ABSTRACT
Plant-specific CDF genes encode a class of transcription factors which play crucial roles in plant flowering, especially in photoperiod-regulated flowering. But little is known about the potential functions of MdCDFs during flower induction in apple. In this study, 11 putative MdCDFs were identified and named systematically. Bioinformatic analysis revealed a functional and structural similarity among the MdCDFs. Analysis of tandem and synteny revealed several paired CDF homologs between apple and Arabidopsis. RT-qPCR revealed that MdCDFs exhibited distinct expression patterns in different apple tissues and most of them displayed high expression levels in flowers and leaves. Further expression profiles of MdCDFs were investigated in different-flowering varieties of apple and in response to sugar treatment. The results indicated that MdCDFs were responsive to floral induction. Meanwhile, the expressions of all MdCDFs were inhibited in buds during different floral induction stages after sugar treatment. The expressions of flowering-related and photoperiod pathway-related genes showed differences and may indicate that most of the MdCDFs may function as a light response flowering inhibitor and could be repressed by the sugar-mediated floral induction. Collectively, the comprehensive analysis of MdCDFs provides valuable information on their expression during floral induction that will serve as a foundation for further studies.
1. Introduction
Transcriptional regulation in plants plays a very important role in many biological processes, including signal transduction and response to environment stress. As a result of the wide range of responses that are regulated at the transcriptional level, there are many plant specific transcription factors with specific regulatory functions that have evolved (Riechmann et al. Citation2000). Transcription factors (TFs), also known as trans-acting factors, can bind to specific promoter sequences of genes and activate or inhibit their transcription. TFs typically contain four functional regions: regions that bind to specific DNA-sequences and regulate transcription, an oligomerization site, and a nuclear localization sequence (Liu et al. Citation1999). TFs can interact with promoter sequences, as well as other transcription factors, and use these interactions under specific conditions to regulate gene expression. TFs are grouped into different gene families and can be further partitioned into subgroups based on the number and spacing of conserved domains and functional domains located outside of the conserved domains (Liu et al. Citation1999).
Dof (DNA binding with one finger) proteins are transcription factors that occur exclusively in plants, typically comprising 200–400 amino acids with a conserved 52 amino acids sequence with a Cys2Cys2-type zinc finger at the N-terminal portion of the protein (Yanagisawa Citation2002). The Dof domain recognizes the specific cis-element 5′-T/AAAG-3′ in the promoter region of its’ target genes (Plesch et al. Citation2001; Yanagisawa Citation2004). The C-terminal region of Dof proteins contains a transcriptional regulation domain with diverse functions including the interaction with various regulatory proteins and the activation of gene expression. The N- and C-terminal regions of Dof proteins may interact with various regulatory proteins or intercept signals to mediate the activation or repression of target genes (Noguero et al. Citation2013), and thereby regulate many biological processes, such as floral induction (Imaizumi et al. Citation2005; Fornara et al. Citation2009), flower abscission (Wei et al. Citation2010), seed germination (Gualberti Citation2002; Gabriele et al. Citation2010; Santopolo et al. Citation2015), and seed development (Diaz et al. Citation2005; Marzabal et al. Citation2008).
The first genome-wide report of Dof genes was completed for Arabidopsis, where 36 Dof-encoding genes and one pseudogene were identified (Yanagisawa Citation2002). Phylogenetic studies using the complete set of amino acid sequences of Dof proteins from Arabidopsis, rice, tomato and Brachypodium, revealed four major clusters of orthologous genes or subfamilies (A, B, C and D) (Lijavetzky et al. Citation2003; Hernando-Amado et al. Citation2012; Corrales et al. Citation2014). Group D contains a cluster of Dof factors whose transcripts oscillate under constant light conditions and are known as Cycling Dof Factors (CDF1-5) (Imaizumi et al. Citation2005; Fornara et al. Citation2009). In Arabidopsis, CDFs control photoperiod-regulated flowering by binding directly to the C2C2-Dof sites in the promoter region of CONSTANS (CO) to modulate the diurnal expression of CONSTANS (CO) and consequently FLOWERING LOCUS T (Ibarra et al. Citation2012) expression (Imaizumi et al. Citation2005). Combining loss-of-function mutations for a set of four CDF genes (CDF1, 2, 3 and 5) results in a photoperiod-insensitive early flowering phenotype by increasing the level of CO mRNA (Fornara et al. Citation2009; Corrales et al. Citation2014). The stability of CDF proteins is compromised under long days by a protein complex composed of a flavin-binding Kelch repeat F-box protein (FKF1) and a gigantean (GI) protein (Imaizumi et al. Citation2005; Sawa et al. Citation2007; Fornara et al. Citation2009). Recent data reported by Corrales (Corrales et al. Citation2014) indicates that tomato CDFs (SlCDFs) function as transcriptional regulators with multiple functions. Specifically, the overexpression of tomato SlCDF1 and SlCDF3 in Arabidopsis confers both drought and salt tolerance.
In other flowering plant species, CDF homologs appear to have a largely conserved function. LATE BLOOMER 2 (LATE2) in Pisum sativum and StCDF1 in Solanum tuberosum function as repressors of flowering and tuberization, respectively, in response to photoperiod (Kloosterman et al. Citation2013; Ridge et al. Citation2016). Although several other functions of CDFs have been reported, little information has been reported pertaining to CDFs in woody plants. More specifically, the presence, number, structure, and function of CDFs in apple is unknown.
The flowering process in woody plants is complex, especially since floral induction and flowering occur in two different growing seasons (Kurokura et al. Citation2013). Apple is one of the most economically valuable tree fruits in the world, and as a result, issues associated with flowering are important topics of study (Xing et al. Citation2015). The flowering process in apple is regulated by many different signaling factors, including cytokinin and gibberellin levels (Mutasa-Gottgens and Hedden Citation2009; Amasino Citation2010), sugar signals (Seo et al. Citation2011; Jeong et al. Citation2015), and several different flowering pathway signals (Mutasa-Gottgens and Hedden Citation2009; Yamaguchi et al. Citation2014). Sugars, which act as a kind of carbon and energy source in plants to support plant growth, may also be involved in the regulation of the transition to flowering (Caspari et al. Citation1998; Shalom et al. Citation2012). Previous studies have reported that soluble sugar levels in leaves and buds change significantly during floral induction in ‘Fuji’ apple (Xing et al. Citation2015). CDFs act redundantly to repress the expression of CO and FT (Imaizumi et al. Citation2005; Fornara et al. Citation2009), which belong to the photoperiodic-induced flowering pathway. Genetic analysis and molecular research have revealed that the CDF gene family plays an important role in floral induction (Fornara et al. Citation2009). Despite the role that both sugars and CDFs play in flowering, whether the expression of CDFs is influenced by sugar-mediated flowering is still unknown.
In the present study, CDFs in the apple genome were identified and their expressions were characterized. Eleven putative CDFs were identified, and their chemical characteristics, phylogenetic relationship, structure, sequence homology were analyzed. Tandem duplication events and the expansion of the gene family were also examined. The expression pattern of the identified CDFs was analyzed in five different tissues (buds, flowers, fruits, leaves, and stems) and in two different varieties (‘Yanfu No. 6’ and ‘Nagafu No. 2’). The response of candidate CDFs in apple buds and leaves during floral induction to exogenous spray applications of sugar was also characterized. The results of the study provide insights into the MdCDF gene family in apple and their potential involvement in floral induction, which are definitely helpful for further analyses.
2. Materials and methods
2.1. Identification of Dof genes in apple
All of the AtDof protein sequences including 4 AtCDFs were acquired from TAIR (http://www.arabidopsis.org). And then we used the protein sequences as queries to conduct a BLASTP search of the apple Genome database (Malus domestica Genome database v1.0, http://www.rosaceae.org/). The obtained MdDof sequences were then aligned using a conserved domain search tool (https://www.ncbi.nlm.nih.gov/Structure/cdd/wrpsb.cgi). The isoelectric point and putative molecular weight of the translated proteins of all of the non-redundant sequences were obtained with the aid of proteomic and sequence analysis tools on the ExPASy proteomics server (http://expasy.org/). DNAMAN was used for the multiple sequence alignments, and sequences were graphically annotated with the Weblogo online tool (http://weblogo.berkeley.edu/logo.cgi).
2.2. Phylogenetic analysis
Multiple alignments of the full-length protein sequences were performed using Clustal Omega (http://www.ebi.ac.uk/Tools/msa/clustalo/). The phylogenetic tree was constructed and drawn using MEGA6.0 software (http://www.megasoftware.net/) (Tamura et al. Citation2013) by the neighbor-joining method and Maximum Likelihood (ML) method with 1000 bootstrap replicates. Only clades with a test value higher than 50 were selected for the consensus tree.
2.3. Chromosomal location, tandem duplication, and synteny analysis
The Genome Database for Rosaceae (http://www.rosaceae.org/) was used to obtain candidate Dof gene annotations and their chromosomal locations. MapDraw was used to display precise gene locations on each chromosome. The Plant Genome Duplication Database (http://chibba.agtec.uga.edu/duplication/) was used to identify syntenic blocks. The diagrams were constructed using Circos version 0.63 (http://circos.ca/). Tandem duplications of MdCDFs were identified based on their physical locations on individual chromosomes in the apple genome. Genes having an adjacent homologous CDF gene on the same apple chromosome with no more than one intervening gene were considered to be tandemly duplicated.
2.4. Analysis of gene structure
The online tool, Multiple Expectation for Motif Elicitation (MEME), version 4.9.1 (http://meme-suite.org/) was used to identify conserved and shared motifs for all of the full-length Dof protein sequences (Bailey et al. Citation2006). The web-based Gene Structure Display Server (http://gsds.cbi.pku.edu.cn) was used to identify CDF exon–intron structures, including exon positions and gene length (Hu et al. Citation2015). A 1.5 kb region upstream from the transcriptional start site of each of MdCDFs was obtained using the apple genome sequence. The upstream regions were queried within the PlantCARE database (http://bioinformatics.psb.ugent.be/webtools/plantcare/html/) to identify putative cis-elements (Tan et al. Citation2014).
2.5. Plant material and spray treatments
Fifty-four uniform six-year-old ‘Fuji’/T337/Malus robusta Rehd. apple trees, growing at the experimental orchard of the Northwest Agriculture and Forestry University in Yangling (108°04′E, 34°16′N), China, were randomly divided into two groups: twenty-seven were treated with sucrose sprays and 27 were sprayed with water as a control. Each treatment group was divided into three blocks with three replicates in each block. The experiment was conducted from 30 to 80 days after full bloom (DAFB) in 2015. Trees were sprayed twice on clear mornings at 29 and 36 DAFB (May 9 and May 16) with 1.5%(w/v) and 2.0% sucrose, respectively, or water (control). All treatments were applied with a low-pressure hand-wand sprayer. Terminal buds on current-year spurs (<5 cm) were collected at 30, 40, 50, 60, 70 and 80 DAFB. Buds, fruits, leaves, and stems were also collected at 50 DAFB, while flowers were collected at full bloom (0 DAFB). All of the sampled tissues were immediately placed in liquid nitrogen and stored at −80 °C for gene expression analyses.
2.6. Analysis of low- and abundant-flowering apple varieties
Buds from two apple varieties (‘Yanfu No. 6’ and ‘Nagafu No. 2’) that exhibit contrasting rates of flowering were collected at 30, 40, 50, 60, 70, and 80 DAFB from 18 uniform six-year-old trees in 2015. ‘Yanfu No. 6’ is a spontaneous mutation of ‘Nagafu No. 2.’ ‘Yanfu No. 6’ flowers more readily and abundantly than ‘Nagafu No. 2.’ ‘Yanfu No. 6’ also exhibits a higher proportion of spur shoots, shorter internodes, larger buds, and a greater number of flowers.
2.7. RNA extraction and cDNA synthesis
Total RNA was extracted from the collected tissue samples using the cetyltrimethylammonium bromide (CTAB) method with slight modifications (Gambino et al. Citation2008). Briefly, 900 μL extraction buffer [including 2% CTAB, 2.5% PVP-40, 2 M NaCl, 25 mM EDTA (pH 8.0), and 2% β-mercaptoethanol] was preheated at 65 °C and placed in 2 mL microcentrifuge tubes. Then, 200 mg of plant tissue were ground to a powder in liquid nitrogen using a mortar and pestle, added to the tubes containing the extraction buffer, and mixed. After shaking each tube vigorously for 5 min and incubating them at 65°C for 30 min, an equal volume of chloroform: isoamyl alcohol (24:1, v/v) was added. The tubes were again vigorously shaken, and then centrifuged at 12,000×g for 10 min at 4 °C. Supernatant was collected into a new tube and re-extracted with an equal volume of chloroform/isoamyl alcohol (24:1, v/v). The supernatant was transferred into a new 2 mL tube and LiCl (3 M final concentration) was subsequently added. The mixture was incubated at −20°C for 4 h and the RNA was selectively pelleted by LiCl after centrifugation at 18,000×g for 20 min at 4°C. The pellet was resuspended in 500 μL of SSTE buffer (10 mM Tris–HCl (pH 8.0), 1 mM EDTA (pH 8.0), 1% SDS, and 1 M NaCl), preheated to 65 °C in an equal volume of chloroform: isoamyl alcohol. The mixture was then centrifuged at 12,000×g for 10 min at 4 °C. The supernatant was transferred to a new microcentrifuge tube, and the RNA was precipitated with 2.5 volumes of cold ethanol at −80 °C for at least 30 min and centrifuged at 12,000×g for 20 min at 4 °C. Finally, the pellets were washed with 70% ethanol and resuspended in diethylpyrocarbonate-treated water. Total RNA integrity was verified by running samples on 2% agarose gels. First-strand cDNA was synthesized from 1 μg of total RNA using a PrimeScript RT Reagent kit with gDNA Eraser (Takara Bio, Shiga, Japan) following the manufacturer's instructions.
2.8. Expression analysis by reverse transcription – quantitative PCR (RT-qPCR)
The expression levels for all of the identified MdCDFs were analyzed by RT-qPCR using gene-specific primer pairs designed with Primer 6.0 (Table S3). Because coding sequences of MdCDF1-1, MdCDF1-2, and MdCDF1-3, as well as MdCDF4-1 andMdCDF4-2, and MdCDF6-1 and MdCDF6-2 were very similar, their amplification products could not be distinguished from each other. The same primer pair was thus used to analyze the expression of MdCDF4-1, MdCDF4-2, and MdCDF6-1, MdCDF6-2. And a single primer pair was used to analyze the expression of MdCDF1-1, MdCDF1-2, and MdCDF1-3. Collectively, seven pairs of primers were designed against 11 MdCDF genes. Each primer pair was tested via standard RT–PCR to check the size specificity of the amplified product by 1% agarose gel electrophoresis.
The PCR mix (20 μL) for RT-qPCR consisted of 2 μL cDNA samples (diluted 1:8), 10 μL 2× SYBR Premix ExTaq II (Takara Bio), 1 μL of each primer (10 μM), and 6 μL distilled deionized H2O. Each RT-qPCR assay was run on an iCycler iQ Real-Time PCR Detection System (Bio-Rad) with an initial denaturation at 95 °C for 30 s, followed by 40 cycles at 95 °C for 30 s, 60 °C for 35 s, and 72 °C for 30 s; 39 cycles for melting curve analysis. The apple EF-1α gene (GenBank accession No. DQ341381) was used as an internal control to normalize all mRNA expression levels. The 2−ΔΔCt method was used to calculate relative expression (Livak and Schmittgen Citation2001).
2.9. Statistical analysis
Data were analyzed using variance (ANOVA) and means were compared using a t-test in SPSS 17.0 program (SPSS Inc., Chicago, IL, USA). Statistical significance was set at P < 0.05. Figures were prepared using Origin 8.0 (Microcal Software Inc., Northampton, MA, USA).
3. Results
3.1. Identification of CDF genes in Malus domestica
To identify the CDF transcription factor genes in the Malus domestica genome, the Arabidopsis CDF protein sequences were used as a query to perform a blast search against the Malus domestica genome (GDR, Genome database for Rosaceae, http://www.rosaceae.org). Meanwhile a total of 60 candidate Dof gene sequences were initially identified in the Malus domestica genome. Each of the identified 60 Dof amino acid sequences exhibited a Dof domain with a significant E value < 1e-5. Subsequent analysis of the Dof-domain genes identified 11 putative CDF genes. The protein sequences of MDP0000467560, MDP0000728083, MDP0000440066 were all the same, while a similar condition occurred for MDP0000282334, MDP0000232292 and MDP0000598637, MDP0000269678. These genes were designated MdCDF1-1 until MdCDF7. The full-length coding sequences of the MdCDFs ranged from 1398 bp (MdCDF2) to 1620 bp (MdCDF4-2). The size of deduced CDF proteins varied from 465 to 539 amino acids (Smallwood et al. Citation2014), with an average of approximately 495 aa. The projected molecular weight (Shalom, et al. Citation2012) varied from 50.89 to 57.34 kDa, and the theoretical pI of the MdCDF genes ranged from 5.56 (MdCDF1-1/2/3) to 6.43 (MdCDF3) ().
Table 1. MdCDF transcription factor genes identified and characterized in Malus domestica.
3.2. Phylogenetic analysis of the CDF gene family in apple
To gain a better understanding of the phylogenetic relationships and evolutionary history of the CDF transcription factor family in M. domestica, an unrooted phylogenetic tree was constructed with the Neighbor-Joining method utilizing 56 MdDof protein sequences with all 36 Arabidopsis Dof protein sequences (Figure S1). The phylogenetic tree analysis indicated that the Dof transcription factor family can be divided into four groups designated Group A to Group D, which could be further divided into 10 subgroups: A1.1, A1.2, A2, B1, B2, C1.1, C1.2, C2, D1, D2. Within the groups, subgroup D1 constituted the largest clade containing 16 members (19.4%), with all of the CDFs placed within this subgroup D1. And the subgroup D2 comparing with D1 without the similar structures wasn't defined as CDF family. The phylogenetic relationship between Arabidopsis and M. domestica CDFs were also analyzed by the Neighbor-Joining and Maximum Likelihood method (, Figure S1). All of the 11 MdCDF genes contained a conserved Cys2/Cys2 Zn2+ finger in the Dof domain (Figure S2).
3.3. Chromosomal location and duplication of CDF members
As shown in , ten of the MdCDF genes are dispersed on 6 of the 17 apple chromosomes. One member (MdCDF3), however, could not be anchored on any of the apple chromosomes. Chromosome 14 possesses the most (3 of 11) CDF genes. In contrast, only one MdCDF gene was found on each of chromosomes 6, 13, and 17 (). As gene duplication events occur in the evolution of plant genomes, the duplication of MdCDFs were also analyzed. Adjacent homologous genes on a single chromosome, with no more than one intervening gene, are likely the result of a tandem duplication. Three tandem duplication events, MdCDF1-1/2/3, MdCDF4-1/2, and MdCDF6-1/2, were identified on chromosome 9, 14, and 16, respectively (). To determine the expansion pattern of the CDF gene family in apple, duplicated blocks within the apple genome were examined and compared with the chromosomal locations of all the MdCDFs. Ten paired MdCDF genes (MdCDF2-MdCDF6-2, MdCDF2-MdCDF1-2, MdCDF6-1-MdCDF5, MdCDF6-1-MdCDF4-1, MdCDF6-1-MdCDF7, MdCDF6-2-MdCDF4-1, MdCDF6-2-MdCDF7, MdCDF5 -MdCDF4-1, MdCDF5-MdCDF7, and MdCDF4-1-MdCDF7) were located in paired duplicated genomic regions ( and Table S1). All of the paired duplicated genomic regions containing paired MdCDF genes that were distributed on two different apple chromosomes. Because apple chromosomes 6–14, 9–17, and 13–16 are homologous pairs (Velasco et al. Citation2010), MdCDF1-2/MdCDF2, MdCDF4-1/MdCDF5, MdCDF6-1/MdCDF7 and MdCDF6-2/MdCDF7 were probably produced by a whole-genome duplication event. Meanwhile some gene pairs within the two chromosomes were highly similar to each other. The MdCDF2/MdCDF6-2, MdCDF4-1/MdCDF6-1, MdCDF4-1/MdCDF6-2, MdCDF4-1/MdCDF7, MdCDF5/MdCDF6-1 and MdCDF5/MdCDF7 gene pairs were probably generated by segmental duplications because they are located on different chromosomes, and not homologous. Collectively, these observations indicate that both segmental and tandem duplications of MdCDF genes are evident and likely played an important role in the expansion of this gene family in apple.
3.4. Evolutionary relationship between apple and Arabidopsis CDF gene families
Since Arabidopsis is a commonly-studied model plant species and the functions of AtCDFs have been well-characterized, a comparative syntenic map between apple and Arabidopsis genomes was created () to further analyze the origin, evolutionary history, and potential function of MdCDFs. According to this syntenic map, five paired MdCDF-AtCDF genes, including the following ortholog pairs: MdCDF2-CDF3, MdCDF2-CDF1, MdCDF5-CDF5, MdCDF1-2-CDF3 and MdCDF4-1-CDF5 were located in five pairs of duplicated genomic regions between the apple and Arabidopsis genomes (Table S2). This indicates that many of the apple CDF genes and their Arabidopsis CDF counterparts appear to have been derived from a common ancestor. Therefore, the function of several MdCDFs could be inferred based on their Arabidopsis homologs. This facilitated our interpretation of the potential roles of the various CDF genes in apple.
3.5. Analysis of gene structure and conserved motifs
A phylogenetic tree of the 11 MdCDF protein sequences was constructed by the neighbor-joining method with 1000 bootstrap replicates to further analyze their evolutionary relationships ((A)). The 11 MdCDF genes were analyzed using the Gene Structure Display Server to determine their exon-intron structure. As shown in (B), two exons were predominantly predicted among the MdCDFs, with the exception of MdCDF7 which contained three. The similarity in the structure of MdCDF genes may reflect similarities in their function in apple. Notably, duplicated genes shared the same gene structure. For example, MdCDF1-1/2/3 are duplicated genes and they have the same number of exons and introns. The MEME tool was also used to identify motifs present in the 11 MdCDFs (Figure S3). The MdCDFs shared motifs 1–4, 6–9, 11, and 18, except MdCDF3 which only possesses motifs 1, 3, 7, 9.
3.6. Tissue-specific expression of MdCDF genes
Characterizing the expression profiles of MdCDFs in different apple tissues could provide information that could be used to better understand their potential function. MdCDFs are highly homologous with the CDFs in Arabidopsis thaliana, where these genes have an important role in the photoperiodic regulation of flowering. The primer pairs used in the RT-qPCR analysis were listed in Table S3. Therefore, the expression patterns of MdCDF1, MdCDF2, MdCDF3, MdCDF4, MdCDF5, MdCDF6, and MdCDF7 were examined in different apple tissues (). The expression profiles indicated that 2 out of 7 MdCDFs were constitutively expressed in every organ examined. In contrast, MdCDF3 was barely expressed in flowers and leaves, and MdCDF6 was not expressed in fruits. Other MdCDFs exhibited similar expression patterns. For example, all of the MdCDFs, except MdCDF3, exhibited high levels of expression in flowers. Additionally, MdCDF4, MdCDF5, and MdCDF7 exhibited high expression levels in all of the examined tissues examined, while MdCDF1 and MdCDF2 were preferentially expressed in flowers and leaves. Cluster analysis of gene expression showed that MdCDF1/ MdCDF2 and MdCDF4/MdCDF5 had similar expression patterns in different tissues.
Figure 6. Tissue-specific expression profiles of MdCDF transcription factors. (A) cluster analysis of the expression of MdCDF genes; (B) the expression pattern of MdCDFs determined by RT-qPCR. Each value represents the mean ± standard error of three biological replicates. Means followed by different letters are significantly different at a p ≤ 0.05.
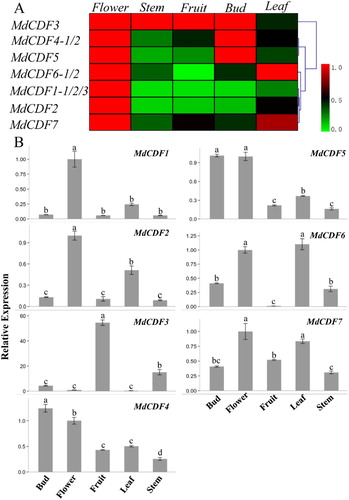
3.7. Expression profiles of MdCDF genes in two different apple varieties
The expression of MdCDFs in buds of ‘Nagafu No. 2’ and ‘Yanfu No. 6’ was determined by RT-qPCR (). ‘Yanfu No.6,’ a bud mutation of ‘Nagafu No. 2,’ flowers more readily and abundantly than ‘Nagafu No.2.’ It was found that the expression pattern of MdCDFs in bud were very different. The expression levels were lower in ‘Yanfu No.6’ than they were in ‘Nagafu No.2’ of MdCDF1 and MdCDF7, which expression patterns were similar. In contrast, four of the analyzed MdCDF genes (MdCDF2, MdCDF3, MdCDF5 and MdCDF6) exhibited higher expression in ‘Yanfu No. 6’ than in ‘Nagafu No. 2’ during the time period that buds were sampled. And the expression MdCDF2, MdCDF5 and MdCDF6 reached the peak at 40 or 50 DAFB. The expression of MdCDF4 exhibited a higher level of expression in ‘Yanfu No.6’ than in ‘Nagafu No.2’ in the early stage of floral induction, whereas the expression was lower in ‘Yanfu No.6’ in the late stage. In addition to MdCDF7, the expression of other MdCDFs increased first and then decreased in both ‘Nagafu No.2’and ‘Yanfu No.6.’ Furthermore, cluster analysis showed that MdCDF2/MdCDF5, MdCDF4/MdCDF6 and MdCDF1/MdCDF7 were expressed similarly in different varieties.
Figure 7. Expression level of MdCDF genes in apple buds of two different varieties. (A) cluster analysis of the expression of MdCDF genes; (B) the expression pattern of MdCDFs by RT-qPCR. Each value represents the mean ± standard error of three biological replicates. Means followed by different letters are significantly different at a p ≤ 0.05.
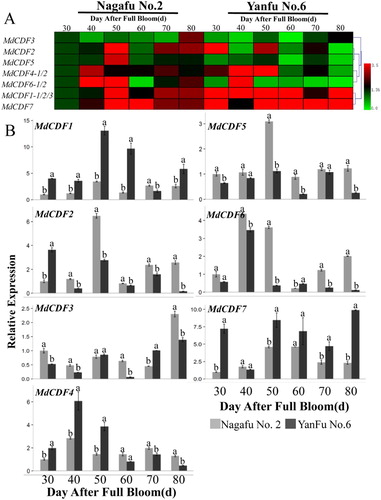
3.8. Expression of MdCDF genes in response to sugar sprays
The responding mechanism and potential function of MdCDFs to exogenous sugars have not been previously investigated. Therefore, we used RT-qPCR to analyze the expression of MdCDFs in apple buds and adjacent leaves in response to the application of sugar sprays. The seven MdCDF genes examined exhibited diversified patterns of expression in response to the sugar sprays that were applied during the floral development phase ( and ). The expression of almost all of the MdCDFs in terminal buds of spur shoots was down-regulated at different time points in response to sucrose sprays (). The expressions of MdCDF1 and MdCDF4 in buds were significantly down-regulated at 30, 70 and 80 DAFB after sucrose spraying. MdCDF2 was significantly up-regulated at 40 and 60 DAFB while down-regulated at other times. In addition, MdCDF3 was only down-regulated at 80 DAFB. Moreover, MdCDF5 and MdCDF7 were significantly down-regulated at 30, 50, 70 DAFB and 50, 60 DAFB, respectively. The expression of MdCDF6 was up-regulated at 60 and 70 DAFB, and significantly down-regulated at other times. In bud-adjacent leaves (), the expression of MdCDF1, MdCDF2, MdCDF6, and MdCDF7 was inhibited, especially at 50 DAFB, and exhibited lower expression levels, relative to untreated leaves, at all time points except 80 DAFB. In contrast, MdCDF4 and MdCDF5 were induced at all of the examined time points and exhibited higher levels of expression relative to their expression in untreated buds and leaves. Meanwhile MdCDF3 was obviously up-regulated at the early stage and down-regulated at the late stage during floral induction. Analysis the cluster of MdCDF expression patterns in buds and leaves found that MdCDF1/MdCDF2 and MdCDF6/MdCDF7, MdCDF1/MdCDF2, MdCDF4/MdCDF5 and MdCDF6/MdCDF7 were very similar to each other, respectively.
Figure 8. Expression level of MdCDF genes in apple terminal buds in response to a sucrose treatment applied during the period of floral induction. (A) cluster analysis of the expression of MdCDF genes; (B) the expression pattern of MdCDFs determined by RT-qPCR. Each value represents the mean ± standard error of three biological replicates. Means followed by different letters are significantly different at a p ≤ 0.05.
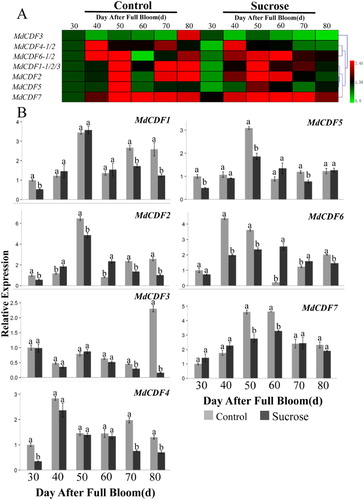
Figure 9. Expression level of MdCDF genes in apple leaves in response to the application of a sucrose treatment applied during the period of floral induction. (A) cluster analysis of the expression of MdCDF genes; (B) the expression pattern of MdCDFs determined by RT-qPCR. Each value represents the mean ± standard error of three biological replicates. Means followed by different letters are significantly different at a p ≤ 0.05.
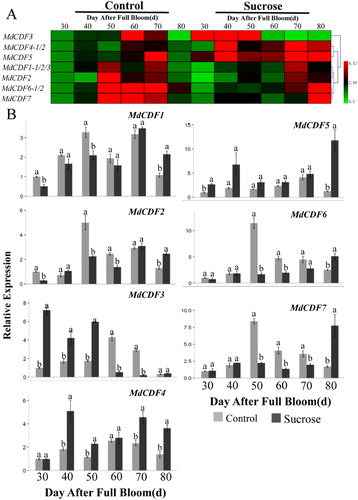
3.9. Expression of photoperiod pathway-related genes and flowering-control genes in response to sugar sprays
To further examine the effect of sugar sprays on gene expression, the RT-qPCR was utilized to examine the expression of select photoperiod pathway-related genes and flowering-control genes in treated and untreated buds and adjacent leaves during the period of floral induction (30-80 DAFB). The photoperiod pathway-related genes examined were MdGI, MdFKF1, MdPHYA, MdPHYB, MdELF3, and MdFWA ( and Figure S4). The flowering-control genes examined were MdCO, MdFT, MdAP1, and MdAFL1. These genes may regulate or be regulated by MdCDFs.
Figure 10. Expression level of photoperiod pathway-related and flowering-control genes in apple buds in response to the application of a sucrose treatment during the period of floral induction. (A) cluster analysis of the expression of photoperiod pathway-related and flowering-control genes; (B) the expression pattern of photoperiod pathway-related and flowering-control genes determined by RT-qPCR. Each value represents the mean ± standard error of three biological replicates. Means followed by different letters are significantly different at a p ≤ 0.05.
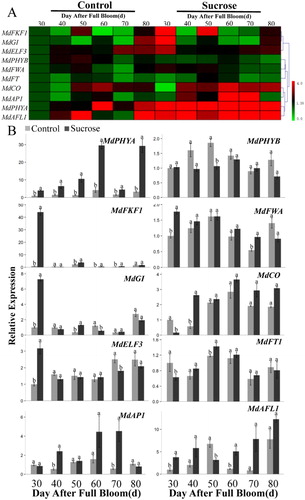
The expression of photoperiod pathway-related genes exhibited distinct differences over the examined time period. All of the photoperiod pathway-related genes (except MdPHYB in buds and MdELF3 in leaves) appeared to be up-regulated at 30 DAFB. The expression of the MdPHYA gene in buds gradually increased during the floral induction period and was significantly higher in sugar-treated buds than untreated buds. In contrast, the MdPHYB expression was significantly lower in treated vs. untreated buds at 40–50 DAFB. In addition, the expression of MdPHYA, MdPHYB, MdGI and MdFKF1 in leaves was up-regulated in response to the sugar sprays at early stage, while MdELF3 and MdFWA were down-regulated at late stage during floral induction.
The flowering-control genes exhibited diverse expression patterns (). MdCO expression in buds gradually increased over the sampling period, and exhibited significantly higher levels in treated buds vs. untreated buds at 40–80 DAFB. The expression of the flower control gene MdFT, was significantly higher in sugar-treated buds than in control buds at 30, 40, and 50 DAFB. The expression of MdAFL1 and MdAP1 genes gradually increased from 30–80 DAFB, and MdAFL1 exhibited high levels of expression at all of the sampled time points, except DAFB 50. The expression of MdAP1 was significantly higher in sugar-treated buds than in untreated, control buds at 40, 60, and 70 DAFB. Meanwhile the expression of MdFT was elevated in response to the sucrose sprays at all of the sampled time points. MdAP1 and MdAFL1 expression gradually increased over the sampling period in both treated and untreated buds.
3.10. Analysis of MdCDF gene family promoters
To further analyze the potential role of MdCDF genes in response to various signals, a 2.0 kb promoter sequence of the candidate MdCDFs, except MdCDF1-2, MdCDF5, and MdCDF6-2, were examined for the presence of cis-elements. In addition to light responsive boxes and stress responsive boxes, promoter regions within the MdCDFs also contained hormone-related cis-elements: including those responsive to MeJA, salicylic acid, gibberellin, auxin, abscisic acid and ethylene. Meanwhile promoter regions also comprised meristem responsive boxes and clock responsive boxes. The light responsive boxes, stress responsive boxes, MeJA and abscisic acid responsive boxes were present in the promoter regions of all of the analyzed MdCDFs (Figure S5). The identified motifs indicated that MdCDFs may be regulated during the growth and development of apple by the presence of various cis-elements in their respective promoters.
4. Discussion
CDF genes, a subgroup of Dof transcription factors that are plant-specific, are ubiquitous in photosynthetic organisms, ranging from green unicellular algae to vascular plants. CDF transcription factors have been implicated in important biological processes in plants. Studies on Dof genes have identified 36 members in Arabidopsis (Yanagisawa Citation2002), 30 members in rice (Lijavetzky et al. Citation2003), 41 in poplar (Yang et al. Citation2006), 26 in barley (Moreno-Risueno et al. Citation2007), 19 in moss (Shigyo et al. Citation2007), 31 in wheat (Shaw et al. Citation2009), 34 in tomato (Cai et al. Citation2013), 46 in maize (Chen and Cao Citation2014), and 25 in grapes (da Silva et al. Citation2016). To date, most of the researches on the function of the CDFs have focused on Arabidopsis (Fornara et al. Citation2009). Therefore, little is known about CDF genes in apple. In the present study, a comparative analysis of Arabidopsis and apple (Malus x domestica) CDF genes was conducted to predict the putative functions of the various MdCDFs, which will be useful to facilitate further functional analyses.
Previous studies demonstrated that CDFs play an important role in flower induction in Arabidopsis and other model plants. The cdf mutants exhibit early flowering in Arabidopsis (Fornara et al. Citation2009). In rice, OsDof4 clusters in the same subgroup as CDFs, and OsDof12 was reported to repress flowering in rice under short-day conditions by down-regulating Hd3a, RFT1, and Ehd1 (Wu et al. Citation2017). In the current study, a bioinformatic approach was used to identify MdCDFs in apple that may be involved in floral induction.
Results indicated the presence of 11 potential CDF genes in the apple genome. An online software tool (ExPASy) was used to characterize the properties of all 11 MdCDF proteins (). In Arabidopsis, the 36 AtDof genes cluster phylogenetically into four groups and nine subgroups (Lijavetzky et al. Citation2003). A combined phylogenetic analysis of both apple and Arabidopsis Dof genes also resulted in four groups but contained a total of ten subgroups (Figure S1). All CDFs cluster in subgroup D1, regardless of species. Further analysis of the identified MdCDF genes confirmed that they all encode a conserved Dof domain containing 52 amino acid residues as part of their DNA sequence (Figure S2). Additionally, results suggested that the D1 subgroup may have undergone duplication events. An analysis of the chromosomal location of MdCDF genes revealed that they are distributed on different chromosomes (). Chromosome 14 possesses the greatest number with 3 MdCDF genes.
One of the main drivers of genomic evolution is gene amplification (Cannon et al. Citation2004), and patterns of gene amplification include tandem repeats and fragment repeats. Three pairs of MdCDFs appear to have resulted from tandem duplication events. Furthermore, according to syntenic analysis, we also found that 7 genes were located in 10 pairs syntenic regions of apple genome (), indicating that their production may be related to large fragment replication of apple chromosome whole-genome duplication. Whole-genome replication, segmental/tandem duplication, and transposition events all appear to have played a role in the expansion of this gene family. Comparative genome analysis is helpful for us to infer the functions of unidentified biological genes through the functions of biological genes that have been studied in depth. Therefore, we can preliminarily infer the functions of apple CDF genes by comparing the functions of CDF family genes in model plant Arabidopsis thaliana. Through syntenic analysis, it was found that 5 pairs of direct homologous genes were identified between the genomes of apple and Arabidopsis thaliana (). However, because the regulation rules of genes would change in the evolutionary process, the specific functions and expression patterns of genes still need to be further studied (Xu et al. Citation2012).
The intron-exon structure of MdCDFs appears to be closely related to the evolutionary relationships between plant species. shows that the intron-exon organization of MdCDFs, and intron number of them, were quite similar to Arabidopsis and rice (Yanagisawa Citation2002). Moreover, a motif analysis indicated that the conserved C2C2-Dof domain ((A)) was uniformly observed in all MdCDF proteins, suggesting that MdCDF TFs are evolutionarily highly conserved in plants.
MdCDF promoters are rich in light responsive boxes, stress responsive boxes and hormone related responsive boxes, indicating that MdCDF genes may respond to light, stress and various hormone signals during all sorts of plant growth and development. The expressions of PIF and TPS are both up-regulated in overexpression of CDF3 Arabidopsis and tomato. In these plants, the photosynthesis efficiency was changed and the resistance was enhanced (Renau-Morata et al. Citation2017) when sucrose content has increased, in the meanwhile, the expression of PIF gene is induced by T6P and sucrose (Liu et al. Citation2011). Therefore, it is speculated that MdCDFs may play an important role in plant development and stress resistance mediated by sugar signals. However, how these functional elements regulate the expression of MdCDF and the specific regulatory mode remains to be studied.
The Dof transcription factor family is a large group of TFs that is found specifically in plants (Yanagisawa Citation2002). CDF transcription factors are key regulators of the timing of CO and FT expression, and the regulation of CDF expression by a circadian clock makes them a primary means by which to shut down CO and FT expression at the beginning of each day. Patterns of gene expression provide important clues for identifying potential gene function. Therefore, we conducted a RT-qPCR analysis of MdCDF expression in root, leaf, stem, bud, and flower tissues to obtain information on the possible roles of MdCDFs in apple growth and development (). Results indicated that MdCDFs exhibit a variety of different expression patterns, suggesting that they have various functions in apple development. MdCDF3, unlike other genes, is highly expressed in fruits and stem, while there is almost no expression in other orangs, indicating that its potential function may have diverged from other MdCDFs. And the gene may be involved in fruit and stem development. In accordance to CDF and OsDof12 expression patterns that were examined in vascular tissues, inflorescences and buds in Arabidopsis and rice respectively, the expression of most of the MdCDF genes was highest in leaves, buds, and flowers (Fornara et al. Citation2009; Li et al. Citation2009). Gene expression in leaves and buds are highly integrated during floral induction and are always independently analyzed for floral biology (Monerri et al. Citation2011). The high expression levels of some of the MdCDFs in leaves and buds suggest that they may play an important role in floral induction and development.
In order to investigate the potential role of MdCDFs in flowering, the expression of selected MdCDFs was analyzed in two different apple varieties that exhibit distinctly different-flowering intensities (). The candidate MdCDF genes showed different expression patterns during the flowering induction of these two varieties. Expressions of all of the selected MdCDFs increased over the time period that flower induction occurs in both varieties. MdCDF1/7 and MdCDF2/3/5/6 exhibited opposite expression profiles during the floral induction period. The expressions of MdCDF1 and MdCDF7 were significantly higher in ‘Yanfu No. 6’ than that in ‘Nagafu No. 2,’ while MdCDF2, MdCDF3, MdCDF5 and MdCDF6 showed opposite phenomenon. That may suggest the genes maybe play contrary roles in the induction process. And we also find that the expression of most of the MdCDFs initially increased and then decreased in both ‘Nagafu No. 2’ and ‘Yanfu No. 6’ trees. The genes except MdCDF1 and MdCDF7 may have been involved in inhibiting flowering, but further research is needed to fully elucidate this phenomenon.
Sugars, which is the main source of energy within plants, regulates flowering by modifying the balance between source and sink tissues (Xing et al. Citation2015). The use of sugar sprays can be a useful way to analyze this with controlled applications. In the current study, sugar sprays were used to help reveal the potential role of MdCDFs in sugar-mediated floral induction. Thus, the expression pattern of MdCDFs in buds and leaves in response to the application of sucrose sprays was analyzed ( and ). Previous studies have demonstrated that sugars play an important role during the floral induction period, and the application of exogenous sugar was reported to increase the rate of flowering in apple trees (Du et al. Citation2017). Our results indicate that exogenous sugar sprays almost completely inhibited the expression of the flowering-related MdCDFs in buds. These results are consistent with the high flowering rate reported in sugar-treated trees (Du et al. Citation2017), suggesting that flowering is inhibited by high levels of MdCDFs or at least regulated in some fashion by carbohydrates. MdCDF3/4/5 expression increased in leaves, which differed from the expression level observed for other flowering-related MdCDF genes in response to a sugar treatment. This indicates that there might be differences in their functions in the leaves. In Arabidopsis, CDF genes participate in photoperiod-regulated flowering (Imaizumi et al. Citation2005). The lower-level of expression of MdCDFs in sugar-treated buds is consistent with the higher flowering rate observed in response to the sugar sprays. MdCDFs were down-regulated in response to the sugar treatment, indicating that they may involve in sugar signaling. The expression of photoperiod pathway-related genes and flowering-control genes in response to the sugar treatment was also examined. Results indicated that both sets of genes were up-regulated in buds and leaves in response to the sugar sprays. The up-regulated genes include MdGI, MdFKF1, MdPHYA, MdCO, and MdFT (). In contrast, the expression of MdPHYB was down-regulated. These results suggest that sucrose has a positive influence on floral induction and development. Meanwhile the differential expression genes may cause the diverse expression patterns of MdCDFs.
In summary, a total of 56 MdDof genes, including 11 MdCDFs, were identified in the apple genome and were subjected to a systematic bioinformatic analysis. Based on a phylogenetic analysis, the MdDof genes were grouped into four clusters, with MdCDFs being clustered in group D. Gene chromosome location and synteny analysis of MdCDFs in the apple genome provided valuable information on the evolution and expansion of this gene family. The expression pattern of MdCDFs in different apple tissues (buds, flowers, fruits, leaves and stems) differed over the floral induction period, and reveals their diversified spatiotemporal pattern of expression. The expression of the majority of MdCDFs was highest in in flowers, leaves, and buds. In addition, the expression patterns of the candidate MdCDF genes in response to the application of sugar sprays indicate that MdCDFs may have an inhibiting function in response to flower development. Collectively, the data obtained in the current study provides new information on apple MdCDFs and can serve as a foundation for further functional studies.
Supplemental Material
Download MS Excel (13.9 KB)Acknowledgements
Siyan Qi, Libo Xing and Mingyu Han designed and conceived the experiments, carried out the principal bioinformatics analysis, and drafted the manuscript. Wenchun Ma, Na An, Xiuhua Gao and Lisha Du performed the experiments. Siyan Qi edited the data, figures and tables. Dong Zhang, Caiping Zhao and Juanjuan Ma contributed reagents/materials/analysis tools. All authors read and approved the final manuscript.
Disclosure statement
No potential conflict of interest was reported by the authors.
Additional information
Funding
Notes on contributors
Siyan Qi
Siyan Qi received her M.S. degree in Agronomy from Northwest A&F University in 2019. Her recent research focuses on apple flower induction.
Wenchun Ma
Wenchun Ma received her M.S. degree in Agronomy from Northwest A&F University in 2020. Her recent research focuses on apple flower induction.
Na An
Na An is an Associate Professor at College of Life Science, Northwest A&F University. She received her Ph.D. degree in Agronomy from Northwest A & F University in 2018. Her recent research focuses on the mechanism of apple flower induction and the protection of genetic resources of medicinal plants.
Xiuhua Gao
Xiuhua Gao is a graduate student. Her recent research focuses on apple flower induction.
Lisha Du
Lisha Du received her M.S. degree in Agronomy from Northwest A&F University in 2017. Her research focused on apple flower induction.
Dong Zhang
Dong Zhang is an Associate Professor at College of Horticulture, Northwest A&F University. He received his Ph.D. degree in Agronomy from Zhejiang University in 2012. His recent research focuses on development biology of apple and intensive and efficient cultivation techniques of dwarf anvil.
Juanjuan Ma
Juanjuan Ma is a lecturer at College of Horticulture, Northwest A&F University. She received her Ph.D. degree in Science from University of Chinese Academy of Sciences in 2014. Her research focuses on the molecular mechanism of cytokinin promoting the generation of apple branches.
Caiping Zhao
Caiping Zhao is an Associate Professor at College of Horticulture, Northwest A&F University. She received her Ph.D. degree in Science from Nanjing Agricultural University in 2005. She mainly engaged in fruit tree genetics and breeding and biological technology fruit tree cultivation physiological research.
Mingyu Han
Mingyu Han was a Professor at College of Horticulture, Northwest A&F University. He received his Ph.D. in Agronomy from Northwest A & F University in 2009. His research focused on germplasm resources and developmental biology of fruit trees, including peach germplasm resources and genetic breeding and the molecular mechanism of apple flowering.
Libo Xing
Libo Xing is Associate Professor at College of Horticulture, Northwest A&F University. His research focused on the developmental biology of fruit trees, and the key content is apple flower induction and their regulation theory. His research aims to understand the molecular regulatory mechanisms of apple floral induction, and solve the serious problems, such as fewer and inferior flower buds, a long juvenile phase, and alternate bearing in ‘Fuji' apple variety.
References
- Amasino R. 2010 Mar. Seasonal and developmental timing of flowering. Plant J. 61:1001–1013. doi: 10.1111/j.1365-313X.2010.04148.x
- Bailey TL, Williams N, Misleh C, Li WW. 2006 Jul. MEME: discovering and analyzing DNA and protein sequence motifs. Nucleic Acids Res. 1(34):W369–W373. doi: 10.1093/nar/gkl198
- Cai X, Zhang Y, Zhang C, Zhang T, Hu T, Ye J, Zhang J, Wang T, Li H, Ye Z. 2013. Genome-wide analysis of plant-specific Dof transcription factor family in tomato. J Integr Plant Biol. 55:552–566. doi: 10.1111/jipb.12043
- Cannon SB, Mitra A, Baumgarten A, Young ND, May G. 2004 Jun. The roles of segmental and tandem gene duplication in the evolution of large gene families in Arabidopsis thaliana. BMC Plant Biol. 1(4):10. doi: 10.1186/1471-2229-4-10
- Caspari HW, Lang A, Alspach P. 1998. Effects of girdling and leaf removal on fruit set and vegetative growth in grape. Am J Enol Viticult. 49:359–366.
- Chen Y, Cao J. 2014. Comparative analysis of Dof transcription factor family in maize. Plant Mol Biol Report. 33:1245–1258. doi: 10.1007/s11105-014-0835-9
- Corrales AR, Nebauer SG, Carrillo L, Fernandez-Nohales P, Marques J, Renau-Morata B, Granell A, Pollmann S, Vicente-Carbajosa J, Molina RV, et al. 2014 Mar. Characterization of tomato cycling Dof factors reveals conserved and new functions in the control of flowering time and abiotic stress responses. J Exp Bot. 65:995–1012. doi: 10.1093/jxb/ert451
- da Silva DC, da Silveira Falavigna V, Fasoli M, Buffon V, Porto DD, Pappas GJ, Jr., Pezzotti M, Pasquali G, Revers LF. 2016. Transcriptome analyses of the Dof-like gene family in grapevine reveal its involvement in berry, flower and seed development. Hortic Res. 3:16042. doi: 10.1038/hortres.2016.42
- Diaz I, Martinez M, Isabel-LaMoneda I, Rubio-Somoza I, Carbonero P. 2005 Jun. The DOF protein, SAD, interacts with GAMYB in plant nuclei and activates transcription of endosperm-specific genes during barley seed development. Plant J. 42:652–662. doi: 10.1111/j.1365-313X.2005.02402.x
- Du L, Qi S, Ma J, Xing L, Fan S, Zhang S, Li Y, Shen Y, Zhang D, Han M. 2017 Nov. Identification of TPS family members in apple (Malus domestica Borkh. and the Effect of Sucrose Sprays on TPS Expression and Floral Induction. Plant Physiol Biochem. 120:10–23. doi: 10.1016/j.plaphy.2017.09.015
- Fornara F, Panigrahi KC, Gissot L, Sauerbrunn N, Ruhl M, Jarillo JA, Coupland G. 2009 Jul. Arabidopsis DOF transcription factors act redundantly to reduce CONSTANS expression and are essential for a photoperiodic flowering response. Dev Cell. 17:75–86. doi: 10.1016/j.devcel.2009.06.015
- Gabriele S, Rizza A, Martone J, Circelli P, Costantino P, Vittorioso P. 2010 Jan. The Dof protein DAG1 mediates PIL5 activity on seed germination by negatively regulating GA biosynthetic gene AtGA3ox1. Plant J. 61:312–323. doi: 10.1111/j.1365-313X.2009.04055.x
- Gambino G, Perrone I, Gribaudo I. 2008 Nov–Dec. A Rapid and effective method for RNA extraction from different tissues of grapevine and other woody plants. Phytochem Anal. 19:520–525. doi: 10.1002/pca.1078
- Gualberti G. 2002. Mutations in the Dof zinc finger genes DAG2 and DAG1 influence with opposite Effects the germination of Arabidopsis Seeds. Plant Cell. 14:1253–1263. doi: 10.1105/tpc.010491
- Hernando-Amado S, Gonzalez-Calle V, Carbonero P, Barrero-Sicilia C. 2012 Nov. The family of DOF transcription factors in Brachypodium distachyon: phylogenetic comparison with rice and barley DOFs and expression profiling. BMC Plant Biol. 5(12):202. doi: 10.1186/1471-2229-12-202
- Hu B, Jin J, Guo AY, Zhang H, Luo J, Gao G. 2015 Apr. GSDS 2.0: an upgraded gene feature visualization server. Bioinformatics. 15(31):1296–1297. doi: 10.1093/bioinformatics/btu817
- Ibarra CA, Feng X, Schoft VK, Hsieh TF, Uzawa R, Rodrigues JA, Zemach A, Chumak N, Machlicova A, Nishimura T, et al. 2012 Sep. Active DNA demethylation in plant companion cells reinforces transposon methylation in gametes. Science. 14(337):1360–1364. doi: 10.1126/science.1224839
- Imaizumi T, Schultz TF, Harmon FG, Ho LA, Kay SA. 2005 Jul. FKF1F-BOX protein mediates cyclic degradation of a repressor of CONSTANS in Arabidopsis. Science. 8(309):293–297. doi: 10.1126/science.1110586
- Jeong EY, Seo PJ, Woo JC, Park CM. 2015 May. AKIN10 delays flowering by inactivating IDD8 transcription factor through protein phosphorylation in Arabidopsis. BMC Plant Biol. 1(15):110. doi: 10.1186/s12870-015-0503-8
- Kloosterman B, Abelenda JA, MdMC G, Oortwijn M, de Boer JM, Kowitwanich K, Horvath BM, van Eck HJ, Smaczniak C, Prat S, et al. 2013. Naturally occurring allele diversity allows potato cultivation in northern latitudes. Nature. 495:246–250. doi: 10.1038/nature11912
- Kurokura T, Mimida N, Battey NH, Hytonen T. 2013 Nov. The regulation of seasonal flowering in the rosaceae. J Exp Bot. 64:4131–4141. doi: 10.1093/jxb/ert233
- Li D, Yang C, Li X, Gan Q, Zhao X, Zhu L. 2009 May. Functional characterization of rice OsDof12. Planta. 229:1159–1169. doi: 10.1007/s00425-009-0893-7
- Lijavetzky D, Carbonero P, Vicente-Carbajosa J. 2003 Jul. Genome-wide comparative phylogenetic analysis of the rice and Arabidopsis Dof gene families. BMC Evol Biol. 23(3):17. doi: 10.1186/1471-2148-3-17
- Liu LS, White MJ, MacRae TH. 1999 Jun. Transcription factors and their genes in higher plants. functional domains, evolution and regulation. Eur J Biochem. 262:247–257. doi: 10.1046/j.1432-1327.1999.00349.x
- Liu Z, Zhang Y, Liu R, Hao H, Wang Z, Bi Y. 2011 Oct. Phytochrome interacting factors (PIFs) are essential regulators for sucrose-induced hypocotyl elongation in Arabidopsis. J Plant Physiol. 15(168):1771–1779. doi: 10.1016/j.jplph.2011.04.009
- Livak KJ, Schmittgen TD. 2001 Dec. Analysis of relative gene expression data using real-time quantitative PCR and the 2(-Delta Delta C(T)) method. Methods. 25:402–408. doi: 10.1006/meth.2001.1262
- Marzabal P, Gas E, Fontanet P, Vicente-Carbajosa J, Torrent M, Ludevid MD. 2008 Jul. The maize Dof protein PBF activates transcription of gamma-zein during maize seed development. Plant Mol Biol. 67:441–454. doi: 10.1007/s11103-008-9325-5
- Monerri C, Fortunato-Almeida A, Molina RV, Nebauer SG, García-Luis A, Guardiola JL. 2011. Relation of carbohydrate reserves with the forthcoming crop, flower formation and photosynthetic rate, in the alternate bearing ‘salustiana’ sweet orange (Citrus sinensis L.). Sci Hortic. 129:71–78. doi: 10.1016/j.scienta.2011.03.009
- Moreno-Risueno MA, Martinez M, Vicente-Carbajosa J, Carbonero P. 2007 Apr. The family of DOF transcription factors: from green unicellular algae to vascular plants. Mol Genet Genomics. 277:379–390. doi: 10.1007/s00438-006-0186-9
- Mutasa-Gottgens E, Hedden P. 2009. Gibberellin as a factor in floral regulatory networks. J Exp Bot. 60:1979–1989. doi: 10.1093/jxb/erp040
- Noguero M, Atif RM, Ochatt S, Thompson RD. 2013 Aug. The role of the DNA-binding One zinc finger (DOF) transcription factor family in plants. Plant Sci Int J Exp Plant Biol. 209:32–45.
- Plesch G, Ehrhardt T, Mueller-Roeber B. 2001. Involvement of TAAAG elements suggests a role for Dof transcription factors in guard cellspecific gene expression. Plant J. 28:455–464. doi: 10.1046/j.1365-313X.2001.01166.x
- Renau-Morata B, Molina RV, Carrillo L, Cebolla-Cornejo J, Sanchez-Perales M, Pollmann S, Dominguez-Figueroa J, Corrales AR, Flexas J, Vicente-Carbajosa J, et al. 2017. Ectopic expression of CDF3 genes in tomato Enhances Biomass production and Yield under Salinity stress conditions. Front Plant Sci. 8:660. doi: 10.3389/fpls.2017.00660
- Ridge S, Sussmilch FC, Hecht V, Vander Schoor JK, Lee R, Aubert G, Burstin J, Macknight RC, Weller JL. 2016 Oct. Identification of LATE BLOOMER2 as a CYCLING DOF FACTOR Homolog reveals conserved and Divergent Features of the flowering response to photoperiod in Pea. Plant Cell. 28:2545–2559. doi: 10.1105/tpc.15.01011
- Riechmann JL, Heard J, Martin G, Reuber L, Jiang CZ, Keddie J, Adam L, Pineda O, Ratcliffe OJ, Samaha RR, et al. 2000 Dec. Arabidopsis transcription factors: genome-wide comparative analysis among eukaryotes. Science. 15(290):2105–2110. doi: 10.1126/science.290.5499.2105
- Santopolo S, Boccaccini A, Lorrai R, Ruta V, Capauto D, Minutello E, Serino G, Costantino P, Vittorioso P. 2015 Mar. DOF AFFECTING GERMINATION 2 is a positive regulator of light-mediated seed germination and is repressed by DOF AFFECTING GERMINATION 1. BMC Plant Biol. 4(15):72. doi: 10.1186/s12870-015-0453-1
- Sawa M, Nusinow DA, Kay SA, Imaizumi T. 2007 Oct. FKF1 and GIGANTEA complex formation is required for day-length measurement in Arabidopsis. Science. 12(318):261–265. doi: 10.1126/science.1146994
- Seo PJ, Ryu J, Kang SK, Park C-M. 2011. Modulation of sugar metabolism by an INDETERMINATE DOMAIN transcription factor contributes to photoperiodic flowering in Arabidopsis. Plant J. 65:418–429. doi: 10.1111/j.1365-313X.2010.04432.x
- Shalom L, Samuels S, Zur N, Shlizerman L, Zemach H, Weissberg M, Ophir R, Blumwald E, Sadka A. 2012. Alternate bearing in citrus: changes in the expression of flowering control genes and in global gene expression in ON- versus OFF-crop trees. PLoS One 7:e46930. doi: 10.1371/journal.pone.0046930
- Shaw LM, McIntyre CL, Gresshoff PM, Xue GP. 2009 Nov. Members of the Dof transcription factor family in Triticum aestivum are associated with light-mediated gene regulation. Funct Integr Genomics. 9:485–498. doi: 10.1007/s10142-009-0130-2
- Shigyo M, Tabei N, Yoneyama T, Yanagisawa S. 2007 Jan. Evolutionary processes during the formation of the plant-specific Dof transcription factor family. Plant Cell Physiol. 48:179–185. doi: 10.1093/pcp/pcl044
- Smallwood SA, Lee HJ, Angermueller C, Krueger F, Saadeh H, Peat J, Andrews SR, Stegle O, Reik W, Kelsey G. 2014 Aug. Single-cell genome-wide bisulfite sequencing for assessing epigenetic heterogeneity. Nat Methods. 11:817–820. doi: 10.1038/nmeth.3035
- Tamura K, Stecher G, Peterson D, Filipski A, Kumar S. 2013 Dec. MEGA6: molecular evolutionary Genetics analysis version 6.0. Mol Biol Evol. 30:2725–2729. doi: 10.1093/molbev/mst197
- Tan Y, Wang S, Liang D, Li M, Ma F. 2014 Jun. Genome-wide identification and expression profiling of the cystatin gene family in apple (Malus x domestica Borkh.). Plant Physiol Biochem. 79:88–97. doi: 10.1016/j.plaphy.2014.03.011
- Velasco R, Zharkikh A, Affourtit J, Dhingra A, Kalyanaraman A, Fontana P, Bhatnagar SK, Troggio M, Pruss D, Salvi S, et al. 2010. The genome of the domesticated apple (Malus x domestica Borkh.). Nat Gen. 42:833–839. doi: 10.1038/ng.654
- Wei PC, Tan F, Gao XQ, Zhang XQ, Wang GQ, Xu H, Li LJ, Chen J, Wang XC. 2010 Jul. Overexpression of AtDOF4.7, an Arabidopsis DOF family transcription factor, induces floral organ abscission deficiency in Arabidopsis. Plant Physiol. 153:1031–1045. doi: 10.1104/pp.110.153247
- Wu Q, Liu X, Yin D, Yuan H, Xie Q, Zhao X, Li X, Zhu L, Li S, Li D. 2017 Oct. Constitutive expression of OsDof4, encoding a C2-C2 zinc finger transcription factor, confesses its distinct flowering effects under long- and short-day photoperiods in rice (Oryza sativa L. BMC Plant Biol. 19(17):166. doi: 10.1186/s12870-017-1109-0
- Xing LB, Zhang D, Li YM, Shen YW, Zhao CP, Ma JJ, An N, Han MY. 2015 Oct. Transcription profiles reveal sugar and hormone signaling Pathways Mediating flower induction in apple (Malus domestica Borkh. Plant Cell Physiol. 56:2052–2068. doi: 10.1093/pcp/pcv124
- Xu G, Guo C, Shan H, Kong H. 2012 Jan. Divergence of duplicate genes in exon-intron structure. Proc Natl Acad Sci U S A. 24(109):1187–1192. doi: 10.1073/pnas.1109047109
- Yamaguchi N, Winter CM, Wu MF, Kanno Y, Yamaguchi A, Seo M, Wagner D. 2014 May. Gibberellin acts positively then negatively to control onset of flower formation in Arabidopsis. Science. 9(344):638–641. doi: 10.1126/science.1250498
- Yanagisawa S. 2002 Dec. The Dof family of plant transcription factors. Trends Plant Sci. 7:555–560. doi: 10.1016/S1360-1385(02)02362-2
- Yanagisawa S. 2004 Apr. Dof domain proteins: plant-specific transcription factors associated with diverse phenomena unique to plants. Plant Cell Physiol. 45:386–391. doi: 10.1093/pcp/pch055
- Yang X, Tuskan GA, Cheng MZ. 2006 Nov. Divergence of the Dof gene families in poplar. Arabidopsis, and rice suggests multiple modes of gene evolution after duplication. Plant Physiol. 142:820–830. doi: 10.1104/pp.106.083642