ABSTRACT
Sorghum (Sorghum bicolor (L.) Moench) is an important crop originated in Africa that shows susceptibility to herbivores. In this study, we identified two sorghum genotypes with highly contrasting levels of stem damage caused by the caterpillars of Asian stem borer (Ostrinia furnacalis Guenée). Recombinant inbred lines (RILs) from genetic cross between resistant (BTx623) and susceptible (NOG) sorghum were used to perform a quantitative trait locus (QTL) analysis in the field. Two major QTLs responsible for higher NOG infestation by stem borer in three independent field seasons were detected on chromosomes 7 and 9, interestingly in positions that overlapped with two major QTLs for plant height. As plant height and stem borer damage were highly correlated, we propose that sorghum height-associated morphological or physiological traits could be important for stem borer establishment and/or damage in sorghum.
Introduction
Sorghum (Sorghum bicolor (L.) Moench) is the world’s fifth most important cereal crop in terms of production and area of coverage, after wheat, rice, maize, and barley (Ahmed et al. Citation2000). Because of drought tolerance and high biomass, sorghum became an important asset in the semiarid areas of sub-Saharan Africa (Wortmann et al. Citation2009; Ogbaga et al. Citation2014). For example, in Uganda it takes the second place amongst cereals after maize (Wortmann et al. Citation2009). Sorghum also has great potential as a biofuel crop, as high sorghum biomass (stalks, leaves, and grains) can be converted into liquid (bioethanol and bio-oil), gas (biohydrogen, biogas, and syngas), and solid (biochar) biofuels (Stamenkovic et al. Citation2020). Sorghum is also utilized as feedstock for animals (Ahmed et al. Citation2000), thus complementing an extremely broad spectrum of uses in this economically important C4 plant (Xin et al. Citation2021).
Despite having such enormous potential, serious annual yield losses are reported in sorghum due to environmental stresses (Perumal et al. Citation2018; Fakrudin et al. Citation2021). Biotic stress conditions mainly include pathogens, as well as various types of herbivores, for which the stem borers can heavily reduce the yield of grain crops, including sorghum (van Rensburg and Flett Citation2010; Mulaa et al. Citation2011). In addition to stem borers, other pests such as sugarcane aphids (Melanaphis sacchari Zehntner), greenbugs (Schizaphis graminum Rondani), and shoot fly (Atherigona soccata), and recently the invasive sorghum aphid (Melanaphis sorghi Theobald), represent imminent threats in sorghum production (Zhu-Salzman et al. Citation2004; Satish et al. Citation2009; Wang et al. Citation2013; Mohammed et al. Citation2016; Tetreault et al. Citation2019; Elkins et al. Citation2022). Although plants evolved elaborate defense mechanisms against herbivores (Howe and Jander Citation2008; Wu and Baldwin Citation2010; Guo et al. Citation2019; Wari et al. Citation2022), only certain varieties and/or their wild predecessors show effective resistance to insect pests. This also applies to stem borers that disintegrate plant stems and eventually interrupt nutrition and water transport to developing grains (Muturi et al. Citation2012; Visarada et al. Citation2016). In addition, larvae after hatching start feeding on the young leaves that reduces photosynthesis and diverts essential plant resources from growth to defense. In many crops, such as maize, genetic manipulations, and use of Bacillus thuringiensis (Bt) toxin genes partially relieved stress imposed by insect pests (Li et al. Citation2021). However, this approach requires existence of efficient transformation methods for each target crop and favorable country policies for release and use of GMO plants. Some sorghum cultivars could be transformed but technology is far from routine and sorghum remains recalcitrant to genetic transformation (Visarada et al. Citation2016). Therefore, in addition to use of pesticides for stem borer control (Cui et al. Citation2017) and intercropping and using push–pull strategy (Shiferaw and Dargo Citation2019), traditional breeding and genetic selection methods remain of high importance as novel sources of stem borer resistant germplasm (Ananda et al. Citation2020).
Rapid progress in molecular genetics and access to whole genome sequence in sorghum enabled improvement of sorghum stress resilience (Paterson et al. Citation2009; Zheng et al. Citation2011; Mace et al. Citation2013; Xin et al. Citation2021). Previous studies on abiotic stress resistance, such as drought, already highlighted some major genetic components that regulate drought stress tolerance in sorghum (Crasta et al. Citation1999; Kebede et al. Citation2001; Sanchez et al. Citation2002; Sabadin et al. Citation2012; Borrell et al. Citation2014). Genes for physiological and morphological traits have also been actively investigated in sorghum (Disasa et al. Citation2018; Takanashi et al. Citation2021). Recently, a RAD-seq-based high-density linkage map was constructed to study the genetics of sorghum biomass-related traits, using the Japanese landrace Takakibi (NOG) as novel source of natural variation (Kajiya-Kanegae et al. Citation2020).
The BTx623 (standard) and NOG (landrace) sorghum varieties differ in many morphological and physiological characters as shown by Kajiya-Kanegae et al. (Citation2020). Takakibi is a generic name of grain sorghums used in Japan but its origin probably lies in China, where it was domesticated into local accessions with high genetic diversity (Shehzad and Okuno Citation2015; Ohnishi et al. Citation2019). In contrast, BTx623 is an inbred cultivar of US origin, which is the source of sorghum reference genome (Burow et al. Citation2011). According to population structure analysis, BTx623 and NOG belong to divergent phylogenetic groups, representing Southern African and Asian accessions, respectively. To explore this high genetic diversity, 3710 single nucleotide polymorphism (SNP) markers have been generated by RAD-based sequencing, and genetic information was applied to F6 generation of 213 recombinant inbred lines (RILs) originated from BTx623/ NOG genetic cross (Kajiya-Kanegae et al. Citation2020). In addition to morphological and physiological traits (Kajiya-Kanegae et al. Citation2020), mechanisms that limit excess cadmium accumulation in sorghum grains were recently identified using this resource (Wahinya et al. Citation2022). Furthermore, NB-LRR-encoding genes conferring susceptibility to organophosphate pesticides (Jing et al. Citation2021), and the dominant gene inhibiting awn formation in sorghum (Takanashi et al. Citation2022) were reported with aid of this RIL population.
In the current study, a set of 213 RILs have been planted in field to identify genetic loci associated with insect damage. Tiller production, heading time, and plant height parameters were included as complementary traits. We found that susceptibility of NOG to stem borers associates with two major QTLs located on sorghum chromosomes 7 and 9. As QTLs for height in the RILs were located in very similar position, as those for stem borers, we propose that plant height might be one of the important traits that determine insect boring levels under natural field conditions.
Materials and methods
Plant materials and growth conditions
Two parental lines, BTx623 and NOG, and recombinant inbred lines (RIL) populations were established as previously (Kajiya-Kanegae et al. Citation2020). Seeds obtained from individual RILs in F6 and successive generations were bulked to produce a set of 210 individual genotypes used in the 2016 field season (207 in 2017; 179 in 2018). Seeds were germinated in commercial gardening soil mixture (Takii, Japan) in standard cellular trays. Established seedlings were transplanted to field plot at the Institute of Plant Sciences and Resources (IPSR) (latitude: 34°35′31″N, longitude: 133°46′7″E) in Kurashiki city, Okayama prefecture (Japan) at the age of 3–4 weeks, at spacing of 50 cm between plants in rows and 1 m between rows. Each RIL and parents were planted in three different positions in a 20 × 20 m field plot in 2016 and 2017, and in a single position in 2018. In each plot position, a single plant with multiple tillers was used for data collection. Tillers were scored separately for stem borer and height, and then averaged for each plant to reduce the individual differences between plants. Tillers and heading were scored at plant level as described below. Ortran granular pesticide (Sumitomo, Japan) was applied to soil at approximately 10 g per square meter for reducing early damage from aphids and other herbivores on juvenile plants before establishment. Soil was covered with black plastic sheets to suppress weeds, and each plantlet was introduced into soil via 10 cm opening in the plastic sheet. Plantlets were watered on rainless days with ca. 1 L tap water per plant per day until young seedlings could establish (approximately 10 days after transplant), after which the sorghum was left to natural field and weather conditions.
Phenotyping and field screening for stem borer damage
After initial field observations of BTx623 and NOG parental lines in 2015, F6 and higher generations of RILs and their parents were subjected to full extent field trials with natural herbivore infestation in 2016, 2017, and additional reduced-scale supporting experiment in 2018. During 2016 growth season, total damage on leaves of parental genotypes, caused by chewing herbivores, was scored as percentage of total leaf area removed from each plant at three different dates, on 21st July, 4th August, and 30th August. Due to variable number of tillers in each plant, all values were calculated on tiller basis to avoid a possible bias in plants with more tillers. Practically, leaves attached to each tiller were scored (scale 0% for no visible damage to 100% for complete leaf missing) and then averaged to whole plant (n = 5) if the individual had more than one tiller. In inbred line population experiments, each RIL was observed for the first opened panicle (n = 3), which was denoted as heading time (2016, 2017). At the end of each growing season, plant height (2016, n = 3; 2018, n = 1) and tiller number (n = 3) (2016, 2017) were determined. Afterwards, sorghum plants (2016, 2017, n = 3; 2018, n = 1) were longitudinally cut-open with a sharp knife to inspect for stem borer infestations in each individual tiller. Larval galleries in tillers belonging to separate O. furnacalis larvae were scored and averaged for the whole plant values to represent herbivore damage. In parental line screens in 2015 (n = 10) and 2016 (n = 5), numbers of entry/exit holes (2015) and Ostrinia furnacalis Guenée (Lepidoptera: Crambidae) larvae (2015) in each tiller were also counted and averaged to plant level.
QTL analysis of morphological and phenotypic values
QTL analyses were performed on morphological parameters denominated as plant height (PH), heading time (HT), and tiller number (TN), and on the estimates of stem boring (SB) damage caused by O. furnacalis. Analysis was performed with averaged means from the independent field replicates (n = 3) for morphological characters in 2016 and 2017, and single plant replica (n = 1) in 2018. Stem borer damage was averaged on tiller basis for each plant and then used for analysis with averaged means of field replicates, when available, i.e. in 2016 and 2017 seasons. For exact data interpretations, PH represented maximal distance from soil level to tip of the plant measured by tape measure tool; HT was time from germination to first opened panicle observed on the plant; TN was scored at the end of growing season and represented number of all tillers attached to the same plant; SB means a number of stem tunnels caused by individual Asian stem borer larva obtained after tiller dissection as described above. A high-density genetic map using 3710 SNP markers from 213 F6 RILs was used as previously (Kajiya-Kanegae et al. Citation2020). Software, R studio 2022.07.1 build 554 for Windows and R version 4.2.1 (R Core Team Citation2022), were used to perform QTL mapping and Pearson correlations (Broman et al. Citation2003). QTLs were determined by composite interval mapping (CIM) using R/qtl package (Kajiya-Kanegae et al. Citation2020). LOD significance thresholds for detecting significant QTLs were calculated by performing 1000 iterations using the R/qtl permutation test, and reported in , together with other QTLs that showed LOD values >3. Pearson correlations were performed using the R/corr package (Broman et al. Citation2003).
Table 1. Summary of QTL with LOD > 3 in sorghum RILs (2016–2018).
Results
Sorghum plants transplanted in local field have soon become attacked by various chewing herbivores, mostly lepidopterous ((A,B)), and suffered major damage when stem borers damaged plant stems at maturity ((A–C)). Lack of nutrient supply to panicles caused symptoms known as ‘dead heart’ ((E,F)) and ‘white ear’ ((D)). The main herbivore species attacking sorghum in the field were caterpillars of Asian corn borer (O. furnacalis; (C,D)), which was also responsible for dead heart symptoms ((E,F)). Another herbivore found on sorghum was Loreyi armyworm (Mythimna loreyi Duponchel) which is also feeding on rice (Shinya et al. Citation2016). As chewing damage on leaves from O. furnacalis and M. loreyi could not be clearly distinguished, a total leaf damage from both chewing herbivores is reported in the following text. At the time of heading, sorghum plants were infested by aphids that became dominant but transiently occurring field pest of sorghum in mid-August/September.
Figure 1. Leaf damage on field sorghum during 2016. Sorghum seedlings were transplanted in field plot and observed for herbivores and damage. (A) Leaf damage caused by chewing herbivores on young plants. (B) ‘Shot hole’ damage caused by stem borer during young leaf rolled stage and before moving to stem. (C) Caterpillar of the most abundant chewing herbivore Asian corn borer (O. furnacalis). (D) Adult moth of the same species.
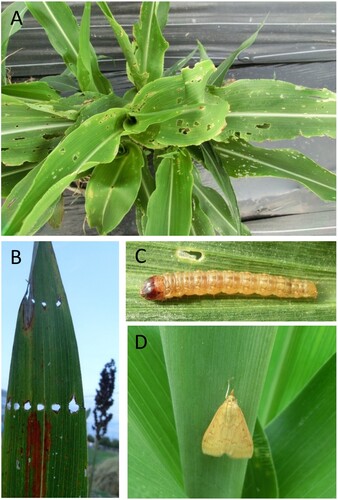
Figure 2. Stem and panicle damage on field sorghum. Field plants were observed for stem and panicle damage and stem borer presence. (A) Displaced purple pigmented pith in stem. (B) Caterpillar of Asian corn stem borer (O. furnacalis) in stem of sorghum showing purple colored internal gallery. (C) Clean/un-attacked section of stem. (D) Effect of stem borer on panicle and grain development and formation of dead hearts; red square area is further depicted in (E), (F). (E) Entry/exit holes of the larval stage of stem borers. (F) Stem borer damage in sorghum stem with caterpillar of Asian corn borer (O. furnacalis). Photos taken during 2016–2018 seasons.
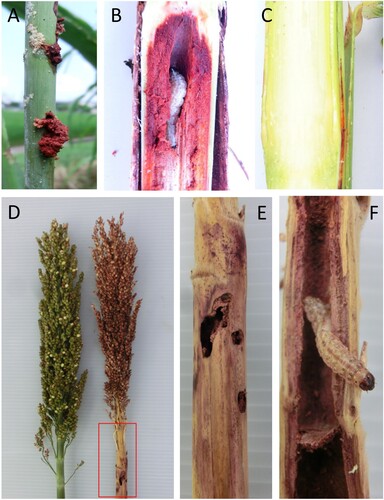
After initial observations of field damage by herbivores, two experimental sorghum varieties previously used in the field experiments in Japan (Kajiya-Kanegae et al. Citation2020) were used as genetic material to dissect sorghum plant–insect interactions. Firstly, BTx623 is an inbred line with short stems, while Takakibi (NOG) is local Japanese landrace with longer tillers and premature stress-induced senescence phenotype (Ohnishi et al. Citation2019). In 2015 and 2016 field seasons, we examined if parental genotypes could show any variation in their anti-herbivore resistance. Although subjective leaf damage from chewing herbivores was somewhat lower in NOG compared to BTx623, levels in three consecutive screens in 2016 remained variable and thus less suitable for genotyping purposes ((A)). In contrast, a clear significant difference was found between BTx623 and NOG in their stem-boring damage that was recorded at the time of termination in 2015 and 2016. In initial screen in 2015, we used 10 individual plants and scored damage in September. First, number of entry holes in each stem, taken as measure of invasive attempt of O. furnacalis larva, was recorded. There were significantly more entry holes in NOG compared to BTx623 ((B)). As proof of establishment, stem galleries (tunnels) and survival (larvae), were counted after cut-opening each tiller. Both parameters were significantly higher in NOG compared to BTx623 plants ((B)). Interestingly, displaced pith ((A)) and internal stem areas around insect tunnels were often purple pigmented ((B)) in contrast to un-attacked yellow–green stems ((C)), suggesting that strong defense reaction and accumulation of secondary metabolites is triggered in sorghum stems.
Figure 3. Quantification of sorghum damage caused by Asian corn borer. Sorghum plants of varieties BTx623 and NOG were scored for damage from O. furnacalis in two successive seasons. (A) Total leaf area damage (%) in three assessments (21st July, 4th August, 30th August 2016). Note that percentage of leaf area damage is reduced in time due to plant growth. (B) Entry holes, stem galleries, and larvae per tiller in BTx623 and NOG plants in two field seasons. Asterisks denote statistically significant differences between cultivars at P < .05 (*) and P < .01 (**) using Student’s t-test; ns indicates not significant.
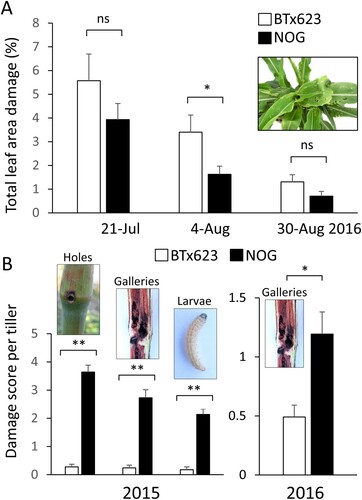
A RIL population derived from cross between BTx623 and NOG (Kajiya-Kanegae et al. Citation2020; ) was then used for identification of QTLs associated with stem borer resistance and/or susceptibility. In this population, genotypes of each RIL were established in F6 generation as previously described (Kajiya-Kanegae et al. Citation2020), and our field experiments were performed using subsequent F8, F9, and F10 populations in each year from 2016 to 2018, respectively. Field data collected in 2016, 2017, and 2018 were applied to high-density linkage map information based on 3710 RAD-seq markers for QTL analysis. From previous data shown in (B), we used number of stem galleries as one of the most reliable signs of O. furnacalis establishment. Using R with qtl package and custom parameters designed by Kajiya-Kanegae et al. (Citation2020), two major QTLs associated with stem borer (SB) infestation and high logarithm of odds (LOD) scores could be consistently found on sorghum’s chromosomes 7 and 9 in each season (; ). Interestingly, when maximal plant height (PH) at the time of stem borer scoring was measured and analyzed, two major QTLs at positions similar to SB loci on chromosomes 7 and 9 were detected (). From loading plots, SB and PH loci were associated with more galleries and higher stems, respectively, in NOG compared to BTx623 (). When heading time (HT) was investigated in 2016 and 2017, a major QTL was identified on chromosome 8 ((A)), which was clearly distinct from the SB and PH loci. On the other hand, a dominant QTL for tiller number was identified on chromosome 7 (; ), which was in close proximity of SB loci on this chromosome. Overall, stem parameters seemed to associate with corn borer activity, which was, however, unrelated to flowering time.
Figure 4. Scheme of RILs used for QTL detection. Parental sorghum plants of NOG (male) and BTx623 (female) were crossed to produce F1 plants. The F1 generation and recurrent self-pollination were used to get F6 (for RAD-sequence and mapping population) and higher generation seeds to establish 213 RILs for this field study. The actual number of RILs in each year varied, as shown in text, because of lines that did not establish in some years.
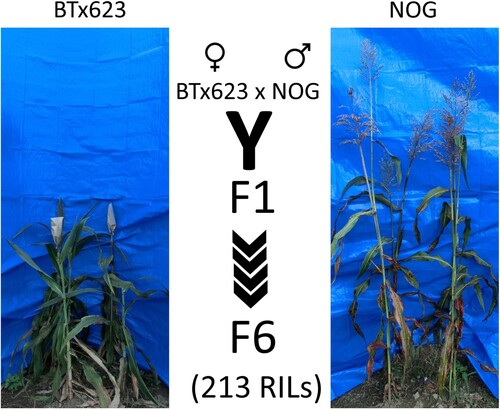
Figure 5. QTLs detected in sorghum RILs in 2016 season. Field data from RILs were used to get QTLs: (A) Heading time (HT) QTL on chromosome 8. (B) Plant height (PH) QTLs on chromosomes 7 and 9. (C) Tiller number QTLs on chromosomes 2, 3, and 7. (D) Stem borer QTLs on chromosomes 7 and 9. Gray horizontal lines show LOD = 3; red lines show significant LODs levels based on a permutation test with 1000 iterations.
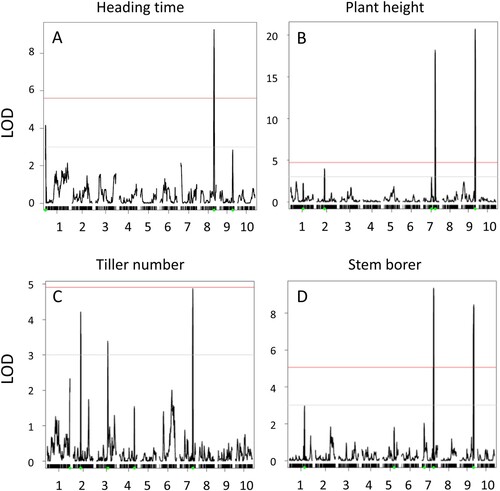
Figure 6. Effector plots for growth and herbivory-related QTLs in sorghum RILs. Effector plots of different herbivory and growth QTLs show that BB genotype (NOG) is associated with higher trait values (SB, PH, and TN) as compared to AA genotype (BTx623).
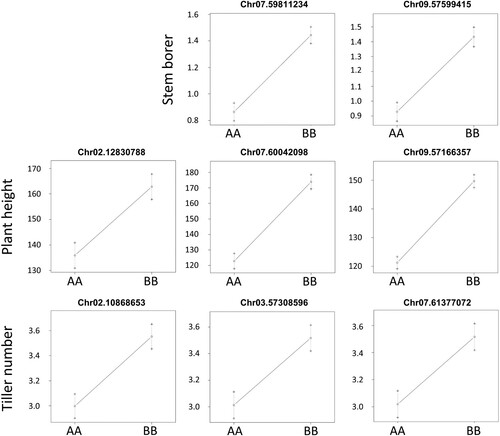
To further corroborate these ideas, correlation matrix for all four parameters was created with corr function in R. As significantly high correlation (r = 0.56) was found between PH and SB (), it further supported our view that plant height and stem-boring activities might be linked as discussed in following section.
Table 2. Correlations between stem borer (SB), plant height PH), tiller number (TN), and heading time (HT) in RILs in 2016.
Discussion
We show that sorghum plants in open environment are under constant attack from chewing herbivores. The main damage was caused by larvae of Asian corn borer O. furnacalis, notorious pest of corn and other seed crops in Asia (He et al. Citation2003; Cui et al. Citation2017). O. furnacalis is closely related to European corn borer (Ostrinia nubilalis Hübner), which is, however, geographically distributed in Europe and USA (Wu et al. Citation2018; Han et al. Citation2020). In our genetic analysis, two major QTLs that associated with higher stem-boring damage in NOG plants were identified.
In recent report by Muturi et al. (Citation2021), QTLs associated with stem borer infestation in sorghum have been reported with a different insect system, showing that, amongst others, QTLs for stem tunneling caused by African stem borer (Busseola fusca Fuller) are located on chromosomes 7 and 9 (Muturi et al. Citation2021). A high correlation between stem tunneling and exit holes (r = 0.70) was reported in the study, which is consistent with O. furnacalis data shown in (B). However, we found a strong correlation between plant height and O. furnacalis galleries (r = 0.56), which was not true for B. fusca (r = 0.12). We assume that species-specific behavioral differences in sorghum colonization by stem-boring insects may be involved in this difference. Overall, our observations suggest that taller plants, despite benefits, such as high biomass, may inherit some disadvantages and attract more damage from insects in the field. At this point, although we found that SB QTLs and PH QTLs are located in similar positions on chromosomes 7 and 9, it is not yet clear if the same genes are responsible for both traits. To this end, fine mapping is necessary for qSB7 and qSB9 to identify the causative genes, that could be compared with known sorghum genes contributing to plant height, Dw1 (Sobic.009G230800, Chr09:57093313–57095643; Hilley et al. Citation2016; Yamaguchi et al. Citation2016) and Dw3 (Sobic.007G163800, Chr07:59821905–59829910; Multani et al. Citation2003).
However, even if genes are identical as purported in this study, it will be still difficult to interpret stem borer phenotype because molecular functions of Dw1 and Dw3 remain unclear (Multani et al. Citation2003; Hilley et al. Citation2016; Yamaguchi et al. Citation2016). In the following text, we discuss potential reasons for taller sorghum plants to be more damaged by O. furnacalis. As taller NOG plants could accommodate more insect individuals in the same plant, relative to short variant Btx623, space sensing by herbivores is proposed as one of the plausible mechanisms. This would mean that larvae can estimate ‘space’ needed for their own development and they would not bore into stems already occupied by (conspecific) competitors. It has been shown previously that tunnel length is often correlated with plant height (PH) and stem diameter (SD) (corn, SD, Ali et al. Citation2015; corn, PH, López-Malvar et al. Citation2021; rice, SD, PH, Hosseini et al. Citation2010), suggesting that taller and/or wider plants may indeed provide a better opportunity for insect development. Previously, QTLs for PH and tunnel length in maize have co-localized on chromosome 3 (Ordas et al. Citation2010) and chromosome 9 (Samayoa et al. Citation2014).
Alternatively, short BTx623 stems could be tougher to penetrate due to more condensed stem structure. This would be consistent with Diezel et al. (Citation2011) showing that plant’s apparency, stem hardness, and pith direct defenses collectively contribute to resistance against stem borers. In addition, Joo et al. (Citation2021) reported that lignin provides inducible stem-specific barrier that protects coyote tobacco (Nicotiana attenuata Torr. ex S. Watson) from stem-boring insects. It is noteworthy that stripped stem borer (Chilo suppressalis Walker) infestation in rice was suppressed by low nitrogen supply which, coincidentally, promoted accumulation of phenolic acids and flavonoids, and increased lignin formation as part of the constitutive rice defense against C. suppressalis (Zheng et al. Citation2021). Higher silica uptake by rice was associated with reduced feeding and impediment of larval penetration (Tripathy and Rath Citation2017). Silicon amendment complemented defense against pink stem borer (Sesamia inferens Walker) in finger millet (Eleusine coracana (L.) Gaertn.) (Jadhao et al. Citation2020), suggesting that plant toughness is indeed one of the most important parameters that control stem borer damage in plants.
Finally, sugar Brix (BR) QTLs were identified in relative proximity of SB7 and SB9.1 (qBR7: Murray et al. Citation2008; Guan et al. Citation2011; Bai et al. Citation2017; qBR9: Shiringani et al. Citation2010; Felderhoff et al. Citation2012). It is plausible that sweetness could be another reason for corn borers to select NOG over BTx623, since NOG would provide a higher nutritional value due to high sugar content. Murray et al. (Citation2008) already reported a significant positive correlation between Brix and plant height, lending support to our hypothesis that tallness in NOG could be linked to higher sugar, which may all together attract stem borers as observed in this study. However, it has also been shown that high sugar concentration in sweet sorghum is not conditionally linked to tallness (Shukla et al. Citation2017), suggesting that newly developed sweet but short sorghum varieties could also be target of stem-boring insects. Further research is needed to unravel the molecular basis of stem borer infestation, possibly as a function of plant height and/or sugar content.
Previously, Kong et al. (Citation2013) and Kajiya-Kanegae et al. (Citation2020) reported robust QTL for heading time on chromosome 8. On the other hand, El Mannai et al. (Citation2012) and Casto et al. (Citation2019) also reported QTLs for sorghum flowering time on other chromosomes, for example, chromosome 2. In our study, main heading time QTL was detected on chromosome 8, suggesting that difference in flowering time may not be directly linked to stem-boring activity in NOG/BTx623 model system. In contrast, significant QTL for tiller number was identified on chromosome 7, which was in the close proximity of SB7. TN7 QTL is consistent with previous report and presence of dominant QTL for vegetative branching on chromosome 7 (Kong et al. Citation2014), although other QTLs on chromosomes 1, 2, 3, and 8 also contributed to this trait.
Conclusions and future perspectives
In the current study, QTLs for stem borer resistance and height in sorghum co-localized on the chromosomes 7 and 9, implying that these traits might be useful targets for selection of plants resistant to stem-boring pests. Hopefully, applied genetics and discovery of new genes in sorghum, and applications in other crops, will support sustainable food and bioenergy production in the future.
Author contribution
Cyprian Osinde performed experiments, collected data, wrote paper; Wataru Sakamoto provided experimental materials; Hiromi Kajiya-Kanegae supervised QTL analyses; Islam S. Sobhy performed experiments, collected data; Arthur K. Tugume and Anthony M. Nsubuga guided in writing; Ivan Galis conceived and designed experiments, collected data, wrote paper.
Acknowledgements
We thank to Japan Society for Promotion of Science (JSPS) for funding of CO travels to Japan under auspices of the Africa-Asia Science platform program; Fiona Wacera and Makoto Ishii (Institute of Plant Sciences and Resources) for help with statistical analyses and field preparation, respectively; members of the Plant-Insect Interaction (PII) Group for invaluable support during field work. This study was supported by MEXT Joint Research Program implemented at Okayama University Institute of Plant Science and Resources.
Disclosure statement
No potential conflict of interest was reported by the author(s).
Additional information
Funding
Notes on contributors
Cyprian Osinde
Cyprian Osinde is currently an Assistant Lecturer at the Department of Plant Sciences Microbiology and Biotechnology, College of Natural Sciences, Makerere University in Uganda. His research interests are in the field of plant physiology, chemical ecology of plant–insect interactions, exploitation of plant defense mechanisms, plant biofertilizers, and stress resistance in plants.
Wataru Sakamoto
Wataru Sakamoto is a Professor at the Plant Light Acclimation Research Group, Institute of Plant Science and Resources, Okayama University in Japan.
Hiromi Kajiya-Kanegae
Hiromi Kajiya-Kanegae is currently a Principal Scientist at Research Center for Agricultural Information Technology, NARO in Japan. She is interested in modeling relationships among various data, including genomes and crop measurements.
Islam S. Sobhy
Islam S. Sobhy is currently a Lecturer at the School of Biosciences, Cardiff University, United Kingdom. His research interests are in the fields of applied plant biology, chemical ecology of plant –microbe –insect interactions, and exploitation of induced plant defense for crop protection.
Arthur K. Tugume
Arthur K. Tugume is currently a Professor of Plant Pathology and Virology and Dean, School of Biosciences at Makerere University in Uganda. His research team at the Department of Plant Sciences, Microbiology and Biotechnology focuses on plant–pathogen interactions especially on vegetatively propagated plants.
Anthony M. Nsubuga
Anthony M. Nsubuga is currently a faculty member at Makerere University, Department of Plant Sciences, Microbiology and Biotechnology. His research team is interested in early detection, characterization, and evolution of vectors and their arboviruses, including gaining some insights into the anthropogenic and ecological drivers of arbovirus disease (re-)emergence.
Ivan Galis
Ivan Galis is working as a Professor at Okayama University, Institute of Plant Science and Resources in Japan. His group of Plant–Insect Interactions focuses on mechanisms of plant defense against herbivorous pests, mainly in monocot crops such as rice and sorghum.
References
- Ahmed MM, Sanders JH, Nell WT. 2000. New sorghum and millet cultivar introduction in Sub-Saharan Africa: impacts and research agenda. Agric Sys. 64:55–65.
- Ali A, Khalil N, Abbas M, Tariq R, Zia-ullah HD. 2015. Plant traits as resistance influencing factors in maize against Chilo partellus (Swinhoe). J Entomol Zool Stud. 3:246–250.
- Ananda GKS, Myrans H, Norton SL, Gleadow R, Furtado A, Henry RJ (2020) Wild sorghum as a promising resource for crop improvement. Front Plant Sci 11: 1108
- Bai CM, Wang CY, Wang P, Zhu ZX, Cong L, Li D, Liu YF, Zheng WJ, Lu XC. 2017. QTL mapping of agronomically important traits in sorghum (Sorghum bicolor L.). Euphytica. 213:285.
- Borrell AK, Mullet JE, George-Jaeggli B, van Oosterom EJ, Hammer GL, Klein PE, Jordan DR. 2014. Drought adaptation of stay-green sorghum is associated with canopy development, leaf anatomy, root growth, and water uptake. J Exp Bot. 65:6251–6263.
- Broman KW, Wu H, Sen S, Churchill GA. 2003. R/qtl: QTL mapping in experimental crosses. Bioinformatics. 19:889–890.
- Burow GB, Klein RR, Franks CD, Klein PE, Schertz KF, Pederson GA, Xin Z, Burke JJ. 2011. Registration of the BTx623/IS3620C recombinant inbred mapping population of sorghum. J Plant Regist. 5:141–145.
- Casto AL, Mattison AJ, Olson SN, Thakran M, Rooney WL, Mullet JE. 2019. Maturity2, a novel regulator of flowering time in Sorghum bicolor, increases expression of SbPRR37 and SbCO in long days delaying flowering. PLoS One. 14:e0212154.
- Crasta OR, Xu WW, Rosenow DT, Mullet J, Nguyen HT. 1999. Mapping of post-flowering drought resistance traits in grain sorghum: association between QTLs influencing premature senescence and maturity. Mol Gen Genet MGG. 262:579–588.
- Cui L, Rui CH, Yang DB, Wang ZY, Yuan HZ. 2017. De novo transcriptome and expression profile analyses of the Asian corn borer (Ostrinia furnacalis) reveals relevant flubendiamide response genes. BMC Genomics. 18:20.
- Diezel C, Kessler D, Baldwin IT. 2011. Pithy protection: Nicotiana attenuata’s jasmonic acid-mediated defenses Are required to resist stem-boring weevil larvae. Plant Physiol. 155:1936–1946.
- Disasa T, Feyissa T, Admassu B, Fetene M, Mendu V. 2018. Mapping of QTLs associated with brix and biomass-related traits in sorghum using SSR markers. Sugar Tech. 20:275–285.
- Elkins BH, Eubanks MD, Faris AM, Wang HH, Brewer MJ. 2022. Landscape complexity has mixed effects on an invasive aphid and its natural enemies in sorghum agroecosystems. Environ Entomol. 51:660–669.
- El Mannai Y, Shehzad T, Okuno K. 2012. Mapping of QTLs underlying flowering time in sorghum [Sorghum bicolor (L.) Moench]. Breed Sci. 62:151–159.
- Fakrudin B, Lakshmidevamma TN, Ugalat J, Gunnaiah R, Gautham Suresh SP, Apoorva KA, Doddamani M, Kadam S, Rashmi K, Mamathashree MN, et al. 2021. Genomic designing for biotic stress resistance in sorghum. In: C Kole, editor. Genomic designing for biotic stress resistant cereal crops. Cham: Springer; p. 213–255.
- Felderhoff TJ, Murray SC, Klein PE, Sharma A, Hamblin MT, Kresovich S, Vermerris W, Rooney WL. 2012. QTLs for energy-related traits in a sweet × grain sorghum [Sorghum bicolor (L.) Moench] mapping population. Crop Sci. 52:2040–2049.
- Guan YA, Wang HL, Qin L, Zhang HW, Yang YB, Gao FJ, Li RY, Wang HG. 2011. QTL mapping of bio-energy related traits in sorghum. Euphytica. 182:431–440.
- Guo JF, Qi JF, He KL, Wu JQ, Bai SX, Zhang TT, Zhao JR, Wang ZY. 2019. The Asian corn borer Ostrinia furnacalis feeding increases the direct and indirect defence of mid-whorl stage commercial maize in the field. Plant Biotechnol J. 17:88–102.
- Han X, Chen RZ, Li LB, Wei X, Qu MB, Klein MG, Wang KQ. 2020. Phylogenetic relationships and biological features reveal that Male Ostrinia furnacalis (Lepidoptera: Crambidae) in Northeast China can be categorized into postmedial line-based clades. Zootaxa. 4786:53–68.
- He KL, Wang ZY, Zhou DR, Wen LP, Song YY, Yao ZY (2003) Evaluation of transgenic Bt corn for resistance to the Asian corn borer (Lepidoptera: Pyralidae). J Econ Entomol 96: 935-940
- Hilley J, Truong S, Olson S, Morishige D, Mullet J. 2016. Identification of Dw1, a regulator of sorghum stem internode length. PLoS One. 11:e0151271.
- Hosseini SZ, Babaeian-Jelodar N, Bagheri N. 2010. Evaluation of resistance to striped stem borer in rice. Biharean Biologist. 4:67–71.
- Howe GA, Jander G. 2008. Plant immunity to insect herbivores. Annu Rev Plant Biol. 59:41–66.
- Jadhao KR, Bansal A, Rout GR. 2020. Silicon amendment induces synergistic plant defense mechanism against pink stem borer (Sesamia inferens Walker.) in finger millet (Eleusine coracana Gaertn.). Sci Rep. 10:4229.
- Jing ZH, Wacera WF, Takami T, Takanashi H, Fukada F, Kawano Y, Kajiya-Kanegae H, Iwata H, Tsutsumi N, Sakamoto W. 2021. NB-LRR-encoding genes conferring susceptibility to organophosphate pesticides in sorghum. Sci Rep. 11:19828.
- Joo Y, Kim H, Kang M, Lee G, Choung S, Kaur H, Oh S, Choi JW, Ralph J, Baldwin IT, Kim SG. 2021. Pith-specific lignification in Nicotiana attenuata as a defense against a stem-boring herbivore. New Phytol. 232:332–344.
- Kajiya-Kanegae H, Takanashi H, Fujimoto M, Ishimori M, Ohnishi N, Wacera WF, Omollo EA, Kobayashi M, Yano K, Nakano M, et al. 2020. RAD-seq-based high-density linkage map construction and QTL mapping of biomass-related traits in sorghum using the Japanese landrace Takakibi NOG. Plant Cell Physiol. 61:1262–1272.
- Kebede H, Subudhi PK, Rosenow DT, Nguyen HT. 2001. Quantitative trait loci influencing drought tolerance in grain sorghum (Sorghum bicolor L. Moench). Theor Appl Genet. 103:266–276.
- Kong WQ, Guo H, Goff VH, Lee TH, Kim C, Paterson AH. 2014. Genetic analysis of vegetative branching in sorghum. Theor Appl Genet. 127:2387–2403.
- Kong WQ, Jin HZ, Franks CD, Kim C, Bandopadhyay R, Rana MK, Auckland SA, Goff VH, Rainville LK, Burow GB, et al. 2013. Genetic analysis of recombinant inbred lines for Sorghum bicolor x Sorghum propinquum. G3-Genes Genomes Genetics. 3:101–108.
- Li GP, Feng HQ, Ji TJ, Huang JR, Tian CH. 2021. What type of Bt corn is suitable for a region with diverse lepidopteran pests: A laboratory evaluation. Gm Crops Food-Biotechnol Agri Food Chain. 12:115–124.
- López-Malvar A, Resendiz Z, Santiago R, Jimenez-Galindo JC, Malvar RA. 2021. Relationships between stalk resistance and corn borers, agronomic traits, and cell wall hydroxycinnamates in a set of recombinant inbred lines from a maize MAGIC population. Agronomy. 11:1132.
- Mace ES, Tai SS, Gilding EK, Li YH, Prentis PJ, Bian LL, Campbell BC, Hu WS, Innes DJ, Han XL, et al. 2013. Whole-genome sequencing reveals untapped genetic potential in Africa’s indigenous cereal crop sorghum. Nat Commun. 4:2320.
- Mohammed R, Are AK, Munghate RS, Bhavanasi R, Polavarapu KKB, Sharma HC. 2016. Inheritance of resistance to sorghum shoot fly, Atherigona soccata in sorghum, Sorghum bicolor (L.) Moench. Front Plant Sci. 7:543.
- Mulaa MM, Bergvinson DJ, Mugo SN, Wanyama JM, Tende RM, De Groote H, Tefera TM. 2011. Evaluation of stem borer resistance management strategies for Bt maize in Kenya based on alternative host refugia. Afr J Biotechnol. 10:4732–4740.
- Multani DS, Briggs SP, Chamberlin MA, Blakeslee JJ, Murphy AS, Johal GS. 2003. Loss of an MDR transporter in compact stalks of maize br2 and sorghum dw3 mutants. Science. 302:81–84.
- Murray SC, Sharma A, Rooney WL, Klein PE, Mullet JE, Mitchell SE, Kresovich S. 2008. Genetic improvement of sorghum as a biofuel feedstock: I. QTL for stem sugar and grain nonstructural carbohydrates. Crop Sci. 48:2165–2179.
- Muturi PW, Mgonja M, Rubaihayo P, Mwololo JK. 2021. QTL mapping of traits associated with dual resistance to the African stem borer (Busseola fusca) and spotted stem borer (Chilo partellus) in sorghum (Sorghum bicolor). Int J Genomics. 2021:1.
- Muturi PW, Rubaihayo P, Mgonja M, Kyamanywa S, Sharma HC, Hash CT. 2012. Novel source of sorghum tolerance to the African stem borer, Busseola fusca. Afr J Plant Sci. 6:295–302.
- Ogbaga CC, Stepien P, Johnson GN. 2014. Sorghum (Sorghum bicolor) varieties adopt strongly contrasting strategies in response to drought. Physiol Plant. 152:389–401.
- Ohnishi N, Wacera WF, Sakamoto W. 2019. Photosynthetic responses to high temperature and strong light suggest potential post-flowering drought tolerance of sorghum Japanese landrace Takakibi. Plant Cell Physiol. 60:2086–2099.
- Ordas B, Malvar RA, Santiago R, Butron A. 2010. QTL mapping for Mediterranean corn borer resistance in European flint germplasm using recombinant inbred lines. BMC Genomics. 11:174.
- Paterson AH, Bowers JE, Bruggmann R, Dubchak I, Grimwood J, Gundlach H, Haberer G, Hellsten U, Mitros T, Poliakov A, et al. 2009. The Sorghum bicolor genome and the diversification of grasses. Nature. 457:551–556.
- Perumal R, Magill CW, Peterson GC, Prom LK, Bashir EM, Tesso T. 2018. Sorghum breeding for biotic stress tolerance. In: B Roney, editor. Achieving sustainable cultivation of sorghum, Volume 1: genetics, breeding and production techniques. Sawston: Burleigh Dodds Science Publishing; p. 189–226.
- R Core Team. 2022. R: A language and environment for statistical computing. R Foundation for Statistical Computing, Vienna, Austria. https://www.R-project.org/.
- Sabadin PK, Malosetti M, Boer MP, Tardin FD, Santos FG, Guimaraes CT, Gomide RL, Andrade CLT, Albuquerque PEP, Caniato FF, et al. 2012. Studying the genetic basis of drought tolerance in sorghum by managed stress trials and adjustments for phenological and plant height differences. Theor Appl Genet. 124:1389–1402.
- Samayoa LF, Butron A, Malvar RA. 2014. QTL mapping for maize resistance and yield under infestation with Sesamia nonagrioides. Mol Breed. 34:1331–1344.
- Sanchez AC, Subudhi PK, Rosenow DT, Nguyen HT. 2002. Mapping QTLs associated with drought resistance in sorghum (Sorghum bicolor L). Plant Mol Biol. 48:713–726.
- Satish K, Srinivas G, Madhusudhana R, Padmaja PG, Reddy RN, Mohan SM, Seetharama N. 2009. Identification of quantitative trait loci for resistance to shoot fly in sorghum [Sorghum bicolor (L.) Moench]. Theor Appl Genet. 119:1425–1439.
- Shehzad T, Okuno K. 2015. QTL mapping for yield and yield-contributing traits in sorghum (Sorghum bicolor (L.) Moench) with genome-based SSR markers. Euphytica. 203:17–31.
- Shiferaw T, Dargo F. 2019. Evaluating sorghum stem borer Chilo partellus (Swinhoe) (Lepidoptera: Crambidae) management alternatives in eastern Ethiopia. Agri Res Technol. 20:556134.
- Shinya T, Hojo Y, Desaki Y, Christeller JT, Okada K, Shibuya N, Galis I. 2016. Modulation of plant defense responses to herbivores by simultaneous recognition of different herbivore-associated elicitors in rice. Sci Rep. 6:32537.
- Shiringani AL, Frisch M, Friedt W. 2010. Genetic mapping of QTLs for sugar-related traits in a RIL population of Sorghum bicolor L. Moench. Theor Appl Genet. 121:323–336.
- Shukla S, Felderhoff TJ, Saballos A, Vermerris W. 2017. The relationship between plant height and sugar accumulation in the stems of sweet sorghum (Sorghum bicolor (L.) Moench). Field Crops Res. 203:181–191.
- Stamenkovic OS, Siliveru K, Veljkovic VB, Bankovic-Ilic IB, Tasic MB, Ciampitti IA, Dalovic IG, Mitrovic PM, Sikora VS, Prasad PVV. 2020. Production of biofuels from sorghum. Renewable Sustainable Energy Rev. 124:109769.
- Takanashi H, Kajiya-Kanegae H, Nishimura A, Yamada J, Ishimori M, Kobayashi M, Yano K, Iwata H, Tsutsumi N, Sakamoto W. 2022. DOMINANT AWN INHIBITOR encodes the ALOG protein originating from gene duplication and inhibits AWN elongation by suppressing cell proliferation and elongation in sorghum. Plant Cell Physiol. 63:901–918.
- Takanashi H, Shichijo M, Sakamoto L, Kajiya-Kanegae H, Iwata H, Sakamoto W, Tsutsumi N. 2021. Genetic dissection of QTLs associated with spikelet-related traits and grain size in sorghum. Sci Rep. 11:9398.
- Tetreault HM, Grover S, Scully ED, Gries T, Palmer NA, Sarath G, Louis J, Sattler SE. 2019. Global responses of resistant and susceptible sorghum (Sorghum bicolor) to sugarcane aphid (Melanaphis sacchari). Front Plant Sci. 10:145.
- Tripathy S, Rath LK. 2017. Silicon induced resistance expression in rice to yellow stem borer. J Entomol Zool Stud. 5:12–15.
- van Rensburg JBJ, Flett BC. 2010. A review of research achievements on maize stem borer, Busseola fusca (Fuller) and Diplodia ear rot caused by Stenocarpella maydis (Berk. Sutton). S Afr J Plant Soil. 27:74–80.
- Visarada K, Prasad GS, Royer M. 2016. Genetic transformation and evaluation of two sweet sorghum genotypes for resistance to spotted stemborer, Chilo partellus (Swinhoe). Plant Biotechnol Rep. 10:277–289.
- Wahinya FW, Yamazaki K, Jing ZH, Takami T, Kamiya T, Kajiya-Kanegae H, Takanashi H, Iwata H, Tsutsumi N, Fujiwara T, Sakamoto W. 2022. Sorghum ionomics reveals the functional SbHMA3a allele that limits excess cadmium accumulation in grains. Plant Cell Physiol. 63:713–728.
- Wang FM, Zhao SM, Han YH, Shao YT, Dong ZY, Gao Y, Zhang KP, Liu X, Li DW, Chang JH, Wang DW. 2013. Efficient and fine mapping of RMES1 conferring resistance to sorghum aphid Melanaphis sacchari. Mol Breed. 31:777–784.
- Wari D, Aboshi T, Shinya T, Galis I. 2022. Integrated view of plant metabolic defense with particular focus on chewing herbivores. J Integr Plant Biol. 64:449–475.
- Wortmann CS, Mamo M, Mburu C, Letayo E, Abebe G, Kayuki KC, Chisi M, Mativavarira M, Xerinda S, Ndacyayisenga T. 2009. Atlas of Sorghum (Sorghum bicolor (L.) Moench): production in eastern and Southern Africa. In: The board of regents of the University of Nebraska-Lincoln. INTSORMIL Scientific Publications. 2.
- Wu JQ, Baldwin IT. 2010. New insights into plant responses to the attack from insect herbivores. Annu Rev Genet. 44:1–24.
- Wu LH, Hill MP, Thomson LJ, Hoffmann AA. 2018. Assessing the current and future biological control potential of Trichogramma ostriniae on its hosts Ostrinia furnacalis and Ostrinia nubilalis. Pest Manage Sci. 74:1513–1523.
- Xin ZG, Wang ML, Cuevas HE, Chen JP, Harrison M, Pugh NA, Morris G. 2021. Sorghum genetic, genomic, and breeding resources. Planta. 254:114.
- Yamaguchi M, Fujimoto H, Hirano K, Araki-Nakamura S, Ohmae-Shinohara K, Fujii A, Tsunashima M, Song XJ, Ito Y, Nagae R, et al. 2016. Sorghum Dw1, an agronomically important gene for lodging resistance, encodes a novel protein involved in cell proliferation. Sci Rep. 6:28366.
- Zheng LY, Guo XS, He B, Sun LJ, Peng Y, Dong SS, Liu TF, Jiang SY, Ramachandran S, Liu CM, Jing HC. 2011. Genome-wide patterns of genetic variation in sweet and grain sorghum (Sorghum bicolor). Genome Biol. 12:R114.
- Zheng YQ, Zhang XY, Liu X, Qin NN, Xu KF, Zeng RS, Liu J, Song YY. 2021. Nitrogen supply alters rice defense against the striped stem borer Chilo suppressalis. Front Plant Sci. 12:691292.
- Zhu-Salzman K, Salzman RA, Ahn JE, Koiwa H. 2004. Transcriptional regulation of sorghum defense determinants against a phloem-feeding aphid. Plant Physiol. 134:420–431.