These results suggest that the allelochemicals of A. altissima could negatively influence R. pseudoacacia growth and may be the reason why A. altissima inhibits nodulation of R. pseudoacacia. A direct measure of nitrogen fixation by gas chromatographic or stable isotope techniques would strengthen the proof that A. altissima inhibits nitrogen fixation by R. pseudoacacia.
4.5. Chinese A. altissima were more competitive than US A. altissima
Previous research found A. altissima had the competitive advantage over R. pseudoacacia primarily because of a dominant root biomass (Call & Nilsen Citation2005). Although A. altissima was not the winner of competition in this study, it did produce more roots when competing with R. pseudoacacia. Therefore, competition belowground is important for A. altissima competitive success.
The significantly higher root/shoot ratio found for the A. altissima seedlings from the US seed lot as compared to those from the Chinese seed lot suggests that the high root/shoot ratio might have been part of the mechanism for successful invasion for A. altissima in the USA. Some researchers suggest that the superior competitive ability of invasive species over native species is closely related to more efficient resource acquisition or resource conservation (Tecco et al. Citation2010; Gioria & Osborne Citation2014). A variety of invasive species have higher resource-use efficiency than native species, especially in low-resource environments (Funk & Vitousek Citation2007). Ailanthus altissima has high physiological plasticity (Mou et al. Citation2013), which is an important physiological trait for this exotic invasive species to adapt to changing environments and be an ‘opportunist’ when resource supply is variable. However, when grown in a relatively stable environment with a reliable supply of nutrients, similar to the experimental design used in our study, A. altissima did not show a tendency to be more competitive (Mou et al. Citation2013).
The two seed sources of A. altissima did not alter the allometric relationships between height and biomass of R. pseudoacacia. Yet, the two seed sources of A. altissima had different biomass accumulation, different responses to nutrient level and different competitive ability in their interaction with R. pseudoacacia. However, the only advantage of the Chinese A. altissima seed was the positive effect of nutrient increase on biomass production, which was a stronger effect than that of the US seedlings. This suggests that the A. altissima genotypes in the USA may not need as high of a nutrient level to thrive as would the Chinese A. altissima genotypes. This could be related to rapid evolution of A. altissima in the US populations to low nutrition in US disturbed habitats as has occurred for other introductions (Barney et al. Citation2009). Post-introduction adaptation is possible because A. altissima was introduced to the USA more than 300 years ago and significant adaptation could occur over this duration (Prentis et al. Citation2008). However, the likelihood of post-introduction adaptive evolution is species specific (Harris et al. Citation2012). Also, it is possible that the introduced population of A. altissima, 300 years ago, was selected to grow well in poor nutrient conditions. As a result, the US populations would have greater nutrient use efficiency than the source population in China. Differences among seedling responses to nutrient limitation have been shown to influence competition between seedlings of other tree species (Yi et al. Citation2015). However, we did not find that US A. altissima had a higher competitive ability than the Chinese A. altissima when challenged with R. pseudoacacia. This is because there was a lower biomass and a lower RYA for US A. altissima, and no difference in RYR, based on total biomass production, between the two seed sources. The resource allocation (root/shoot ratio and leaf biomass production) of R. pseudoacacia was changed significantly when grown with the different seed sources of A. altissima. Ailanthus altissima from its invasive range seemed to stimulate more root production of R. pseudoacacia. However, the seeds we used in this experiment were collected from a relatively small area in the USA and China, and therefore our conclusions only represent the differences between these two specific seed lots. We are not aware of any studies in the literature on genetic differences between Chinese A. altissima populations and those in the U.S. related to nutrient use efficiency or competitive effectiveness. Thus, more studies are needed to verify these observed differences in competitive ability of A. altissima genotypes in the USA compared to those in China.
4.6. Conclusion
In summary, this study revealed that the exotic invasive species was not always a winner at the seedling stage, especially when competing with a nitrogen-fixing, fast-growing native plant in low nutrient environments. Nitrogen fixation by R. pseudoacacia seedlings did not facilitate the growth of A. altissima seedlings. However, A. altissima roots can inhibit nodulation and growth of nitrogen-fixing species, perhaps by allelochemical means. Also, there was evidence to support the theory that invasive species have high nutrient acquisition ability. We found some evidence for different phenotypic response patterns between the invasive species from their invaded ecosystem compared with the invasive species from their native ecosystem. However, further analysis would be required to support the suggestion that there are genetically determined differences in response patterns. During early stages of succession, when A. altissima seedlings come into close proximity with R. pseudoacacia seedlings, R. pseudoacacia will have the upper hand unless the disturbed site is highly nutritious. However, if A. altissima seedlings survive they will inhibit nodulation of the R. pseudoacacia seedlings and perhaps change the trajectory of succession at the site. Longer-term research, in a more natural setting, through the sapling stage is needed to further understand the interactions between these species during forest development.
Supplemental data
Supplemental data for this article can be accessed at 10.1080/17429145.2015.1070208.
Supplementary_Material.pdf
Download PDF (133.9 KB)Acknowledgements
Thanks to Jacob Barney, Lisa Belden, Cynthia Huebner and Robert Jones for comments on earlier drafts of this manuscript. Thanks to Cynthia Huebner for the A. altissima seeds from the invaded range. Thanks to Austin Jackson, Nigel Temple, Tatpong Tulyanon and Deborah Wiley for help harvesting the plants.
Disclosure statement
No potential conflict of interest was reported by the authors.
Additional information
Funding
References
- Barney JN, Tekiela DR, Dollete ESJ, Tomasek BJ. 2013. What is the ‘real’ impact of invasive plant species? Front Ecol Environ. 11:322–329.
- Barney JN, Whitlow TH, DiTommaso A. 2009. Evolution of an invasive phenotype: shift to belowground dominance and enhanced competitive ability in the introduced range. Plant Ecol. 202:275–284.
- Batish D, Lavanya DK, Singh H, Kohli R. 2007. Phenolic allelochemicals released by Chenopodium murale affect the growth, nodulation and macromolecule content in chickpea and pea. Plant Growth Regul. 51:119–128.
- Besaw LM, Thelen GC, Sutherland S, Metlen K, Callaway RM. 2011. Disturbance, resource pulses and invasion: short-term shifts in competitive effects, not growth responses, favour exotic annuals. J Appl Ecol. 48:998–1006.
- Boring LRS, Swank WT. 1984a. Symbiotic nitrogen fixation in regenerating black locust (Robinia pseudoacacia L.) stands. Forest Sci. 30:528–537.
- Boring LRS, Swank WT. 1984b. The role of black locust (Robinia Pseudoacacia) in forest succession. J Ecol. 72:749–766.
- Call LJ, Nilsen ET. 2005. Analysis of interactions between the invasive tree-of-heaven (Ailanthus altissima) and the native black locust (Robinia pseudoacacia). Plant Ecol. 176:275–285.
- Callaway RM, Don C, Barto K, Thelen GC, Hallett SG, Prati D, Stinson K, Klironomos J. 2008. Novel weapons: invasive plant suppresses fungal mutualists in America but not in its native Europe. Ecology. 89:1043–1055.
- Callaway RM, Ridenour WM. 2004. Novel weapons: invasive success and the evolution of increased competitive ability. Front Ecol Environ. 2:436–443.
- Castro-Díez P, González-Muñoz N, Alonso A, Gallardo A, Poorter L. 2008. Effects of exotic invasive trees on nitrogen cycling: a case study in Central Spain. Biol Invasions. 11:1973–1986.
- Dostál P, Müllerová J, Pyšek P, Pergl J, Klinerová T. 2013. The impact of an invasive plant changes over time. Ecol Lett. 16:1277–1284.
- Finzi AC, Van Breeman N, Canham CD. 1998. Canopy tree–soil interactions within temperate forests: species effects on soil carbon and nitrogen. Ecol Appl. 8:440–446.
- Fowler N. 1982. Competition and coexistence in a North Carolina grassland: III. Mixtures of component species. J Ecol. 70:77–92.
- Funk JL, Vitousek PM. 2007. Resource-use efficiency and plant invasion in low-resource systems. Nature. 446:1079–1081.
- Gentle CB, Duggin JA. 1997. Allelopathy as a competitive strategy in persistent thickets of Lantana camara L. in three Australian forest communities. Plant Ecol. 132:85–95.
- Gioria M, Osborne BA. 2014. Resource competition in plant invasions: emerging patterns and research needs. Front Plant Sci. 5:501–522.
- Gómez-Aparicio L, Canham CD. 2008. Neighbourhood analyses of the allelopathic effects of the invasive tree Ailanthus altissima in temperate forests. J Ecol. 96:447–458.
- Halsall D, Leigh J, Gollasch S, Holgate M. 1995. The role of allelopathy in legume decline in pastures. II. Comparative effects of pasture, crop and weed residues on germination, nodulation and root growth. Aust J Agric Res. 46:189–207.
- Harris CJ, Dormontt EE, Le Roux JJ, Lowe A, Leishman MR. 2012. No consistent association between changes in genetic diversity and adaptive response of Australian acacias in novel ranges. Evol Ecol. 26:1345–1360.
- Heisey RM. 1990. Allelopathic and herbicidal effects of extracts from tree of heaven (Ailanthus altissima). Am J Bot. 77:662–670.
- Heisey RM. 1996. Identification of an allelopathic compound from Ailanthus altissima (Simaroubaceae) and characterization of its herbicidal activity. Am J Bot. 83:192–200.
- Hoshovsky MC. 1988. Element stewardship abstract for Ailanthus altissima. Arlington, VA: The Nature Conservancy.
- Hu SY. 1979. Ailanthus. Arnoldia. 39:29–50.
- Keane RM, Crawley MJ. 2002. Exotic plant invasions and the enemy release hypothesis. Trends Ecol Evol. 17:164–170.
- Lee CE. 2000. Evolutionary genetics of invasive species. Trends Ecol Evol. 17:386–391.
- Levine JM, Vilà M, Antonio CMD, Dukes JS, Grigulis K, Lavorel S. 2003. Mechanisms underlying the impacts of exotic plant invasions. Proc R Soc Lond B: Biol Sci. 270:775–781.
- Lincoln JM. 2012. Effects of Ailanthus altissima soil leachates on nodulation and expression of two genes that regulate nodulation of Trifolium pratense [master's thesis]. [Allendale (MI)]: Grand Valley State University.
- Mergen F. 1959. A toxic principle in the leaves of Ailanthus. Bot Gaz. 121:32–36.
- Mou P, Jones R, Tan Z, Bao Z, Chen H. 2013. Morphological and physiological plasticity of plant roots when nutrients are both spatially and temporally heterogeneous. Plant Soil. 364:373–384.
- Prentis PJ, Wilson JRU, Dormontt EE, Richardson DM, Lowe AJ. 2008. Adaptive evolution in invasive species. Trends Plant Sci. 13:288–294.
- Ricciardi A, Hoopes MF, Marchetti MP, Lockwood JL. 2013. Progress toward understanding the ecological impacts of nonnative species. Ecol Monogr. 83:263–282.
- Rice E. 1984. Allelopathy. Orlando, FL: Academic Press.
- Shea K, Chesson P. 2002. Community ecology theory as a framework for biological invasions. Trends Ecol Evol. 17:170–176.
- Simberloff D, Von Holle B. 1999. Positive interactions of nonindigenous species: invasional meltdown? Biol Invasions. 1:21–32.
- Tajima R, Lee ON, Abe J, Lux A, Morita S. 2007. Nitrogen-fixing activity of root nodules in relation to their size in peanut (Arachis hypogaea L.). Plant Prod Sci. 10:423–429.
- Tecco PA, Díaz S, Cabido M, Urcelay C. 2010. Functional traits of alien plants across contrasting climatic and land-use regimes: do aliens join the locals or try harder than them? J Ecol. 98:17–27.
- Tilman D. 1982. Resource competition and community structure. Princeton, NJ: Princeton University Press.
- Tsutsui ND, Suarez AV, Holway DA, Case TJ. 2000. Reduced genetic variation and the success of an invasive species. Proc Natl Acad Sci USA. 97:5948–5953.
- Vilà M, Espinar JL, Hejda M, Hulme PE, Jarošík V, Maron JL, Pergl J, Schaffner U, Sun Y, Pyšek P. 2011. Ecological impacts of invasive alien plants: a meta-analysis of their effects on species, communities and ecosystems. Ecol Lett. 14:702–708.
- Vlachodimos K, Papatheodorou E, Diamantopoulos J, Monokrousos N. 2013. Assessment of Robinia pseudoacacia cultivations as a restoration strategy for reclaimed mine spoil heaps. Environ Monit Assess. 185:6921–6932.
- Von Holle B, Joseph K, Largay E, Lohnes R. 2006. Facilitations between the introduced nitrogen-fixing tree, Robinia pseudoacacia, and nonnative plant species in the glacial outwash upland ecosystem of Cape Cod, MA. Biodivers Conserv. 15:2197–2215.
- Weston LA, Putnam AR. 1985. Inhibition of growth, nodulation, and nitrogen fixation of legumes by quackgrass. Crop Sci. 25:561–565.
- Weston LA, Putnam AR. 1986. Inhibition of legume seedling growth by residues and extracts of quackgrass (Agroyron repens). Weed Sci. 34:366–372.
- Williams AC, McCarthy BC. 2001. A new index of interspecific competition for replacement and additive designs. Ecol Res. 16:29–40.
- Wurst S, van Beersum S. 2009. The impact of soil organism composition and activated carbon on grass–legume competition. Plant Soil. 314:1–9.
- Wyckoff PH, Webb SL. 1996. Understory influence of the invasive Norway maple (Acer platanoides). Bull Torrey Bot Club. 123:197–205.
- Yi XM, Zhang Y, Wang XW, Wang YX, Ji LZ. 2015. Effects of nitrogen on the growth and competition between seedlings of two temperate forest tree species. Scand J Forest Res. 30:276–282.