ABSTRACT
Induction of defense-related enzymes, such as phenylalanine ammonia lyase (PAL), peroxidase (PO), polyphenol oxidase (PPO), superoxide dismutase (SOD) and catalase (CAT) due to bacterial antagonists viz., Pseudomonas fluorescens (Pf1) and Bacillus subtilis (EPCO16) and plant-derived lipoxygenase volatile compound hexanal, were studied in mango fruits against Lasiodiplodia theobromae causing stem-end rot disease. The results showed increased induction of all the defense-related enzymes in mango fruits 3–5 days after dipping treatment with combination of bacterial antagonists and hexanal when compared to untreated control treatment and treatment with fungicide carbendazim in storage condition. The increased activity was observed up to 3 days after treatment and thereafter declined. Further, increased expression of specific isoforms of PO, PPO, SOD and CAT were also observed in the treatment effect of P. fluorescens (0.5%) + hexanal (0.02%) treated fruits against L. theobromae. From the results obtained, it is inferred that due to the enhancement of defense-related enzymes via the phenylpropanoid pathway and due to secretion of secondary metabolites that would play significant role in hindering the pathogen quiescence and further invasion in mango fruits and thereby prevent the fruit rot.
Introduction
The mango (Mangifera indica L.), belongs to the Anacardiaceae family, is one of the world’s most important and reputable fruits and is widely known as the ‘King of fruits’ (Purseglove Citation1972). In India, mango is a very important cultural and religious symbol (Litz Citation2009). The area under mango cultivation and production have both increased manifold in the past three decades, but expected productivity is very low when compared to that of other countries. However, trade of mango has been limited by the perishable nature of this fruit which is susceptible to extremes of temperature and physical injury and due to postharvest losses of mango fruits accounting to 25–40% annually worldwide (Pathak Citation1980). Around 27 different genera of fungi are known to cause diseases in mango fruits (Prakash & Srivastava Citation1987) of which stem-end rot caused by Lasiodiplodia theobromae Pat. (Griffon & Maubl.) is the most important one (Johnson & Coates Citation1993).
This stem-end rot disease can render the fruits completely ineffective as it destroys the developed or developing fruits in storage condition. Stem-end rot affected trees produce completely unmarketable fruit by disrupting carbohydrate–sugar synthesis, accumulation and translocation. Even though the expanded use of chemical fungicides remains the main means of control, they are now being supplanted by non-chemical measures that are more specific towards the target pathogen and hence more eco-friendly. Potential antagonists, especially Pseudomonas fluorescens, Bacillus subtilis and plant-derived lipoxygenase volatile compound hexanal, are promising candidates as bio-protectants. Antagonists compete with pathogens for nutrients and space, and trigger induced immunity reaction in the fruit (Vivekananthan et al. Citation2004a). Hexanal, an inhibitor of phospholipase D, has been successfully applied for the pre- and postharvest treatment of fruits, vegetables and flowers (Tiwari & Paliyath Citation2011). The antimicrobial activity against decomposing microbial species of hexanal was already tested in model as well as in real systems (Gardini et al. Citation1997). The efficacy of hexanal as a metabolizable organic fungicide and the improvement of the aroma production by the interconversion to other aroma volatiles in minimally processed apples (Song et al. Citation1998). Indeed, it positively overwhelmed the shelf life by reducing the growth of natural occurring pathogen population during storage at 4°C and 15°C (Lanciotti et al. Citation2004). In addition, hexanal is commercially approved safer food additive by the U.S. food and drug administration program on everything added to food in the United States and has an ORL-MAM LD50 of 3700 mg kg−1 (Thavong et al. Citation2011).
Induced systemic resistance (ISR) is defined as an enhancement of the plant defensive capacity against a broad spectrum of pathogens and pests that is acquired after appropriate stimulation (Reddy Citation2013). Defense reaction occurs due to the accumulation of PR-proteins, phytoalexins, chalcone synthase, peroxidase (PO), polyphenol oxidase (PPO), phenylalanine ammonia lyase (PAL), β-1-3 glucanase, chitinase and total phenol (Ganeshamoorthi et al. Citation2008). It is well known that induction of systemic resistance is mechanism by which plant defense networks are activated by bacteria to fight against pathogen infection (Kloepper et al. Citation2004). Combined application of two or more biocontrol agents with preferred substrates, which in turned out to be more efficient than either of them alone (Narayanan et al. Citation2016). Therefore, use of compatible bacterial antagonist as consortia would emphasize more effective against postharvest diseases (Sangeetha et al. Citation2010). Induction of defense enzymes has been reported against several postharvest pathogens by bio-inoculants (Peeran et al. Citation2014; Wang et al. Citation2014; Guo et al. Citation2015). With this background the present study was taken up with the objective to unravel the induction of various defense-related genes encoding proteins incriminated in strengthening of host cell walls due to effective combination of lipoxygenase compound hexanal and bacterial biocontrol agent’s treatments in response to infection by L. theobromae.
Materials and methods
Pathogen source
The cultivar Neelum mango fruits exhibiting stem-end rot symptoms caused by L. theobromae were collected from Kanyakumari region of Tamil Nadu State, India. The pathogen was isolated as pure culture (L. theobromae KKNT fungal strain) and subcultured on the potato dextrose agar (PDA) medium supplemented with vitamin B complex (100 ppm) and incubated at room temperature (28 ± 2°C) for 5 days. After attaining the full radial mycelial growth of pathogen on plates immediately exposed to near UV light for sporulation. The suspension of conidia was prepared from 7–12-day-old culture, by inundating the plates with sterile water, vortexing the suspension and filtering through cheese cloth. Then the suspension was adjusted to 10−4 spores ml−1 using a hemocytometer.
Pathogenicity
Uniform-sized fruits were selected, washed under running tap water, blot dried and surface sterilized with 70% ethanol. The surface of the fruits were gently pricked with a sterile needle in 4–5 places and a 5 mm mycelial disc taken from actively growing culture was placed on the injured area. Which was further covered with a thin layer of sterilized moist cotton and the inoculated fruits were incubated in polythene covers to maintain humid conditions for induction of disease symptoms.
Bacterial antagonists and hexanal
Bacterial antagonist’s viz., P. fluorescens Pf1 isolated from black gram and B. subtilis EPCO16 isolated from cotton were supplied by Department of Plant Pathology, Tamil Nadu Agricultural University, Coimbatore. Lipoxygenase volatile compound hexanal was obtained from Department of Nano Science and Technology, Tamil Nadu Agricultural University, Coimbatore.
Compatibility assay
One milliliter of the bacterial cultures of P. fluorescens and B. subtilis was transferred separately to a 250 ml conical flask containing 100 ml of King’s B and nutrient broth amended with hexanal at three different minimum inhibitory concentrations (0.02%, 0.04% and 0.06%). Control was maintained without addition of hexanal in King’s B and nutrient broth. The flasks were incubated at 28 ± 2°C in a mechanical shaker. Three replications were maintained in each concentration. The optical density value of the culture broth was determined in UV–Visible spectrophotometer (Varian Cary 50 UV-VIS spectrophotometer) at 610 nm at regular intervals of 12 h and the observations were made over a period of 24, 48, 72 h interval, respectively.
In vitro screening of bio-inoculants
P. fluorescens Pf1 and B. subtilis EPCO16 were tested for their efficacy against L. theobromae by the dual culture technique (Dennis & Webster Citation1971) in PDA medium. Three replications were done with each bio-inoculants. The Petri plates were incubated at room temperature (28 ± 2°C) and the inhibition area was measured (Kishore et al. Citation2005).
Inoculation of pathogens and postharvest treatments
Healthy, even size and mature green mangoes of susceptible cv. Neelum were collected from mango growing area of Tamil Nadu state, India. The fruits were surface sterilized in 1% sodium hypochlorite solution for 15 min and rinsed with sterile water thrice. Six holes were made randomly at the equatorial region of fruit surface with a borer (3 mm diameter, 3 mm depth). Each hole was inoculated with 30 µl of Lasiodiplodia spore suspension using a micropipette. Control fruits were spotted by sterile deionized water. Then, the pathogen inoculated fruits were further treated by dipping with bacterial antagonists (2 × 109 CFU ml−1) in combination with and without hexanal (0.02%). Fruits treated with hexanal alone (0.02%), individual antagonists alone, fungicide carbendazim (0.1%) alone and untreated healthy control and untreated pathogen inoculated control were stored at room temperature (28 ± 2°C) and analyzed from 0–7 days after treatment. Each experiment was repeated thrice.
Sample collection and enzyme extraction
To study the induction of defense enzymes in response to postharvest pathogens in mango fruits, samples were collected from individual treatments starting from day 0 until day 7 at 48 h intervals. Samples were extracted by using suitable buffer at chilled condition. The homogenate was centrifuged for 20 min at 10,000 rpm. Protein extracts prepared from fruit samples were used for estimation of defense enzymes.
Assay of defense-related enzymes and compounds
Phenylalanine ammonia lyase
For preparation of the enzyme extract, 1 g of each peel and pulp of the fruit was homogenized in 5 ml of ice cold 0.1 M sodium borate buffer (pH 7.0) containing 1.4 mm of mercaptoethanol and 50 mg of insoluble polyvinyl pyrrolidone using chilled pestle and mortar. The homogenate was filtered through cheese cloth and the filtrate was centrifuged at 10,000 rpm for 20 min at 4°C. The supernatant was used for the assay of PAL activity. Assay of PAL activity was determined at 30°C by direct spectrophotometric measurement of the conversion of l-phenylalanine to trans-cinnamic acid at 290 nm (Dickerson et al. Citation1984). The reaction mixture contained 3.1 ml of 0.1 M sodium borate buffer (pH 8.8), 0.2 ml of the enzyme extract and 0.1 ml of 12 mM l-phenylalanine prepared in the same buffer. The blank was run by taking 3.1 ml of 0.1 M sodium borate buffer (pH 8.8) and 0.2 ml of the enzyme extract. The reaction mixture and the blank were incubated at 30°C for 30 min and the reaction was stopped by adding 0.1 ml of 3 N HCL. An extinction coefficient of 9630/mole cm−1 was determined for trans-cinnamic acid in 0.1 M borate buffer (pH 8.8) (Zucker Citation1965). The extinction coefficient was used to calculate the amount of product synthesized min−1. Phenylalanine ammonia lyase enzyme activity was expressed in ηmol of trans-cinnamic acid produced min−1 g−1 on fresh tissue.
Peroxidase
Using chilled pestle and mortar, 1 g of each peel and pulp sample from fruit was homogenized in 5 ml of ice cold 0.1 M sodium phosphate buffer (pH 6.5). To which a bit of polyvinyl pyrrolidone (PVP) was added. The homogenate was centrifuged at 10,000 rpm for 10 min at 4°C and the supernatant was used as the enzyme source for the assay of PO activity. PO (EC 1.11.1.7) activity was assayed using the modified method of Hartee (Citation1955). The reaction mixture consisting of 1.5 ml of 0.05 M pyrogallol, 0.5 ml of enzyme extract and 0.5 ml of 1% H2O2 was incubated at room temperature (28 ± 2°C). At the beginning of enzyme reaction, the absorbance of the assay mixture was set to zero at 420 nm in the UV–Visible spectrophotometer (Varian Cary 50 UV-VIS spectrophotometer) and the change in the optical density absorbance was recorded at 30 s intervals for 3 min. Boiled enzyme preparation served as control. The PO enzyme activity was expressed as change in the absorbance of the reaction mixture min−1 g−1 on fresh weight basis (Hammerschmidt et al. Citation1982).
Polyphenol oxidase
Enzyme extract was prepared as per the procedure given for the estimation of PO. Polyphenol oxidase (PPO) (EC 1.14.18.1) activity was assayed using a slight modification of the method described by Mayer et al. (Citation1965). The standard reaction mixture contained 1.5 ml of 0.1 M sodium phosphate buffer (pH 6.5), 0.5 ml of enzyme extract preparation, 200 µl of 0.01 M catechol. The reaction mixture was incubated at room temperature (28 ± 2°C). To initiate the reaction, 200 µl of 0.01 M catechol was added and the activity was expressed as changes in optical density absorbance were recorded at 3 s intervals for 2 min at 495 nm min−1 g−1 fresh weight basis.
Superoxide dismutase
For preparation of the enzyme extract, 1 g each peel and pulp sample from fruit was homogenized in 2 ml of pre cooled 0.2 M citrate phosphate buffer adjusted with pH 6.5 at chilled condition. The homogenate was centrifuged at 15,000 g at 4°C for 30 min. The supernatant served as enzyme source and SOD activity (EC 1.15.1.1) was determined as its ability to inhibit the photochemical reduction of NBT (Giannospolitis & Ries Citation1977). The assay sample mixture (3 ml) contained 50 mM sodium phosphate buffer (pH 7.8), 13 mM methionine, 0.1 mM EDTA, 75 µM NBT, 2 µM riboflavin and 100 µl of the enzyme extract and the riboflavin was added at the end. Tubes were shaken gently and placed under a 40 W fluorescent light at 25°C. The reaction was initiated and terminated by adjusting the duration of fluorescent light and dark, respectively. The absorbance at 560 nm was measured against identical non-illuminated in parallel to the sample mixture tubes for blank. Each assay extract was subtracted from the blank value and mathematical difference was then divided by blank and multiplied by 100 to obtain the percentage inhibition of NBT photo-reduction. The SOD activity was expressed in SOD units mg−1 tissue (50% NBT inhibition = 1 unit) (Belid El-Moshaty et al. Citation1993).
Catalase
Catalase (CAT) defense-related enzyme activity (EC 1.11.1.6) was detected spectrophotometrically as described by Chaparro-Giraldo et al. (Citation2000) using 3 ml sample mixture containing 100 mM potassium phosphate buffer (pH 7.5) and 2.5 mM H2O2 prepared immediately before use and 100 µl enzyme extract. The activity was quantified by monitoring the degradation of H2O2 using UV–Visible spectrophotometer at 240 nm over 1 min, against a treated plant extract free blank. The decrease in H2O2 was followed as the decline in optical density at 240 nm, activity was estimated using the extinction coefficient (ε240nm = 40 mM−1 cm−1) for H2O2 and expressed in μmol min−1 mg−1 of plant tissue.
Native polyacrylamide gel electrophoresis analysis
For analysis of isoform, fruit samples were obtained in the day of which exhibit maximum enzyme activities under colorimetric assay.
Peroxidase
To reveal the expression pattern of PO isoforms in response different treatments, native gel electrophoresis was executed. For native anionic polyacrylamide gel electrophoresis (PAGE), resolving gel of 8% and stacking gel of 4% were prepared. After completing electrophoresis, the hyaline gels were submerged incubated in the solution containing 0.15% benzidine dissolved in 6% NH4Cl for 30 min in dark condition. Then few drops of 30% H2O2 were added with gentle shaking till the reddish brown color bands appear. After staining, the gel was slowly washed with de-ionized water and photographed (Sindhu et al. Citation1984).
Polyphenol oxidase
Enzyme was extracted by homogenizing 1 g of fruit tissue in pre cooled 0.01 M potassium phosphate buffer (pH 7.0). The homogenate was centrifuged at 20,000 × g for 15 min at 4°C and the obtained supernatant was used as enzyme source. After native electrophoresis, the gel was bring to a chemical stasis for 30 min in 0.1% p-phenylenediamine diluted in 0.1 M potassium phosphate buffer (pH 7.0) followed by adding of 10 mM catechol in the prepared buffer. The addition of catechol was followed by a gentle shaking which resulted in appearance of dark brown discrete bands (Jayaraman et al. Citation1987).
Superoxide dismutase
Activity gel electrophoresis was carried out in 8% polyacrylamide gels for superoxide dismutase (SOD) activity staining. Electrophoresis running conditions and gels were prepared as described by Laemmli (Citation1970) lacking sodium dodecyl sulfate. Equal amounts of enzyme extract (40 µg) were loaded on to each lane. SOD activity was determined on native PAGE gels as described by Beauchamp and Fridovich (Citation1971) and modified by Azevedo et al. (Citation1998). The gels were rinsed in deionized water and incubated in the dark condition for 30 min at room temperature (28 ± 2°C) in an assay mixture containing 50 mM potassium phosphate buffer (pH 7.8), 1 mM EDTA, 0.05 mM riboflavin, 0.1 mM nitrobluetetrazolium and 0.3% (v/v) N,N,Ń,Ń-tetra methyl ethylene diamine (TEMED). At the end of this incubation period, the gels were rinsed with deionized water and replaced in fresh deionized water and exposed on a light illuminator for 5–10 min at room temperature until the development of colorless bands of SOD activity in a purple-stained gel was visible. The reaction was stopped by transferring the gels to freshly prepared 6% (v/v) acetic acid.
Catalase
Activity gel electrophoresis was experimented using 8% polyacrylamide gels for CAT activity staining. Electrophoresis conditions and gels were prepared as described by Laemmli (Citation1970) which lacking SDS. Equal amounts of enzyme extracts (40 µg) were loaded on to each lane. Gels were incubated in 0.003% H2O2 for 10 min and developed in a 1% (w/v) FeCl3 and 1% (w/v) K3Fe (CN6) solution for 10 min.
Statistical analysis
All the analyses were repeated once with similar results. The data were statistically analyzed by IRRISTAT package version 92 developed by Biometrics Unit of International Rice Research Institute, Philippines (Gomez & Gomez Citation1984). The treatments means were compared by DMRT analysis.
Results
The results of in vitro screening, colorimetric assay and isoform analysis revealed that combinatorial application of P. fluorescens (Pf1) and lipoxygenase hexanal significantly inhibited the pathogen growth and increased activities of defense-related enzymes viz., PAL, PO, PPO, SOD and CAT in the mango fruits against postharvest stem-end rot disease under storage condition.
Pathogenicity
The symptoms produced by artificial inoculation of L. theobromae showed typical black lesions starting from the stem end of the fruit and progressing towards the basal end. The lesions were expressed by the inoculated fruits 7 days after inoculation, which enlarged rapidly and the whole fruit turned black within 3–4 days ().
Evaluation of bacterial antagonist’s and its compatibility with hexanal
Both bacterial antagonists’s P. fluorescens Pf1 and B. subtilis EPCO16 tested significantly reduced the mean mycelial growth of L. theobromae. Among three different hexanal dilutions tested compatibility on antagonists, 0.06% concentration recorded higher inhibition of bacterial population, followed by 0.04% and 0.02% ().
Table 1. Population count of P. fluorescens (Pf1) in Kings-B broth and B. subtilis (EPCO16) in nutrient broth supplemented with different concentrations of hexanal.
Phenylalanine ammonia lyase
Significant increase in the PAL activity was observed in the fruits which were dipped in 0.5% P. fluorescens (Pf1) + 2% hexanal cross inoculated with L. theobromae (0.28) simultaneously followed by dipping in 0.5% P. fluorescens (Pf1) and dipping in 0.5% B. subtilis (EPCO16) + 2% hexanal (0.24) on 3rd day after treatment, when compared to healthy control (0.14). The increased activity was observed up to 3 days after treatment and thereafter declined (; ).
Figure 1. Influence of application of hexanal, biocontrol formulations and carbendazim on phenylalanine ammonia lyase (PAL) activity of mango fruit tissue challenged with L. theobromae.
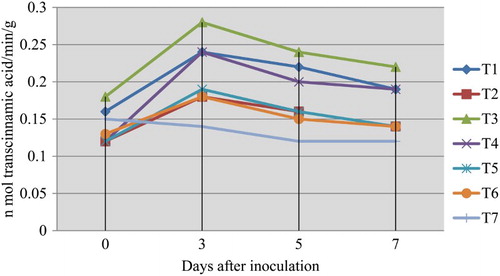
Table 2. Influence of bio-inoculants and carbendazim on PAL activity of mango fruit tissue challenged with L. theobromae.
Peroxidase
In the present colorimetric assay results revealed that the PO activity was significantly increased in the inoculated mango fruits dipped in 0.5% P. fluorescens (Pf1) + 2% hexanal followed by postharvest dipping of 0.5% P. fluorescens (Pf1) alone when compared to inoculated control upon challenge inoculated with L. theobromae. The increased activity was observed up to 3 days after treatment and thereafter gradually decreased (). Native PAGE analysis revealed the induction of two isoforms viz., PO1 and PO2 in inoculated and postharvest dipped mango fruits when compared to healthy control. In addition to two isoforms, expression of another isoforms was observed in T3 (postharvest dipped in 0.5% P. fluorescens (Pf1) + 2% hexanal) ().
Polyphenol oxidase
Significant induction of PPO activity was observed in the mango fruits challenged with the pathogen L. theobromae, and dipped in 0.5% P. fluorescens (Pf1) + 2% hexanal. The increased activity was observed up to 3 days after treatment and thereafter gradually decreased (). Native PAGE analysis revealed the induction of three isoforms viz., PPO1, PPO2 and PPO3 in the pre-inoculated and dipped mango fruits when compared to healthy control ().
Superoxide dismutase
SOD activity was increased in the mango fruits challenged with the L. theobromae, which were dipped in 0.5% P. fluorescens (Pf1) + 2% hexanal simultaneously followed by dipping in 0.5% P. fluorescens (Pf1) at 3rd day after treatment, when compared to healthy control. The increased activity was observed up to 3 days after treatment and thereafter declined (). Native PAGE analysis revealed the induction of two isoforms viz., SOD1 and SOD2 in the pre-inoculated and dipped mango fruits when compared to healthy control ().
Catalase
CAT enzyme activity was increased in the fruits challenged with the pathogen L. theobromae, which were dipped in 0.5% P. fluorescens (Pf1) simultaneously followed by dipping in 0.5% P. fluorescens (Pf1) + 2% hexanal at 3rd day after treatment, when compared to healthy control (). The increased activity was observed up to 3 days after treatment and thereafter declined. Native PAGE analysis revealed the induction of isoforms in the pre-inoculated and dipped mango fruits when compared to healthy control ().
Discussion
Incidence of postharvest decay and shelf-life reduction due to stem-end rot disease caused by L. theobromae play a significant role in diminishing the quality of export mango fruits apart extensive losses in native markets. Postharvest pathogens affect the fruit after harvesting or while fruit is still attached to the tree as quiescent infection. Moreover, the development of fungicidal resistance in pathogen strains (Ippolito & Nigro Citation2000) and the disposal of large quantities of fungicidal dipping solutions and lots could also affect the environment. Presently consumers and industries prefer purchasing mango fruits that is not treated with chemical pesticides, which is free from hazardous materials and safe for consumption. Therefore, it is mandatory need to find an eco-friendly alternative approach to postharvest disease control and shelf-life enhancement. Natural plant protecting and shelf-life-inducing compounds, such as bio-inoculants and their components, that show antimicrobial and shelf-life-enhancing activities, least mammalian toxicities and less environmental effects (Burt Citation2004).
Inducing the inherent defense pathways in plant system by prior application of biological inducer is a novel ecological-based plant protection approach. Bio-inoculants like bacterial antagonists can play a vital role in such approaches, and could emphasize the extended protection to the commodity through spatial competition, antibiosis and induction of defense pathways (Manikandan & Raguchander Citation2014). Combining different biocontrol agents can contribute to better control of pathogens (Spadaro & Gullino Citation2005). Recent study imply that combined application of bacterial antagonists like P. fluorescens, B. subtilis with hexanal drastically reduced the pathogen population in vitro and strengthen the cell wall structures resulting in restriction of pathogen infection (Ahemad & Kibret Citation2014; Wang et al. Citation2014). The recent approach is to design the non-chemical strategy by combining the positively compatible approaches to achieve effective disease control. In this regard, a combination of acibenzolar-s-methyl and P. fluorescens Pf2 provided better control of Ralstonia solanacearum on tomato than either agent applied singly (Abo-Elyousr et al. Citation2012). Application of fungal biocontrol agent and inorganic resistant inducer leads to increase resistance-related compounds in cotton (Abo-Elyousr et al. Citation2009). Hexanal is one of the most widespread fungicidal volatile compounds naturally occurring in the plant tissue and products (Thavong et al. Citation2011). Fan et al. (Citation2006) reported that spore viability of Penicillium expansum was reduced by 94% following exposure to hexanal vapor of 40 μmol l−1 for 24 h, compared with 20% at 9 μmol l−1. Hexanal vapor at 900 μl l−1 for 12 or 24 h or 450 μl l−1 for 24 h was sufficient to inhibit all tested pathogens while treatment with 200 μl l−1 for 24 h had only a limited effect (Song et al. Citation2007). The phospholipases synthesis which serve facilitate survival of the pathogen in vivo, invasion and dissemination throughout the host, expression of virulence traits and evasion of host immune defense mechanisms was inhibited by hexanal (Tiwari & Paliyath Citation2011; Karthika et al. Citation2015). In the present study maximum increase in defense-related enzyme content was achieved by combinatorial application of volatile organic compound hexanal and bacterial antagonist P. fluorescens. It also has been suggested by different researchers that bio-inoculants mixture should be applied to ensure adequate disease control under various conditions. This was most probably interconnected with antagonistic action of multiple components leads to rapid reduction in pathogenic inoculums over the surface of fructosphere. In addition to direct way of antagonism, biocontrol agents like Pseudomonas spp. (Peeran et al. Citation2014) and Bacillus spp. (Alvindia & Acda Citation2015) increased the activities of various defense-related enzymes and compounds in response to pathogen infection and there by inhibited the postharvest disease of perishable fruits through induction of defense-related compounds.
PAL is the first key enzyme involved in phenylpropanoid pathway in plant system and plays a key role in phenolics and phytoalexins biosynthesis (Bashan et al. Citation1985). In the present study, PAL activity was recorded in increased manner in the response of bio-inoculants dipped mango fruits challenged with the pathogen L. theobromae and reached maximum on the 3rd day after challenge inoculation. In the fruits inoculated with pathogen alone, the activity much reduced throughout the experimental period. Invasion of fruit peel tissues by the pathogen might have resulted in such decreased activity, whereas earlier increased activity of PAL due to bio-inoculants application might have prevented invasion of L. theobromae pathogen. An increase in the level of mRNAs encoding for PAL was observed in the early stages of interaction between bean roots and various rhizobacteria (Zdor & Anderson Citation1992). Though most of the bacterial antagonists showed increased activity of PAL, the P. fluorescens (Pf1) strain recorded higher activity of PAL (Ramamoorthy et al. Citation2002; Karthikeyan et al. Citation2007; Manikandan & Raguchander Citation2014). Induction of PAL by fluorescent pseudomonads (FP7) was reported in mango against Colletotrichum gloeosporioides (Vivekanandan et al. Citation2004b). Bacillus megaterium strain L8 enhances the activity of PAL and other defense enzymes (PO, PPO, SOD and CAT) against Pythium aphanidermatum on cucumber (Liang et al. Citation2011).
POs play a vital role in the regulation of plant cell elongation, phenol oxidation, cross-linking of polysaccharides, cross-linking of extension monomers, IAA oxidation, oxidation of hydroxyl-cinnamyl alcohols into free radical intermediates and wound healing (Vidhyasekaran et al. Citation1997). PO is associated with disease resistance in plants (Hammerschmidt & Kuc Citation1995) and enhanced levels of PO were noticed in fluorescent pseudomonads in plants such as sugarcane against Colletotrichum falcatum (Viswanathan & Samiyappan Citation1999), mango against C. gloeosporioides (Vivekanandan et al. Citation2004a, Citation2004b), chilies against Colletotrichum capsici (Bharathi et al. Citation2004), rose against Diplocarpon rosae (Karthikeyan et al. Citation2007). In the present study elevated activity and expression of more isoforms on mango fruits treated with combined application of P. fluorescens (0.5%) + hexanal (0.02%) challenged with L. theobromae. Increased PO activity in treated fruits might have contributed for resistance mechanism against pathogens. Contrarily, in the case of the inoculated control and carbendazim fungicide check, reduction in PO enzyme activity was observed from the beginning.
Polyphenoloxidase (tyrosinase) is a copper-containing oxidase enzyme responsible for browning of fruits that mainly oxidizes phenolics to highly toxic quinines and is involved in the terminal oxidation of diseased plant tissue and role in disease resistance. PPO usually accumulates upon wounding in plants. PPO can be induced via octadecanoid defense signal pathway (Constabel et al. Citation1995). Expression of new PPO isoforms was observed in P. fluorescens (Pf1) treated tomato plants challenged with F. oxysporum f. sp. lycopersici (Ramamoorthy et al. Citation2002). Saravanan et al. (Citation2003) reported that P. fluorescens induced twofold increases in PPO in banana roots against Fusarium wilt disease. Vivekananthan et al. (Citation2004b) reported that the PPO isoforms PPO1, PPO2 and PPO3 were induced in fruits dipped with the P. fluorescens (FP7) + Chitin amendment-based bio-inoculants treatments. In our study, PPO induction by both enzyme level and isoforms were higher in mango fruits treated with combined application of P. fluorescens and least concentration of hexanal. It is suggested that interaction of mango fruits with bio-inoculants triggered the induction of might PPO activity and that would have been responsible for restriction of pathogen.
Fungal infection induces the activity of SOD that catalyze the reaction 2O2− + 2H+ = O2 + H2O2 (Beauchamp & Fridovich Citation1971) and H2O2 causes degradation of membrane and many cellular macromolecules. To restrict its activity, an enzyme called PO and CAT gets activated simultaneously, which act as scavenging enzyme to destroy free radicals and H2O2 (Gangopadhyay et al. Citation1996). Plants produced array of active oxygen species (AOS) such as superoxide anion (O2−), hydrogen peroxide (H2O2) and hydroxyl radical (OH) as one of the earliest signaling mediated responses to attempted infection by pathogens (Grant & Loake Citation2000). In the current study, treatment of the mango fruits with combined bio-inoculants scavenging enzymes SOD and CAT activity was expressed in both quantitative and qualitative experiments and it might be responsible factor for the prevention of disease symptoms in mango. The observed results presented here exemplified that in mango fruits treated with P. fluorescens (Pf1) and hexanal, higher induction of defense-related enzymes, in both colorimetric and activity gel analysis was observed against the invasion of fungal pathogen L. theobromae. In the case of pathogen inoculated control alone, reduced activity of enzymes in colorimetric assay and few bands were observed in activity gel expression analysis. In respect of fungicide, carbendazim least expression of enzymes were noticed which clearly indicates that the application of bio-inoculants either alone or consortia eventually induced the defense enzymes for restriction of postharvest pathogens.
From the above evidences, it is evidenced that treatment with antagonist P. fluorescens and hexanal induced defense-related enzymes such as PO, PPO, PAL, SOD and CAT in mango fruits against stem-end rot pathogen L. theobromae. This offers a promising tool for enhancing the resistance against latent infection of postharvest pathogens in mango under storage conditions.
Disclosure statement
No potential conflict of interest was reported by the authors.
Additional information
Funding
References
- Abo-Elyousr KA, Hashem M, Ali E. 2009. Integrated control of cotton root rot disease by mixing fungal biocontrol agents and resistance inducers. Crop Prot. 28:295–301. doi:10.1016/j.cropro.2008.11.004.
- Abo-Elyousr KAM, Ibrahim YE, Balabel NM. 2012. Induction of disease defensive enzymes in response to treatment with acibenzolar-S-methyl (ASM) and Pseudomonas fluorescens Pf2 and inoculation with Ralstonia solanacearum race 3, biovar 2 (phylotype II). J Phytopathol. 160:382–389. doi:10.1111/j.1439–0434.2012.01915.x.
- Ahemad M, Kibret M. 2014. Mechanisms and applications of plant growth promoting rhizobacteria: current perspective. J King Saud Univ Sci. 26:1–20. doi:10.1016/j.jksus.2013.05.001.
- Alvindia DG, Acda MA. 2015. The antagonistic effect and mechanisms of Bacillus amyloliquefaciens DGA14 against anthracnose in mango cv. ‘Carabao.’ Biocontrol Sci Technol. 25:560–572. doi:10.1080/09583157.2014.996738.
- Azevedo RA, Alas RM, Smith RJ, Lea PJ. 1998. Response of antioxidant enzymes to transfer from elevated carbon di-oxide to air and ozone fumigation, in the leaves and roots of wild-type and a catalase-deficient mutant of barley. Physiol Plant Pathol. 104:280–292. doi:10.1034/j.1399-3054.1998.1040217.x.
- Bashan Y, Okon Y, Henis Y, 1985. Peroxidase, polyphenol oxidase and phenols in relation to resistance against Pseudomonas syringae pv. tomato in tomato. Can J Bot. 65:366–372. doi:10.1139/b87-047.
- Beauchamp CH, Fridovich I. 1971. Superoxide dismutase: improved assays and an assay applicable to acrylamide gels. Anal Biochem. 44:276–287. doi:10.1016/0003-2697(71)90370-8.
- Belid El-Moshaty FIB, Pike SM, Novacky AJ, Seghal OP. 1993. Lipid peroxidation and superoxide production in cowpea (Vigna unguiculata) leaves infected with tobacco ring spot virus or southern bean mosaic virus. Physiol Mol Plant P. 43:109–119. doi:10.1006/PMPP.1993.1044.
- Bharathi R, Vivekananthan R, Harish S, Ramanathan A, Samiyappan R. 2004. Rhizobacteria-based bio-formulations for the management of fruit rot infection in chillies. Crop Prot. 23:835–843. doi:10.1016/j.cropro.2004.01.007.
- Burt S. 2004. Essential oils: their antibacterial properties and potential applications in foods – a review. Int J Food Microbiol. 94:223–253. doi:10.1016/j.ijfoodmicro.2004.03.022.
- Chaparro-Giraldo A, Barata RM, Chabregas SM, Azevedo RA, Silva-Filho MC. 2000. Soybean leghemoglobin targeted to potato chloroplasts influences grow development of transgenic plants. Plant Cell Rep. 19:961–965. doi:10.1104/pp.91.3.889.
- Constabel CP, Bergery DR, Ryan CA. 1995. Systemin activates synthesis of wound-inducible tomato leaf polyphenol oxidase via the octadecanoid defense signaling pathways. Proc Natl Acad Sci U S A. 92:407–412. doi:10.1073/pnas.92.2.407.
- Dennis C, Webster J. 1971. Antagonistic properties of species-groups of Trichoderma II. Production of non-volatile antibiotics. Trans Br Mycol Soc. 57:41–48. doi: 10.1016/s0007-1536(71)80077-3.
- Dickerson DP, Pascholati SF, Hagerman AE, Butler LG, Nicholson RL. 1984. Phenylalanine ammonia-lyase and hydroxy cinnamate CoA ligase in maize mesocotyls inoculated with Helminthosporium maydis. Physiol Mol Plant Pathol. 25:111–123. doi:10.1016/0048-4059(84)90050-X.
- Fan L, Song J, Beaudry RM, Hildebrand PD. 2006. Effect of hexanal vapor on spore viability of Penicillium expansum, lesion development on whole apples and fruit volatile biosynthesis. J Food Sci. 71:M105–M109. doi:10.1111/j.1365-2621.2006.tb15632.x.
- Ganeshamoorthi P, Anand T, Prakasam V, Bharani M, Ragupathi N, Samiyappan R. 2008. Plant growth promoting rhizobacterial (PGPR) bioconsortia mediates induction of defense-related proteins against infection of root rot pathogen in mulberry plants. J Plant Interact. 3:233–244. doi:10.1080/17429140802088097.
- Gangopadhyay G, Sangita Basu BB, Mukherjee B, Gupta S. 1996. Salinity induced changes on peroxidases in Nicotiana tabaccum (var. Jayasri) callus cultures. Indian J Plant Physiol. 1:247–250.
- Gardini F, Lanciotti R, Caccioni DRL, Guerzoni ME. 1997. Antifungal activity of hexanal as dependent on its vapor pressure. J Agric Food Chem. 45:4297–4302. doi:10.1021/jf970347u.
- Giannospolitis CN, Ries SK. 1977. Superoxide dismutase. Plant Physiol. 59:309–314. doi:10.1104/pp.59.2.309.
- Gomez KA, Gomez AA. 1984. Statistical procedure for agricultural research. 2nd ed. New York (NY): Wiley-Interscience.
- Grant JJ, Loake GJ. 2000. Role of reactive oxygen intermediates and cognate redox signalling in disease resistance. Plant Physiol. 124:21–29. doi: 10.1104/pp.124.1.21.
- Guo M, Feng J, Zhang P, Jia L, Chen K. 2015. Postharvest treatment with trans-2-hexenal induced resistance against Botrytis cinerea in tomato fruit. Australas Plant Pathol. 44:121–128. doi:10.1007/s13313-014-0331-6.
- Hammerschmidt R, Kuc J. 1995. Induced resistance to disease in plants. 2nd ed. AZ Dordrecht: Springer. doi:10.1007/978-94-015-8420-3.
- Hammerschmidt R, Nuckles EM, Kuc, J. 1982. Association of enhanced peroxidase activity with induced systemic resistance of cucumber to Colletotrichum lagenarium. Physiol Plant Pathol. 20:73–83. doi:10.1016/0048-4059(82)90025-X.
- Hartee EF. 1955. Haematin compounds. In: Peach K, Tracy K, editors. Modern methods of plant analysis. New York (NY): Springer-Verlag; p. 207–245.
- Ippolito A, Nigro F. 2000. Impact of preharvest application of biological control agents on postharvest diseases of fresh fruits and vegetables. Crop Prot. 19:715–723. doi:10.1016/s0261-2194(00)00095-8.
- Jayaraman KS, Ramanuja MN, Vijayaraghavan PK, Vaidyanathan CS. 1987. Oxidative enzyme in pearl millet. Food Chem. 24:203–209. doi:10.1016/0308-8146(87)90152-X.
- Johnson G, Coates LM. 1993. Postharvest disease of mango. Post Harvest News Inf. 4:27N–34N.
- Karthika S, Kumar NBN, Gunasekaran K, Subramanian KS. 2015. Biosafety of nanoemulsion of hexanal to honey bees and natural enemies. Indian J Sci Technol. 8:1–7. doi:10.17485/ijst/2015/v8i30/52668.
- Karthikeyan M, Bhaskaran R, Mathiyazhagan S, Velazhahan R. 2007. Influence of phylloplane colonizing biocontrol agents on the black spot of rose caused by Diplocarpon rosae. J Plant Interact. 2:225–231. doi:10.1080/17429140701701071.
- Kishore GK, Pande S, Podile AR. 2005. Biological control of collar rot disease with broad-spectrum antifungal bacteria associated with groundnut. Can J Microbiol. 51:123–132. doi:10.1139/W04-119.
- Kloepper JW, Ryu CM, Zhang S. 2004. Induced systemic resistance and promotion of plant growth by Bacillus spp.. Phytopathology. 94:1259–1266. doi:10.1094/PHYTO.2004.94.11.1259.
- Laemmli UK. 1970. Cleavage of structural proteins during the assembly of the head of bacteriophage T4. Nature. 227:680–685. doi:10.1038/227680a0.
- Lanciotti R, Gianotti A, Patrignani F, Belletti N, Guerzoni M, Gardini F. 2004. Use of natural aroma compounds to improve shelf-life and safety of minimally processed fruits. Trends Food Sci Techol. 15:201–208. doi:10.1016/j.tifs.2003.10.004.
- Liang J, Rong-xiang TR, Hao Z, Wang L, Zhang X. 2011. Induction of resistance in cucumber against seedling damping-off by plant growth-promoting rhizobacteria (PGPR) Bacillus megaterium strain L8. Afr J Biotechnol. 10:6920–6927. doi:10.5897/AJB11.260.
- Litz RE. 2009. The mango: botany, production and uses. 2nd ed. Wallingford: CAB International. doi:10.1079/9781845934897.0000.
- Manikandan R, Raguchander T. 2014. Fusarium oxysporum f. sp. lycopersici retardation through induction of defensive response in tomato plants using a liquid formulation of Pseudomonas fluorescens (Pf1). Eur J Plant Pathol. 140:469–480. doi:10.1007/s10658-014-0481-y.
- Mayer AM, Harel E, Shaul RB. 1965. Assay of catechol oxidase a critical comparison of methods. Phytochemistry. 5:783–789. doi: 10.1016/S0031-9422(00)83660-2
- Narayanan P. Parthasarathy S. Rajalakshmi J. Arunkumar K. Vanitha S. 2016. Systemic elicitation of defense related enzymes suppressing Fusarium wilt in mulberry (Morus spp.). Afr J Microbiol Res. 10:813–819. doi:10.5897/AJMR2015.7900.
- Pathak VN. 1980. Diseases of fruit crops. 1st ed. New Delhi: Oxford & IBH Publishing Company.
- Peeran MF, Nagendran K, Karthikeyan G, Raguchander T, Prabakar K. 2014. Water in oil based PGPR formulation of Pseudomonas fluorescens (FP7) showed enhanced resistance against Colletotrichum musae. Crop Prot. 65:186–193. doi:10.1016/j.cropro.2014.07.010.
- Prakash O, Srivastava KC. 1987. Mango diseases and their management – a world review. 1st ed. New Delhi: Tommorow’s printers and publishers.
- Purseglove JW. 1972. Mangoes west of India. Acta Hortic. 24:107–174. doi:10.17660/ActaHortic.1972.24.30.
- Ramamoorthy V, Raguchander T, Samiyappan R. 2002. Induction of defense-related proteins in tomato roots treated with Pseudomonas fluorescens Pf1 and Fusarium oxysporum f. sp. lycopersici. Plant Soil. 239:55–68. doi:10.1023/A:1014904815352.
- Reddy PP. 2013. Recent advances in crop protection. 1st ed. New Delhi: Springer India. doi:10.1007/978-81-322-0723-8.
- Sangeetha G, Thangavelu R, Usha Rani S, Muthukumar A, Udayakumar R. 2010. Induction of systemic resistance by mixtures of antagonist bacteria for the management of crown rot complex on banana. Acta Physiol Plant. 32:1177–1187. doi:10.1007/s11738-010-0513-y.
- Saravanan T, Muthusamy M, Marimuthu T. 2003. Development of integrated approach to manage the fusarial wilt of banana. Crop Prot. 22:1117–1123. doi:10.1016/S0261-2194(03)00146-7.
- Sindhu JS, Ravi S, Minocha JL. 1984. Peroxidase isozyme patterns in primary trisomics of pearl millet. Theor Appl Genet. 68:179–182. doi:10.1007/BF00252335.
- Song J, Hildebrand PD, Fan L, Forney CF, Renderos WE, Campbell-Palmer L, Doucette C. 2007. Effect of hexanal vapor on the growth of postharvest pathogens and fruit decay. J. Food Sci. 72:108–112. doi:10.1111/j.1750-3841.2007.00341.x.
- Song J, Leepipattwit R, Deng W, Beaudry R. 1998. Hexanal vapor acts as residueless antifungal agent that enhances aroma biosynthesis in apple fruit. Acta Hortic. 464:219–224. doi:10.17660/actahortic.1998.464.31.
- Spadaro D, Gullino ML. 2005. Improving the efficacy of biocontrol agents against soilborne pathogens. Crop Prot. 24:601–613. doi:10.1016/j.cropro.2004.11.003.
- Thavong P, Archbold DD, Pankasemsuk T, Koslanund R. 2011. Hexanal vapours suppress spore germination, mycelial growth and fungal-derived cell wall degrading enzymes of postharvest pathogens of longan fruit. Chiang Mai J Sci. 38:139–150.
- Tiwari K, Paliyath G. 2011. Microarray analysis of ripening-regulated gene expression and its modulation by 1-MCP and hexanal. Plant Physiol Biochem. 49:329–340. doi:10.1016/j.plaphy.2011.01.007.
- Vidhyasekaran P, Sethuraman K, Rajappan K, Vasumathi K. 1997. Powder formulations of Pseudomonas fluorescens to control pigeonpea wilt. Biol Control. 8:166–171. doi:10.1006/bcon.1997.0511.
- Viswanathan R, Samiyappan R. 1999. Induction of systemic resistance by plant growth promoting rhizobacteria against red rot disease in sugarcane. Sugar Tech. 1:67–76. doi:10.1007/BF02945166.
- Vivekananthan R, Ravi M, Ramanathan A, Samiyappan R. 2004a. Lytic enzymes induced by Pseudomonas fluorescens and other biocontrol organisms mediate defense against the anthracnose pathogen in mango. World J Microbiol Biotechnol. 20:235–244. doi:10.1023/B:WIBI.0000023826.30426.f5.
- Vivekananthan R. Ravi M. Saravanakumar D. Kumar N. Parakasam V. Samiyappan R. 2004b. Microbially induced defense related proteins against postharvest anthracnose infection in mango. Crop Prot. 23:1061–1067. doi:10.1023/B:WIBI.0000023826.30426.f5.
- Wang X, Wang L, Wang J, Jin P, Liu H, Zheng Y. 2014. Bacillus cereus AR156-induced resistance to Colletotrichum acutatum is associated with priming of defense responses in loquat fruit. PLoS ONE. 9:1–8. doi:10.1371/journal.pone.0112494.
- Zdor RE, Anderson AJ. 1992. Influence of root colonizing bacteria on the defense responses in bean. Plant Soil. 140:99–107. doi:10.1007/BF00012811.
- Zucker M. 1965. Induction of phenylalanine deaminase by light and its reaction to chlorogenic acid synthesis in potato tuber tissue. Plant Physiol. 40:779–784. doi:10.1104/pp.40.5.779.