Abstract
The coat protein (CP)-mediated resistance against Cucumber mosaic virus (CMV) subgroup IA was developed in transgenic lines of Nicotiana tabacum cv. Petit Havana using Agrobacterium tumefaciens-mediated transformation. Ten independently transformed lines have developed, four of which were tested for resistance against CMV using virus challenge inoculations. The transgenic lines exhibiting complete resistance remained healthy and symptomless in their life span and showed reduced or no virus accumulation in their systemic leaves after virus challenge inoculation. These transgenic lines also showed resistance against CMV strains which are not closely related to CMV-Gladiolus strains. This is the first report of CP-mediated transgenic resistance against a CMV subgroup IA member isolated from India showing resistance to all CMV strains occurring in the same vicinity.
1. Introduction
Cucumber mosaic virus (CMV) is a member of the family Bromoviridae and is one of the most important and widespread viruses in the world infecting the largest number of plant species (1200 species) (Zitter & Murphy Citation2009). The CMV isolate occurring on Gladiolus (Gladiolus psittacinus var. Hookeri cv. Red) caused mosaic leaves, stunted plants and color-breaking symptoms in flowers. The genome of CMV consists of three positive sense, single-stranded RNAs (RNA 1, RNA 2 and RNA 3) and a subgenomic RNA (RNA 4) encoded by RNA3 that is involved in encapsidation (Suzuki et al. Citation1991; Palukaitis et al. Citation1992). Several CMV isolates reported from all over the world have been placed into two subgroups I and II, on the basis of serology, nucleic acid hybridization and gene sequences. CMV subgroup I has been recently divided into IA and IB on the basis of gene sequences available for CMV strains and phylogenetic analysis (Roossinck Citation2002). Furthermore, Asian strains of CMV have been placed into subgroup IB (Roossinck Citation2002). First engineered resistance against CMV utilizing the coat protein (CP) gene was demonstrated by Cuozzo et al. (Citation1988). Since then, many examples of CP-mediated resistance (CPMR) in varying degrees have been described, with different constructs in different hosts (Beachy et al. Citation1990; Srivastava & Raj Citation2008). Different mechanisms also appear to be responsible for protection, depending on the virus group or the viral transgene studied (Loesch-Fries et al. Citation1987; Lomonossoff Citation1995; Gururani et al., Citation2012). The mechanism of resistance against virus could be mediated either by the expressed viral protein or by the transcript produced from the transgene, also known as post-transcriptional gene silencing (PTGS), or both (Varma et al. Citation2002). In this report, we demonstrate virus resistance in transgenic N. tabacum model plants expressing the CP gene of CMV isolated from Gladiolus, (CMV-G) in India and characterized as a member of the subgroup IA (Dubey et al. Citation2010). Though there are several reports from different parts of the world demonstrating transgenic resistance utilizing the CP gene from CMV subgroup IB or II, a report from a subgroup IA strain is still awaited from Asian continent as this strain is not common here. This is the first report from India which demonstrates transgenic resistance against subgroup IA as well as subgroup IB.
2. Materials and methods
2.1. Construct preparation and transformation of Nicotiana tabacum cv. Petit Havana plants
The coat protein gene of CMV (Gladiolus isolate, 657 bp, Accession no. DQ295914) was introduced in between the CaMV 35S promoter and the NOS terminator of the binary vector (pBI 121), which also has the NPTII marker gene for kanamycin resistance (Dubey et al. Citation2010). The construct was mobilized into Agrobacterium tumefaciens LBA 4404 through electroporation. One positive conjugant was chosen for transformation of N. tabacum leaf-discs following a procedure based on Horsch et al. (Citation1985). The transformed leaf-discs were regenerated on selective Murashige Skoog's (MS) medium containing Gamborg's B5 vitamins, antibiotics (500 mg/l cefotaxim and 100 mg/l kanamycin) and hormones (1 mg/l 6-benzyl aminopurine [BAP] and 0.1 mg/l α-naphthalene acetic acid [NAA]) and cultured kept in a growth chamber. The callus/shoots regenerating from the explants were subsequently transferred to MS medium containing 1.0 mg/l each of BAP and α-indole acetic acid (IAA) for shoot elongation and finally to a medium containing antibiotics and IAA 0.1 mg/l for rooting (Dubey et al. Citation2010). The putative transgenic plants were finally planted in pots and grown further in a glasshouse.
2.2. PCR and Southern analysis for T0 transgenic plants
The total genomic DNA was extracted from 1 g leaf tissue of N. tabacum as described by (Dellaporta et al. Citation1983). To verify the presence of the gene in T0 plants, polymerase chain reaction (PCR) was carried out using the total DNA isolated from each plant by using the CP-specific primers of CMV-Gladiolus (Dubey et al. Citation2010). The PCR products were electrophoresed with standard DNA in 1% agarose gel. Integration and copy number of the constructs in the T0 plants were confirmed by Southern blotting of plant genomic DNA (20 µg) digested with HindIII. The blotted DNA bands were hybridized with a probe prepared from ∼350 bp sequences located at the 3′ end of the CMV-CP gene so that the exact copy number could be determined. The DNA to be transferred was electrophoresed on 1% agarose gels according to standard procedures described by Sambrook et al. (Citation1989) and blotted on a nylon membrane (Dubey et al. Citation2010). The probes used for hybridization were prepared according to the random primer labeling method. Prehybrization and hybridization were carried out at 42°C with formamide (50%) and the blots were washed as instructed by the manufacturers (Dubey et al. Citation2010).
2.3. Western blot analysis
To determine the protein levels in transgenic plants, SDS–PAGE was performed using 12% polyacrylamide gel for resolving total proteins. Western blots were made by transferring the protein onto nitrocellulose membrane in a mini-trans blot apparatus (Bio-Rad). Expression of the gene in plants at the protein level was determined by using antiserum to CMV (PVAS 242a, ATCC, USA) (Dubey et al. Citation2010). Approximately 20 µg of total soluble protein from each sample was loaded in each lane and the accumulation of CP in uninoculated, inoculated and terminal upper leaves was determined. Antiserum specific to CMV (primary antibody) was used at a dilution of 1:500 and alkaline phosphatase conjugate (Sigma) secondary antibody (goat anti-rabbit conjugate) was used at a dilution of 1:1000 and western blot was developed with BCIP-NBT substrates (Sigma) (Dubey et al. Citation2010).
2.4. Challenge inoculations of transgenic plants for virus resistance
Transgenic plants (4–6-leaf stage) were challenged with 1:10 inoculum (crude extract) prepared from CMV-infected tobacco and CMV from other infected plants reported from the same vicinity, macerated in 0.1 M sodium phosphate buffer, pH 7.0 containing 1% sodium sulphite (1 ml buffer/100 mg tissue). Inoculated plants were observed daily for 15–20 days for the development of symptoms and compared with the control (untransformed challenged) (Dubey et al. Citation2010). Plants that did not develop any symptoms were checked by back inoculation tests to detect latent infection, if any.
3. Results
3.1. Preparation of CMV-CP constructs
Besides the identification of the CP gene from Gladiolus strain of CMV, its cloning and sequencing were carried out with the aim of developing transgenic plants exhibiting resistance against the virus infection. Therefore, constructs of the CP gene of CMV-Gladiolus strain were designed in which the CP gene was introduced in between the CaMV 35S promoter and the NOS terminator gene of a binary vector (pBI 121). The schematic diagram of this has been shown (). The binary vector has the NPT II marker gene that confers resistance to antibiotic, kanamycin. First, both clone pTZ57R/T carrying coat protein gene of CMV and vector pBI 121 were digested separately with restriction enzymes XbaI and Sac I for the purpose of directional cloning. The restricted DNAs of CMV-CP gene and pBI121 were eluted, purified and ligated to each other. Subsequently, competent E. coli (DH5α) cells were transformed with the ligation mixture and the recombinant cells containing the construct were selected on media containing kanamycin at appropriate concentration.
3.2. Screening of constructs containing the CMV-CP gene
Several transformed colonies were randomly picked up and plasmid DNA was isolated through miniprep. The DNA from selected constructs was digested with different sets of enzymes to confirm the presence and size of insert in them. Sets of reactions were set up for each construct screened; one set digested with XbaI and SacI enzymes (as cloning was done at these sites in the binary vector). The results of the restriction digestion showed that two bands one belonging to plasmid and the other one of insert indicated the confirmed ligation of vector and insert, i.e. CMV-CP gene ((a)).The positive constructs were also screened by PCR using the CMV-CP specific primers that yielded the expected ∼660 bp amplicon; further confirmation of construct was done by Southern hybridization ((b) and (c)).
Figure 2. Confirmation of construct pBI CMV-CP. (a) Restriction digestion of recombinant vector plasmid by XbaI and Sac I, showing plasmid pBI 121 and CMV-CP gene, Lane M, λ DNA Eco RI–Hind III marker. (b) PCR of recombinant vector plasmid with CMV-CP specific primer. (c) Southern hybridization of same PCR gel probed with α-P32 labeled cloned CP gene of CMV.
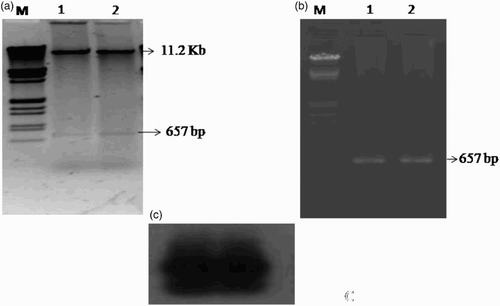
3.3. Mobilization of constructs into Agrobacterium tumefaciens (LBA 4404)
A. tumefaciens (LBA 4404) cell was transformed by the electroporation. Purified binary vector pBI121 having cloned CMV-CP gene was added on the top of frozen competent cells and mixture was placed in an ice-cold electroporation cuvette. Electroporation was performed and the electroporated mixture was plated on appropriate medium (YEP) containing streptomycin, rifampicin and kanamycin in appropriate concentration, and grown at 28°C for two days. The transformed Agrobacterium colonies were selected on YEP plates containing appropriate antibiotics (rifampicin and kanamycin). Miniprep DNA from a few selected colonies was prepared and screened for the presence of construct in them through restriction digestions and PCR analyses. One such positive colony obtained from the construct was chosen for leaf-disc transformation of N. tabacum cv. Petit Havana plants.
3.4. Genetic transformation of Nicotiana tabacum and regeneration of T0 plants
3.4.1. Regeneration of T0 plants
In a succession of initial experiments, callusing, shoot production and rooting from leaf explants of N. tabacum cv. Petit Havana was established on modified MS medium by using different combination of growth regulators and modification of MS medium. Besides this, additional experiment were performed to determine the optimum concentration of kanamycin, which is sufficient to inhibit the regeneration of shoots or resulted in death of explants. The medium containing a combination of hormone concentration of NAA 1 mg/l and BAP 0.1 mg/l was found to be best for callus induction; BAP 1 mg/l and NAA 0.1 mg/l for shooting and ½ MS medium containing NAA 0.1 mg/l was found to be the best for rooting. Murashige and Skoog's (Citation1962) medium (MS) was found to be the best for regeneration of tobacco leaf explants. Dose–response experiments conducted with various concentrations of kanamycin revealed that at 100 mg/l kanamycin concentration was suitable for transformation of tobacco and higher concentration to this was fatal for its growth. Therefore, finally, (MS salts, GR) supplemented with antibiotics, 100 mg/l kanamycin and 500 mg/l Cefotaxim was chosen for leaf-disc transformation of N. tabacum cv. Petit Havana plants. The concentration of kanamycin was reduced to 250 mg/l, and subsequently to 50 mg/l at the rooting stage.
Leaf-disc transformation of tobacco was carried by using Agrobacterium strain LBA 4404, carrying binary vector pBI 121 having CMV-CP gene located between the left and right borders of T-DNA.
Transformation results revealed that large number of leaf explants (about 80%) co-cultivated with A. tumefaciens showed direct callus induction from them after two weeks. Explants produced light green friable callus which induced shoots, when transferred on shoot-inducing medium. Explants showing either direct shoot initiation or callus formation were transferred after 2–3 weeks to the same MS basal medium containing shoot-induction growth regulators. The similar concentrations of kanamycin were maintained throughout the regeneration process, i.e. 100 mg/l kanamycin from shoot initiation to rooting stage. All the explants were kept on selection medium and those showing well-defined shoot initiation were transferred to the same fresh medium for further shoot proliferation. In the shooting medium, each transformed explant capable of producing shoots was treated as an individual line and designated as A to J for 10 independent lines. These explants were subsequently transferred from petri-plates to culture tubes for profuse growth of developing shoots.
When the putatively transformed shoots gained a sufficient height (3–4 cm long), they were transferred to the rooting medium containing ½ MS salts and NAA 0.1 mg/l. Care was taken while transferring shoots to the rooting medium. The shoots should be devoid of callus to avoid re-initiation of callus formation and hindering rooting. To take into account as many independent transformation events as possible, only one shoot per event was excised and transferred to the rooting medium. Ten lines represented at least one regeneration event, and each one was transferred to the rooting medium. These shoots produced well-defined roots within 2–3 weeks. It was further observed that some of the putative transformed shoots did not produce roots on kanamycin containing medium, although they survived for a long time period. On other hand, the controls grown on the MS medium plus kanamycin did not respond to shoot as well as root formation. Further, well-rooted putatively transformed plants were subsequently transferred to Hoagland's solution for hardening for about one week and then transferred to vermiculite for another 7–10 days. In both the instances of hardening, the plants were kept inside a specially designed chamber under controlled conditions. Plants were shifted to soil in the glasshouse and maintained for further acclimatization and habituation to glass house conditions. Acclimatized and putatively transformed plants grew to maturity and produced flowers. However, no morphological differences between the transformed and control plants were observed.
3.5. Analysis of T0 generation transformants
The putatively transformed plants of T0 generation were analyzed by following adopted methods for the presence and expression of CP gene in them.
3.5.1. PCR and Southern and western blot analysis
The PCR was performed from all of the transformants using CMV-CP specific primers, which revealed the presence of CP gene in 80% of plants whereas the untransformed tobacco plants were PCR negative ((a)).
Figure 3. Analysis of T0 generation transformants for CMV-CP gene. (a) Confirmation by PCR of 10 transformed lines, (b) Confirmation by Southern blot of transformed lines, (c) Western blot of transformed lines.
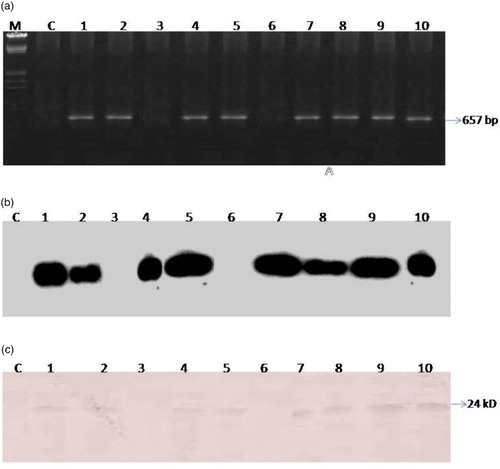
In order to confirm the identity of product as CMV-CP, the same gel was transferred to nylon membrane for hybridization with the α-32P dCTP radio-labeled probe prepared from cloned CP gene of CMV-CP (CMV-CP Gladiolus isolate) by random primer extension with prior denaturation of the template DNA. The positive signal obtained upon hybridization confirmed that the PCR amplicon contained the CP gene of CMV ((b)).
In vivo translation of CP mRNA in the leaves of T0 plants was determined by western blot immunoassay analysis using antibodies to CMV-G. Total proteins extracted were electrophoresed on 12% denaturing gel and transferred to nitrocellulose membrane. The proteins transferred on membrane were treated with CMV specific antibodies. A 24 kDa band of CP was detected in all the T0 tested plants ((c)).
The above results confirmed that transgenic N. tabacum cv. Petit Havana plants thus produced successfully expressed the CP gene of CMV-G.
3.6. Evaluation of resistance in transgenic plants
Transgenic plants thus developed were evaluated for the degree of resistance in them against CMV infection in successive generations. For these four transgenic lines, namely A, D, E and H were selected. Screening for resistance in A, D, E and H transgenic lines was done till T1 generation. The results of resistance achieved are described in the following section.
3.6.1. Inheritance of transgenes in T1 generations and CMV resistance
The seedling progeny from four transgenic lines A, D, E and H were self-fertilized. Approximately 30 seeds from each line were surface sterilized, kept on MS medium containing kanamycin (100 mg/l) and germinated to analyze the percentage survival of plants on kanamycin. Progeny of all four lines was segregated with a standard Mendelian monohybrid ratio of 3:1. The segregation data for resistance or susceptibility to kanamycin indicated that progenies of all four lines carried a single copy gene. For analysis of resistance against CMV infection in T1 generation, 10 plants (3–4-leaf stage) of each line were taken. At each three leaf stage, the plants were first confirmed by PCR and Southern hybridization for the presence of CMV-CP gene in them ((a) and (b)). Then the transgenic plants were mechanically challenged by CMV. Symptom development on these plants, if any was monitored everyday in the glasshouse. It was observed that in control plants the inoculated leaves showed chlorosis in 2–4 days and by the end of first week, mosaic symptoms became visible in control inoculated plants. In contrast, symptoms produced in all the transgenic plants were delayed by 25–30 days post inoculation. Few transgenic progenies of A and E lines which expressed the coat protein did not show any symptoms on the inoculated leaf in the four weeks of post inoculation. The percentage of plants showing susceptibility or resistance among the respective progenies of A, D, E and H lines are shown in . As evident from the table, approximately 20% of the plants in line A and 30% in line E were those which were fully resistant to CMV, whereas other plants of these lines showed delay in symptoms up to 25 dpi. On the other hand, none of the plants of line D and H were fully resistant, although delay in symptoms was observed in these lines up to 25 dpi in few plants, whereas susceptible plants showed symptoms at 15 dpi. These plants either developed very mild to moderate mosaic symptoms at earlier stages.
Table 1. Percentage of A, D, E and H progenies (T1 generation) showing full resistance or delayed symptoms or susceptibility to CM-Gladiolus infection at 25 days post inoculation.
4. Discussion
Recently, due to increasing human population and rapid globalization the demand of food is more than supply. So to achieve this target, application of hazardous chemicals in agriculture practices is increasing day by day. Because of the virus infection to the plants, the overall quality and quantity of food production has been decreased; therefore, it is of prime importance to create virus-resistant plants. Hence, identification and exploitation of genetic resources of resistance to viral pathogens has become as an ideal approach for controlling viral diseases (Gielenet al. Citation1996). Thus, one of the main objectives of the work, therefore, was to study whether CPMR strategy could be applied for raising transgenic plants utilizing the CP gene of CMV-Gladiolus strain and if resistance of any kind (delayed or complete or none) could be achieved in regenerated transgenic plants. If found effective the strategy could very well be applied to other commercially important crops and ornamental plant species that are infected by CMV, where the yield and quality get severely affected every year due to CMV infection ().
Transgenic host resistance has been proposed as a means to protect plants from viruses. The concept of pathogen-derived resistance (PDR) (Sanford and Johnston Citation1985) was first demonstrated in plants by using the CP gene of Tobacco mosaic virus (TMV) in tobacco plants to confer resistance against its homologous virus (Powell-Abel et al. Citation1986). Such transgenic expression of viral CP in plants confers protection against the homologous virus and sometimes to closely related viruses. This strategy, referred to as CP-mediated protection, has been widely tested.
CPMR is a form of PDR to obtain resistance in plants against different viruses which has emerged as one of the most promising approaches in recent times. Attempts for conferring resistance against CMV using the coat protein gene was first initiated by Cuozzo et al. (Citation1988) who generated transgenic tobacco plants which showed high resistance to CMV infection. Apart from CP gene, other genes found in the genome of CMV have also been used by various workers to generate transgenic plants for resistance against CMV infection. CMV is one of the important viruses for which CP-mediated resistance has been aimed. The various reports on CPMR, however, indicate very differently, and sometimes even opposing outcomes, which might be partly due to different levels of CP accumulation obtained on account of different gene constructs and/or different host systems. For all reported successful cases of CP-mediated resistance against CMV, high expression levels of transgenic CP were reached (Cuozzo et al. Citation1988; Quemada et al. Citation1991; Gonsalves et al. Citation1992, Citation1994; Namba et al. Citation1992; Yie et al. Citation1992; Nakajima et al. Citation1993; Yoshioka et al. Citation1993; Okuno et al. Citation1993a; Xue, et al. Citation1994; Kaniewski et al. Citation1999; Jacquemond et al. Citation2001; Shin et al. Citation2002b), although it is known that this did not at all assure resistant phenotype (Jacquemond et al. Citation2001). The resistance responses in the different CMV-host combinations seems to vary considerably, in some cases only a temporary delay in symptom appearance was observed. In other cases milder or attenuated expression of symptoms were reported, and in yet other cases no symptoms at all were observed suggesting full resistance. Previously published data suggested that the levels of CP-mediated resistance against CMV are correlated with the amounts of transgenically produced CP resistant plants (Cuozzo et al. Citation1988; Namba et al. Citation1992; Okuno et al. Citation1993b; Rizos et al. Citation1996; Kaniewski et al. Citation1999). Our data also strengthen these conclusions that among the four CP expressing lines only the progenies of lines A and E showed high level of CP expression whereas low CP accumulation lines D and H showed delayed symptom expression (). Previous studies have reported estimated CP levels of 0.16% and 0.2% of total soluble protein in resistant CP transformed tobacco plants (Quemada et al. Citation1991; Okuno et al. Citation1993b). It cannot be excluded that our approaches to aim for CP-mediated expression were optimal in lines A and E where plants showed resistance to CMV, and expression was too low in D and H lines to provoke resistance. Nonetheless, it is clear that transformation using the CP gene to obtain virus-resistant host plants is a successful approach. Although high levels of CP expression are indeed crucial for obtaining operational levels of resistance. CP expressing lines have always been observed to form a small minority (Kaniewski et al. Citation1999), requiring the transformation and testing of large numbers of lines. Studies carried out by (Kaniewski et al. Citation1999) revealed that the use of the Figwort mosaic virus major promoter to replace the CaMV 35S promoter may lead to a 20-fold enhanced accumulation of transgenic CP corresponding to enhanced resistance levels as well. Hence, this might be one way to improve the methodology for CP-mediated resistance, although the result may turn out to be host dependent. The mechanism by which CP-mediated resistance operates is not fully understood, yet. Experiments in transgenic TMV CP-expressing tobacco protoplasts revealed that an early step in virus replication is blocked, possibly co-translational disassembly (Osbourn et al. Citation1989). In addition, it has been suggested that CP-mediated resistance is based on abortive replication of the invading viral RNAs by the viral RdRp, due to the presence of large amounts of CP in the transgenic plant cells (de Haan Citation1998).
Analysis revealed that the level of resistance in plants varied in different plant lines ranging from near complete resistance to delayed symptom appearance to fully susceptible transgenic plants. In general, more than 50% of the plants showed a fully susceptible phenomenon whereas 30% plants showed delay in symptom appearance. Only two out of 10 plants (20%) in line A and three out of 10 (30%) in line E showed full resistance throughout life. However, in lines D and H none of the plants were found to be fully resistant, although these plants also showed delay in symptom appearance. These plants showed reduced susceptibility to CMV infection as well as decrease in severity of symptoms than the untransformed controls. The symptoms in these plants were delayed to at least 20–25 dpi, whereas susceptible controls in most cases produced 100% symptoms by 10–15 dpi. Susceptible transgenic plants initially showed chlorosis in the inoculated leaves in one week but appearance of systemic mosaic symptoms in them was observed only after 10–15 dpi. These findings are in accordance with those reported by Nelson et al. (Citation1987); Tumer et al. (Citation1987a); Nakajima et al. (Citation1993); Gielen et al. (Citation1996) who showed delaying in the systemic spread of the infecting virus in the transgenic plants (tobacco or tomato) expressing the viral CP. At the same time five plants from each A, D, E, H lines challenged from CMV isolates through mechanical inoculation were showed delay in symptoms appearance and resistant to CMV ().
Table 2. Cucumber mosaic virus challenge inoculation from different host reported from subgroup IB to transgenic lines expressed IA coat protein gene.
T1 plants which did not develop systemic symptoms after inoculation with CMV were characterized by significantly reduced level of virus accumulation in their systemic leaves as compared to their inoculated leaf (). This may be supported by low virus levels detected in their upper systemic leaves. Okuno et al. (Citation1993b) have also reported higher virus accumulation in the inoculated leaves of transgenic plants expressing the CP gene than in the systemic leaves. As reviewed by Beachy et al. (Citation1990), the CPMR is manifested by a reduced rate of systemic spread of infection whereby development of systemic disease symptoms in CP (+) plants is either completely inhibited or much reduced than the non-transgenic controls so that even if the inoculation resulted in infection on the inoculated leaves, the likelihood of the infection becoming systemic is reduced. Similarly, Nelson et al. (Citation1987) have also reported that the delay in systemic symptom development in transgenic tobacco expressing the CP gene of TMV was correlated with reduction in lesion numbers and virus accumulation in the transgenic plants. On the other hand, resistance was also shown by plants that, although accumulated virus at high levels in their systemic leaves as detected by Western blot immunoassay remained symptomless throughout their life cycle. However, accumulation levels in them were not as high as found in the diseased control.
Acknowledgments
Vimal Kumar Dubey acknowledges the Council of Scientific and Industrial Research, New Delhi for providing Senior Research Fellowship.
Disclosure statement
No potential conflict of interest was reported by the authors.
References
- Beachy RN, Loesch-Fries S, Tumer NE. 1990. Coat protein-mediated resistance against virus infection. Annu Rev Phytopathol. 28:451–74.
- Cuozzo M, O'Connell KM, Kaniewski W, Fang R, Chua N, Tumer NE. 1988. Viral protection in transgenic tobacco plants expressing the Cucumber mosaic virus coat protein or its antisense RNA. Biotechnology. 6:549–557.
- Dellaporta SL, Wood J, Hicks JB. 1983. A plant DNA minipreparation: Version II. Plant Mol Biol Rep. 1:19–21.
- Dubey VK, Aminuddin, Singh VP. 2010. Molecular characterization of Cucumber mosaic virus infecting Gladiolus, revealing its phylogeny distinct from the Indian isolate and alike the Fny strain of CMV. Virus Genes. 41:126–134.
- Gielen J, Ultzen T, Bontems S, Loots W, van Schepen A, Westerbock A, de Haan P, van Grinsven M. 1996. Coat protein-mediated protection of cucumber mosaic virus infection in cultivated tomato. Euphytica. 88:139–149.
- Gonsalves C, Xue B, Yepes M, Fuchs M, Ling K, Namba S, Chee P, Slightom JL, Gonsalves D. 1994. Transferring cucumber mosaic virus-white leaf strain coat protein gene into Cucumis melo L. and evaluating transgenic plants for protection against infections. J Amer Soc Hort Sci. 119:345–355.
- Gonsalves D, Chee P, Provvidenti R, Seem R, Slightom JL. 1992. Comparison of coat protein-mediated and genetically-derived resistance in cucumbers to infection by cucumber mosaic virus under field conditions with natural challenge inoculations by vectors. Biotechnology. 10:1562–1570.
- Gururani MA, Venkatesh J, Upadhyaya CP, Nookaraju A, Pandey SK, Park SW. 2012. Plant disease resistance genes: current status and future directions. Physiol Mol Plant Pathol. 78:51–65.
- de Haan P. 1998. Mechanisms of RNA-mediated resistance in plant viruses. In: Methods in molecular biology, Vol. 81: Plant virology protocols. Totowa, NJ: Humana Press; p. 533–546.
- Horsch RB, Fry JE, Hoffmann NL, Eichholtz D, Rogers SG, Frailey RT. 1985. A simple and general method for transferring genes to plants. Science. 227:1229–1231.
- Jacquemond M, Teycheney PY, Carrère I, Navas-Castillo J, Tepfer M. 2001. Resistance phenotypes of transgenic tobacco plants expressing different Cucumber mosaic virus (CMV) coat protein genes. Mol Breed. 8:85–94.
- Kaniewski W, Ilardi V, Tomassoli L, Mitsky T, Layton J, Barba M. 1999. Extreme resistance to cucumber mosaic virus (CMV) in transgenic tomato expressing one or two viral coat proteins. Mol Breed. 5:111–119.
- Loesch-Fries LS, Merlo D, Zinnen T, Burhop L, Hill K, Krahn K, Jarvis N, Nelson S, Halk E. 1987. Expression of alfalfa mosaic virus RNA4 in transgenic plants confers virus resistance. EMBO J. 6:1845–1851.
- Lomonossoff GP. 1995. Pathogen-derived resistance to plant viruses. Annu Rev Phytopathol. 33:323–343.
- Murashige T, Skoog F. 1962. A revised medium for rapid growth and bioassays with tobacco tissue cultures. Physiol Plantarum. 15:473–497.
- Nakajima M, Hayakawa T, Nakamura I, Suzuki M. 1993. Protection against cucumber mosaic virus (CMV) strains O and Y and chrysanthemum mild mottle virus in transgenic tobacco plants expressing CMV-O coat protein. J Gen Virol. 74:319–22.
- Namba S, Ling K, Gonsalves C, Slightom JL, Gonsalves D. 1992. Protection of transgenic plants expressing the coat protein gene of watermelon mosaic virus ii or zucchini yellow mosaic virus against six potyviruses. Phytopathology.82:940–946.
- Nelson RS, Powell Abel P, Beachy RN. 1987. Lesions and virus accumulation in inoculated transgenic tobacco plants expressing the coat protein gene of tobacco mosaic virus. Virology. 158:126–132.
- Okuno T, Nakayama M, Furusawa I. 1993a. Cucumber mosaic virus coat protein-mediated protection.Virology. 4:357–361.
- Okuno T, Nakayama M, Yoshida S, Furusawa I, Komiya T. 1993b. Comparative susceptibility of transgenic tobacco plants and protoplasts expressing the coat protein gene of cucumber mosaic virus to infection with virions and RNA. Phytopathology. 83:542–547.
- Osbourn JK, Watts JW, Beachy RN, Wilson TMA. 1989. Evidence that nucleocapsid disassembly and a later step in virus replication are inhibited in transgenic tobacco protoplasts expressing TMV coat protein. Virology. 172:370–373.
- Palukaitis P, Roossinck M, Dietzgen RG, Francki RIB. 1992. Cucumber mosaic virus. Adv Virus Res. 41:281–348.
- Powell-Abel P, Nelson RS, De B, Hoffmann N, Rogers SG, Fraley RT, Beachy RN. 1986. Delay of disease development in transgenic plants that express the tobacco mosaic virus coat protein gene. Science. 232:738–743.
- Quemada HD, Gonsalves D, Slightom JL. 1991. Expression of coat protein gene from cucumber mosaic virus strain C in tobacco: protection against infections by CMV strains transmitted mechanically or by aphids. Phytopathology. 81:749–802.
- Rizos H, Gillings MR, Pares RD, Gunn LV, Frankham R, Daggard G. 1996. Protection of tobacco plants transgenic for cucumber mosaic cucumovirus (CMV) coat protein is related to the virulence of the challenging CMV isolate. Aust Plant Pathol. 25:179–185.
- Roossinck MJ. 2002. Evolutionary history of cucumber mosaic virus deduced by phylogenetic analyses. J Virol. 76:3382–3387.
- Sambrook J, Fritsch EF, Maniatis T. 1989. Molecular cloning: A laboratory manual. 2nd ed. Cold Spring Harbor, NY: Cold Spring Harbor Laboratory, Press.
- Sanford JC, Johnston SA. 1985. The concept of parasite-derived resistance – Deriving resistance genes from the parasites own genome. J Theor Biol. 113:395–405.
- Shin R, Han JH, Lee GJ, Peak KH. 2002b. The potential use of a viral coat protein gene as a transgene screening marker and multiple virus resistance of pepper plants coexpressing coat proteins of Cucumber mosaic virus and Tomato mosaic virus. Transgenic Res. 11:215–219.
- Srivastava A, Raj SK. 2008. Coat protein-mediated resistance against an Indian isolate of the Cucumber mosaic virus subgroup IB in Nicotiana benthamiana. J Biosci. 33:249–257.
- Suzuki M, Kuwata S, Kataoka J, Masuta C, Nitta N, Takanami Y. 1991. Functional analysis of deletion mutants of cucumber mosaic virus RNA3 using an in vitro transcription system. Virology. 183:106–113.
- Tumer NE, Hemenway C, O'Connell KM, Cuozzo M. 1987a. Expression of coat protein genes in transgenic plants confers protection against alfalfa mosaic virus, cucumber mosaic virus and potato virus X. In: von Wettstein D, Chua N-H, editor. Plant molecular biology. London: Plenum Publishing Corporation; p. 351–356.
- Varma A, Jain RK, Bhat AI. 2002. Virus resistant transgenic plants for environmentally safe management of viral diseases. Indian J Biotechnol. 1:73–86.
- Xue B, Gonsalves C, Provvidenti R, Slightom JL, Fuchs M, Gonsalves D. 1994. Development of transgenic tomato expressing a high level of resistance to cucumber mosaic virus strains of subgroups I and II. Plant Disease. 78:1038–1041.
- Yie Y, Zhao F, Zhao S, Liu Z, Liu YZ, Tien P. 1992. High resistance to cucumber mosaic virus conferred by satellite RNA and coat protein in transgenic commercial tobacco cultivar G-140. Mol Plant Microbe Interact. 5:460–465.
- Yoshioka K, Hanada K, Harada T, Minobe Y and Oosawa K. 1993. Virus resistance in transgenic melon plants that express the cucumber mosaic virus coat protein gene and their progeny. Japan J Breed. 43:629–634.
- Zitter TA, Murphy JF. 2009. The Plant Health Instructor. doi: 10.1094/PHI-I-2009-0518-01.