ABSTRACT
Under cold stress, reactive oxygen species (ROS) are considered the main source of damage to plant cells. Mechanisms of ROS scavenging in wheat are very important during stress and the antioxidant enzymes superoxide dismutase, Catalase and Glutathione peroxidase are key to facilitating ROS scavenging. Molybdenum (Mo) is involved in many plant physiological and biochemical processes including antioxidant enzymes. This study reports research to investigate the effect of Mo application in enhancing antioxidant enzymes in two wheat cultivars. The results confirmed that antioxidant defense is important in wheat that is exposed to abiotic stress and that changes in activities of antioxidant enzymes occurred during exposure of plants to low non-freezing temperatures and by adding Mo. Mo application had a positive effect on gene expression of both Cbf14 and COR15a protein expression, indicating upregulation of the stress response regulon. In addition, Mo enhanced antioxidant enzymes activity and improved frost tolerance.
1. Introduction
Low temperature is one of the most important abiotic stresses affecting plant growth, production and distribution throughout the world. Some plants can improve their tolerance to low temperatures through a process known as cold acclimation when they are exposed to low non-freezing temperature (0–4°C) (Thomashow Citation1999; Fowler Citation2007; Al-Issawi et al. Citation2013a). During the acclimation process, many cellular changes occur such as the accumulation of osmoprotectants such as soluble sugars (Uemura et al. Citation2003), amines, and compatible solutes such as polyols, proline and betaine (Allard et al. Citation1998; Naidu Citation1998), via activation of low-temperature signal transduction pathways, which eventually lead to membrane stability and altered gene expression to provide tolerance (Thomashow Citation2010). Recent studies have reported that gene expression for wheat might be changed not just through low temperature (4°C), but following exposure to some chemicals used for seed priming, for example, Molybdenum (Mo) through upregulation of signal transduction pathway, that is, increased C-repeat Binding Factor (CBF) expression (Sun et al. Citation2009; Al-Issawi et al. Citation2013b). Under biotic and abiotic stresses, reactive oxygen species (ROS) are considered the main source of damage to plant cells (Mittler Citation2002; Vaidyanathan et al. Citation2003; De Gara et al. Citation2003; Candan & Tarhan Citation2003; Baek & Skinner Citation2003). ROS are a partially reduced form of oxygen that is involved in important processes such as photorespiration, photosynthesis and respiration (Uchida et al. Citation2002; Mittler Citation2002). In order to produce water in these processes, four electrons are required for the oxygen reduction reaction. However, the presence of ROS may result from the transfer of one, two and three electrons, respectively, to O2 from Superoxide (), peroxide hydrogen (H2O2) and hydroxyl (HO.) (Mittler Citation2002). These species of oxygen are highly cytotoxic and can seriously react with vital biomolecules such as lipids, proteins, nucleic acids, and other macromolecules causing lipid peroxidation, protein denaturation and DNA mutation (Quiles & López Citation2004). The membranes are considered the first place of abiotic stress responses, which lead to cell and organelle injuries (Candan & Tarhan Citation2003). ROS can react with essential lipids in the plasmalemma or intracellular organelles through a peroxidation process causing a membrane breach leading to leakage of cellular contents, rapid desiccation and cell death (Karabal et al. Citation2003). This damage to the intracellular membranes can also affect respiratory activity in mitochondria and cause a breakdown of pigments that might affect carbon fixation ability in the chloroplast (Scandalios Citation1993). The mechanisms of ROS scavenging in plants are very important and are carried out through the antioxidant enzymes such as superoxide dismutase (SOD:
+
+ 2 H+ ⇔2 H2O + O2), catalase (CAT: 2 H2O2⇔ 2 H2O +O2) and glutathione peroxidase (GPXs: 2glutathione + polyunsaturated fatty acid (PUFA)-OOH⇔glutathione reductase (GSSG) + PUFA + 2H2O), and it has been found that during acclimation, plants increase their stress-related proteins, which improve both the levels and activities of antioxidant enzymes. The antioxidants scavenge the ROS, decrease the photo-oxidation and preserve chloroplast membrane integrity and thereby help to increase the rate of photosynthesis (Waraich et al. Citation2012).
Mo is an essential element for good plant growth and is also involved in many plant physiological processes (Mendel & Hänsch Citation2002; Sun et al. Citation2009). Mo is an important element for more than 40 enzymes, 4 of which have been found in plants (Sun et al. Citation2009) and include nitrate reductase (NR), which is involved in nitrogen fixation and assimilation, xanthine dehydrogenase/oxidase (XDH), which plays an important role in purine catabolism, aldehyde oxidase (AO), which plays an essential part in the synthesis of indole-3 acetic acid (IAA) and abscisic acid (ABA), a stress response hormone, and sulfite oxidase (SO), which has a role in sulfur metabolism (Kaiser et al. Citation2005). AO is a key enzyme for catalyzing the final step of ABA production. In order to improve our understanding of the role of Mo on the development of frost tolerance in wheat and according to the positive results of Al-Issawi et al. (Citation2013b) who showed that Mo is able to upregulate frost tolerance in wheat with or without low-temperature acclimation, this study focuses on the changes of antioxidant enzyme activities and COR15a protein expression under cold stress of two cultivars of wheat under Mo treatments.
2. Materials and methods
2.1. Seed treatment with Mo and plant materials
Seeds of two varieties were used: European winter wheat cv. Claire with moderately high vernalization requirements and moderate frost tolerance, and Iraqi spring wheat cv. Abu-Ghraib, which can be sown as a winter wheat in mild winter climates. Two treatments (+Mo and −Mo) were applied to seeds of both varieties. Half of the seeds from each genotype were soaked in a solution containing 1% molybdate (NH4)6Mo7O24·4H2O] (0.008 M) for 8 h at 20°C (Wang et al. Citation1999; Jafar et al. Citation2012), and the other half were soaked in distilled water for the same period (hydro priming) and then air dried until they reached their original moisture content (14%) (Ahmadi et al. Citation2007). Seeds were then sown in trays in a growth cabinet (Snijder scientific) in order to obtain seedlings at the same physiological age. Seedlings were transplanted into pots (127 × 127 × 152 mm) with nine seedlings per pot, containing John Innes No.1 compost. Seedlings were left to grow in a semi-controlled greenhouse (∼20°C) for 30 days until the 3–4 leaf stage (GS13–14 (Zadoks et al. Citation1974)). Pots of each treatment were subdivided into two groups (n = 7), and placed either in an acclimation chamber at 4°C, 8 h photoperiod (PPFD 177 µmol m−2 s−1) for 14 days, or a growth cabinet at 20°C at the same photoperiod and photosynthetic active radiation. Samples for protein or enzyme extraction were taken after 14 days from transferring plants to the new conditions and were stored at −80°C until analysis.
2.2. Protein and antioxidant enzymes extraction
Plant materials from −80°C were thawed on ice and placed in a mortar and pestle. Two milli liter of extraction buffer (100 mM potassium phosphate buffer) (200 mL of 2 M KPO4 stock solution was prepared by dissolving K2HPO4 63.2 g and KH2PO4 5.0 g in distilled water), pH 7.8, 5% ß-Mercaptoethanol, 1 mM ethylene-diamine-tetra-acetic acid (EDTA), 1% Triton X-100 and 10% glycerol modified from (Ni et al. Citation1996) were added to 1 g of plant tissues and ground. One milli liter of the liquid extract was transferred to a microfuge tube (1.5 mL) and centrifuged at 14,000 × g for 15 min at 4°C in a refrigerated centrifuge (Heraeus: FresCo 21). The supernatant was pipetted into a new microfuge tube (1.5 mL) and centrifuged again for 10 min. Then, the clear supernatant was distributed in aliquots and stored at −80°C until used. The total protein content was evaluated using a bicinchoninic acid (BCA) assay kit (Pierce, product no. 2161297A).
2.3. Enzyme activity and protein assay
2.3.1. Superoxide dismutase (SOD, EC 1.15.1.1) assay
SOD activity was determined according to Giannopolitis and Ries (Citation1977). An assay mix (10 mL) was prepared just before use consisting of 0.5 mM xanthine (prepared fresh at each use), xanthine oxidase diluted with a potassium phosphate buffer (final concentration 0.32 U mL−1) prepared afresh at each use and cytochrome c prepared with phosphate buffer and stored at −20°C and on ice during use. Positive control SOD was prepared afresh by diluting a commercial source (Sigma S8409, from prepared bovine erythrocytes) with the buffer to a final concentration of 960 U mL−1. The assay mixture was sufficient for at least 32 wells of a micro-titer plate. Some wells were used as controls either by replacing the sample with control SOD or with buffer. Xanthine oxidase solution was added immediately before use to the assay mix. The plate was immediately transferred to the VersaMax plate reader (Molecular Devices, Sunnyvale, CA, USA), and absorbance monitored at 550 nm using a kinetic program for at least 5 min. All data are expressed as unit mL−1.
2.3.2. Catalase (CAT, EC 1.11.1.6) assay
CAT activity was determined according to Clairbone (Citation1985). Forty micromolar of H2O2 was added to 50 µL aliquots of the sample in each well of a 96-well plate and the plates were then incubated for 30 min at 37°C. After incubation, 50 µL of reaction buffer containing 100 µM Amplex Red reagent (Amplex Red stock solution was prepared at 10 mM in dimethyl sulfoxide and aliquots stored at −20°C) and 0.4 U mL−1 of horseradish peroxidase (HRP) (Sigma-Aldrich, Poole, UK) were added to each well. A CytoFluor II fluorescence microplate reader (PerSeptiveBiosystems, Framingham, USA) was used with an excitation at 540 nm and emission at 590 nm. CAT activity was calculated from a standard curve prepared using purified CAT (0–2000 U mL−1, Sigma, Poole, UK).
2.3.3. Glutathione peroxidase (GPX, EC 1.11.1.9) assay
GPX activity was determined according to Tappel (Citation1978). The assay mixture was prepared by mixing 1 mM nicotinamide adenine dinucleotide phosphate hydrogen, 100 U mL−1 glutathione reductase (GR; Sigma G3664), 200 mM glutathione peroxidase, 10 mM potassium cyanide with 5 mM potassium N-2-hydroxyethylpiperazine-N'-2-ethanesulfonic acid (4-(2-hydroxyethyl)-1-piperazineethanesulfonic acid) (containing 1 mM dipotassium EDTA). The assay mixture (290 µL) was added to each well of a 96-well plate, followed by 15 µL of sample before mixing. The reaction was started by adding 5 µL of 0.042% (w/w) H2O2 solution to each well. The rate of change of absorbance at 340 nm was measured for at least 10 min in a VersaMax plate reader (Molecular Devices, Sunnyvale, CA, USA). GPx activity was calculated in pmol mL−1 min−1.
2.3.4. Western blotting (immunoblot Analysis) for detection of COR15
Sodium dodecyl sulphate-polyacrylamide gel electrophoresis analysis was performed according to the modified method of (Laemmli Citation1970). Before conducting western blotting, a transfer buffer was prepared and chilled at 4°C for at least 2 hours. The transfer buffer contained 14.4 gL−1 glycine, 3.03 gL−1 Trizma base and 200 mL methanol and the volume was then made up to 1 L with d·H2O. The Bio-Rad procedure was followed for western blotting. The nitrocellulose membrane was soaked in methanol for 15 seconds, in H2O for 2 min and then in transfer buffer for at least 5 min. The sandwich of the western blotting was in the order of electrode sponge/filter paper/GEL page/membrane/filter paper/sponge + electrode. All were tightly clamped after making sure that no bubbles could be formed between the membrane and the gel. The sandwich was then submerged in the transfer buffer so that the gel was close to the negative electrode. The electrical field was 90 volt for 30–35 min. The membrane was then incubated in the blocking buffer consisting of Phosphate Buffer Saline (PBST + 0.05% Tween) + 0.05 milk for 1 hour at room temperature. After the blocking process, the membrane was washed two times with PBST for 5 min each. The membrane was then hybridized with the specific antibody for COR15a (Diluted at 1:1000) obtained from Michael Thomashow's lab (Michigan University, USA) in PBST solution and then put in the cold room (4°C) overnight. The membrane was then washed three times with PBST for 10 min each time. The membrane was incubated with the secondary antibody (abcam) that was diluted at 1:20,000 for 2 hours and then washed three times with PBST for 10 min each time. In order to visualize the bands of interest in the membrane, the chromogenic reaction was performed using a Bio-Rad kit. The reaction for each membrane consists of 1 mL from HRP-Color buffer, 9 mL ddH2O, 60 μL reagent B and 2 mL Reagent A. Finally, the membrane was incubated with the developing solution for 5–10 min and digital images were taken of the membrane.
3. Results
3.1. The activity of antioxidant enzymes in wheat under cold stress
The activity of antioxidant enzymes was readily manipulated by exogenous application of Mo under cold acclimation (). SOD enzyme activity increased dramatically in Mo-treated plants of both cultivars of wheat, but was higher for the winter wheat cultivar compared with the spring wheat cultivar. In the case of untreated plants, the pattern of SOD activity was fairly similar to the difference between the two cultivars in the case of Mo-treated plants.
Figure 1. Activity of SOD enzyme in two wheat varieties (winter wheat: cv. Claire and spring wheat: cv. Abu-Ghraib) as affected by Mo treatment (values are means ± SE).
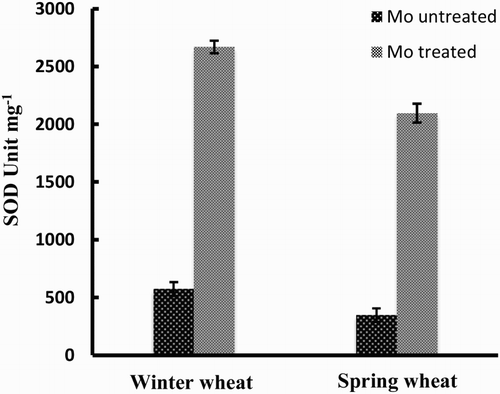
CAT also showed an increase in response to the addition of Mo () and the increase was similar in both winter and spring wheat cultivars.
Figure 2. Activity of CAT enzyme in two wheat varieties (winter wheat: cv. Claire and spring wheat: cv. Abu-Ghraib) as affected by Mo treatment (values are means ± SE).
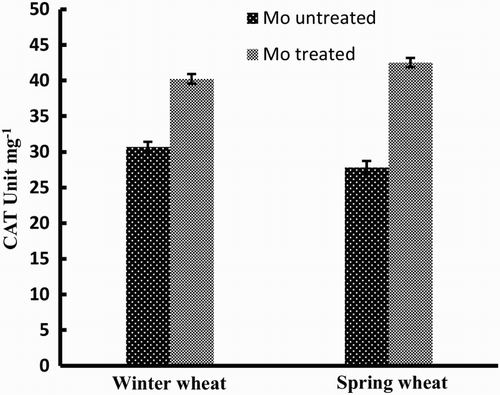
The activity of GPX was also increased dramatically in response to Mo application (). In contrast to SOD, spring wheat showed significantly higher activity of GPX in response to Mo-treated plants.
3.2. COR15a protein expression in wheat
COR15a protein expression was investigated in Mo-treated and untreated wheat of the two cultivars under acclimated (4°C) and non-acclimated (20°C) temperatures. No Western blot bands were detected in untreated plants of the two cultivars grown under warm conditions (20°C); however, there was COR15a protein expression when plants were acclimated (4°C) both under Mo-treated and untreated (). Importantly, COR15a was detected in Mo-treated plants under non-acclimating conditions in the winter wheat cultivar, but not in the spring wheat cultivar. However, the expression of COR15a protein in acclimated and treated plants was higher than its expression in Mo only treated plants.
4. Discussion
The production of ROS such as superoxide radicals (), singlet oxygen (1O2), hydrogen peroxide (H2O2) and hydroxyl radicals (•OH) in a plant is a general reaction in response to various abiotic stresses such as low temperature, drought and salt (Apostolova et al. Citation2006; Gill & Tuteja Citation2010). In order to protect themselves against these toxic oxygen intermediates, plant cells and their organelles such as chloroplasts, mitochondria and peroxisomes, employ antioxidant defense systems. SOD, CAT and GPX enzymes are considered among the major ROS scavengers in plants which can provide cells with a highly efficient machinery for detoxifying
and H2O2. In order to prevent or decrease oxidative injury under stress, plants have evolved several mechanisms including the enhanced expression of SOD, CAT and GPX during conditions that normally precede exposure to severe stress, the so-called acclimating environments. The balance between SOD and different antioxidant enzymes in cells is considered crucial in determining the steady-state level of
and H2O2 (Bowler et al. Citation1991; Asada Citation2006; Quan et al. Citation2008). The results presented in this paper confirmed the activity changes of SOD, CAT and GPX during low-temperature acclimation and to the application of Mo to wheat cultivars through seed priming.
An important role for ROS as signaling molecules is emerging (Quan et al. Citation2008), which suggests that they play a part in sensing the environment and may therefore act in regulating development and growth. However, these authors also reported that ROS are harmful when they are produced in excess, being only beneficial at low concentrations. The results here report that there is significant activity of SOD, CAT and GPX in Mo-treated plants under low-temperature acclimating conditions and also under Mo treatment in the absence of acclimation. There was a synergy between low temperature and Mo with increased activity of the three enzymes, evidencing that Mo is more effective when it is coupled with low-temperature acclimation. These results are consistent with the findings of Al-Issawi (Citation2013) and Sun et al. (Citation2006) where the application of Mo increased the frost tolerance in wheat under low temperature in comparison with either low temperature or Mo alone. Other reports in the literature have also found that exposure to low non-freezing temperature resulted in a decreased antioxidant activity in winter and spring wheat and it has been demonstrated that the deficiency of Mo can result in a lack of frost tolerance development in wheat (Yaneva et al. Citation1996; Apostolova et al. Citation2006; Apostolova et al. Citation2008). The winter wheat cultivar was considered more tolerant to freezing than spring wheat (Al-Issawi Citation2013) and together with the work presented here suggests that plants tolerant to low temperature are also associated with higher activities of antioxidant enzymes. The antioxidant enzymes were both induced in winter and spring wheat after acclimating, but they were only induced by Mo only in the winter wheat cultivar as found in other published work (Apostolova et al. Citation2008; Al-Issawi et al. Citation2013b).
Al-Issawi et al. (Citation2013b) found that Mo can influence the upregulation of the CBF transcription factor and this in turn can lead to COR upregulation, and this has been upheld in the current study. It is well documented that wheat can be acclimated and re-acclimated to low temperature while it is still in the vegetative phase of growth (Mahfoozi et al. Citation2001) and LT50 results presented by Al-Issawi et al. (Citation2013b) confirmed that winter wheat was more tolerant to freezing when acclimated than spring wheat. These findings combined with the results of the current study may offer an opportunity to suggest that Mo could be used to upregulate the CBF pathway and stimulate acclimation to frost in situations where acclimating temperatures may be marginal or absent. The results of this study suggest that in spring wheat cultivars, Cbf14 upregulation might not be followed by activation of COR genes, and the improvement in Cbf14 expression was possibly because of another pathway. Further studies are needed in order to clarify this response in spring wheat.
Whilst the results presented here do not fully explain the effects of Mo in frost tolerance, they do confirm that Mo can help to upregulate the important transcription factor of cold acclimation Cbf14 and thereby enhance frost tolerance in wheat. However, in the absence of acclimating temperatures, this increase did not lead to an increase in frost tolerance. When acclimating temperatures were imposed, Mo treatment enhanced the development of frost tolerance in both winter and spring cultivars, but not necessarily to the same degree. This may be linked to the inherent quantitative level of frost tolerance in the cultivars or some other mechanism. It has been reported that the constitutive overexpression of COR15 enhances the frost tolerance of the chloroplast and reduces the damage to photosystems in non-acclimating plants (Artus et al. Citation1996) and it is known that COR15a is transported into the chloroplast and accumulates in the stromal compartment (Shimamura et al. Citation2006) where it acts in a dehydrin-like manner.
Sun et al. (Citation2009) thought that Mo maintained the activity of the photosynthetic system through the regulation of the redox state in wheat chloroplasts (Takumi et al. Citation2003). Sun et al. (Citation2006) and Yu et al. (Citation2006) also found that low-temperature stress and deficiency of Mo decreased the rate of net photosynthesis (Pn), and the application of Mo enhanced frost tolerance as well as photosynthesis through the regulation of ABA-independent COR gene expression.
Further studies to investigate the use of different concentrations of Mo and different methods of application will be useful in order to see whether the Mo-enhancing effect can be optimized further. Furthermore, it would be useful to investigate the effect of Mo application to wheat genotypes at later growth stages to investigate whether it can be used to overcome the development-limited expression of frost resistance in wheat during floral development.
5. Conclusion
It can be concluded that exogenously applied Mo plays a part in increasing wheat tolerance to abiotic stresses via activation of the major antioxidant enzymes as well as by the upregulation of the expression of COR15a. Mo and low temperature act synergistically in the activation of plant defenses against freezing stress when imposed together on plants. Mo clearly stimulates the upregulation of antioxidant enzymes, which make an important contribution to low-temperature tolerance under experimental conditions. Some genetic variation between winter and spring wheat was found in the response to Mo application, especially in terms of COR15a expression and with less variation in terms of the antioxidant system. These results add to previously available data and provide good information for further studies of the role of Mo in abiotic stress. It can be recommended that the effect of Mo on the frost tolerance during flowering stages of wheat could be very beneficial to scientists and indeed growers threatened by late-season frosts in their crops.
Disclosure statement
No potential conflict of interest was reported by the authors.
References
- Ahmadi A, Mardeh AS, Poustini K, Jahromi ME. 2007. Influence of osmo and hydropriming on seed germination and seedling growth in wheat (Triticum aestivum L.) cultivars under different moisture and temperature conditions. Pak J Biol Sci. 10:4043–4049. doi: 10.3923/pjbs.2007.492.497
- Al-Issawi M, Rihan HZ, El-Sarkassy N, Fuller MP. 2013a. Frost hardiness expression and characterisation in wheat at ear emergence. J Agron Crop Sci. 199:66–74. doi: 10.1111/j.1439-037X.2012.00524.x
- Al-Issawi M, Rihan HZ, Woldie WA, Burchett S, Fuller MP. 2013b. Exogenous application of molybdenum affects the expression of CBF14 and the development of frost tolerance in wheat. Plant Physiol Biochem. 63:77–81. doi: 10.1016/j.plaphy.2012.11.010
- Al-Issawi MHE. 2013. The characterisation of the freezing damage response during flowering in European and Middle Eastern wheat cultivars (Triticum aestivum L) [PhD Doctorate]. University Of Plymouth.
- Allard F, Houde M, Kröl M, Ivanov A, Huner NPA, Sarhan F. 1998. Betaine improves freezing tolerance in wheat. Plant Cell Physiol. 39:1194–1202. doi: 10.1093/oxfordjournals.pcp.a029320
- Apostolova P, Yaneva I, Apostolova P, Yaneva I. 2006. Antioxidative defence in winter wheat plants during early cold acclimation. Gen Appl Plant Physiol. 101–108.
- Apostolova P, Yordanova R, Popoval L. 2008. Response of antioxidative defence system to low temperature stress in two wheat cultivars. Gen Appl Plant Physiol. 34(3–4):281–294.
- Artus NN, Uemura M, Steponkus PL, Gilmour SJ, Lin C, Thomashow MF. 1996. Constitutive expression of the cold-regulated Arabidopsis thaliana COR15a gene affects both chloroplast and protoplast freezing tolerance. Proc Natl Acad Sci. 93:13404–13409. doi: 10.1073/pnas.93.23.13404
- Asada K. 2006. Production and scavenging of reactive oxygen species in chloroplasts and their functions. Plant Physiol. 141:391–396. doi: 10.1104/pp.106.082040
- Baek K-H, Skinner DZ. 2003. Alteration of antioxidant enzyme gene expression during cold acclimation of near-isogenic wheat lines. Plant Sci. 165:1221–1227. doi: 10.1016/S0168-9452(03)00329-7
- Bowler C, Slooten L, Vandenbranden S, De Rycke R, Botterman J, Sybesma C, Van Montagu M, Inzé D. 1991. Manganese superoxide dismutase can reduce cellular damage mediated by oxygen radicals in transgenic plants. EMBO J. 10:1723–1732.
- Candan N, Tarhan L. 2003. The correlation between antioxidant enzyme activities and lipid peroxidation levels in Mentha pulegium organs grown in Ca2+, Mg2+, Cu2+, Zn2+ and Mn2+ stress conditions. Plant Sci. 165:769–776. doi: 10.1016/S0168-9452(03)00269-3
- Clairbone A. 1985. Catalase activity. Handbook of methods for oxygen radical research. Boca Raton: CRC Press; p. 283–284.
- De Gara L, De Pinto MC, Tommasi F. 2003. The antioxidant systems vis-à-vis reactive oxygen species during plant–pathogen interaction. Plant Physiol Biochem. 41:863–870. doi: 10.1016/S0981-9428(03)00135-9
- Fowler DB. 2007. Cold hardy wheat. Proceedings Saskatchewan Soil Conservation Association 19th Annual Conference; p. 33–38.
- Giannopolitis CN, Ries SK. 1977. Superoxide dismutases: II. Purification and quantitative relationship with water-soluble protein in seedlings. Plant Physiol. 59:315–318. doi: 10.1104/pp.59.2.315
- Gill SS, Tuteja N. 2010. Reactive oxygen species and antioxidant machinery in abiotic stress tolerance in crop plants. Plant Physiol Biochem. 48:909–930. doi: 10.1016/j.plaphy.2010.08.016
- Jafar MZ, Farooq M, Cheema MA, Afzal I, Basra SMA, Wahid MA, Aziz T, Shahid M. 2012. Improving the performance of wheat by seed priming under saline conditions. J Agron Crop Sci. 198:38–45. doi: 10.1111/j.1439-037X.2011.00485.x
- Kaiser BN, Gridley KL, Ngaire Brady J, Phillips T, Tyerman SD. 2005. The role of molybdenum in agricultural plant production. Ann Bot. 96:745–754. doi: 10.1093/aob/mci226
- Karabal E, Yücel M, Öktem HA. 2003. Antioxidant responses of tolerant and sensitive barley cultivars to boron toxicity. Plant Sci. 164:925–933. doi: 10.1016/S0168-9452(03)00067-0
- Laemmli UK. 1970. Cleavage of structural proteins during the assembly of the head of bacteriophage T4. Nature. 227:680–685. doi: 10.1038/227680a0
- Mahfoozi S, Limin AE, Fowler DB. 2001. Developmental regulation of low-temperature tolerance in winter wheat. Ann Bot. 87:751–757. doi: 10.1006/anbo.2001.1403
- Mendel RR, Hänsch R. 2002. Molybdoenzymes and molybdenum cofactor in plants. J Exp Bot. 53:1689–1698. doi: 10.1093/jxb/erf038
- Mittler R. 2002. Oxidative stress, antioxidants and stress tolerance. Trends Plant Sci. 7:405–410. doi: 10.1016/S1360-1385(02)02312-9
- Naidu BP. 1998. Separation of sugars, polyols, proline analogues, and betaines in stressed plant extracts by high performance liquid chromatography and quantification by ultra violet detection. Funct Plant Biol. 25:793–800.
- Ni M, Dehesh K, Tepperman JM, Quail PH. 1996. GT-2: in vivo transcriptional activation activity and definition of novel twin DNA binding domains with reciprocal target sequence selectivity. Plant Cell. 8:1041–1059. doi: 10.1105/tpc.8.6.1041
- Quan LJ, Zhang B, Shi WW, Li HY. 2008. Hydrogen peroxide in plants: a versatile molecule of the reactive oxygen species network. J Integr Plant Biol. 50:2–18. doi: 10.1111/j.1744-7909.2007.00599.x
- Quiles MAJ, López NI. 2004. Photoinhibition of photosystems I and II induced by exposure to high light intensity during oat plant growth: effects on the chloroplast NADH dehydrogenase complex. Plant Sci. 166:815–823. doi: 10.1016/j.plantsci.2003.11.025
- Scandalios JG. 1993. Oxygen stress and superoxide dismutases. Plant Physiol. 101:7–12.
- Shimamura C, Ohno R, Nakamura C, Takumi S. 2006. Improvement of freezing tolerance in tobacco plants expressing a cold-responsive and chloroplast-targeting protein WCOR15 of wheat. J Plant Physiol. 163:213–219. doi: 10.1016/j.jplph.2005.06.008
- Sun X, Hu C, Tan Q, Liu J, Liu H. 2009. Effects of molybdenum on expression of cold-responsive genes in abscisic acid (ABA)-dependent and ABA-independent pathways in winter wheat under low-temperature stress. Ann Bot. 104:345–356. doi: 10.1093/aob/mcp133
- Sun XC, Hu CX, Tan QL. 2006. Effects of molybdenum on antioxidative defense system and membrane lipid peroxidation in winter wheat under low temperature stress. Zhi Wu Sheng Li Yu Fen Zi Sheng Wu Xue Xue Bao. 32:175–182.
- Takumi S, Koike A, Nakata M, Kume S, Ohno R, Nakamura C. 2003. Cold-specific and light-stimulated expression of a wheat (Triticum aestivum L.) Cor gene Wcor15 encoding a chloroplast-targeted protein. J Exp Bot. 54:2265–2274. doi: 10.1093/jxb/erg247
- Tappel A. 1978. Glutathione peroxidase and hydroperoxides. Methods Enzymol. 52:506–513. doi: 10.1016/S0076-6879(78)52055-7
- Thomashow MF. 1999. Plant cold acclimation: freezing tolerance genes and regulatory mechanisms. Annu Rev Plant Physiol Plant Mol Biol. 50:571–599. doi: 10.1146/annurev.arplant.50.1.571
- Thomashow MF. 2010. Molecular basis of plant cold acclimation: insights gained from studying the CBF cold response pathway. Plant Physiol. 154:571–577. doi: 10.1104/pp.110.161794
- Uchida A, Jagendorf AT, Hibino T, Takabe T, Takabe T. 2002. Effects of hydrogen peroxide and nitric oxide on both salt and heat stress tolerance in rice. Plant Sci. 163:515–523. doi: 10.1016/S0168-9452(02)00159-0
- Uemura M, Warren G, Steponkus PL. 2003. Freezing sensitivity in the sfr4 mutant of arabidopsis is due to low sugar content and is manifested by loss of osmotic responsiveness. Plant Physiol. 131:1800–1807. doi: 10.1104/pp.102.013227
- Vaidyanathan H, Sivakumar P, Chakrabarty R, Thomas G. 2003. Scavenging of reactive oxygen species in NaCl-stressed rice (Oryza sativa L.) – differential response in salt-tolerant and sensitive varieties. Plant Sci. 165:1411–1418. doi: 10.1016/j.plantsci.2003.08.005
- Wang ZY, Tang YL, Zhang FS. 1999. Effect of molybdenum on growth and nitrate reductase activity of winter wheat seedlings as influenced by temperature and nitrogen treatments. J Plant Nutr. 22:387–395. doi: 10.1080/01904169909365636
- Waraich EA, Ahmad R, Halim A, Aziz T. 2012. Alleviation of temperature stress by nutrient management in crop plants: a review. J Soil Sci Plant Nutr. 12:221–244. doi: 10.4067/S0718-95162012000200003
- Yaneva I, Mäck G, Vunkova-Radeva R, Tischner R. 1996. Changes in nitrate reductase activity and the protective effect of molybdenum during cold stress in winter wheat grown on acid soil. J Plant Physiol. 149:211–216. doi: 10.1016/S0176-1617(96)80197-0
- Yu M, Hu C-X, Wang Y-H. 2006. Effects of molybdenum on the intermediates of chlorophyll biosynthesis in winter wheat cultivars under low temperature. Agr Sci China. 5:670–677. doi: 10.1016/S1671-2927(06)60109-0
- Zadoks JC, Chang TT, Konzak CF. 1974. A decimal code for the growth stages of cereals. Weed Res. 14:415–421. doi: 10.1111/j.1365-3180.1974.tb01084.x