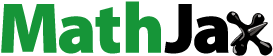
ABSTRACT
This study investigated a typical pine-oak mosaic mixed forest in the Qinling Mountains, China. In the sample plot, the population structure and spatial distribution of the stems were analyzed for the predominant species, to identify the mechanisms of species coexistence and successional trends of the forest. The population structures of all species were bimodally distributed, with young trees (DBH <1 cm) more abundant than older trees. The population structures of Quercus aliena var. acuteserrata was bimodal and rather continuous. However, Pinus tabuliformis and Pinus armandii were discontinuously bimodal, with distinct size deficiencies. Q. aliena var. acuteserrata trees were clumped throughout the plot, although those of P. tabuliformis and P. armandii were clumped at small scales. Notable negative spatial associations between Q. aliena var. acuteserrata and P. tabuliformis were found at almost scales. P. armandii and Q. aliena var. acuteserrata were negatively spatially associated at small scales but positively associated at large scales. Our findings suggest that interspecific competition gradually develops among the predominant tree species. The dynamics of the pine-oak mosaic mixed forest formed a mosaic distribution of uniformly mixed types, with the slow infiltration of Q. aliena var. acuteserrata populations that would eventually establish a pure stand.
Introduction
The spatial patterns of tree species are limited by various biological factors and environmental conditions in the community (Harms et al. Citation2001; Liu et al. Citation2014). These patterns are affected by wind, water flow, light intensity, animals and species characteristics such as type of regeneration, seed dispersal and unknown factors resulting from technical limitations of the aforementioned factors (Hutchinson Citation1953; Myster Citation2013; Duman et al. Citation2016).
The spatial distribution patterns of plants differ based on different processes and forces such as seed dispersal, intra- and interspecific competition, disturbance or environmental heterogeneity (Bonnet et al. Citation2005; Frazer et al. Citation2005), and the analysis from different spatial scales helps us to identify its underlying processes (Perry et al. Citation2002). The spatial patterns play key roles in the interactions between tree species in plant communities. These interactions affect the ecological processes of species dynamics, including growth, regeneration and mortality (Bieng et al. Citation2013). The spatial distribution pattern and species interaction of trees at different life stages are due to the mutual interaction among populational and environmental factors (Gray & He Citation2010; Guo et al. Citation2011). The spatial distribution pattern of trees differs among species, and the distribution pattern of a single species may vary with developmental stage and when individuals grow in diverse habitat (Zhang et al. Citation2012).
The Qinling Mountains with abundant species are a key region of biodiversity of global importance (Chai & Wang Citation2016a). Its geographical location in China is important and is influenced by climate and the distribution of vegetation (Wang et al. Citation2009). Pine–oak (Pinus–Quercus) mixed forests are dominant forest types in the mountains (Yu et al. Citation2013a; Zhang et al. Citation2015a), generally have high species richness and distinctive species compositions and play an irreplaceable role in biodiversity and ecosystemic function (Chai & Wang Citation2016b). How to optimize the resources of mixed forests, while at the same time preserving them, is therefore an important question for forest research. To reach this goal, the forestry managers need better knowledge and a more precise description of the factors that influence tree species growth in mixtures (Bieng et al. Citation2013). The spatial pattern is known to have a significant impact on species interactions which, in turn, impact ecological processes in plant communities (Mokany et al. Citation2008). Bieng et al. (Citation2013) investigated the effect of spatial pattern on productivity in mature mixed forests of sessile oak and Scots pine and showed that the productivity of both species is higher in an intimate mixture than in a patchy mixture, and intraspecific competition was more severe than interspecific competition. Some authors used numerous scale-dependent measures to describe spatial pattern of mixed forest. For example, Saei et al. (Citation2014) studied the spatial patterns of oak and almond trees with Ripley’s K function. The results showed that the distribution patterns of oak species to 235 m and almonds species to 115 m were statistically significant and clustered. The univariate O-ring function was used to analyze the spatial distribution patterns of a Pinus tabuliformis–Quercus wutaishanica mixed forest in Lingkong Mountain, China. The data indicated P. tabuliformis being dominant over Q. wutaishanica in the community and all tree populations being in relatively steady state (Huo et al. Citation2014). Therefore, to clarify the pattern and process of forest change and conjecture the trend, analyzing a spatial pattern of tree species is essential.
In our work, we study a typical pine–oak mixed forest (in a 1.2-ha plot) in the Qinling Mountains to determine the biological characteristics and interspecific relationships among the tree species in the community with spatial distribution and diameter class structure. Because of the strongly mixed nature of pine–oak mixed forests, which often produces low population densities of each tree species and random tree fall gaps caused by tree death (Condit et al. Citation2000), we expect clumped patterns in the pine–oak mixed forest and assumed the strong competition for resources and space among the predominant tree species. This study aims to illuminate the importance of the effects of interactions among species on species coexistence and developmental dynamics of the pine–oak mixed forest, which can provide a theoretical basis for designing forest developmental type and the restoration and reconstruction of vegetation, especially for planning forest cultivation.
Materials and methods
Study site
The study site was in the Huoditang forest region (108°21′–108°29′E, 33°18′–33°28′N) in the middle of Qinling Mountains, Shaanxi Province, northwestern China. The climate is transitional between northern subtropical and warm temperate. The elevation ranges from 1450 to 2470 m, and the average slope gradient is about 35°. The dominant soil is a brown forest soil with an average thickness of about 50 cm. The mean annual temperature ranges from 8°C to 10°C, with a maximum of 28.6°C in summer and a minimum of −9.5°C in winter. The average annual relative humidity is 77%, and the mean annual precipitation is 1000–1200 mm, with a mean annual evaporation is of 800–950 mm. The rainy season is during the summer, and snow falls between late October and early April (Yu et al. Citation2013b).
Field sampling
After the field reconnaissance, a 1.2-ha (100 × 120 m) plot with typical and light disturbance was established in the Huoditang forest region, which is an important habitat of pine–oak mixed forests in the Qinling Mountains (). The sample plot was further divided into 30 subplots (20 × 20 m), and the altitude, slope gradient and slope aspect were recorded for each subplot using global positioning system equipment and a compass. All woody plants in each subplot were measured, registered and identified, and trees with a diameter at breast height (DBH, at 1.3 m) ≥1 cm were marked. The species, heights, diameters, clear-bole heights, crown diameters and vitalities (levels 1, 2, 3 and 4 defined as strong, moderate, weak and dying wood, respectively) of the trees were determined, and the coordinates of the trees were recorded using an Electronic Total Station, with the southwestern corner of the plot as the origin. All seedlings (DBH <1 cm) in the sample plot were noted, including the species, basal diameters, heights, coordinates, origins (seedling stand or initiation), vitalities and ages. This work was conducted based on Forestry Standards ‘Observation Methodology for Long-term Forest Ecosystem Research’ of People’s Republic of China (LY/T 1952–2011).
Statistical analysis
All data acquired were used for statistical analysis. Diameter classes and age structures may differ, but the response to the environmental filtering is consistent under the same environmental conditions (Niu et al. Citation2008; Kanagaraj et al. Citation2011). We thus used the diameter to represent the age structure of the main tree species. The trees of each species were classified into three life stages based on DBH instead of growth stage: I, DBH <10 cm, saplings; II, 10 cm ≤ DBH < 20 cm, middle-aged trees and III, DBH ≥20 cm, adult trees (Kang et al. Citation2014; Chang et al. Citation2016).
Analysis of spatial pattern
Ripley’s K functions do not accurately represent information at small scales form circles due to the effects of scale accumulation, but the O-ring function uses rings instead of circles and has the advantage of isolating specific distance classes and effectively eliminating the accumulation effect. We thus used the univariate O-ring function (O(r)) to analyze the spatial distribution patterns of the main tree species and used the bivariate O-ring function (O12(r)) to analyze associations, including the interspecific associations of the main tree species, intraspecific associations at different life stages and interspecific associations between the same life stages of the main tree species.
The bivariate O-ring function (Wiegand & Moloney Citation2004) is calculated asHere
and
where
is the ring with radius r and width w centered in the ith point of pattern 1, n1 is the total number of points of pattern 1 in the study region, the operator Points [X] counts the points of pattern 2 in a region X, and the operator Area [X] determines the area of the region X. S(x, y) is an identifier for each cell (x, y), if a cell with coordinates (x, y) is inside the boundaries of the study region S(x, y) = 1, otherwise S(x, y) = 0 and with two additional marks P1(x, y) and P2(x, y) that give the number of points of pattern 1 and pattern 2 lying within the cell, respectively.
is a ring with radius r and width w around the ith point with coordinates (xi, yi) and z2 is the area of one cell.
The complete spatial randomness (CSR) null model should be carefully chosen before using O-ring statistics at the data-preprocessing stage to avoid misjudging the spatial patterns (Wiegand & Moloney Citation2004). CSR was used for the univariate O-ring functions when species distributions were not obviously aggregated; otherwise, a heterogeneous Poisson model was used. An antecedent condition null model was used for the bivariate O-ring functions due to the asymmetric competition among different species and life stages. Assuming that adults affect the growth of juveniles and other tree species affect the growth of the main tree species, whereas no effect exists.
A Monte-Carlo simulation was repeated 99 times for a 99% confidence interval for each process with the corresponding null model, and the threshold spatial scale was 0–50 m. O(r) values within, higher than and lower than the confidence interval indicated random dispersions or independent correlations, clumped spatial distributions or positive correlations and uniform spatial distributions or negative correlations, respectively.
Software
O-ring statistics were obtained using Programita 2008 and spatial arrangements were obtained using Origin 8.1. Figures were plotted using ArcGIS 10.0 and Origin 8.1.
Results
Species composition and population structure
Populational parameters varied among all tree species (). A total of 1696 free-standing live individuals (DBH ≥ 1 cm) were identified, belonging to 43 species, 31 genera and 19 families. Quercus aliena var. acuteserrata, P. tabuliformis and Pinus armandii were the most abundant, accounting for 48.53%, 12.03% and 7.72%, respectively, of the total number of free-standing individuals, so we identified them as the dominant species in the forest community.
Table 1. Species composition in a 1.2-ha plot in the pine–oak mosaic mixed forest.
The DBH classes of all species in the sample plot were bimodally distributed, with young trees (DBH <1 cm) more abundant than older trees, and 78.96% of the individuals were in the DBH class <10 cm (), indicating abundant recruitment beneath the forest canopy. Q. aliena var. acuteserrata, however, had a higher capacity for natural regeneration than P. tabuliformis and P. armandii. According to the DBH class of tree species, the distribution of Q. aliena var. acuteserrata DBH was bimodal and continuous, similar to that of all species in the sample plot. P. tabuliformis and P. armandii DBHs had similar, evident discontinuously bimodal distributions, with abundant smaller trees and distinct size deficiencies (2 cm < DBH <8 cm and 3 cm < DBH <10 cm, respectively), suggesting an adequate number of seedlings, but a lack of saplings under the canopy.
Spatial pattern of the main tree species
The analysis of spatial distribution for the three main tree species indicated segregation in the center of the plot (). Q. aliena var. acuteserrata had a strong aggregated distribution at most distances but become randomly distributed as distance increased (). The P. tabuliformis distribution was aggregated at 0–18 and 34–40 m and random at other scale intervals. P. armandii was randomly distributed at small scales of less than 2 m, and aggregated at scales of 5–7 m and larger scales of 11–16 m.
Figure 3. Spatial distribution of the three main tree species of different stages in a 1.2 ha (100 × 120 m) pine–oak mosaic mixed forest plot in the Qinling Mountains. QA, Quercus aliena var. acuteserrata; PT, Pinus tabuliformis; PA, Pinus armandii. Sapling, DBH <10 cm; middle-aged trees, 10 cm ≤ DBH < 20 cm; adult trees, DBH ≥20 cm.
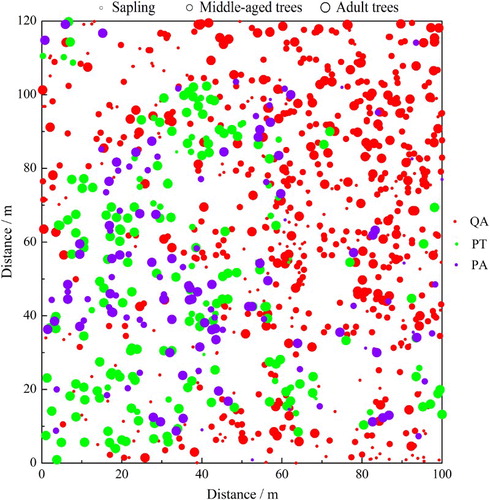
Intra- and interspecific spatial associations between the main tree species
Intra- and interspecific spatial associations were analyzed by bivariate O-ring functions ( and ). The intraspecific spatial associations among the life stages of the three main tree species indicated that middle-aged Q. aliena var. acuteserrata trees were positively associated with saplings and adult trees at most scales, middle-aged P. tabuliformis trees were positively associated with adult trees at small scales of 0–7 m and middle-aged P. armandii trees were positively associated with adult trees at scales of 5, 7–17 and 21–22 m. In addition, the result of interspecific spatial associations identified that Q. aliena var. acuteserrata had a negative association with P. tabuliformis at scales of 2–27 m. Q. aliena var. acuteserrata trees were associated negatively with P. armandii trees at 7–22 m and positively at larger scales of 44–46 m. P. tabuliformis trees were positively associated with P. armandii trees at scales of 3–4, 8–10 and 15–41 m.
Figure 5. Analysis of intraspecific spatial patterns of the three main tree species. Black lines indicate bivariate O-ring statistics (O12(r)); dashed lines indicate upper and lower limits of the 99% confidence intervals. QA, Quercus aliena var. acuteserrata; PT, Pinus tabuliformis; PA, Pinus armandii; I, saplings, DBH <10 cm; II, middle-aged trees, 10 cm ≤ DBH < 20 cm; III, adult trees, DBH ≥20 cm.
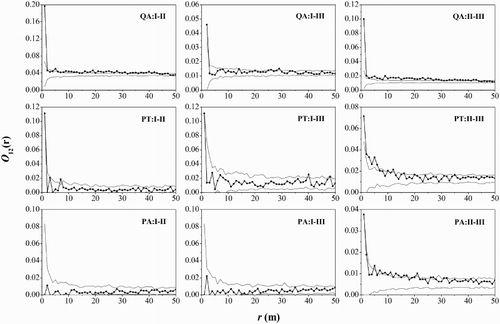
Figure 6. Interspecific spatial associations of the three main tree species. Black lines indicate bivariate O-ring statistics (O12(r)); dashed lines indicate upper and lower limits of the 99% confidence intervals. QA, Quercus aliena var. acuteserrata; PT, Pinus tabuliformis; PA, Pinus armandii.
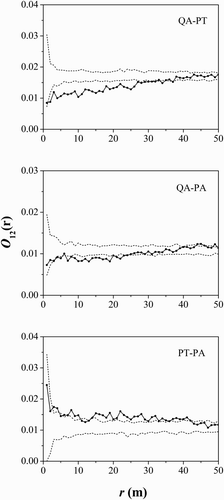
The result of interspecific spatial associations among different life stages of main tree species () showed that saplings of the three main species were uncorrelated at any scale. The interspecific associations between the middle-aged Q. aliena var. acuteserrata and P. tabuliformis trees were weaker negative association at scales of 8–9 and 14–16 m, and adult trees were negatively associated at a scale of 4–30 m. The middle-aged and adult Q. aliena var. acuteserrata trees were negatively associated with P. armandii trees at 8–14 and 10–13 m, respectively. The adult P. tabuliformis trees were positively associated with P. armandii trees at most scales (8–11, 15–16 and 18–42 m).
Figure 7. Interspecific spatial associations between the life stages of the main tree species. Black lines indicate bivariate O-ring statistics (O12(r)); dashed lines indicate upper and lower limits of the 99% confidence intervals. QA, Quercus aliena var. acuteserrata; PT, Pinus tabuliformis; PA, Pinus armandii; I, saplings, DBH <10 cm; II, middle-aged trees, 10 cm ≤ DBH < 20 cm; III, adult trees, DBH ≥20 cm.
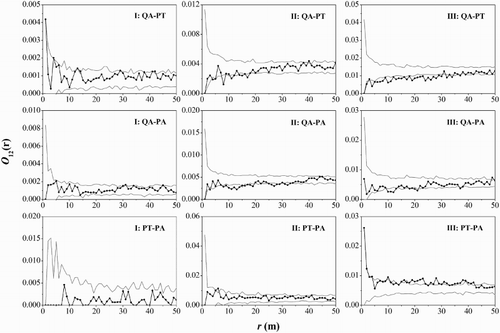
Discussion
Seedlings of the main tree species were abundant, but the numbers of later stages differed among the species
The population structure differs significantly among the main tree species in the plot. A distinct bottleneck at a DBH of 4 cm indicated that the Q. aliena var. acuteserrata seedlings had high mortality under the forest canopy (), which has also been reported previously (Wang & Gao Citation2005; Belden & Pallardy Citation2009; Yuan et al. Citation2011; Yu et al. Citation2013a; Huo et al. Citation2014). The Quercus species had a low survival rate between the seedling and sapling stages, because Q. aliena var. acuteserrata produced many seedlings, which suffered from intense interspecific competition for light, moisture and nutrients, thereby decreasing growth and increasing mortality during seedling growth. Q. aliena var. acuteserrata can therefore survive bottlenecks by strong regenerative sprouting to produce a stable population. The population structures of P. tabuliformis and P. armandii were similar and tended to decrease, although they could still maintain a stable populational distribution for decades. These findings were in part supported by other studies (Niu et al. Citation2008; Yuan et al. Citation2011). The distributions of these two Pinus species were discontinuously bimodal, but the frequencies of the DBH classes differed significantly. The distinct size deficiencies should be addressed by forest management. Appropriate measures of artificial management that thin the highly dense stands should therefore produce more space for growth and improve sapling regeneration.
The main tree species aggregated or were uniformly distributed
The spatial pattern for species that regenerate from seeds, such as oaks, depends on the distribution of the seeds (Calviño-Cancela Citation2002), which was created by seed dispersal ability (Tamme et al. Citation2010; Zhang et al. Citation2011). Oaks depend entirely on animals for the dispersal of their seeds, because they have not evolved any wind-dispersal structures (Jensen & Nielsen Citation1986). In contrast, most Pinus species, such as P. tabuliformis, have small seeds with samara-shaped wings for dispersal by wind (Wall Citation2003). Some Pinus species (e.g. P. armandii), however, rely on animals for seed dispersal (Chang & Wang Citation2012; Yu et al. Citation2013). Previous studies have suggested that Q. aliena var. acuteserrata and P. armandii have aggregated distributions at most scales (Yuan et al. Citation2011; Chang et al. Citation2016). Q. aliena var. acuteserrata has large seeds and produces fewer seeds in the present study than P. tabuliformis and P. armandii (). These seeds have high fat and tannin contents and are gathered by small rodents and cached in the soil (Chen et al. Citation2016). These characteristics reduce the distance of seed propagation, so the seeds were centered on the parent trees. P. tabuliformis individuals, however, tended to group together, and the pattern gradually changed from aggregated to random as the scale increased. Zhang et al. (Citation2009) found that P. tabuliformis in three plots in northern China mainly had an aggregated distribution, and the spatial pattern varied with spatial scale. The spatial distribution pattern depends on intraspecific spatial associations and varies with developmental stage (Xie et al. Citation1999; Kang et al. Citation2014). The intraspecific associations in our study indicated that the intraspecific positive correlation for Q. aliena var. acuteserrata gradually increased from the saplings to the adult trees. P. tabuliformis and P. armandii uniformly tended to have positive correlations between the middle-aged and adult individuals at small scales. This situation may be due to the DBH structures of the main species; the few saplings had high mortality and intense self-thinning during seedling growth. The aforementioned results indicated that the spatial pattern of a tree species depends on its biological characteristics, intraspecific associations and stand age.
Table 2. Comparison of biological and ecological attributes of the three main tree species in the pine–oak mosaic mixed forest.
Competition gradually increased with the development of the populations in the mosaic distributional area of the pine–oak mixed forest
Intra- and interspecific competition are crucial to understand the effect of mixture on forest dynamic (Kelty Citation2006). Interspecific competition was not strong early during succession, because resource supplies were adequate and there was almost minimal competition (Getzin et al. Citation2006). Interspecific associations gradually change as forests develop, increasing as the trees age, and determining the spatial distribution pattern (Liu et al. Citation2014). Interspecific competition occurs at small scales, and the effect of environmental heterogeneity (resources and space) dominates the distributional patterns of different species (Lin et al. Citation2011; Kang et al. Citation2014). Our analysis showed that negative association between Q. aliena var. acuteserrata and P. tabuliformis in our study was dominant at most scales, indicating that intense competition exists among the two main species. Q. aliena var. acuteserrata was negatively associated with P. armandii at small scales but was not correlated or was positively correlated as the scale increased. The spatial patterns of Q. aliena var. acutiserrata and P. armandii forests reported by Yuan et al. (Citation2011) were consistent with those in our study. These authors suggested that intraspecific competition was weakened and interspecific completion was intensified in the relatively stable forest communities. We also found that P. tabuliformis and P. armandii trees were positively correlated at most scales, implying that the species have similar habitat preferences or occupy different forest layers (Hai et al. Citation2014). The bivariate analyses of the life stages provided a closer view of the interspecific associations and indicated that the degree of interspecific competition between Q. aliena var. acuteserrata and P. tabuliformis gradually increased from the sapling to the adult stages. The interspecific correlation between P. armandii and Q. aliena var. acuteserrata had a similar trend, implying that the competition between adults was intense, because Q. aliena var. acuteserrata seedlings are shade tolerant () and occur obvious competition with light and space in the forest canopy when they become adult trees. P. tabuliformis and P. armandii showed an opposite trend, with similar habitat preferences.
Some studies have shown that P. tabuliformis and P. armandii are pioneer tree species during succession and are usually invaded by late-successional Q. aliena var. acuteserrata in mixed pine–oak forests (Gracia et al. Citation2002; Yu et al. Citation2014; Zhang et al. Citation2015b; Chai & Wang Citation2016b). Q. aliena var. acuteserrata have a disadvantage during regeneration and juvenile stages, but produces seeds with hard shells and high nutritional content that produce high-quality offspring with strong competitive abilities (Chai & Wang Citation2016b). Shade-tolerant Quercus seedlings also ensure that the Q. aliena var. acuteserrata juveniles can be established earlier than Pinus species in relatively dense old pine stands (Mosandl & Kleinert Citation1998). Q. aliena var. acuteserrata has high survival probability and a strong competitive advantage in the middle-aged and adult stages of development. The longevity of Q. aliena var. acuteserrata ensures its ultimate dominance, because long-lived tree species are able to maintain their share in a forest stand even if they only successfully reproduce once over many years (Warner & Chesson Citation1985). This feature therefore has direct implications for Q. aliena var. acuteserrata populations, which will gradually attain an advantageous position in pine–oak mosaic mixed forests. So we infer that the dynamics of these forests should thus develop a mosaic distribution of uniformly mixed types, with Q. aliena var. acuteserrata populations slowly infiltrating, and eventually producing pure stands.
The abundance of the three species varied from 131 to 823 individuals in the plot. A comparison of the population structure, spatial pattern and interspecific spatial associations suggested that differences in seed dispersal, competitive ability and the responses to stand conditions and light environments developed among these species. In summary, the comparative analysis of the main species at the forest scale illustrated both similarities and differences in the population structure and spatial pattern. The influences of life strategy, topography and light environment on the spatial distribution of these species have been ascertained, but these factors are still unable to account for the coexistence of these species.
Conclusion
The species composition of tree species was comparatively complex in the pine–oak mosaic mixed forest in the Qinling Mountains. Q. aliena var. acuteserrata, P. tabuliformis and P. armandii trees showed aggregated behavior in the successional stages. Intra- and interspecific competition occurred between the pines and oaks; the former may affect the natural regeneration of the main tree species in the study area, and the latter was obviously showed fierce between the adult individuals for resources and space. The pine–oak mixed forest in the Qinling Mountains is currently a stable community, but the pines are gradually being replaced by late-successional oak species in the long term. The dynamics of pine–oak mosaic mixed forests produce a mosaic distribution to uniformly mixed types, with Q. aliena var. acuteserrata populations slowly infiltrating, which will eventually reach pure stands. The pine–oak mixture types generally exhibits high species richness and a distinctive species composition (Stone Citation2006). Identifying the mechanism of coexistence and the forest dynamics of pine–oak mosaic mixed forests is therefore complicated and a long-term challenge. Further study of the relationships between species and other ecological factors should help to identify the mechanism of this coexistence.
Acknowledgements
We sincerely thank Qinling National Forest Ecosystem Research Station at Huoditang, Ningshaan County, Shaanxi Province and Xiaolongshan National Forest Ecosystem Research Station at Tianshui City, Gansu Province for support during our field investigation. We also thank Dr Xinping Zhang, Fei Yu and Di Kang provided helpful suggestions for analyzing data. Yue Pang, Defang Fan, Jibin Hu and Qiang Jin provided important help in the field.
Disclosure statement
No potential conflict of interest was reported by the authors.
Additional information
Funding
References
- Belden AC, Pallardy SG. 2009. Successional trends and apparent Acer saccharum regeneration failure in an oak-hickory forest in central Missouri, USA. Plant Ecol. 204(2):305–322. doi: 10.1007/s11258-009-9593-4
- Bieng MAN, Perot T, Coligny FD, Goreaud F. 2013. Spatial pattern of trees influences species productivity in a mature oak–pine mixed forest. Eur J Forest Res. 132(5–6):841–850. doi: 10.1007/s10342-013-0716-z
- Bonnet VH, Schoettle AW, Shepperd WD. 2005. Postfire environmental conditions influence the spatial pattern of regeneration for Pinus ponderosa. Can J Forest Res. 35(1):37–47. doi: 10.1139/x04-157
- Calviño-Cancela M. 2002. Spatial patterns of seed dispersal and seedling recruitment in Corema album (Empetraceae): the importance of unspecialized dispersers for regeneration. J Ecol. 90(5):775–784. doi: 10.1046/j.1365-2745.2002.00711.x
- Chai ZZ, Wang DX. 2016a. A comparison of species composition and community assemblage of secondary forests between the birch and pine–oak belts in the mid-altitude zone of the Qinling Mountains, China. Peer J. 4(l–2):1–23.
- Chai ZZ, Wang DX. 2016b. Environmental influences on the successful regeneration of pine–oak mixed forests in the Qinling Mountains, China. Scand J Forest Res. 31(4):368–381. doi: 10.1080/02827581.2015.1062912
- Chang W, Dang KL, Wu PH, Li MY. 2016. Spatial pattern of secondary Quercus aliena var. acuteserrata forests in the Qinling Mountains. Acta Ecol Sin. 36(4):1021–1029.
- Chang G, Wang KF. 2012. Effect of forest rodents on predation and dispersal of Pinus armandii seeds in Qinling Mountains. Acta Ecol Sin. 32(10):3177–3181. doi: 10.5846/stxb201104130487
- Chen XN, Zhang B, Chen YJ, Hou X, Wang J, Chang G. 2016. Effect of forest rodents on predation and dispersal of Castanea mollissima seeds and Quercus aliena seeds in South and North slope of Qingling Mountains. Acta Ecol Sin. 36(5):1303–1311. doi: 10.1016/j.chnaes.2016.06.008
- Condit R, Ashton PS, Baker P, Bunyavejchewin S, Gunatilleke S, Gunatilleke N, Hubbell SP, Foster RB, Itoh A, LaFrankie JV, et al. 2000. Spatial patterns in the distribution of tropical tree species. Science. 288(5470):1414–1418. doi: 10.1126/science.288.5470.1414
- Duman T, Trakhtenbrot A, Poggi D, Cassiani M, Katul GG. 2016. Dissipation intermittency increases long-distance dispersal of heavy particles in the canopy sublayer. Bound-Lay Meteorol. 159(1):41–68. doi: 10.1007/s10546-015-0112-y
- Frazer GW, Wulder MA, Niemann KO. 2005. Simulation and quantification of the fine-scale spatial pattern and heterogeneity of forest canopy structure: a lacunarity-based method designed for analysis of continuous canopy heights. Forest Ecol Manag. 214(1–3):65–90. doi: 10.1016/j.foreco.2005.03.056
- Getzin S, Dean C, He FL, Trofymow JA, Wiegand K, Wiegand T. 2006. Spatial patterns and competition of tree species in a Douglas-fir chronosequence on Vancouver Island. Ecography. 29(5):671–682. doi: 10.1111/j.2006.0906-7590.04675.x
- Gracia M, Retana J, Roig P. 2002. Mid-term successional patterns after fire of mixed pine–oak forests in NE Spain. Acta Oecol. 23(6):405–411. doi: 10.1016/S1146-609X(02)01169-4
- Gray L, He FL. 2010. Spatial point-pattern analysis for detecting density-dependent competition in a boreal chronosequence of Alberta. Forest Ecol Manag. 259(1):98–106. doi: 10.1016/j.foreco.2009.09.048
- Guo YX, Kang B, Li G, Wang DX, Yang GH, Wang DW. 2011. Species composition and point pattern analysis of standing trees in secondary Betula albo-sinensis forest in Xiaolongshan of west Qinling Mountains. Chin J Appl Ecol. 22(10):2574–2580.
- Hai NH, Wiegand K, Getzin S. 2014. Spatial distributions of tropical tree species in northern Vietnam under environmentally variable site conditions. J Forest Res. 25(2):257–268. doi: 10.1007/s11676-014-0457-y
- Harms KE, Condit R, Hubbell SP, Foster RB. 2001. Habitat associations of trees and shrubs in a 50-ha neotropical forest plot. J Ecol. 89(6):947–959. doi: 10.1111/j.1365-2745.2001.00615.x
- Huo MM, Guo DG, Zhang J, Wang ZM, Shangguan TL, Li RQ. 2014. Tree community characteristics and spatial patterns of the forms Pinus tabuliformis–Quercus wutaishanica in Lingkong Mountain. Acta Ecol Sin. 34(20):5925–5935.
- Hutchinson GE. 1953. The concept of pattern in ecology. Proc Acad Natl Sci Philadelphia. 105:1–12.
- Jensen TS, Nielsen OF. 1986. Rodents as seed dispersers in a heath – oak wood succession. Oecologia. 70(2):214–221. doi: 10.1007/BF00379242
- Kanagaraj R, Wiegand T, Comita LS, Huth A. 2011. Tropical tree species assemblages in topographical habitats change in time and with life stage. J Ecol. 99(6):1441–1452. doi: 10.1111/j.1365-2745.2011.01878.x
- Kang D, Guo YX, Ren CJ, Zhao FZ, Feng YZ, Han XH, Yang G. 2014. Population structure and spatial pattern of main tree species in secondary Betula platyphylla forest in Ziwuling Mountains, China. Sci Rep. 4:6873. doi:10.1038/srep06873.
- Kelty MJ. 2006. The role of species mixtures in plantation forestry. Forest Ecol Manag. 233(2–3):195–204. doi: 10.1016/j.foreco.2006.05.011
- Lin YC, Chang LW, Yang KC, Wang HH, Sun IF. 2011. Point patterns of tree distribution determined by habitat heterogeneity and dispersal limitation. Oecologia. 165(1):175–184. doi: 10.1007/s00442-010-1718-x
- Liu YY, Li FR, Jin GZ. 2014. Spatial patterns and associations of four species in an old-growth temperate forest. J Plant Interact. 9(1):745–753. doi: 10.1080/17429145.2014.925146
- Mokany K, Ash J, Roxburgh S. 2008. Effects of spatial aggregation on competition, complementarity and resource use. Austral Ecol. 33(3):261–270. doi: 10.1111/j.1442-9993.2007.01813.x
- Mosandl R, Kleinert A. 1998. Development of oaks (Quercus petraea (Matt.) Liebl.) emerged from bird-dispersed seeds under old-growth pine (Pinus silvestris L.) stands. Forest Ecol Manag. 106(1):35–44. doi: 10.1016/S0378-1127(97)00237-5
- Myster RW. 2013. The role of seed predation in the maintenance of the cross timbers ecotone of Oklahoma, USA. J Plant Interact. 8(2):134–139. doi: 10.1080/17429145.2012.707234
- Niu LL, Yu XX, Yue YJ. 2008. Spatial patterns of different age-class individuals in Pinus tabulaeformis forest in Songshan nature reserve of Beijing, China. Chin J Appl Ecol. 19(7):1414–1418.
- Perry JN, Liebhold AM, Rosenberg MS, Dungan J, Miriti M, Jakomulska A, Citron-Pousty S. 2002. Illustrations and guidelines for selecting statistical methods for quantifying spatial pattern in ecological data. Ecography. 25(5):578–600. doi: 10.1034/j.1600-0587.2002.250507.x
- Saei EK, Abedini S, Ghaderi N, Soltani A. 2014. Spatial distribution pattern of oak and almond trees on Chahartagh forest reservation site. Int J Biosci. 4(3):158–163.
- Stone R. 2006. Ecology: a threatened nature reserve breaks down Asian borders. Science. 313(5792):1379–1380. doi: 10.1126/science.313.5792.1379
- Tamme R, Hiiesalu I, Laanisto L, Szava-Kovats R, Partel M. 2010. Environmental heterogeneity, species diversity and coexistence at different spatial scales. J Veg Sci. 21(4):796–801.
- Wall SBV. 2003. Effects of seed size of wind-dispersed pines (Pinus) on secondary seed dispersal and the caching behavior of rodents. Oikos. 100(1):25–34. doi: 10.1034/j.1600-0706.2003.11973.x
- Wang ZL, Gao XM. 2005. The regeneration of Quercus aliena var. acuteserrata: acorn status, seedling pool and size structure. Acta Ecol Sin. 25(5):986–993.
- Wang DX, Lin YY, Lei RD, Yang T, Wang Q, Yong XH. 2009. Community composition and classification of natural forest of Chinese Pine (Pinus tabulaeformis Carr.) in Qinling Mountains. Acta Bot Boreali-Occident Sin. 29(5):867–873.
- Warner RR, Chesson PL. 1985. Coexistence mediated by recruitment fluctuations: a field guide to the storage effect. Am Nat. 125(6):769–787. doi: 10.1086/284379
- Wiegand T, Moloney KA. 2004. Rings, circles, and null-models for point pattern analysis in ecology. Oikos. 104(2):209–229. doi: 10.1111/j.0030-1299.2004.12497.x
- Xie ZQ, Chen WL, Liu ZY, Jiang MX. 1999. Spatial distribution pattern of cathaya argyrophylla population. Acta Bot Sin. 41(1):95–101.
- Yu F, Shi XX, Yi XF, Wang DX. 2013. Effects of relative abundance of Quercus mongolica acorns on five tree species seed dispersal in Xiaoxing’an Mountains, Northeast China. Chin J Appl Ecol. 24(6):1531–1535.
- Yu F, Wang DX, Shi XX, Chen LL, Huang QP, Song B. 2013a. Species composition and regeneration characteristics of main woody plant seedlings in a pine–oak mixed forest in Qinling Mountains. Acta Bot Boreali-Occident Sin. 33(3):592–598.
- Yu F, Wang DX, Shi XX, Yi XF, Huang QP, Hu YN. 2013b. Effects of environmental factors on tree seedling regeneration in a pine–oak mixed forest in the Qinling Mountains, China. J Mount Sci. 10(5):845–853. doi: 10.1007/s11629-013-2548-1
- Yu F, Wang DX, Yi XF, Shi XX, Huang YK, Zhang HW, Zhang XP. 2014. Does animal-mediated seed dispersal facilitate the formation of Pinus armandii–Quercus aliena var. acuteserrata forests? PLoS ONE. 9(2):396–401.
- Yuan ZL, Zhuang JJ, Zhu XL, Ye CY, Ye YZ. 2011. The species composition and spatial patterns of Quercus aliena var. acutiserrata–Pinus armandii forest in Baotianman natural reserve. J Henan Agric Univ. 45(4):37–41, 43–44,49.
- Zhang YT, Li JM, Chang SL, Li X, Lu JJ. 2012. Spatial distribution pattern of Picea schrenkiana population in the Middle Tianshan Mountains and the relationship with topographic attributes. J Arid Land. 4(4):457–468. doi: 10.3724/SP.J.1227.2012.00457
- Zhang LW, Mi XC, Shao HB, Ma KP. 2011. Strong plant–soil associations in a heterogeneous subtropical broad-leaved forest. Plant Soil. 347(1):211–220. doi: 10.1007/s11104-011-0839-2
- Zhang MX, Wang DX, Peng SL, Huang YK, Zhang GG. 2015a. Community stability analysis for the oak–pine mixed forest in Qinling Mountains. Acta Ecol Sin. 35(8):2564–2573.
- Zhang GG, Wang DX, Zhang MX, Liu WZ, Guo XL. 2015b. Interspecific association and correlation of main species in the succession process of pine oak forest community on the South-facing Slopes in Qinling Mountain. Acta Bot Boreal-Occident Sin. 35(8):1657–1668.
- Zhang Y, Zhao XH, Zhang CY. 2009. Spatial distribution pattern of Pinus tabulaeformis populations in North China. J Northeast Forest Univ. 37(11):43–45, 51.