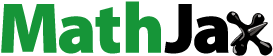
ABSTRACT
The experiments were conducted on tomato plants to study the interactive responses of water levels (W70%: 70% of water consumption and W90%: 90% water consumption) and nitrogen rates (N100%: 100% of recommended and N80%:80% of N1.0) under two straw mulching conditions (NS; no straw introduced and WS: with buried straw layer) on growth and physiological parameters for two fruit growing years to assess the interactive responses of fertigation under buried straw layer on the changes in plant fresh and dry biomass, roots biomass, photosynthesis rate (PN), stomatal conductance (GS) and chlorophyll fluorescence of tomato plants. Buried straw layer was proved to improve plant biomass, photosynthesis rate and other physiological traits such as chlorophyll contents (Chl), maximum electron transport rate (ETRmax), maximum quantum yield (FV/FM) and GS under the lower fertilizer (N80%) and irrigation levels (W70%). However, increasing fertilizer and irrigation level decreased these parameters significantly (p < .05 to p < .001) under buried straw layer. Conversely, increased fertilizer (N100%) and irrigation (W90%) levels increased these parameters significantly (p < .05 to p < .001) under no straw condition. The overall findings revealed that buried straw layer could relieve stress developed by limited irrigation water and fertilizer and benefit plant growth, physiological parameters and fruit yield of tomato plant.
1. Introduction
Tomato is a widely grown plant due to the high nutritional value of its fruit and great economic importance (Savić et al. Citation2008). China is one of the leading tomato producing countries with a production of 50,552,200.0 metric tons, and it is 6.82% of the world’s grown tomatoes (FAO Citation2016). Tomato growth parameters are strongly influenced by two significant cultural manageable factors such as nutrients and irrigation water (Ozbahce and Tari Citation2010; Chen et al. Citation2013). Inefficient and overuse of nitrogen fertilizer is a severe issue in China with an adverse economic and environmental impacts (Zhang et al. Citation2015). According to the investigation by the Chinese Ministry of Agriculture, the amount of fertilizer applied is higher and beyond the safe level (Shuqin and Fang Citation2018).
Conventional methods of high input of nutrient and water management are still being used by most of the farmer’s community to achieve higher yield. However, the extreme usage of nutrients and water have also led to decrease fertilization efficiency by increasing leaching of nutrients and resulted in severe degradation of soil environment especially in the Southern China, which produces most of the food (Delang Citation2017) as well as water pollution (Zhang et al. Citation2015). Therefore an appropriate management is a crucial measure to improve crop yield, quality and fertilizer use efficiency (Kennedy et al. Citation2013; Luo and Li Citation2018).
Fertigation is a method by which irrigation and fertilization can be applied precisely. However, fertigation requires sufficient skills and knowledge of soil and vegetables that farmers are lacking in many vegetable production areas. Over fertilization and irrigation are still common under fertigation. A higher application of nitrogen fertilizer may cause leaching of NO3–N correspondingly (Ullah et al. Citation2017) and ultimately disturb the nitrate contents in fruits of tomato (Wang et al. Citation2015). At the same time, higher application of water will inevitably promote leaching and waste of fertilizer (Ullah et al. Citation2017; Li et al. Citation2018). To fulfill the high demand of food, there is conflict to recommendations to lessen the use of fertilizer in China as it is considered as a risk of production decrease, despite the extensive evidence. Too much fertilization results in low fertilizer use efficiency, increases costs of production, and needlessly larger losses of nutrients triggering severe ecological problems such as groundwater nitrate contamination and greenhouse gas emissions. In the current situation, additional measures are immensely needed to minimize fertilizer wastage and mitigate the soil degradation (Delang Citation2017).
Photosynthesis is the key physiological process of plants and can provide 90% of plant biomass (Wang et al. Citation2018). Leaves are the main contributors to the crop productivity (Ashraf and Bashir Citation2003), and their photosynthetic activities are crucial significantly for harvestable fruit yield. Leaf gas-exchange such as photosynthesis rate and stomatal conductance or chlorophyll fluorescence parameters such as light curve, maximum electron transport rate and maximum quantum yield based on the operating quantum efficiency of electron transport can be used to estimate leaf photosynthetic capacities.
Water deficit negatively affects water potential of plants which results in, dehydration, stomatal conductance reduction and changes in fluorescence (Pessarakli Citation2005). The decline in photosynthesis can be credited to a decrease in intercellular carbon dioxide concentration partially to metabolic elements (Lawlor and Cornic Citation2002) and partially because of closing of stomata, which subsequently leads to photo-inhibitory damage of PSII reaction centers (Souza et al. Citation2004). The deficiency of nutrients can directly disturb photosynthetic actions and restrict partitioning of assimilates from the leaves to the fruits (Kanai et al. Citation2011). On the other hand, applied nutrients can be used to synthesize the components of the photosynthetic apparatus (Sugiharto et al. Citation1990), including rubisco, chlorophyll and carotenoid containing membrane proteins (Bungard et al. Citation1997).
The crop yield of plant is mainly based on its photosynthetic capacity (Pessarakli Citation2005). Leaf photosynthesis is the main reason for the majority of the variation in biomass and crop production (Takai et al. Citation2010). However, three differing correlations between leaf photosynthesis and crop yield have been reported by different researchers: no correlation (Chongo and McVetty Citation2001), positive (Ashraf Citation2003; Hubbart et al. Citation2007) and negative (Long et al. Citation2006). Lawlor (Citation1995) described that these clashing findings could be clarified by the fact that limiting plant and environmental dynamics interact strongly for regulating photosynthesis, which subsequently affects crop yield. Therefore, it is important to improve understanding of the approaches underlying biomass production under different conditions (Jaimez et al. Citation2008).
The response of either fertilizer or irrigation on photosynthetic effects of tomato plants have been well studied, however, the interactive effects of fertigation under an isolated straw layer on these factors have not been studied. Furthermore, how could leaf photosynthesis affects other physiological traits under these conditions? Therefore, the objectives of this study were to assess the interactive responses of different fertilizer and irrigation levels under a buried straw layer on growth, photosynthesis rate, stomatal conductance and Chlorophyll fluorescence parameters for two growing season.
2. Materials and methods
2.1. Site description and cropping conditions
The experiments were carried out in April–July 2017 and 2018 in a greenhouse without temperature control under nature light environments, situated at State Key Laboratory of Efficient Irrigation and Drainage of Hohai University, Nanjing, China at 31°95′ N, 118°83′ E and 15 m above the sea level. Microclimatic parameters inside the greenhouse are shown in . The soil physical and chemical properties are presented in . Six-week-old uniform seedlings of tomato (Solanum lycopersicum L.) were transplanted in beds, and each bed consisted of three tomato plants having a distance of 50 cm between two plants. Plant density was maintained as 3.33 plants.m−2. A nylon cord was used for plants vertical support. Pruning was also done to uphold the appropriate growth by following the well managed local agronomic practices.
Table 1. Microclimatic parameters inside the greenhouse during growing seasons.
Table 2. Soil physical and chemical properties.
2.2. Experimental design
Three factors randomized complete block design with three replications was established to assess the effects of irrigation and nitrogen regimes under buried straw layer on growth and physiological traits of greenhouse-grown tomato. Two water levels (W70%: 70% of water consumption; and W90%: 90% water consumption) and two nitrogen rates (N100%: 100% of recommended and N80%:80% of N100%) under two straw mulching conditions (NS; no straw introduced and WS: with buried straw layer) were applied (Table ). A 5 cm thick straw layer was buried at a 20 cm depth in the soil. Each block had eight rows and 24 plants. Before transplanting, the top soil was rototilled and the beds were manually raised. For N100%, 225 kg.ha−1 and for N80%, 180 kg.ha−1 of pure nitrogen was applied in the form of CO(NH2)2, 112.5 kg.ha−1 of P2O5 was applied to all the treatments in the form of KH2PO4 and 135 kg.ha−1 of K2O was applied to all the treatments in the form of KH2PO4 and K2SO4.
Table 3. Treatments information.
2.3. Irrigation requirements
The same level of irrigation water was applied for the first 21 days to ensure the proper growth of the plants and treatments were imposed on day 22. Volumetric soil moisture contents θv were monitored by a Time Domain Reflectometer with 4-days interval during both growing seasons. The irrigation frequency was kept at every two days from commencement of irrigation regimes until the harvesting of the crop. Irrigation was done by the gravity drip irrigation bags having a capacity of 1.5 L. The storage bags were hanged at a height of 1.5 m to store irrigation water to irrigate a single plant. Every bag had two drippers, which were buried at a depth of 2 cm on either side of a plant at a distance of 10 cm from the stem of the plant. Fertigation was done in four equal splits along the season.
Soil water deficit (WSD) was computed by using Equation (1).
(1)
(1) where soil water deficit (mm), θt1 and θt2 are the volumetric water contents (%) in root zone at time t1 and t2, respectively and Zrt is the depth of root zone (cm)
Amounts of irrigation water for treatments were computed by using Equation (2).
(2)
(2) where WI is irrigation water (mm), WSD is water deficit before irrigation (mm) and Kn is the rate of water deficit (0.9 for W90% and 0.7 for W70%).
Crop consumptive use (ET) was estimated using the following water balance method:
(3)
(3) where ET (mm) is the crop consumptive use, IW (mm) is irrigation water, ΔS (mm) is a change in soil water storage, D and R, are losses due to deep percolation and run-off (mm), respectively. Deep percolation and Run-off can be ignored for drip irrigation system (Yuan et al. Citation2001; Chen et al. Citation2015). Thus, Equation (3) can be simplified:
(4)
(4)
2.4. Plant growth parameters
The plant material was collected at the end of the experiments for both seasons and washed by tap water. After recording fresh weight, all the material was dried at 70° until the sample weight was held constant. Then the dry weight of biomass and roots were recorded.
2.5. Photosynthesis rate and stomatal conductance
Photosynthesis rate (PN) and stomatal conductance (GS) of two mature leaflets in the upper canopy were measured during both years on 38, 64 and 86 DAT after transplanting for flowering, fruiting and harvesting stages respectively using a portable photosynthesis system (Li-6400) from 9:00 to 11:00 in the morning under natural conditions. The measurements were conducted at temperature natural conditions and CO2 at 400 µmol. mol−1.
2.6. Chlorophyll fluorescence
Light response curves and FV/FM were measured using Mini-PAM II (Walz, Germany) on 36, 62 and 85 days after transplanting during both years. Light curve measurements were done at 12 PAR levels from 0 to 1500 µmol.m−2.s−1. ETRmax was determined using the following method developed by (Silsbe and Kromkamp Citation2012).
(5)
(5)
Using the above expression, ETRmax can be determined as:
(6)
(6)
where ETR; electron transfer rate, FV/FM; maximum quantum yield PARsat; saturation light intensity (µmol.m−2.s−1) and α, β and I are leaf absorptance coefficient, distribution of light energy between PSI and PSII and light intensity (µmol.m−2.s−1) respectively and were derived from the light response curves.
2.7. Chlorophyll content
A non-destructive chlorophyll meter SPAD-502 (Japan) was used to record the relative leaf greenness using the same leaves used for other physiological parameters.
2.8. Statistical analysis
Three-way analysis of variance was achieved using Statistix 8.1 statistical software and LSD was applied for means comparisons at p < .05 significance level. Linear regression was performed on Microsoft excel 2016 to test the correlation of maximum electron transport rate, chlorophyll contents and stomatal conductance with photosynthesis rate for both seasons.
3. Results
3.1. Water consumption
The amount of water consumed under different treatments during different growth stages is shown in . During the seedling stage, the same quantity of irrigation was applied to all the treatments for plant optimum establishment. The lower fertilizer and irrigation levels under buried straw layer utilized lowest amount of water for both years. On the other hand, higher fertilizer and high irrigation levels with no straw layer used highest amount of water during both years.
Table 4. Irrigation water consumed under different growth stages in 2017 and 2018 growing seasons.
3.2. Plant and roots fresh and dry biomass
The aboveground plant biomass, root biomass and root length at harvesting time are shown in . In general, plant fresh weight (PFW) and plant dry weight (PDW) were both significantly increased under buried straw layer in both growing seasons (p < .001). The mean values of FPW increase with increasing fertilizer significantly (p < .05 in 2017 and p < .001 in 2018) and no significant increase was observed in PDW with increasing fertilizer in 2017 but a significant increase was noticed in 2018 (p < .05). Similarly, increasing irrigation water also increased both FPW and DPW in both experimental seasons (p < .001). The interactions of the buried straw layer with fertilizer, fertilizer with irrigation and irrigation with buried straw layer increased both FPW and DPW significantly (p < .001) in both growing seasons. The highest PFW and PDW values were recorded under the T5 (NSN100%W90%) treatment (645.25 and 96.66 g, respectively, in 2017; and 683.39 and 116.12 g, respectively, in 2018) followed by T4 (WSN80%W70%). The smallest FPW and DPW values were observed under T7 (NSN100%W70%) treatment (342.36 and 53.88 g, respectively, in 2017; and 380.47 and 60.94, respectively, in 2018).
Table 5. Tomato plant growth parameters grown under two fertilizer levels and two irrigation regimes with and without buried straw layer during 2017 and 2018 growing seasons.
The buried straw layer significantly increased roots fresh weight (RFW) (p < .001) and root dry weight (RDW) (p < .01) for both experimental season. Increasing fertilizer did not increase RFW significantly for both seasons but RDW was significantly increased in 2017 experimental season (p < .01). Irrigation also had significant effect on both RFW and RDW and increased RFW for both seasons (p < .001) but different significance was observed in RDW (p < .05 in 2017 and p < .001 in 2018). The interactions of the buried straw layer with fertilizer, fertilizer with irrigation and irrigation with buried straw layer significantly increased RFW and RDW (p < .01 to p < .001) in both growing seasons. The T4 (WSN80%W70%) treatment resulted in the highest RFW values (34.18 and 35.44 g) followed by T5 (NSN100%W90%) (33.59 and 37.35 g) in 2017 and 2018, respectively. The lowest values of RFW (18.04 and 21.03 g) were recorded in T3 (NSN80%W70%) in 2017 and 2018 respectively. The highest RDW was achieved in T5 treatment (7.76 and 8.17 g) followed by T4 treatment (7.35 and 7.76 g) in consecutive seasons. The lowest RDW values (5.01 and 5.39 g) were recorded in T1 (NSN80%W90%) and T7 (NSN100%W70%) in 2017 and 2018 respectively. Root length (RL) was significantly decreased under buried straw layer in 2017 (p < .05) but no significant effect was observed in 2018. RL was significantly increased by increasing fertilizer and irrigation water (p < .001). The interaction of buried straw layer and fertilizer had no significant effect on RL. A significant increase in RL was noticed when fertilizer interacted with irrigation in 2017 but no significant effect was observed in 2018. Similarly, the interaction of irrigation and buried straw mulching had no significant effect on RL in 2017 but RL increased significantly in 2018. The highest (53.48 and 53.95 cm) and lowest (39.30 and 36.00 cm) RL was observed in T5 and T4.
3.3. Photosynthesis and stomatal conductance
The effects of buried straw layer, fertilizer, irrigation and their interactions on PN and GS is presented in . During both years, at the flowering stage no significant change in PN and GS was observed among all the treatments. The interaction between fertilizer and irrigation significantly affected the PN (p < .01 and p < .001 respectively) and GS (p < .05 and p < .001 respectively) at the flowering stage for both years. At the fruiting stage, all the factors and their interactions affected both PN and GS significantly. In 2017 the highest and lowest decrease in PN was observed in T7 (NSN100%W70%) (48.24%) and T4 (WSN80%W70%) (5.98%) respectively whereas T8 (WSN100%W70%) (44.84%) and T4 (6.91%) had highest and lowest decrease in 2018 when compared with T5 (NSN100%W90%) at the fruiting stage. While studying the effect of different treatments on GS at fruiting stage, the lowest and highest decrease was recorded in T4 (7.58%) and T7 (46.54%) in 2017 experimental season when compared with T5 however in 2018, highest and lowest decrease was noted in T3 (NSN80%W70%) (48.21%) and T4 (8.21%) respectively. The interactive factors significantly affected PN during the harvesting stage for both years (p < .01 to p < .001). Comparing with T5, the highest and lowest decrease in PN was observed in T7 (42.76 and 30.62%) and T4 (6.21 and 5.61%) in 2017 and 2018 respectively. During the harvesting stage, all the factors and their interaction affected GS significantly except their combined interaction (S*N*W) for both years (p < .05 to p < .001). The lowest and highest decrease in GS was observed in T4 (7.91 and 7.29%) and T3 (NSN80%W70%) (49.64 and 34.11%) for 2017 and 2018 respectively.
Table 6. Effect of buried straw layer, fertilizer, irrigation and their interactions on PN and GS.
3.4. Fluorescence parameters
The response of the buried straw layer, fertilizer levels, irrigation regimes and their interactions on chlorophyll contents (Chl) and maximum electron transport rate (ETRmax) are represented in . A polynomial relationship between light intensity and electron transport rate was fitted () in all three growth stages (p < .001). During the flowering stage, irrigation affected the Chl significantly whereas irrigation and the interactive effect of fertilizer and irrigation had significant effects on ETRmax (p < .05) for both growing seasons. Comparing with T5 (NSN100%W90%), highest decrease in Chl was noticed in T3 (NSN80%W70%) (7.18%) and T7 (NSN100%W70%) (6.24%) during 2017 and 2018 respectively, however, T8 (WSN100%W70%) had highest decrease in ETRmax for both years during flowering stage. Fertilizer, irrigation, interactive effects of buried straw layer and fertilizer, and the interactions of straw layer and irrigation significantly affected Chl but fertilizer, interactive effect of fertilizer and buried straw layer, and the interaction between irrigation and buried straw layer significantly affected ETRmax during both years (p < .05 to p < .001) at the fruiting stage. Comparing with T5, the highest decrease in Chl and ETRmax was noted in T6 (WSN100%W90%) (8.98%) in 2017 whereas in 2018, highest decrease was observed in T4 (4.39%) and T3 (NSN80%W70%) (19.65%) respectively during the fruiting stage. However, for both years, T3 gave highest decrease in ETRmax (17.64 and 16.92%) at fruiting stage while comparing with T5. During the harvesting stage, Chl was significantly affected by buried straw layer, fertilizer, the interaction between straw layer and fertilizer and the interactive effects of straw layer and irrigation (p < .05 to p < .001) for both years. On the other hand, fertilizer, interaction of fertilizer with buried straw layer, irrigation with buried straw layer and fertilizer with irrigation significantly affected ETRmax (p < .05 to p < .001) during both years Comparing with T5, highest decrease in Chl was observed in T3 (21.70%) and T2 (WSN80%W90%) (16.36%) in 2017 and 2018 respectively during the harvesting stage. On the other hand, T3 had highest decrease in ETRmax (19.60 and 18.91%) during harvesting stage for consecutive years. The shows the effects of buried straw layer, fertilizer levels, irrigation regimes and their interactions on the maximum quantum yield (FV/ FM) for flowering, fruiting and harvesting stages during 2017 and 2018 growing seasons. At the flowering stage, fertilizer significantly affected FV/FM during 2017 (p < .05) and 2018 (p < .01) respectively. Comparing with T5 (NSN100%W90%), T6 (WSN100%W90%) and T3 (NSN80%W70%) had lowest (0.29 and 4.81%) and highest (17.0 and 19.76%) decrease in FV/FM during 2017 and 2018 respectively. During the fruiting stage, the interactive effects of buried straw layer and irrigation significantly affected FV/FM (p < .05) in both years whereas the interaction of straw with fertilizer and irrigation with fertilizer had a significant effect on FV/FM (p < .05) only in 2018. The highest and lowest decrease was observed in T3 (16.20 and 14.66%) and T4 (WSN80%W70%) (7.44 and 1.01%) respectively in the consecutive years when compared with T5 at the fruiting stage. At the harvesting stage, a significant interactive effect of straw and irrigation was noted during both years (p < .05) whereas straw and fertilizer significantly affected FV/FM in 2018 season. Comparing with T5, T4 and T3 had lowest (4.79 and 5.24%) and highest (19.66 and 22.80%) decrease in FV/FM during consecutive growing years at the harvesting stage.
Figure 1. Light curves as affected by the interaction of fertilizer and irrigation levels with and without buried straw layer at flowering stages (a) in 2017 and (b) in 2018, fruiting stages (c) in 2017 and (d) in 2018, and harvesting stages (e) in 2017 and (f) in 2018. Each curve was constructed using three replicates for all the treatments for both years.

Table 7. Effect of buried straw layer, fertilizer, irrigation and their interactions on Chl and ETRmax.
Table 8. Effect of buried straw layer, fertilizer, irrigation and their interactions on FV/FM and Fruit Yield.
3.5. Correlation of GS, ETRmax and Chl with PN
The linear regression relationships of GS, ETRmax, Chl and GS with PN during 2017 and 2018 is shown in which shows that correlations obtained for ETRmax, Chl and GS with PN during both years were highly significant (p < .001). The linear regression coefficients (R2) between PN and GS were 0.963 for 2017 and 0.9173 for 2018. Although, the correlation between ETRmax and PN was significant but its values were 0.3819 and 0.4847 in 2017 and 2018, respectively. The correlation between Chl and PN was also significant and the R2 of these relations were 0.6677 for 2017 and 0.3441 for 2018.
3.6. Fruit yield
During both years, the highest decrease in yield was observed in T7 (MNFCI70) when compared to T5 (MNFCI90). The highest fruit yield (8.73 and 10.58 kgm−2) was achieved by T5 (NSN100%W90%) followed by T4 (WSN80%W70%) (6.57 and 9.19 kgm−2) whereas lowest fruit yield (4.47 and 6.76 kgm−2) were achieved inT7 (NSN100%W70%) in 2017 and 2018 respectively (). T5 resulted with the highest yield, but it increased the input cost of irrigation water and fertilizer. On the other hand, T4 has no significant decrease in the fruit yield but it decreased the input costs of the water and fertilizer respectively.
4. Discussions
Irrigation is a substantial factor of plant growth therefore increased irrigation deficiency can lead to decrease plant vegetative measurements by decreasing soil water constants and consequently reduce water availability to the plant growth. On the other hand, the buried straw layer can hold moisture and decreases the chances of plant stress. In our findings, buried straw mulching significantly affected the soil water contents when compared with the treatments having no straw layer. Similar trends have been reported by (Zhang et al. Citation2010; Mingze et al. Citation2016). The treatments T4 and T5 (NSN100%W90%) recorded lowest and highest water consumption but no significant difference for most vegetative measurements was noticed between these two treatments except the plant root attributes. Similar trends for roots have been reported by Man et al. (Citation2016) and (Rasool et al. Citation2019) who stated that keeping soil water contents at a moderate level enhances the root distribution in the topsoil layers. Li et al. (Citation2010) also noted that deficit irrigation levels increase root density in the topsoil layers. These results match with our findings which reveal that optimum levels of moisture in the root zone can improve roots density compared to deficit or full irrigation.
It was concluded by Harris (Citation1992) that root length is possibly a better measure of the absorbing capability of roots, which is also proved by our results that under W90% treatments, roots had vertical whereas roots had a horizontal pattern under W70% treatments due to the difference in wetting pattern of irrigation under different irrigation levels. There is a close correlation between plant nutrient absorption and water uptake. During the process of water absorption by plant roots, the nutrients move to the roots surface. Limiting the plant water uptake also restricts the delivery of nutrients to the plant roots. The processes of diffusion and mass flow, responsible for bathing the plant roots with nutrients slow down as the soil dries. The tomato plant requires a balanced management of fertilizer for optimum growth because it is a heavy remover of nutrients and responds well to the fertigation (Hebbar et al. Citation2004). In addition, deficit irrigation and fertilizer levels may lead to adversely affect the plant growth. On the other hand, buried straw layer can decrease the impact of water and fertilizer deficiency by holding water and nutrients in the topsoil layer (Zhao et al. Citation2014). As in our findings, application of higher water and fertilizer with no straw layer and lower water and fertilizer with buried straw layer had no significant difference in most vegetative growth parameters. Similar trends have also been reported by (Chen et al. Citation2016; Chen et al. Citation2019). It is found in our results that plant growth parameters are more sensitive to mulching and irrigation than fertilizer. It is also reported by (Xiukang and Yingying Citation2016) that irrigation has more effects on plant growth than fertilizer.
The interactive effect of fertilizer and irrigation significantly affected PN and GS at the flowering stage during both years (p < .001) but no significant difference was notice because all the plants were treated with same practices for the day 1 to day 21 and treatments were established on 22nd day. At fruiting and harvestings stages, combination of higher levels of fertilizer and irrigation improved PN and GS under no buried straw conditions whereas lower fertilizer (N80%) and irrigation (W70%) levels had a positive effect on PN and GS under buried straw layer. The reason of improved PN and GS by the lower irrigation and fertilizer under buried straw layer treatment (T4) could be the effect of better plant growth under T4, which was improved due to the horizontal distribution of roots at the topsoil layer, which increased plant nutrients and water uptake. Under increased fertilizer and lower irrigation, both PN and GS were decreased at fruiting and harvesting stages. Liu et al. (Citation2012) advised that nitrogen and water are closely associated, and drought tolerance cab be enhanced by applying nitrogen at a lower rate which subsequently can improve water uptake and photosynthetic capacity. Consequently, an optimal low nitrogen supply would be adopted under the deficit conditions. It is also reported by Garcia et al. (Citation2007) that the water-stressed tomato plants showed a decrease in stomatal conductance due to an increase in leaf osmotic potential and decrease in leaf water potential. The optimal addition of nitrogen can contribute to recover the adversative drought effect by a process of elastic and osmotic adjustment. In our findings, a direct relation was observed between PN and GS, as PN was significantly increased with the rise in GS () .
Hebbar et al. (Citation2004) stated that chlorophyll contents of tomato were significantly affected by the interaction between fertilization and irrigation. However, no significant effect of the interaction between fertilization and irrigation was noticed during our study but the interactive effects of buried straw layer with fertilizer as well as with irrigation affected Chl significantly. Photosynthesis was significantly influenced by chlorophyll contents () which is also stated by Hebbar et al. (Citation2004) and Xiukang and Yingying (Citation2016), who described that photosynthesis is the main function of leaves however, fertilization and irrigation are the main factors which progress leaves growth and subsequently improves plant growth, crop production and crop quality. Chlorophyll fluorescence analysis allows near-instantaneous, non-invasive measurement of main aspects of electron transport and photosynthetic light capture (Campbell et al. Citation1998) and responds to quantum yield, state of energy distribution in the thylakoid membrane and the extent of photoinhibition (Wang et al. Citation2007). ETRmax and PN were found to have a significant correlation (, p < .001). The possible reason due to which T4 (WSN80%W70%) could get higher yield and T6 (WSN100%W90%) decreased the fruit yield is horizontal distribution of plant roots in T4, which supports higher nutrients availability. While, under T6, most part of the roots was in the lower side of straw layer, which reduced the nutrients uptake ability of the plants as most of the nutrients, sustained by straw layer. Also, the straw layer could reduce leaching loss of nitrogen and water due to the characteristic of straw-lack of capillary (Zhao et al. Citation2014). If the soil moisture of the upper-layer is decreased due to evapotranspiration loss then straw use could overcome the adversative effects of water stress by increasing plant water status, improving plant growth and water use efficiencies (Abd El-Mageed et al. Citation2018). The increment in nitrogen fertilization reduced tomato yield under no straw and W70% treatment (T7). This result indicated that increasing nitrogen rate under water deficit will be relatively inefficient, could be because of an adverse effects of excessive nitrogen on yields (El Mokh et al. Citation2015; Ullah et al. Citation2017).
5. Conclusions
It was revealed in the present study that growth and physiological parameters were all affected by fertilizer, irrigation and buried straw layer. Buried straw layer improved both growth and physiological parameters under lower fertilizer (N80%) and irrigation levels (W70%) but decreased under higher fertilizer (N100%) and irrigation levels (W90%) during both years. Plant and roots fresh and dry biomass, photosynthesis, stomatal conductance, ETRmax, FV/FM and chlorophyll contents were significantly improved in T5 (NSN100%W90%) followed by T5 (WSN80%W70%). PN was noticed to be correlated with GS, ETRmax, and Chl significantly suggesting that osmotic effect was the key factor influencing the photosynthetic process. It was observed that under limited water conditions, buried straw layer could be applied to relieve the adverse effect of deficit irrigation and fertilizer levels for improving plant growth and physiology and fruit yield.
Disclosure statement
No potential conflict of interest was reported by the authors.
Additional information
Funding
Notes on contributors
Ghulam Rasool
Ghulam Rasool is PhD candidate at Hohai University in the College of Agricultural Engineering. His research interests include Agricultural Soil and Water Engineering, Soil and Water Conservation, and Soil, Water and Plant relationship.
Xiangping Guo
Xiangping Guo is Professor at Hohai University in the College of Agricultural Engineering. His research focuses Soil and Water Engineering, and Water Saving irrigation.
Zhenchang Wang
Zhenchang Wang is Associate Professor at Hohai University in the College of Agricultural Engineering. His research interests are; deficit irrigation, Salinity, Soil, Water and Plant relationship.
Sheng Chen
Sheng Chen is Post-doctorate researcher at Hohai University in the College of Water Conservancy and Hydropower Engineering. His research focus is agricultural water engineering.
Ikram Ullah
Ikram Ullah is Post-doctorate researcher at Jiangsu University, Zhenjiang in the School of Agricultural Equipment Engineering. His research directions are Agricultural Soil and Water Engineering, and Soil, Water and Plant relationship.
References
- Abd El-Mageed TA, El- Samnoudi IM, Ibrahim AE-AM, Abd El Tawwab AR. 2018. Compost and mulching modulates morphological, physiological responses and water use efficiency in sorghum (bicolor L. Moench) under low moisture regime. Agric Water Manag. 208:431–439.
- Ashraf M. 2003. Relationships between leaf gas exchange characteristics and growth of differently adapted populations of Blue panicgrass (Panicum antidotale Retz.) under salinity or waterlogging. Plant Sci. 165(1):69–75.
- Ashraf M, Bashir A. 2003. Relationship of photosynthetic capacity at the vegetative stage and during grain development with grain yield of two hexaploid wheat (Triticum aestivum L.) cultivars differing in yield. Eur J Agron. 19(2):277–287.
- Bungard RA, McNeil D, Morton JD. 1997. Effects of nitrogen on the photosynthetic apparatus of Clematis vitalba grown at several irradiances. Funct Plant Biol. 24(2):205–214.
- Campbell D, Hurry V, Clarke AK, Gustafsson P, Öquist G. 1998. Chlorophyll fluorescence analysis of cyanobacterial photosynthesis and acclimation. Microbiol Mol Biol Rev. 62(3):667–683.
- Chen J, Kang S, Du T, Qiu R, Guo P, Chen R. 2013. Quantitative response of greenhouse tomato yield and quality to water deficit at different growth stages. Agric Water Manag. 129:152–162.
- Chen RQ, Kang SZ, Hao XM, Li FS, Du TS, Qiu RJ, Chen JL. 2015. Variations in tomato yield and quality in relation to soil properties and evapotranspiration under greenhouse condition. Sci Hortic. 197:318–328.
- Chen S, Wang Z, Guo X, Rasool G, Zhang J, Xie Y, Yousef AH, Shao G. 2019. Effects of vertically heterogeneous soil salinity on tomato photosynthesis and related physiological parameters. Sci Hortic. 249:120–130.
- Chen S, Zhang Z, Wang Z, Guo X, Liu M, Hamoud YA, Zheng J, Qiu R. 2016. Effects of uneven vertical distribution of soil salinity under a buried straw layer on the growth, fruit yield, and fruit quality of tomato plants. Sci Hortic. 203:131–142.
- Chongo G, McVetty P. 2001. Relationship of physiological characters to yield parameters in oilseed rape (Brassica napus L. Can J Plant Sci. 81(1):1–6.
- Delang CO. 2017. Causes and distribution of soil pollution in China. Environmental & Socio-Economic Studies. 5(4):1–17.
- El Mokh F, Nagaz K, Masmoudi MM, Mechlia NB. 2015. Yield and water productivity of drip-irrigated potato under different nitrogen levels and irrigation regime with saline water in arid Tunisia. Am J Plant Sci. 6(04):501.
- FAO. 2016. FAO Statistical Database (Internet). [accessed]. http://faostat.fao.org/default.aspx.
- Garcia A, Marcelis L, García-Sánchez F, Nicolas N, Martínez V. 2007. Moderate water stress affects tomato leaf water relations in dependence on the nitrogen supply. Biologia Plantarum. 51(4):707–712.
- Harris RW. 1992. Root-shoot ratios. J Arboric. 18(1):39–42.
- Hebbar S, Ramachandrappa B, Nanjappa H, Prabhakar M. 2004. Studies on NPK drip fertigation in field grown tomato (Lycopersicon esculentum Mill. Eur J Agron. 21(1):117–127.
- Hubbart S, Peng S, Horton P, Chen Y, Murchie E. 2007. Trends in leaf photosynthesis in historical rice varieties developed in the Philippines since 1966. J Exp Bot. 58(12):3429–3438.
- Jaimez RE, Santos N, Añez B, Vásquez J, Espinoza W. 2008. Photosynthesis of field-grown Arracacha (Arracacia xanthorriza Bancroft) cultivars in relation to root-yield. Sci Hortic. 118(2):100–105.
- Kanai S, Moghaieb RE, El-Shemy HA, Panigrahi R, Mohapatra PK, Ito J, Nguyen NT, Saneoka H, Fujita K. 2011. Potassium deficiency affects water status and photosynthetic rate of the vegetative sink in green house tomato prior to its effects on source activity. Plant Sci. 180(2):368–374.
- Kennedy TL, Suddick EC, Six J. 2013. Reduced nitrous oxide emissions and increased yields in California tomato cropping systems under drip irrigation and fertigation. Agric Ecosyst Environ. 170:16–27.
- Lawlor DW. 1995. Photosynthesis, productivity and environment. J Exp Bot. 46(10):1449–1461.
- Lawlor DW, Cornic G. 2002. Photosynthetic carbon assimilation and associated metabolism in relation to water deficits in higher plants. Plant Cell Environ. 25(2):275–294.
- Li Q, Dong B, Qiao Y, Liu M, Zhang J. 2010. Root growth, available soil water, and water-use efficiency of winter wheat under different irrigation regimes applied at different growth stages in North China. Agric Water Manag. 97(10):1676–1682.
- Li Y, Li J, Gao L, Tian Y. 2018. Irrigation has more influence than fertilization on leaching water quality and the potential environmental risk in excessively fertilized vegetable soils. PLoS ONE. 13(9):e0204570.
- Liu B, Cheng L, Li M, Liang D, Zou Y, Ma F. 2012. Interactive effects of water and nitrogen supply on growth, biomass partitioning, and water-use efficiency of young apple trees. Afr J Agric Res. 7(6):978–985.
- Long SP, Zhu XG, Naidu SL, Ort DR. 2006. Can improvement in photosynthesis increase crop yields? Plant Cell Environ. 29(3):315–330.
- Luo H, Li F. 2018. Tomato yield, quality and water use efficiency under different drip fertigation strategies. Sci Hortic. 235:181–188.
- Man J, Shi Y, Yu Z, Zhang Y. 2016. Root growth, soil water variation, and grain yield response of winter wheat to supplemental irrigation. Plant Prod Sci. 19(2):193–205.
- Mingze Y, Bo L, Tieliang W, Xue F. 2016. Effects of straw size in buried straw layers on water movement in adjacent soil layers. Int J Agric Biol Eng. 9(2):74–84.
- Ozbahce A, Tari AF. 2010. Effects of different emitter space and water stress on yield and quality of processing tomato under semi-arid climate conditions. Agric Water Manag. 97(9):1405–1410.
- Pessarakli M. 2005. Handbook of photosynthesis. 2nd. Boca Raton, FL: CRC Press.
- Rasool G, Xiangping G, Zhenchang W, Sheng C, Alhaj Hamoud Y, Javed Q. 2019. Response of fertigation under buried straw layer on growth, yield, and water-fertilizer productivity of Chinese cabbage under greenhouse conditions. Commun Soil Sci Plant Anal. 50(8):1030–1043.
- Savić S, Stikić R, Radović BV, Bogičević B, Jovanović Z, Šukalović VH-T. 2008. Comparative effects of regulated deficit irrigation (RDI) and partial root-zone drying (PRD) on growth and cell wall peroxidase activity in tomato fruits. Sci Hortic. 117(1):15–20.
- Shuqin J, Fang Z. 2018. Zero growth of chemical fertilizer and Pesticide Use: China's objectives, progress and Challenges. J Resour Ecol. 9(1):50–58.
- Silsbe GM, Kromkamp JC. 2012. Modeling the irradiance dependency of the quantum efficiency of photosynthesis. Limnol Oceanography Methods. 10(9):645–652.
- Souza R, Machado E, Silva J, Lagôa A, Silveira J. 2004. Photosynthetic gas exchange, chlorophyll fluorescence and some associated metabolic changes in cowpea (Vigna unguiculata) during water stress and recovery. Environ Exp Bot. 51(1):45–56.
- Sugiharto B, Miyata K, Nakamoto H, Sasakawa H, Sugiyama T. 1990. Regulation of expression of carbon-assimilating enzymes by nitrogen in maize leaf. Plant Physiol. 92(4):963–969.
- Takai T, Kondo M, Yano M, Yamamoto T. 2010. A quantitative trait locus for chlorophyll content and its association with leaf photosynthesis in rice. Rice. 3(2-3):172–180.
- Ullah I, Hanping M, Chuan Z, Javed Q, Azeem A. 2017. Optimization of irrigation and nutrient concentration based on economic returns, substrate salt accumulation and water use efficiency for tomato in greenhouse. Arch Agronomy Soil Sci. 63(12):1748–1762.
- Wang C, Gu F, Chen J, Yang H, Jiang J, Du T, Zhang J. 2015. Assessing the response of yield and comprehensive fruit quality of tomato grown in greenhouse to deficit irrigation and nitrogen application strategies. Agric Water Manag. 161:9–19.
- Wang X, Wang W, Huang J, Peng S, Xiong D. 2018. Diffusional conductance to CO2 is the key limitation to photosynthesis in salt-stressed leaves of rice (Oryza sativa). Physiol Plant. 163(1):45–58.
- Wang H, Wang F, Wang G, Majourhat K. 2007. The responses of photosynthetic capacity, chlorophyll fluorescence and chlorophyll content of nectarine (Prunus persica var. Nectarina Maxim) to greenhouse and field grown conditions. Sci Hortic. 112(1):66–72.
- Xiukang W, Yingying X. 2016. Evaluation of the effect of irrigation and fertilization by drip fertigation on tomato yield and water use efficiency in greenhouse. Int J Agronomy. 2016. https://doi.org/10.1155/2016/3961903.
- Yuan BZ, Kang YH, Nishiyama S. 2001. Drip irrigation scheduling for tomatoes in unheated greenhouse. Irrig Sci. 20:149–154.
- Zhang S, Gao P, Tong Y, Norse D, Lu Y, Powlson D. 2015. Overcoming nitrogen fertilizer over-use through technical and advisory approaches: A case study from Shaanxi Province, northwest China. Agric Ecosyst Environ. 209:89–99.
- Zhang S, Kong D, Chang X, Zhai L. 2010. Effect of straw deep application on soil water storage capacity. J Northeast Agric Univ. 6:034.
- Zhao Y, Pang H, Wang J, Huo L, Li Y. 2014. Effects of straw mulch and buried straw on soil moisture and salinity in relation to sunflower growth and yield. Field Crops Res. 161:16–25.