ABSTRACT
Dark septate endophytes (DSEs), one of the most common fungal colonizers of roots, are considered to overlap in function with mycorrhizal fungi. However, there is little knowledge on the distribution and identity of DSEs in ‘non-mycorrhizal’ plants. In the current study, colonization and diversity of DSEs colonizing the roots of eight typically ‘non-mycorrhizal’ families were assessed. In total, 120 root samples of 31 plant species were all colonized by DSEs. Intensity of DSE colonization varied greatly among different plant species, with a range of 0.56–47.56%, 8.13% on average. Cladosporium, Cyphellophora and Phialophora were the dominant genera, with a relative abundance of more than 60% over a total of 90 isolates. Our results showed that diverse DSE species colonized the roots of ‘non-mycorrhizal’ plants, especially they were more common in degraded mine tailings than in the undisturbed site, but their integral roles to the functional roots are in need of further experimental demonstration.
Introduction
In natural ecosystems, the plant roots are regarded as a large reservoir of many types of mutualistic microorganisms, including bacteria, archaea, fungi, collectively designated as the plant–microbiome or phytomicrobiome (Quiza et al. Citation2015), the most common of which are the arbuscular mycorrhizal (AM) fungi, formed symbiotic association intimately with the roots of more than 80% terrestrial plants, growing inter- and intracellularly in the root cortex (Smith & Read Citation2008). Both fossil evidence and molecular data indicate that this widespread AM association existed in the earliest land plants, and may have enabled plants to colonize land (Bravo et al. Citation2016; Remy et al. Citation1994; Wang et al. Citation2010). However, it is found interestingly that some plants, for example, most of the plants of Caryophyllaceae, Brassicaceae, Chenopodiaceae, Juncaceae and Polygonaceae are insusceptible to infection with mycorrhizal fungi (Newman & Reddell Citation1987), and defined as the ‘possibly non-mycorrhizal or rarely mycorrhizal’ plants, which do not usually form mycorrhizas of any of the well-recognized kinds (Smith & Read Citation2008). But, one must keep in mind that some families have both mycorrhizal and non-mycorrhizal members and, even in predominantly non-mycorrhizal groups, some mycorrhizal species do occur. For example, Miller et al. (Citation1999) survey the mycorrhizal status of the genus Carex (belonging to the generally considered ‘non-mycorrhizal’ Cyperaceae), and find both the obligately non-mycorrhizal species and AM members of Carex, and find that mycorrhizal colonization appears to occur in response to many factors, both environmental and phylogenetic.
Increasing evidence reveals that a miscellaneous group of ascomycetous anamorphic fungi called dark septate endophytes (DSEs), a term broadly classified as conidial and sterile septate fungal endophytes with diverse morphological features such as dark septate hyphae and microsclerotia, dominantly colonize root tissues intracellularly and intercellularly from the tropics to arctic and alpine habitats (Jumpponen & Trappe Citation1998; Vohník et al. Citation2015; Casazza et al. Citation2017; Hewitt et al. Citation2017). Even under some extreme environments, for example, old slag heaps resulting from the processing of zinc ores, DSE gradually becomes the most prevailing root colonizers with the decreasing AM colonization at increasing environmental stresses (Deram et al. Citation2008, Citation2011). Recently, Huusko et al. (Citation2016) also report a shift of fungal community from an AM-dominated to a DSE-dominated colonization in Deschampsia flexuosa roots along a postglacial primary successional land uplift gradient. More importantly, the increasing evidence supports the conclusion that the prevalence of DSEs in extreme habitats functionally and ecologically overlaps with mycorrhizal fungi. For example, with regard to the alleged roles in protecting plants from abiotic and biotic stresses via habitat adapted symbiosis, the DSE can enhance nutrient availability to plants, and contribute to plant stress resistance to pathogens, metal pollution, etc. (Jumpponen Citation2001; Mandyam & Jumpponen Citation2014; Newsham Citation2011; Wang et al. Citation2016).
Although there has been a continual increase of interest in DSE fungi (Card et al. Citation2016; Monica et al. Citation2015; Jumpponen & Trappe Citation1998; Newsham Citation2011), there is a need for studies on the characterization of DSE in the different ‘non-mycorrhizal’ species or families, which may have evolved compensatory mechanisms of DSE for the effective nutrient uptake. In the present study, 120 root samples of 31 plant species belonging to 8 ‘non-mycorrhizal’ families were studied in Kunming Changchong Mountain (CM) and in Niubahuang Sn/Pb/Zn/ mine tailings pond in Gejiu, in Yunnan Province, Southwest (SW) China. The colonization characteristics and diversity of DSE colonizing the roots of these typically ‘usually non-mycorrhizal or rarely mycorrhizal plant families’ were assessed and the ecological roles of DSE were also discussed.
Materials and methods
Sample sites and sample collection
In total, two sample sites including a natural ecosystem of CM in Kunming city, and an old abandoned Niubahuang Sn/Pb/Zn mine tailings pond in Gejiu city, were selected in Yunnan province, SW China (). CM is located at 102°42′31″ E, 25°13′11″ N, in Kunming city, where has low-latitude monsoon climate with sunlight of 2198 h, frost-free period of 230 days, annual mean temperature of 14.9°C, and mean annual precipitation of about 1190.3 mm, nearly 88% occurring from May to October. The forest is co-dominated by warm temperate coniferous, semi-humid evergreen broadleaf and mixed conifer–broadleaf trees (Zhang Citation2011). With a distance of approximately 220 km, Niubahuang Sn/Pb/Zn mine tailings pond (NTP, 103°10′42.2″ E, 23°17′41.7″ N) is an old abandoned polymetallic tin ore tailings pond, near to the world-class tin production region, known as the super large tin copper polymetallic mining area (∼300 Mt of Sn, and ∼700 Mt of Cu, Pb, Zn, Sb, Ag, Mo, Au and Bi) (Cheng et al. Citation2010). The climate of the area belongs to tropical and subtropical monsoon climate with mean annual temperature of 15.9°C and average annual precipitation of 1069.7 mm. The native vegetation is rare for the massive deforestation and rapid pollution until now in NTP, scattered with sparse Pinus yunnanensis, shrubs and herbs.
Figure 1. Geographic localization of the two collecting sites, Kunming CM and Gejiu Niubahuang Sn/Pb/Zn mine tailings pond (NTP), in SW China.
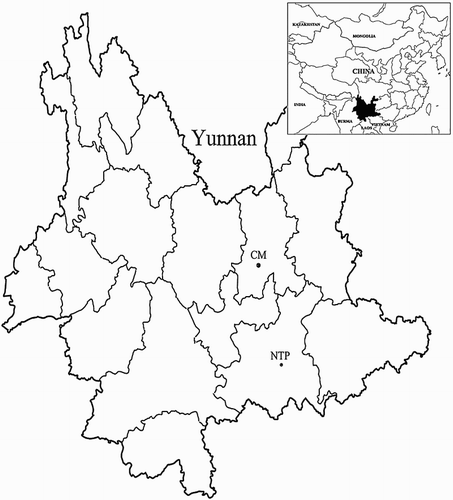
In total, 120 root samples of 31 plant species belonging to 8 ‘non-mycorrhizal’ families were collected from the two samples sites. Roots and rhizosphere soils (5–25 cm depth) of each plant species with at least 3 replicates were collected randomly, including 20 plants species of 8 families from CM and 15 plants species of 7 families from NTP, respectively. The sampled roots were immediately brought back to the laboratory and subjected to anatomical observation of DSE colonization and the isolation of fungal endophytes described as follows.
DSE colonization
For anatomical observation of DSE colonization, the roots (with tips) were firstly washed thoroughly under tap water, and then cleared in 10% (w/v) KOH at 90°C in a water bath for 1 hour, and then stained with acid fuchsin (Berch & Kendrick Citation1982). Compared with the red-stained AM fungal structures (i.e. vesicles, arbuscules, hyphae, etc.), the dark and septate hyphae and intracellular microsclerotia of DSE were observed without visually stained color. For each sample, at least eight 2-cm-long root fragments were chosen for examination under a compound-light microscope (OLYMPUS-BX31) at 400× magnification. The colonization status, including the DSE hyphae or microsclerotia, was observed. The total root colonization intensity of DSE (DSE%), including the intensity by intraradical DSE hyphae (H%) and intracellular microsclerotia (M%), were estimated using the magnified intersection method by checking over 200 intersections for each sample (Massicotte et al. Citation2005; McGonigle et al. Citation1990).
DSE isolation
Fresh root samples were carefully cleaned under the running tap water to remove soil particles and sterilized with 75% ethanol for 5 min and 10% NaClO for 5 min, respectively. Then, the sterilized roots were rinsed three times in sterile distilled water, finally air-dried under sterile conditions, cut into 3–5 mm pieces and cultured on the both malt extract agar (MEA) and potato dextrose agar (PDA) isolation media described as follows (Arnold et al. Citation2000). Over 80 root pieces of each plant species were deposited on 1% MEA (malt extract 20 g, peptone 5 g, agar 18 g, and water 1000 mL, pH 6.4) and PDA (potato extract 200 g, dextrose 20 g, agar 18 g and water 1000 mL) at 28°C in the dark for 30 days, respectively. Streptomycin (100 mg L−1) and ampicillin (100 mg L−1) were also added to the above two isolation media to avoid bacterial contamination (Silvani et al. Citation2008). During cultivation, root samples were checked every other day, and only new melanized fungal colonies growing out of the plant root tissues were transferred to PDA slants. The isolation ratio was calculated as the total number of endophytic fungal isolates divided by the total number of DSE-colonized plant species. Also, the relative abundance (RA%) was calculated as the number of fungal isolates in one clade divided by the total number of all fungal isolates (Li et al. Citation2012).
DSE identification
For the identification of fungi, phylogenetic analyses of fungal internal transcribed spacer (ITS) rDNA sequences (ITS1-5.8S-ITS2) from all fungal isolates were used. Initially, the total genomic DNA of each DSE strain was extracted and purified using the cetyltrimethyl ammonium bromide (CTAB) method (Sharrock et al. Citation2004). The primer set ITS1-F/ITS4 was used to amplify the ITS1-5.8S-ITS2 regions (White et al. Citation1990). The PCR reactions were carried out in a total volume of 50 µL containing 39 µL sterile distilled water, 5 µL 10× PCR buffer (containing Mg2+), 2 µL dNTP, 1 µL of each 10 µM primers, 0.25 µL Taq DNA polymerase (5U µL−1, Takara Biotechnology Co. LTD., Japan), and 1 µL DNA template. The amplifications were performed on a Mastercycler (Eppendorf, Germany) under the following conditions: an initial pre-denaturing at 95°C for 4 min, 32 cycles at 94°C for 45 s, 55°C for 45 s, 72°C for 1 min, and a final extension at 72°C for 5 min. The amplification products were electrophoresed in 1% agarose gel and purified using Gel Extraction Kit (UNIQ-10, Sangong, Shanghai, China), then sequenced on an ABI 3730 DNA Analyzer (Applied Biosystems, Inc.). Subsequently, a dataset including the ITS sequences and the similar sequences retrieved from GenBank using the NCBI BLAST program (Altschul et al. Citation1997) was aligned by the MegAlign program of Lasergene Package (DNAStar Inc., Madison, WI, USA) and subjected to a neighbor-joining (NJ) analysis using PAUP*4.0b10 (Swofford Citation2002) with support values estimated using 1000 bootstrap. Modeltest 3.06 was used to determine the evolutionary model that best fit the dataset (Posada & Crandall Citation1998). The ITS gene sequences reported in this study have been deposited in GenBank under accession numbers KY987519–KY987540. Only representative sequences were deposited.
Data analysis
The statistical analysis was performed using SPSS software (version 22.0). The assays were carried out in at least three replicates and the results for colonization intensity of DSE hyphae (H%), microsclerotia (M%) and the total colonization intensity of DSE (DSE%) are expressed as mean values ± standard deviations (n ≥ 3). Then, the data on the DSE colonization intensities including H%, M% and DSE% were subjected to independent T-test to evaluate the differences between all the samples from two sample sites. The differences of the totally average DSE colonization intensity were considered statistically significance if the p value was less than .05.
Results
DSE colonization
Of the 31 species analyzed, only 16.1% were colonized by AM fungi (5 plant species belonging to Polygonaceae and Commelinaceae families). On the contrary, all root samples were found to be colonized by typical ‘dark and septate’ endophytic fungi (), and various morphological structures of DSEs were observed (). Under compound-light microscope, sparse melanized or slightly dark running hyphae and a variety of morphological microsclerotia of DSE, such as band-shaped, peloton-like, brain-like, colonized the epidermis and cortex cells of most roots, including the most commonly encountered plant species in Caryophyllaceae and Polygonaceae surveyed (). The total colonization intensity of DSE (DSE%) showed the minimum value (0.56%) in the roots of Achyranthes bidentata from CM, and peaked in Polygonum capitatum from Niubahuang Sn/Pb/Zn mine tailings pond with the maximum colonization intensity of 47.56% (), and there was an average colonization of 8.13% ± 10.68% in total. Interestingly, we noted that 53.33% of the ‘non-mycorrhizal’ families (8/15 families) were colonized by DSE microsclerotia in Gejiu NTP site, but this value was reduced to 30% (6/20 families) in Kunming CM site.
Figure 2. Morphological characteristics of the DSE colonizing the roots of the studied ‘non-mycorrhizal’ plants in Kunming CM and Gejiu Niubahuang Sn/Pb/Zn mine tailings pond (NTP), SW China. (A) dark and septate hyphae in Stellaria media in CM; (B) microsclerotia in Chenopodium album in NTP; (C) mycelial labyrinth in Silene gallica in CM; (D) dark and septate hyphae in Polygonum sp.1 in NTP; (E) brain-like microsclerotium in Polygonum capitatum in NTP; (F) dark and septate hyphae and microsclerotia in Dianthus sp. in NTP.
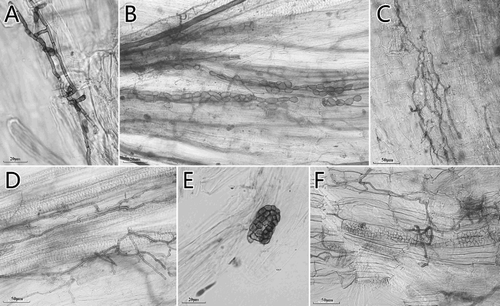
Table 1. Colonization intensity of DSE (mean ± SE, n ≥ 3) in plant roots in Kunming CM and Gejiu Niubahuang Sn/Pb/Zn mine tailings pond (NTP), SW China.
In Kunming CM site, the majority of plants had a low colonization intensity of DSE, ranging from 0.56% to 18%, with an average of 4.76% ± 0.80%. While, in Gejiu NTP site, the average DSE colonization intensity was 10.15 ± 1.44%, which was significantly higher than that in Kunming CM site (, p < .01). Interestingly, we found that there were approximately 55% plant species (11/20) with above 5% colonization intensity in Gejiu NTP site, and there were 5 plant species (25%) with above 10% DSE colonization intensity (). Meantime, we also noted that DSE colonization intensity varied greatly across plant species and even across different ecotypes of the same plant species from different ecological habitats. For example, the mean DSE colonization of Juncus effusus from Kunming CM site (2.22% ± 0.29%) was lower than that from Gejiu NTP site (6.46% ± 1.78%). Similar trends of DSE colonization were also observed in the roots of Chenopodium album, Fagopyrum dibotrys and Chenopodium ambrosioides from Gejiu NTP site (with a 3.55%, 3.45% and 3.89% DSE colonization intensity, respectively), significantly higher compared with the average DSE colonization of 2.45%, 1.89% and 1.44% in Kunming CM site, respectively. We also noted a distinct pattern of DSE colonization intensity in the 8 plant families surveyed, and the maximum value of 11.95 ± 6.68% occurred in the plants of Caryophyllaceae, then followed by Polygonaceae, Cyperaceae, Juncaceae, Chenopodiaceae, Cruciferae, and there was the lowest colonization intensity of 2.19% ± 1.91% in Amaranthaceae averagely. While, we also noted that the total DSE colonization intensity reached 35.56 ± 1.46%, but only one species of Commelina communis in Commelinaceae with three replicates from Gejiu NTP site.
Table 2. Results of independent T-test to compare the total average DSE colonization intensity (mean ± SE) in the plant roots from Kunming CM (n = 45) and GejiuNiubahuang Sn/Pb/Zn mine tailings pond (NTP, n = 75), SW China, including hyphae (H%), microsclerotia (M%) and the total DSE structures (DSE%).
Isolation and identification of DSE strains
In total, 90 root-associated melanized fungi were isolated, including 54 strains isolated from Gejiu NTP site and 36 strains from Kunming CM site (). Moreover, the isolation ratios did not differ significantly in Gejiu NTP site (the total isolation ratio was 2.7:1) from the Kunming CM site (the total isolation ratio was 2.4:1). According to the phylogenetic analysis, 90 fungal isolates were identified. Interestingly, the most common fungi colonizing the roots of these ‘non-mycorrhizal’ plants were related to the well-known DSE fungi, for example, Phialophora mustea, Cadophora luteo-olivacea. We found that the fungal species of Cladosporium, Cyphellophora and Phialophora were the most abundant with a total RA over 60% (). The RA of fungi from Spirosphaera, Pleosporales, Paraphoma and Cadophora was above 4%, while the remaining species were closely related to the fungi of Minimelanolocus, Rhizoscyphus, Dothideomycetes, Tetraplosphaeria, Ophiosphaerella and Bipolaris and were present at very low percentages ().
Figure 3. NJ phylogram of ITS sequences of cultured melanized fungal isolates colonizing the roots of ‘non-mycorrhizal’ plants in Kunming CM and Gejiu Niubahuang Sn/Pb/Zn mine tailings pond (NTP), SW China, based on the TrN + G substitution model determined by Modeltest 3.06 (Posada and Crandall Citation1998), rooted tree by Melampsora epitea as outgroup. Numbers above the nodes indicate the bootstrap support in NJ analysis, 1000 times. RA here represents the ratio of the numbers of fungal isolates in a given clade relative to the total number of fungal isolates. Arabic numbers in parentheses indicate the number of fungal isolates originating from NTP (above slash) and from CM (below slash), respectively.
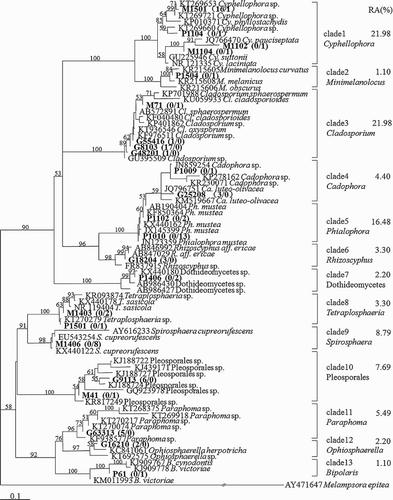
Discussion
In the present study, plant species belonging to Caryophyllaceae, Chenopodiaceae, Cyperaceae, Polygonaceae, Juncaceae, Cruciferae, Amaranthaceae and Commelinaceae were studied, which are conventionally accepted as non-AM families (Smith & Read Citation2008). In contrast to the almost absence of a mycorrhizal colonization, we found that all the root samples surveyed in this study were frequently colonized by DSEs, which suggested that these fungi were one of the most common fungal colonizers of the roots of the ‘non-mycorrhizal’ plant families. Increasing evidence showed that DSEs are ubiquitous, occurring in a variety of habitats ranging from South African coastal plains and lowlands to tropical, temperate, subalpine, alpine, maritime Antarctic and arctic zones (Jumpponen & Trappe Citation1998; Peterson et al. Citation2004; Vohník et al. Citation2015; Casazza et al. Citation2017; Hewitt et al. Citation2017). In fact, the co-existence between DSE and AMF symbionts occupying terrestrial environments have been extensively documented, but more field surveys also supported that a given plant species in specific microhabitats tends to favor the colonization by DSE over AM fungi (Weishampel & Bedford Citation2006). For example, Angel et al. (Citation2016) found that Poa attenuata, a plant growing at an elevation of 6150 m a.s.l., was intensively colonized by DSE, instead of AM fungi. Similarly, Vohnik et al. (Citation2016) also reported the DSE association with the roots of Posidonia oceanica, a submerged marine macrophyte without mycorrhizal colonization in the northwest Mediterranean Sea.
Additionally, we also noted the difference in DSE colonization intensity among specimens of Juncus effusus collected in the two sample sites (6.46 ± 1.78% in NTP vs. 2.22 ± 0.29% in CM). Evidently, differences in, for example, edaphic and climatic factors between the two different sample sites might also have influenced the process of DSE colonization, although the phylogenetic features of the host plants may play a decisive role in colonization (Miller et al. Citation1999; Sivakumar Citation2013). For example, Binet et al. (Citation2017) observed that by increasing the ambient temperature in Hummocks significantly enhanced the DSE root colonization of Andromeda polifolia but there was no effect on DSE colonization in warmed lawns, and they argued that the response of fungal root colonization to climate warming depends on the microhabitats. However, the abundant DSE colonization in ‘non-mycorrhizal’ plant roots observed in this study seems to suggest that root colonization by DSE and AMF and particularly the signaling, recognition and transduction process involved in these events relay on different molecular mechanisms and we think that it need further attention (Genre & Russo Citation2016).
In total, 90 endophytic fungi were isolated, and most fungi were closely related to the well-known DSE fungi, such as Cadophora spp. (anamorph, Phialophora spp.), Leptodontidium spp., Pleosporales spp. and Cyphellophora spp. (Newsham Citation2011). We found that Cyphellophora, Cladosporium and Phialophora were the most dominant genera colonizing the roots of all ‘non-mycorrhizal’ plant families surveyed in SW China. Pure-culture and culture-independent molecular analyses indicated that fungi from Cyphellophora, Cladosporium and Phialophora have been repeatedly isolated from various plant species (Bonfim et al. Citation2016; Coleman-Derr et al. Citation2016; Zhang et al. Citation2013). Accumulating evidence showed that under land cover changes or abrupt envrionmental shift, some habitats contained a rapid succession of fungi. For example, marine sediments in polluted conditions following the Deepwater Horizon Oil Spill, these fungi (e.g. Cladosporium spp.), who can extensively utilize hydrocarbon compounds, can thrive in hostile conditions, which appear to be intolerable for the other marine fungi (Bik et al. Citation2012). We argued that these common root-associated fungal assemblages, which presumedly contain the inherit merits of rapid succession to abrupt environmental change, give way to dominant fungal communities in Niubahuang Sn/Pb/Zn mine tailing pond.
Interestingly, certain members from Cladosporium and Cyphellophora which were commonly characterized as fungal pathogens to animals and plants (Feng et al. Citation2013; Gao et al. Citation2015). However, our endophytic fungal isolates from Cladosporium and Cyphellophora were found to live inside healthy tissues as quiescent microthalli. It was consistent with our previous findings that Exophiala pisciphila, previously reported as a fish-pathogenic fungus, dominantly colonized the roots of numerous plants without any disease symptoms in an ancient Pb-/Zn- slag heap in China (Zhang et al. Citation2013), instead showed putatively mutualistic behavior by conferring a marked cadmium tolerance to its host plant (Wang et al. Citation2016). Recently, similar phenomena were reported by Hiruma et al. (Citation2016) for the root endophytic Colletotrichum tofieldiae, a common member of destructive pathogens, which, under phosphorus-deficient conditions, confers plant fitness benefits by transferring the macronutrient phosphorus to shoots, promoting plant growth and increasing soil fertility.
Increasing reports of positive impacts of DSE colonization on their plant hosts support the view that DSEs indeed have a beneficial role in plant growth and development, plant nutrition and plant tolerance and resistance to abiotic and biotic stresses (Newsham Citation2011; Yuan et al. Citation2010). Direct evidence using isotope labeling revealed that a dematiaceous hyphomycete DSE, Heteroconium chaetospira formed a mutualistic symbiosis with Brassica campestris, a non-mycorrhizal plant of the Cruciferae family, in which the fungus supplied host plants with essential mineral nutrients (mainly N), in exchange for photosynthetically fixed carbohydrates (Usuki & Narisawa Citation2007). Subsequently, more experimental data have provided new insights to understand further aspects of DSE functioning, for example, releasing of phosphorus (P) from soil insoluble phosphates, for example, calcium phosphate to crops (Spagnoletti et al. Citation2017), producing auxin volatile organic compounds to stimulate plant growth (Berthelot et al. Citation2016), increasing the plant tolerance against water deficit (Santos et al. Citation2016), and against fungal disease (Yuan et al. Citation2016). Moreover, differently from AM fungi, having a broad host spectrum and the axenic cultivability on economically viable synthetic media, make DSEs suitable for mass scale inoculum production for application in reforestation of mine spoils. However, more detailed laboratory experiments on the interactions among ‘non-mycorrhizal’ host plants and their root-associated DSE fungi in providing mutual nutritional benefits and protecting hosts against biotic and abiotic stresses are needed.
Acknowledgments
The authors gratefully acknowledge Dr Zhao Dake (School of Agriculture, Yunnan University), for his help to identify all plant specimens.
Disclosure statement
No potential conflict of interest was reported by the authors.
ORCID
Zhiwei Zhao http://orcid.org/0000-0001-9451-9847
Additional information
Funding
References
- Altschul SF, Madden TL, Schäffer AA, Zhang J, Zhang Z, Miller W, Lipman DJ. 1997. Gapped BLAST and PSI-BLAST: a new generation of protein database search programs. Nucleic Acids Res. 25:3389–3402. doi: 10.1093/nar/25.17.3389
- Angel R, Conrad R, Dvorsky M, Kopecky M, Kotilínek M, Hiiesalu I, Schweingruber F, Doležal J. 2016. The root-associated microbial community of the world’s highest growing vascular plants. Microb Ecol. 72:394–406. doi: 10.1007/s00248-016-0779-8
- Arnold AE, Maynard Z, Gilbert GS, Coley PD, Kursar TA. 2000. Are tropical fungal endophytes hyperdiverse? Ecol Lett. 3:267–274. doi: 10.1046/j.1461-0248.2000.00159.x
- Berch SM, Kendrick B. 1982. Vesicular-arbuscular mycorrhizae of southern Ontario ferns and fern-allies. Mycologia. 74:769–776. doi: 10.2307/3792863
- Berthelot C, Leyval C, Foulon J, Chalot M, Blaudez D. 2016. Plant growth promotion, metabolite production and metal tolerance of dark septate endophytes isolated from metal-polluted poplar phytomanagement sites. FEMS Microbiol Ecol. 92:fiw144. doi: 10.1093/femsec/fiw144
- Bik HM, Halanych KM, Sharma J, Thomas WK. 2012. Dramatic shifts in benthic microbial eukaryote communities following the deepwater horizon oil spill. PLoS ONE. 7:e38550. doi: 10.1371/journal.pone.0038550
- Binet P, Rouifed S, Jassey VEJ, Toussaint ML, Chiapusio G. 2017. Experimental climate warming alters the relationship between fungal root symbiosis and Sphagnum litter phenolics in two peatland microhabitats. Soil Biol Biochem. 105:153–161. doi: 10.1016/j.soilbio.2016.11.020
- Bonfim JA, Vasconcellos FRL, Baldesin LF, Sieber TN, Cardoso JBN. 2016. Dark septate endophytic fungi of native plants along an altitudinal gradient in the Brazilian Atlantic forest. Fungal Ecol. 20:202–210. doi: 10.1016/j.funeco.2016.01.008
- Bravo A, York T, Pumplin N, Mueller LA, Harrison MJ. 2016. Genes conserved for arbuscular mycorrhizal symbiosis identified through phylogenomics. Nature Plants. 2:15208. doi: 10.1038/nplants.2015.208
- Card S, Johnson L, Teasdale S, Caradus J. 2016. Deciphering endophyte behaviour: the link between endophyte biology and efficacious biological control agents. FEMS Microbiol Ecol. 92:fiw114. doi: 10.1093/femsec/fiw114
- Casazza G, Lumini E, Ercole E, Dovana F, Guerrina M, Arnulfo A, Minuto L, Fusconi A, Mucciarelli M. 2017. The abundance and diversity of arbuscular mycorrhizal fungi are linked to the soil chemistry of screes and to slope in the Alpic paleo-endemic Berardia subacaulis. PLoS ONE. 12:e0171866. doi: 10.1371/journal.pone.0171866
- Cheng Q, Xia Q, Li W, Zhang S, Chen Z, Zuo R, Wang W. 2010. Density/area power-law models for separating multi-scale anomalies of ore and toxic elements in stream sediments in Gejiu mineral district, Yunnan Province, China. Biogeosciences. 7:4273–4293. doi: 10.5194/bgd-7-4273-2010
- Coleman-Derr D, Desgarennes D, Fonseca-Garcia C, Gross S, Clingenpeel S, Woyke T, North G, Visel A, Partida-Martinez LP, Tringe SG. 2016. Plant compartment and biogeography affect microbiome composition in cultivated and native Agave species. New Phytol. 209:798–811. doi: 10.1111/nph.13697
- Deram A, Languereau-Leman F, Haluwyn CV. 2011. Mycorrhizal and endophytic fungal colonization in Arrhenatherum elatius L. roots according to the soil contamination in heavy metals. Soil Sediment Contam. 20:114–127. doi: 10.1080/15320383.2011.528470
- Deram A, Languereau-Leman F, Howsam M, Petit D, Haluwyn CV. 2008. Seasonal patterns of cadmium accumulation in Arrhenatherum elatius (Poaceae): influence of mycorrhizal and endophytic fungal colonisation. Soil Biol Biochem. 40:845–848. doi: 10.1016/j.soilbio.2007.09.023
- Feng P, Klaassen CHW, Meis JF, Najafzadeh MJ, Ende AHGGVD, Xi L, de Hoog GS. 2013. Identification and typing of isolates of Cyphellophora and relatives by use of amplified fragment length polymorphism and rolling circle amplification. J Clin Microbiol. 51:931–937. doi: 10.1128/JCM.02898-12
- Gao L, Ma Y, Zhao W, Wei Z, Gleason ML, Chen H, Hao L, Sun G, Zhang R. 2015. Three new species of Cyphellophora (Chaetothyriales) associated with sooty blotch and flyspeck. PLoS ONE. 10:e0136857. doi: 10.1371/journal.pone.0136857
- Genre A, Russo G. 2016. Does a common pathway transduce symbiotic signals in plant–microbe interactions? Front Plant Sci. doi:10.3389/fpls.2016.00096.
- Hewitt RE, Chapin FS, III, Hollingsworth TN, Taylor DL. 2017. The potential for mycobiont sharing between shrubs and seedlings to facilitate tree establishment after wildfire at Alaska arctic treeline. Mol Ecol. doi:10.1111/mec.14143.
- Hiruma K, Gerlach N, Sacristán S, Nakano RT, Hacquard S, Kracher B, Neumann U, Ramírez D, Bucher M, O’Connell RJ, Schulze-Lefert P. 2016. Root endophyte Colletotrichum tofieldiae confers plant fitness benefits that are phosphate status dependent. Cell. 165:464–474. doi: 10.1016/j.cell.2016.02.028
- Huusko K, Ruotsalainen AL, Markkola AM. 2016. A shift from arbuscular mycorrhizal to dark septate endophytic colonization in Deschampsia flexuosa roots occurs along primary successional gradient. Mycorrhiza. 27:129–138. doi: 10.1007/s00572-016-0736-x
- Jumpponen A. 2001. Dark septate endophytes – are they mycorrhizal? Mycorrhiza. 11:207–211. doi: 10.1007/s005720100112
- Jumpponen ARI, Trappe JM. 1998. Dark septate endophytes: a review of facultative biotrophic root-colonizing fungi. New Phytol. 140:295–310. doi: 10.1046/j.1469-8137.1998.00265.x
- Li HY, Shen M, Zhou ZP, Li T, Wei YL, Lin LB. 2012. Diversity and cold adaptation of endophytic fungi from five dominant plant species collected from the Baima Snow Mountain, Southwest China. Fungal Divers. 54:79–86. doi: 10.1007/s13225-012-0153-1
- Mandyam K, Jumpponen A. 2014. Unraveling the dark septate endophyte functions: insights from the Arabidopsis model. In: VC Verma, AC Gange, editors. Advances in endophytic research. New Delhi: Springer India; p. 115–141.
- Massicotte HB, Melville LH, Peterson RL. 2005. Structural characteristics of root-fungal interactions for five ericaceous species in eastern Canada. Can J Bot. 83:1057–1064. doi: 10.1139/b05-046
- McGonigle TP, Miller MH, Evans DG, Fairchild GL, Swan JA. 1990. A new method which gives an objective-measure of colonization of roots by vesicular arbuscular mycorrhizal fungi. New Phytol. 115:495–501. doi: 10.1111/j.1469-8137.1990.tb00476.x
- Miller RM, Smith CI, Jastrow JD, Bever JD. 1999. Mycorrhizal status of the genus Carex (Cyperaceae). Am J Bot. 86:547–553. doi: 10.2307/2656816
- Monica IFD, Saparrat MCN, Godeas AM, Scervino JM. 2015. The co-existence between DSE and AMF symbionts affects plant P pools through P mineralization and solubilization processes. Fungal Ecol. 17:10–17. doi: 10.1016/j.funeco.2015.04.004
- Newman EI, Reddell P. 1987. The distribution of mycorrhizas among families of vascular plants. New Phytol. 106:745–751. doi: 10.1111/j.1469-8137.1987.tb00175.x
- Newsham KK. 2011. A meta-analysis of plant responses to dark septate root endophytes. New Phytol. 190:783–793. doi: 10.1111/j.1469-8137.2010.03611.x
- Peterson RL, Massicotte HB, Melville LH, Peterson RL, Massicotte HB, Melville LH. 2004. Mycorrhizas: anatomy and cell biology. Ottawa: NRC Research Press.
- Posada D, Crandall KA. 1998. MODELTEST: testing the model of DNA substitution. Bioinformatics. 14:817–818. doi: 10.1093/bioinformatics/14.9.817
- Quiza L, St-Arnaud M, Yergeau E. 2015. Harnessing phytomicrobiome signaling for rhizosphere microbiome engineering. Front Plant Sci. 6:507. doi: 10.3389/fpls.2015.00507
- Remy W, Taylor TN, Hass H, Kerp H. 1994. Four hundred-million-year-old vesicular arbuscular mycorrhizae. P Natl Acad Sci USA. 91:11841–11843. doi: 10.1073/pnas.91.25.11841
- Santos S, Silva P, Garcia AC, Zilli JÉ, Berbara RLL. 2016. Dark septate endophyte decreases stress on rice plants. Braz J Microbiol. doi.org/10.1016/j.bjm.2016.09.018.
- Sharrock RA, Sinclair FL, Gliddon C, Rao IM, Barrios E, Mustonen PJ, Smithson P, Jones DL, Godbold DL. 2004. A global assessment using PCR techniques of mycorrhizal fungal populations colonising Tithonia diversifolia. Mycorrhiza. 14:103–109. doi: 10.1007/s00572-003-0243-8
- Silvani VA, Fracchia S, Fernandez L, Pergola M, Godeas A. 2008. A simple method to obtain endophytic microorganisms from field-collected roots. Soil Biol Biochem. 40:1259–1263. doi: 10.1016/j.soilbio.2007.11.022
- Sivakumar N. 2013. Effect of edaphic factors and seasonal variation on spore density and root colonization of arbuscular mycorrhizal fungi in sugarcane fields. Ann Microbiol. 63:151–160. doi: 10.1007/s13213-012-0455-2
- Smith SE, Read DJ. 2008. Mycorrhizal symbiosis. London, UK: Academic Press.
- Spagnoletti FN, Tobar NE, Fernández Di Pardo A, Chiocchio VM, Lavado RS. 2017. Dark septate endophytes present different potential to solubilize calcium, iron and aluminum phosphates. Appl Soil Ecol. 111:25–32. doi: 10.1016/j.apsoil.2016.11.010
- Swofford D. 2002. PAUP: Phylogenetic Analysis Using Parsimony (*and other methods). Sunderland (MA): Sinauer Associates.
- Usuki F, Narisawa K. 2007. A mutualistic symbiosis between a dark septate endophytic fungus, Heteroconium chaetospira, and a nonmycorrhizal plant, Chinese cabbage. Mycologia. 99:175–184. doi: 10.1080/15572536.2007.11832577
- Vohnik M, Borovec O, Kolarik M. 2016. Communities of cultivable root mycobionts of the seagrass Posidonia oceanica in the northwest Mediterranean Sea are dominated by a hitherto undescribed Pleosporalean dark septate endophyte. Microb Ecol. 71:442–451. doi: 10.1007/s00248-015-0640-5
- Vohník M, Borovec O, Župan I, Vondrášek D, Petrtýl M, Sudová R. 2015. Anatomically and morphologically unique dark septate endophytic association in the roots of the Mediterranean endemic seagrass Posidonia oceanica. Mycorrhiza. 25:663–672. doi: 10.1007/s00572-015-0642-7
- Wang B, Yeun LH, Xue JY, Liu Y, Ane JM, Qiu YL. 2010. Presence of three mycorrhizal genes in the common ancestor of land plants suggests a key role of mycorrhizas in the colonization of land by plants. New Phytol. 186:514–525. doi: 10.1111/j.1469-8137.2009.03137.x
- Wang J, Li T, Liu G, Smith JM, Zhao Z. 2016. Unraveling the role of dark septate endophyte (DSE) colonizing maize (Zea mays) under cadmium stress: physiological, cytological and genic aspects. Sci Rep. 6:22028. doi: 10.1038/srep22028
- Weishampel PA, Bedford BL. 2006. Wetland dicots and monocots differ in colonization by arbuscular mycorrhizal fungi and dark septate endophytes. Mycorrhiza. 16:495–502. doi: 10.1007/s00572-006-0064-7
- White TJ, Bruns T, Lee S, Taylor J. 1990. Amplification and direct sequencing of fungal ribosomal RNA genes for phylogenetics. In: N Innis, D Gelfand, J Sninsky, T White, editors. PCR protocols: a guide to methods and applications. San Diego (CA): Academic Press; p. 315–322.
- Yuan Z, Su Z, Zhang C. 2016. Understanding the biodiversity and functions of root fungal endophytes: the ascomycete Harpophora oryzae as a model case. In: IS Druzhinina, CP Kubicek, editors. Environmental and microbial relationships. The Mycota. Berlin: Springer International; p. 205–214.
- Yuan Z, Zhang C, Lin F. 2010. Role of diverse non-systemic fungal endophytes in plant performance and response to stress: progress and approaches. J Plant Growth Regul. 29:116–126. doi: 10.1007/s00344-009-9112-9
- Zhang N. 2011. Stand characteristics of artificial Pinus armandii forest in Kunming city. J West China Forestry Sci. 40:43–47. Chinese.
- Zhang Y, Li T, Zhao ZW. 2013. Colonization characteristics and composition of dark septate endophytes (DSE) in a lead and zinc slag heap in Southwest China. Soil Sediment Contam. 22:532–545. doi: 10.1080/15320383.2013.750267