ABSTRACT
A library of natural sesquiterpene and phenolic compounds from Petasites japonicus are being investigated through different computational techniques to study their ability to target BMP. Lipinski rule, ADMET, molecular docking studies and metabolism were used to reach promising candidates with proposed activity against BMP. Four sesquiterpenes (kablicin, petisinol, bakkenolide D and bakkenolide IIIa) and four phenolic compounds exhibited drug-like properties (caffeic acid, petasiphenol, petasitesin A and petasitesin B), so they deserve further clinical exploration as bone loss modulators. The phenolic compounds specially fukinolic acid and petasiphenol showed lower binding energy with both BMPRIA and BMPRII than Icariin agonist and sesquiterpenes. Bakkenolide IIIa showed dual potential on both BMPRIA and BMPRII with binding energies equal – 7.82 and – 9.9 Kcal/mol respectively, which is more better score than Betulinic acid agonist. This research is focusing on plant-human interactions and exploring the ability of plant constituents to modulate a human protein such as BMP.
1. Introduction
Bone fractures are a major public health concern and a significant financial burden, particularly for those who have osteoporosis. Fractures are a significant burden on individuals, communities and healthcare systems because they can result in loss of productivity, incapacity, diminished quality of life, health decline and high health-care expenditures (Wu et al. Citation2021). Osteoporosis is one of the most prevalent metabolic skeletal illnesses; it is characterized by reduced bone strength, limited osteogenic activity, and increased osteoclast resorption, which increases the risk of bone fractures (Ferrari et al. Citation2018). Fractures caused by osteoporosis result in a considerable decrease in quality of life, as well as an increase in morbidity, mortality, and disability (Varacallo and Fox Citation2014). Numerous variables contribute to osteoporosis and fractures. Major predisposing factors are food and physical activity. Fracture history in the family and personal fracture history are both risk factors for osteoporosis. More than 8.9 million fractures occur annually due to osteoporosis, resulting in a fracture every three seconds (Johnell and Kanis Citation2006). Consequently, there is an urgent need to develop novel treatments for this disease. Osteoblasts facilitate the process of bone formation by secreting bone matrix and boosting calcium deposition. Nonetheless, osteoclasts mediate the remodeling process by resorbing bone matrix (Guo et al. Citation2021). Bone morphogenetic proteins (BMPs) are traditional growth factors that play crucial roles in the regulation of proper bone formation and bone resorption (Baek et al. Citation2021). BMPs regulate anabolic and catabolic mechanisms by controlling the development and activity of osteoblasts (OBs) and osteoclasts (OCs), the principal cell types responsible for bone production and resorption, respectively. BMPs are released from the hydrolyzed bone and transported by OC-like cells to stimulate osteogenesis, which is triggered by the recruitment of mesenchymal OB progenitors. Next, differentiating chondrocytes and activated OBs produce the organic bone matrix, which is then matured by the addition of inorganic salts. In mature calcified bone, OBs undergo apoptosis or develop into quiescent osteocytes (Segredo-Morales et al. Citation2018; Wang et al. Citation2021). More than fifteen known BMPs are structurally related and can be further classified based on amino acid or nucleotide similarity. Due to their pervasive expression and role as body-wide regulators, a loss in BMP synthesis or function typically results in pronounced abnormalities or severe diseases (Zhang et al. Citation2021). Usually, alterations in TGF-b/BMP activity are associated with a vast array of clinical consequences, including skeletal and extra-skeletal abnormalities, autoimmune disorders, cancer, and cardiovascular diseases (Tang et al. Citation2021) Some BMPs can act as bone formation inhibitors. BMP3, for instance, is a negative regulator of bone density, whereas BMP13 is a potent inhibitor of bone development. Based on their powerful bone-inducing activities, BMP2, 4, 6, 7, and 9 are usually referred to as osteogenic BMPs (Lademann et al. Citation2020). BMP-2 is one of the most osteogenic BMPs capable of considerably promoting bone formation. Although BMP2 is unnecessary for bone production, it is necessary for the onset of fracture healing and induced both activity of osteoblast and osteoclast (Lowery et al. Citation2011). BMP-2 deficiency can slow the transition of osteoprogenitor cells into osteoblasts (Wang et al. Citation2014). The Food and Drug Administration (FDA) has approved BMP2 for the treatment of long bone fractures and spinal fusion operations and recombinant human BMP2 (rhBMP-2) for human usage (James et al. Citation2016; Chao et al. Citation2021). BMP-2 can strongly promote bone growth in osteoblasts via the SMAD signaling pathway (Fitzpatrick et al. Citation2017). BMP2 can bind to the type I receptor (BMPRIa) and type II receptor, which are involved for beginning the canonical and non-canonical signaling cascades, respectively (BMPRII) (). Signaling caused by BMP2 begins when the protein either recruits the dimerization of two receptors or binds to receptors that are already dimerized (Okamoto et al. Citation2006; Bragdon et al. Citation2011; Durbano et al. Citation2020).The type II receptor will phosphorylate the type I receptor's glycine-serine-rich (GS) box homeodomain once all three components have formed a complex (Mace et al. Citation2006). Consequently, SMAD-independent and – dependent signaling may be stimulated (Yang et al. Citation2014; Wang et al. Citation2020).
Petasites japonicas (butterbur), is known as giant butterbur () and sweet-coltsfoot, the plant family is Asteraceae, it has medicinal significance as it has been used in treatment and prophylaxis of migraine, headache and tension in China, Japan and Korea, It has antiallergic effects, anti-asthma, anti-cancer, antioxidant activity, neuroprotective, Improvement of metabolism and anti-inflammatory properties (Lee et al. Citation2019; Hiemoriikondo Citation2020; Borlak et al. Citation2022).
Figure 2. Petasites japonicus retrieved from (https://www.gardenfundamentals.com/petasites-japonicus/ last access on 26 Jan 2023).
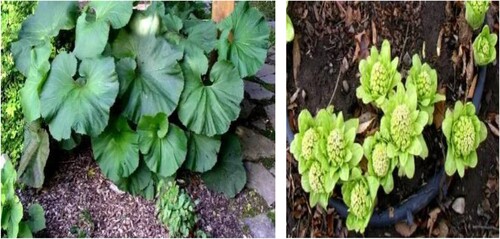
Moreover, Petasites japonicus, a perennial member of the Compositae family that grows wild across much of Japan from Hokkaido to Kyushu, is known as the Japanese butterbur (Iwamoto Citation2009). Butterbur (Petasites japonicus) consists of herbaceous perennial plants with huge, hat-shaped leaves and thick, creeping underground rhizomes ().
Sesquiterpene analogs have played a good role in bone biology as they had proved to play an important role towards the development of new and effective approaches to prevent and treat osteolytic bone diseases (Qin et al. Citation2015). Additionally, it has been reported that some sesquiterpenes can inhibit osteoclastogenesis (Qin et al. Citation2015). As instance, sesquiterpen lactone compound I has been shown to inhibit bone morphogenic protein (BMP) with an IC50 value of 51 μM (Ohte et al. Citation2021). Natural Sesquiterpenes II–IV were also reported to Inhibit bone resorption and osteoclast formation (Qin et al. Citation2015). The natural phenolic compounds oleuropein and resveratrol () have important roles in bone formation. Puel et al. found that oleuropein reduced bone loss (Puel et al. Citation2006). Resveratrol has shown ability to inhibit the MAP kinase pathways in osteoblasts. Furthermore, resveratrol inhibited the expression of bone morphogenic protein-4 (BMP-4) (Nicolin et al. Citation2019).
Also, Petasites japonicus (Siebold & Zucc.) is one of Petasites species which is known with its content of sesquiterpenes and phenolic acids constituents (Kulinowski et al. Citation2022), so we decided in this research work to investigate the possible interactions of P. Japonicous sesquiterpenes and polyphenols with bone morphogenetic protein.
These findings encouraged us to investigate the natural sesquiterpenes and phenolic constituents from Butterbur as an alternative strategy to enhance BMP signaling by using these naturally extracted products.
Icariin is a flavonoid glucoside, and various studies have demonstrated that treatment with Icariin increases osteogenic differentiation and decreases bone loss in vivo and in vitro (). Icariin augments BMP2-mediated osteoblastic development of myoblast cells (Chen et al. Citation2019). Betulinic acid is a pentacyclic triterpenoid that occurs naturally in a variety of fruits and vegetables. Betulinic acid can boost the osteogenic potentials of BMP2 () in vivo, possibly through activating the Smad 1/5/8 and p38 pathways, and the combination of both drugs can be regarded as a treatment for bone disorders (Choi et al. Citation2016).
In this research work, we will try to find new modulating agents for BMP-2 that may be useful in repairing bones. We decided to investigate some sesquiterpenes and phenolic compounds (Bradford et al. Citation2019) for their potentials to act as BMP-2 up-regulators. The selected library ( and ) was subjected to different computational techniques as Lipinski rule, ADMET, toxicity and docking studies to get new promising candidates with expected activity against BMP-2.
Figure 4. The chemical structures of the sesquiterpene compounds from P. Japonicous.
2. Results and discussion
2.1. Drug-likeness properties and admet analysis
In this work, a library of 37 sesquiterpene compounds and 12 phenolic constituents of Butterbur (Petasites japonicus) compounds ( and ) were subjected to various computational techniques to investigate the effect of chemical structure changes on the ability of these two scaffolds to target BMP-2 receptors, leading to promising candidates with proposed agonist activity on BMP-2 receptors.
2.1.1 Lipinski’s and veber’s rules
As per the guidelines of Veber's and Lipinski's rule of five, a computer analysis was performed in this study to ascertain the number of rotatable bonds, topological polar surface area (TPSA), and other physicochemical features of the examined candidates.
2.1.1.1. Sesquiterpene
Table 1S (Supp Data) showed no violation with either Lipinski's or Veber's rules, indicating that they all have a potential for pharmaceutical use. Hydrogen bond acceptors (HBA) in the sesquiterpene compounds ranged from 0 to 6, which is below the threshold of 10 to meet the first parameter of Lipinski's rule. Furthermore, concerning the number of hydrogen bond donors (HBD), all investigated sesquiterpene compounds have HBD ranging from 0–2 (i.e. less than 5) which meets the second parameter of Lipinski’s rule. The third parameter of Lipinski's rule is supported by the fact that all of these molecules have a molecular weight of less than 500. The logP values of these compounds were also quite varied, going from 1.70–4.63. The results are consistent with Lipinski's rule's fourth parameter, which states that logP values below 5 indicates promising formulated candidates. The molecular flexibility and oral bioavailability of the drug candidates were evaluated by determining the number of rotatable bonds using Veber's principles. The study showed that sesquiterpene molecules had between 1 and 7 rotatable bonds, which is within the range required by Veber's rules for such compounds. Furthermore, the number of TPSA a physicochemical characteristic defining the polarity of such molecules is less than 140 Ų, which is within the allowed values (range from 0 to 104.2 Ų) (Table 2S, Supp Data).
2.1.1.2. Phenolic compounds
There are four molecules with drug-like molecular structures (). Caffeic acid, Petasiphenol, Petasitesin A, and Petasitesin B all had sufficient numbers of rotatable bonds, HBA, HBD, logP, and TPSA values (, 3S and 4S, Supp Data). As demonstrated in Tables 3S and 4S, the other phenolic compounds showed some violations of Lipinski's rule or Veber's rule because either their molecular weight exceeds 500 or the number of HBA and HBD exceeds appropriate levels (Supp. Data).
Table 1. Physicochemical properties of compounds that follow Lipinskís and veber rules.
2.1.2 Pharmacokinetic properties parameters
Blood-brain barrier (BBB) penetrability, intestinal absorption, and plasma protein binding (PPB) characteristics were determined for all compounds. In details represented in (, 1S, and 3S), 32 sesquiterpene compounds revealed ability to permeate BBB. Eleven Phenolic compounds, on the other hand, did not traverse BBB and were therefore predicted to be safe for the CNS. In general, all sesquiterpene compounds except eremophilene showed high intestinal absorption, and caffeic acid, petasiphenol, petasitesinA, and petasitesin B exhibited high intestinal absorption.
Table 2. Pharmacokinetic parameters using Swissadme website.
Furthermore, absorption percent (%ABS) was predicted using the equation %ABS = 109 − (0.345 × TPSA) (Zhao et al. Citation2002), 37 sesquiterpenes and 12 phenolic compounds had a range between 36.10% to 109%, indicating that the necessary cell membrane permeability of sesquiterpene compounds (73.05–109%) and caffeic acid (82.17%) was greater than that of other phenolic compounds (36.10–72%) (Table 2S and 4S, Supp. Data). Bioavailability, which is an indicator of the amount of drug content in plasma, is the most significant factor influencing absorption. Interestingly, the bioavailability of all sesquiterpene compounds and just four phenolic molecules was determined to be high.
Due to the fact that none of the sesquiterpenes compounds (with the exception of furanofukinol) are Pgp protein substrates, they have a low risk of effluxing out of the cell, resulting in a maximal effect. Five phenolic compounds out of twelve are not Pgp protein substrates. These include chlorogenic acid and caffeic acid, fukinolic acid, fukiic acid, and petasiphenol.
On the basis of the results obtained from previous physicochemical studies, the Pharmacokinetic properties of compounds that obey Lipinski's and do not pass the BBB, have high bioavailability scores and high gastrointestinal absorption were evaluated. Four sesquiterpenes compounds (kablicin, petisinol, bakkenolide D, and bakkenolide IIIa) and four phenolic compounds exhibit drug-like properties (caffeic acid, petasiphenol, petasitesin A, and petasitesin B). Using the Molsoft programme to predict the solubility value of the compounds, it was determined that the derivatives met the solubility criteria with values ranging from 19.91–6782.68 mg/l, which is greater than 0.0001 mg/l. The PreADME website was also utilized to assess oral drug delivery, central nervous system disposition, and plasma-protein binding (PPB) model efficacy (Thangavelu et al. Citation2017). Human colon adenocarcinoma-derived Caco2 cells were examined for their permeability. In the Caco2 cell model, the medium cell permeability scores for the tested compounds ranged from 18.07–35.51 nm/s, as shown in . The most permeable molecule to MDCK (Mandin Darby Canine Kidney) cells in vitro was petasitesin A, while the least permeable compound was bakkenolide IIIa. Moreover, intestinal absorption (HIA) values for humans ranged from 67.60% to 99.22%, indicating that substances are highly absorbed. According to the PPB abilities investigation, Kablicin, Bakkenolide D, Bakkenolide IIIa, and petasitesin A revealed strong protein binding (above 90%), but petasinol caffeic acid, petasiphenol, and Petasitesin B showed low protein binding (less than 90%). The DataWarrior programme was used to investigate the mutagenicity and tumorigenicity profiles of eight compounds, none of which displayed mutagenicity or carcinogenicity with the exception of caffeic acid, which exhibited strong tumorigenicity ().
Table 3. Pharmacokinetic properties, mutagenicity, tumorigenicity studies, and solubility value using Pre-ADME, DataWarrior and molsoft wbsites.
2.2 Docking studies
Docking studies serve to validate the model's quality and comprehend the binding manner of drugs to their biological targets in terms of affinity. Consequently, docking research was conducted to elucidate the potential binding manner of sesquiterpene and phenolic compounds with distinct activity profiles (Belal et al. Citation2022). Human BMP-2, in its mature form, is an acidic glycoprotein of about 32 kDa. The BMP2 ligand, a homodimer of 114 amino acid residues, has been found to include two receptor-binding motifs, rendering it as a multifunctional growth factor. A high-affinity binding site for BMPRIA is found in the wrist epitope, which contains residues from both BMP2 subunits, whereas a binding site for BMPRII is found in the knuckle epitope but is weaker (Rahman et al. Citation2015). In BMP-2, three intra-chain disulfide bonds are produced by six conserved cysteine residues. There are four glycosylation sites in the human BMP-2 proprotein. The mature form of BMP-2 contains a single glycosylation site in its polypeptide chain. Upregulation of the BMP-2 gene and the activation of multiple downstream signaling pathways result from BMP-2 binding to BMP type I and type II serine/threonine kinase receptors () (Miyazono et al. Citation2010). After binding to the BMPRs, BMP-2 triggers the development of adipocytes, chondrocytes, and osteoblasts via BMPRIA phosphorylation by BMPRII and apoptosis/cell death via BMPRIB phosphorylation (Ebara and Nakayama Citation2002). All compounds docked in the active sites of both BMPRIA and BMPRII. showed docking scores of all compounds, Icariin and Betulinic acid. Figures 6–17 and , and displayed the binding mode of the highest docking scores of sesquiterpene and phenolic compounds.
Figure 6. Binding of BMP-2 to BMPRII and BMPRIA (Miyazono et al. Citation2010).
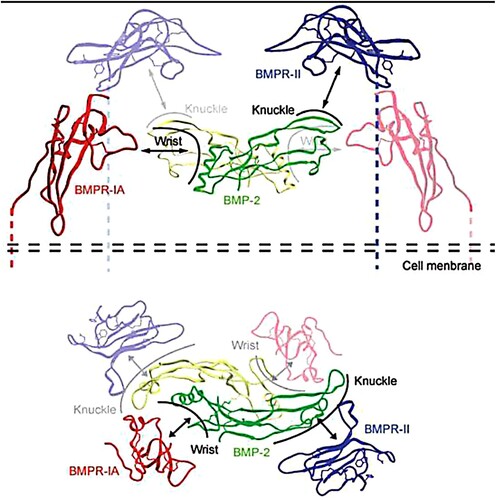
Table 4. The binding energy values (kcal/mol) for all compounds.
Table 5. Binding mode of Icariin, Betulinic acid, 1,5-di-O-caffeoylquinic acid, 3,4,5-tricaffeoylquinic acid, Fukinolic acid and Petasiphenol in the active site of BMPIA receptor.
Table 6. Binding mode and Ki of icariin, betulinic acid, neopetasin, furanopetasin, fukinolic acid, petasiphenol, petasitesin B in active site of BMPII receptor.
2.2.1. BMPRIA receptor docking simulation
During embryonic development, the Bmp receptor activin-like kinase 3 (Alk3; or BMPRIA) is expressed in a wide variety of tissues. However, in the postnatal period, it is mostly expressed in osteo-lineage cells and bone marrow cells. Throughout osteogenesis and chondrogenesis, ALK3 has been repeatedly demonstrated to be a critical receptor for regulating BMP signaling (Mueller and Nickel Citation2012). ALK3 is one of the key factors for regulating the specification of pre-chondrogenic mesenchyme as well as chondro-lineage differentiation and maturation, postnatal chondrogenesis and the maintenance of articular cartilage (Lin et al. Citation2016). Two β-sheets and an α-helix compensate the extracellular domain of BMPRIA. Additional residues from monomer B and helix 1 make up the rest of the wrist epitope. The interaction between Phe 85 amino acid and BMP-2 ligand is a hallmark of type I receptors. Pro45 and His43 found in loop 1 of BMPRIA whose backbone flexibility is restricted among type I receptors (Lin et al. Citation2016). Phe 60, Met 78, and Ile 99 are highly conserved and functionally important residues in BMPRIA receptor. Asn 59, along with Ile 62, Val 63, and Leu 66 of BMP-2 ligand, provides an almost entirely hydrophobic surface that makes contact with receptor residues Glu 81, Phe 85, Gln 86, and Asp 89 (Keller et al. Citation2004; Mace et al. Citation2006). The docking results for BMPRIA revealed that phenolic compounds are superior to sesquiterpene by more than – 8 kcal/mol, while the energy of binding of sesquiterpene is greater than – 7.5 kcal/mol. Consequently, then we can conclude that both are promising regulators, however, sesquiterpene has a lower affinity for the BMPRIA receptor when compared with phenolic compounds. The best phenolic compounds were 3,4,5-tricaffeoylquinic acid, Fukinolic acid, and Petasiphenol because their docking scores were greater than Icariin's (BMP-2 agonist), see . The binding energy was used to calculate the binding affinity constant (Ki value) through the equation (ki = 10 [Binding Energy/ 1.366]) (Farghaly et al. Citation2021) and the most promising target compounds were chosen based on their binding energy and interaction within the binding site, .
The hypothesized binding mechanism of Icariin and Betulinic acid with the BMPIA receptor was depicted in and . Furthermore, the binding energy of icariin was lower than that of Betulinic acid, – 10.62 versus – 7.49 kcal/mole, indicating a greater affinity of Icariin for the BMPIA receptor than Betulinic acid. Icariin is anchored by multiple forms of hydrogen bonds with Asp89, Lys92, Ser90, His43, Phe85, and Gln86. Icariin also forms four hydrophobic bonds with Arg97 and Asp89 (). Betulinic acid has one hydrogen bond and four hydrophobic interactions ().
Bakkenolide IIIA, which has a favorable ADME profile and the highest (sesquiterpene compound) docking score of – 7.82 kcal/mol at the BMPIA receptor, is held in place by hydrogen bonding connections with Ser90 and four hydrophobic contacts with the essential amino acids Phe85, Lys88, and Pro91 ().
Figure 9. 2D and 3D representation of predicted binding mode of Bakkenolide IIIA with BMPIA receptor.
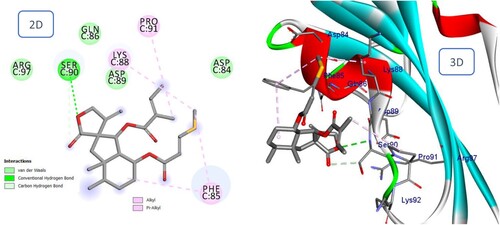
In the postulated binding mode of the phenolic compound 3,4,5-tricaffeoylquinic acid, two catechol moieties are anchored by hydrogen bonding interactions with Gln86, Asp89, and Asp84, as well as the formation of carbon hydrogen bonds and Pi-doner-hydrogen interactions with Asp89, and Phe85 ().
Figure 10. 2D and 3D representation of predicted binding mode of 3,4,5-tricaffeoylquinic acid with BMPIA receptor.
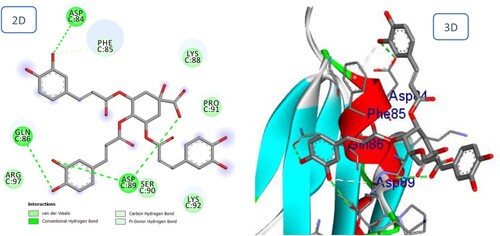
The final results for Fukinolic acid and Petsiphenol with the BMPIA receptor are consistent with the general pattern observed for Icariin with high docking scores (−14.07, 13.90, and – 10.62 kcal/mol respectively). The hydrogen bonding interactions continue to exist as before. shows that the di hydroxy phenyl moieties of Fukinolic acid interacted favorably with Gln86, Asp89, Pro91, Phe85, and Lys88. Also, in Petasiphenol, the two catechol rings may be important for the compound's accommodation at the binding site ().
2.2.2. BMPRII receptor docking simulation:
BMPRII is a serine threonine kinase receptor of type II TGF-b that is essential to the BMP signaling pathway. Crystals of the BMPRII ectodomain were produced in two distinct forms, and both forms diffracted with a high degree of resolution (Miyazono et al. Citation2010). The tetragonal form exhibited some disorder, whereas the orthorhombic form included the full polypeptide. They exhibit various conformations of the A-loop at the periphery of the hypothesized ligand-binding interface, as well as a disulfide bridge rearrangement within the loop. This specific disulfide (Cys94 – Cys117) is exclusive to BMPRII and activin receptors, suggesting that it is essential for their shared manner of binding. Cys99 and Cys116 are connected by an extra disulfide bridge in BMPRII. His87 has a crucial part in ligand recognition (Guo et al. Citation2022). Based on the docking scores and interactions with the binding site, the most promising natural compounds were selected for binding affinity predictions. Equation (ki = 10 [Binding Energy/ 1.366]) was used to get the binding affinity (Ki value) using the binding energy scores (Farghaly et al. Citation2021).
Icariin binds to the BMPRII receptor via multiple interactions, particularly those with Ser119, Cys94, Pro91, and His87 that are of critical importance (). Betulinic acid interacts via three hydrogen bonds and four alkyl interactions, similar to Icariin (), but with a higher binding energy. The docking scores (S) for Icariin and Betulinic acid were – 11.60 and – 7.50 kcal/mol, respectively.
Figure 14. 2D and 3D representation of predicted binding mode of Betulinic acid with BMPII receptor.
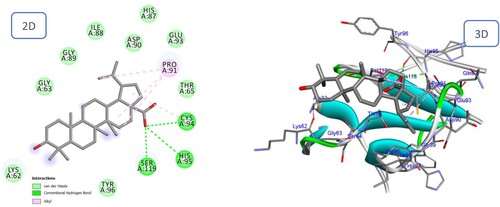
As shown in and , the sesquiterpene compounds Furanopetasin and Bakkenolide IIIa formed multiple interactions with the binding site of the BMPII receptor. Furanopetasin's octahydronaphtho[2,3-b]furan formed five interactions with the essential residue Cys94, as well as interactions with Gly89, Ile88 and Ser119. In addition, each carbonyl group served as an acceptor for a hydrogen bond with the Asp90 amino acid. Finally, a Pi-Sigma bond was formed between the methyl group of the side chain and the crucial residue His87. The docking score for Bakkenolide IIIa was predicted to be favourable (−9.90 kcal/mole). Bakkenolide IIIa's furan-3,2'-inden-2-one formed a hydrogen bond with Thr65, a sulfur bond with Cys94, and an alkyl bond with Pro91, whereas one of the carbonyl groups formed a hydrogen bond with Ser119.
Figure 16. 2D and 3D representation of predicted binding mode of Bakkenolide IIIa with BMPII receptor.
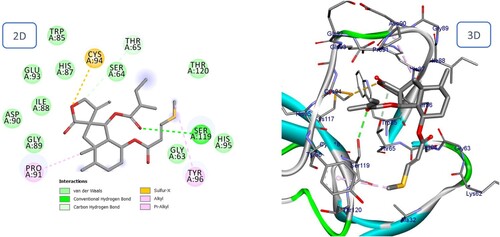
The majority of phenolic compounds demonstrated superior docking scores compared to sesquiterpene compounds. The two highest compounds were Fukinolic acid and Petasiphenol, whose catechol groups significantly contributed to the binding interactions at the active site by forming a hydrogen bond with the His87 residue. In addition, Fukinolic acid formed five hydrogen bonds with the residues Thr65, Asp90, Pro91, and Ser119 (). Likewise, it binds via Pi-cation to His 87 and Pi-Anion to Glu93. Four additional hydrogen bonds were observed between the hydroxyl of Petasiphenol and Gln92, Cys94, and His95, in regards to the Pi-Alkyl interaction with the essential amino acid Cys94 ().
2.3. Metabolic study
Bakkenolide IIIa and Petasiphenol were selected for metabolic investigations due to their satisfactory ADME profiles and high docking scores. Using Biotransformer, the metabolic pathways and predicted metabolites of both compounds were estimated by (http://biotransformer.ca) (Djoumbou-Feunang et al. Citation2019). The chosen substances were uploaded to the server. Then, metabolic changes during phases I and II were selected. The prediction of phase I metabolites for both compounds did not show any results. The resultant data was arranged into metabolic processes that described the expected metabolites and the enzymes of phase II transformation that may perform this role in humans. The outcomes are depicted in and . In the metabolic investigations of substances Bakkenolide IIIa and Petsiphenol, respectively, three likely metabolic pathways and eight predicted metabolites were identified. depicts the metabolic steps involving thioether S-methylation and GSH-conjugation of alpha, beta-unsaturated carbonyl in Bakkenolide IIIa. GSH-conjugation of alpha, beta-unsaturated carbonyl by Glutathione transferase accounted for the majority of the anticipated phase II metabolites of Bakkenolide IIIa. In contrast, Petasiphenol's metabolic pathways included aromatic OH-glucuronidation by UDP-glucuronosyltransferase and catechol O-methylation by catechol O-methyltransferase ().
3. Conclusion
In this research work which represent a journey through natural phyto-constituents to investigate their ability to act as modulators for bone morphogenic protein, several computational tools were used to assess the pharmacokinetic profile of the selected library. Molecular docking studies reveled more promising natural compounds than the reported BMP agonists, which indicate their high promiscuity to act against bone damage, Bakkenolide IIIa showed dual potential on both BMPRIA and BMPRII with docking scores – 7.82 and – 9.9 Kcal/mol respectively, this is more better score than Betulinic acid agonist. The phenolic compounds Fukinolic acid and Petasiphenol showed more better docking scores with both BMPRIA and BMPRII than Icariin agonist. All these findings support the need for further preclinical investigations for these hopefully bone regulating candidates to treat bone loss and damage.
4. Experimental
4.1. Computational admet studies
Physicochemical characteristics and ADME were evaluated using the SwissADME web server (http://www.swissadme.ch/index.php), the Molsoft server (https://www.molsoft.com), and the PreADME server (https://preadmet.bmdrc.kr), as well as the DataWarrior programme.
4.2. Docking studies
Preparation of proteins: Crystallographic structure of BMPRIA [PDB ID: 1rew] (Keller et al. Citation2004) and BMPRII [PDB ID: 2hlr] (Miyazono et al. Citation2010) were obtained from the Protein Data Bank (http://www.pdb.org) and regarded as docking simulation targets. The docking study was performed utilizing MOE2019 software (Chemical Computing Group Inc., Montreal, Quebec, Canada) to assess the free energy and interaction modes of molecules with respect to BMP-2 receptors. The crystal structure was initially prepared by eliminating water molecules while preserving the crucial chain. The protein structure was then protonated, the missing hydrogens were inserted to provide the desired ionization states, and the hydrogen atoms were concealed. Then, the amount of energy was reduced. With the default settings, the MOE Alpha Site Finder was used to identify the active site. The obtained alpha spheres were used to construct the inactive site's dummy atoms.
Preparation of ligands: Using ChemBioDraw Ultra 16.0, the 2D structures of the investigated drugs were sketched and saved in MDL-SD format. After opening the saved files with MOE, 3D structures were protonated. Next, energy reduction was implemented.
Molecular docking calculations: Molecular docking of the tested drugs against the target receptor was done using the default technique. Using genetic algorithm searches, docked structures were created for each instance. The forcefield's refinement code was MMFF94X.
Selection of best pose: The poses of the compounds with the highest ratings are chosen. Visualizations: We utilized the Biovia discovery-studio 2020 visualizer to examine the representation of protein-ligand interactions in the active site of the complex. In conclusion, the H-bonds, Pi – interactions, and hydrophobic are regarded as the most significant interactions discovered.
4.3. Metabolic study
(http://biotransformer.ca) estimated the metabolic pathways and predicted metabolites of both compounds using Biotransformer. Firstly, the smile of compounds Bakkenolide IIIa and Petasiphenol were obtained from chemdraw program and were used in http://biotransformer.ca to predicate phase I and II metabolism.
Acknowledgments
The authors are thankful to the Researchers Supporting Project number (RSP2023R516) at King Saud University, Riyadh, Saudi Arabia. Authors are thankful to Princess Nourah bint Abdulrahman University Researchers Supporting Project number (PNURSP2023R171), Princess Nourah bint Abdulrahman University, Riyadh, Saudi Arabia.)
Disclosure statement
No potential conflict of interest was reported by the author(s).
Additional information
Funding
Notes on contributors
Amany Belal
Amany Belal a full professor in the field of Medicinal chemistry at Beni-Suef University, Egypy, Interested in drug discovery and natural products.
Mona H. Ibrahim
Mona H. Ibrahim Assistant professor at the Department of Pharmaceutical Medicinal Chemistry and Drug Design, Faculty of Pharmacy (Girls), Al-Azhar University. Google scholar account: https://scholar.google.com/citations?user = ZigNJ34AAAAJ&hl = en
Wael A. Mahdi
Wael A. Mahdi Associate professor in Department of Pharmaceutics, College of Pharmacy, King Saud University, Web site: https://faculty.ksu.edu.sa/ar/wmahdi
Sultan Alshehri
Sultan Alshehri Associate professor in Department of Pharmaceutics, College of Pharmacy, King Saud University, Web site: https://faculty.ksu.edu.sa/en/salshehri1/course/211655
Hasnaa Ali Ebrahim
Hasnaa Ali Ebrahim Assistant professor in Department of Basic Medical Sciences, College of Medicine, Princess Nourah bint Abdulrahman University. Web site: https://faculty.pnu.edu.sa/haebrahim/Pages/default.aspx
Mohammed M. Ghoneim
Mohammed M. Ghoneim Associate professor in Department of Pharmacy Practice, College of Pharmacy, AlMaarefa University, Web site: https://www.um.edu.sa/en/node/120
Mohamed El-Sherbiny
Mohamed El-Sherbiny Associate professor in Department of Basic Medical Sciences, College of Medicine, AlMaarefa University, web site: https://www.um.edu.sa/en/node/39
Ahmed B. M. Mehany
Ahmed B. M. Mehany Associate professor in Zoology Department, Faculty of Science, Al-Azhar University, researchgate account: https://www.researchgate.net/profile/Ahmed-Mehany
Heba I. Ghamry
Heba I. Ghamry Assistant professor of nutrition in Department of Home Economics, College of Home Economics, King Khalid University, google scholar: https://scholar.google.com/citations?user = CEoxahoAAAAJ&hl = ar
References
- Baek HJ, hyung Kim I, Yun PY, Kim YK. 2021. Prognosis of single tooth implants following alveolar ridge preservation with two recombinant human bone morphogenetic protein-2 delivery systems. BMC Oral Health. 21.
- Belal A, Elkady H, Al-Karmalawy AA, Amin AH, Ghoneim MM, El-Sherbiny M, Al-Serwi RH, Abdou MA, Ibrahim MH, Mehany ABM. 2022. Discovery of some heterocyclic molecules as bone morphogenetic protein 2 (BMP-2)-inducible kinase inhibitors: virtual screening, ADME properties, and molecular docking simulations. Molecules. 27.
- Borlak J, Diener HC, Kleeberg-Hartmann J, Messlinger K, Silberstein S. 2022. Petasites for migraine prevention: New data on mode of action, pharmacology and safety. A narrative review. Front Neurol. 13.
- Bradford STJ, Ranghini EJ, Grimley E, Lee PH, Dressler GR. 2019. High-throughput screens for agonists of bone morphogenetic protein (BMP) signaling identify potent benzoxazole compounds. J Biol Chem. 294.
- Bragdon B, Moseychuk O, Saldanha S, King D, Julian J, Nohe A. 2011. Bone morphogenetic proteins: A critical review. Cell Signal. 23.
- Chao YL, Wang TM, Chang HH, Lin LD. 2021. Effects of low-dose rhBMP-2 on peri-implant ridge augmentation in a canine model. J Clin Periodontol. 48.
- Chen M, Cui Y, Li H, Luan J, Zhou X, Han J. 2019. Icariin promotes the osteogenic action of BMP2 by activating the cAMP signaling pathway. Molecules. 24.
- Choi H, Jeong BC, Kook MS, Koh JT. 2016. Betulinic acid synergically enhances BMP2-induced bone formation via stimulating Smad 1/5/8 and p38 pathways. J Biomed Sci. 23.
- Djoumbou-Feunang Y, Fiamoncini J, Gil-de-la-Fuente A, Greiner R, Manach C, Wishart DS. 2019. Biotransformer: A comprehensive computational tool for small molecule metabolism prediction and metabolite identification. J Cheminform. 11.
- Durbano HW, Halloran D, Nguyen J, Stone V, McTague S, Eskander M, Nohe A. 2020. Aberrant BMP2 signaling in patients diagnosed with osteoporosis. Int J Mol Sci. 21.
- Ebara S, Nakayama K. 2002. Mechanism for the action of bone morphogenetic proteins and regulation of their activity. Spine (Phila PA 1976). 27.
- Farghaly TA, Althagafi I, Ibrahim MH, Al-Qurashi NT, Farooq U. 2021. Synthesis under microwaves irradiation, structure elucidation, docking study for inhibiting COVID-19 and DFT calculations of novel azoles incorporated indole moiety. J Mol Struct. 1244.
- Ferrari SL, Abrahamsen B, Napoli N, Akesson K, Chandran M, Eastell R, El-Hajj Fuleihan G, Josse R, Kendler DL, Kraenzlin M, et al. 2018. Diagnosis and management of bone fragility in diabetes: an emerging challenge. Osteop Int. 29.
- Fitzpatrick V, Fourel L, Destaing O, Gilde F, Albigès-Rizo C, Picart C, Boudou T. 2017. Signal mingle: micropatterns of BMP-2 and fibronectin on soft biopolymeric films regulate myoblast shape and SMAD signaling. Sci Rep. 7.
- Guo J, Liu B, Thorikay M, Yu M, Li X, Tong Z, Salmon RM, Read RJ, ten Dijke P, Morrell NW, Li W. 2022. Crystal structures of BMPRII extracellular domain in binary and ternary receptor complexes with BMP10. Nat Commun. 13.
- Guo YF, Su T, Yang M, jun Li C, Guo Q, Xiao Y, Huang Y, Liu Y, Luo XH. 2021. The role of autophagy in bone homeostasis. J Cell Physiol. 236.
- Hiemoriikondo M. 2020. Antioxidant compounds of Petasites japonicus and their preventive effects in chronic diseases: a review. J Clin Biochem Nutr. 67:10–18.
- Iwamoto Y. 2009. Breeding of Japanese butterbur (Petasites japonicus) by using flowerhead culture. Plant Biotechnol. 26:189–196.
- James AW, LaChaud G, Shen J, Asatrian G, Nguyen V, Zhang X, Ting K, Soo C. 2016. A review of the clinical side effects of bone morphogenetic protein-2. Tissue Eng Part B Rev. 22.
- Johnell O, Kanis JA. 2006. An estimate of the worldwide prevalence and disability associated with osteoporotic fractures. Osteop Int. 17.
- Keller S, Nickel J, Zhang JL, Sebald W, Mueller TD. 2004. Molecular recognition of BMP-2 and BMP receptor IA. Nat Struct Mol Biol. 11:481–488.
- Kulinowski Ł, Luca SV, Minceva M, Skalicka-Woźniak K. 2022. A review on the ethnobotany, phytochemistry, pharmacology and toxicology of butterbur species (Petasites L.). J Ethnopharmacol. 293.
- Lademann F, Hofbauer LC, Rauner M. 2020. The bone morphogenetic protein pathway: The osteoclastic perspective. Front Cell Dev Biol. 8.
- Lee JS, Jeong M, Park S, Ryu SM, Lee J, Song Z, Guo Y, Choi JH, Lee D, Jang DS. 2019. Chemical constituents of the leaves of butterbur (Petasites japonicus) and their anti-inflammatory effects. Biomolecules. 9.
- Lin S, Svoboda KKH, Feng JQ, Jiang X. 2016. The biological function of type i receptors of bone morphogenetic protein in bone. Bone Res. 4.
- Lowery JW, Pazin D, Intini G, Kokabu S, Chappuis V, Capelo LP, Rosen V. 2011. The role of BMP2 signaling in the skeleton. Crit Rev Eukaryot Gene Expr. 21.
- Mace PD, Cutfield JF, Cutfield SM. 2006. High resolution structures of the bone morphogenetic protein type II receptor in two crystal forms: implications for ligand binding. Biochem Biophys Res Commun. 351.
- Miyazono K, Kamiya Y, Morikawa M. 2010. Bone morphogenetic protein receptors and signal transduction. J Biochem. 147:35–51.
- Mueller TD, Nickel J. 2012. Promiscuity and specificity in BMP receptor activation. FEBS Lett. 586.
- Nicolin V, de Tommasi N, Nori SL, Costantinides F, Berton F, di Lenarda R. 2019. Modulatory effects of plant polyphenols on bone remodeling: A prospective view from the bench to bedside. Front Endocrinol (Lausanne). 10.
- Ohte S, Yamazaki H, Takahashi O, Rotinsulu H, Wewengkang DS, Sumilat DA, Abdjul DB, Maarisit W, Kapojos MM, Zhang H, et al. 2021. Inhibitory effects of sesquiterpene lactones from the Indonesian marine sponge Lamellodysidea cf. herbacea on bone morphogenetic protein-induced osteoblastic differentiation. Bioorg Med Chem Lett. 35.
- Okamoto M, Murai J, Yoshikawa H, Tsumaki N. 2006. Bone morphogenetic proteins in bone stimulate osteoclasts and osteoblasts during bone development. J Bone Miner Res. 21.
- Puel C, Mathey J, Agalias A, Kati-coulibaly S, Mardon J, Obled C, Davicco MJ, Lebecque P, Horcajada MN, Skaltsounis AL, Coxam V. 2006. Dose-response study of effect of oleuropein, an olive oil polyphenol, in an ovariectomy/inflammation experimental model of bone loss in the rat. Clin Nutr. 25.
- Qin S, Ang E, Dai L, Yang X, Ye D, Chen H, Zhou L, Yang M, Teguh D, Tan R, et al. 2015. Natural germacrane sesquiterpenes inhibit osteoclast formation, bone resorption, RANKL-induced NF-κB activation, and IκBα degradation. Int J Mol Sci. 16:26599–26607.
- Rahman MS, Akhtar N, Jamil HM, Banik RS, Asaduzzaman SM. 2015. TGF-β/BMP signaling and other molecular events: regulation of osteoblastogenesis and bone formation. Bone Res. 3.
- Segredo-Morales E, García-García P, Reyes R, Pérez-Herrero E, Delgado A, Évora C. 2018. Bone regeneration in osteoporosis by delivery BMP-2 and PRGF from tetronic–alginate composite thermogel. Int J Pharm. 543.
- Tang H, Zhang X, Xue G, Xu F, Wang Q, Yang P, Hong B, Xu Y, Huang Q, Liu J, Zuo Q. 2021. The biology of bone morphogenetic protein signaling pathway in cerebrovascular system. Chin Neurosurg J. 7.
- Thangavelu R, Sai Harika M, Raj Kumar T, Siva Sankar Reddy L. 2017. Docking studies of benzimidazole derivatives using HEX 8.0 synthesis and microbial evaluation of amino acid anti metabolites view project anti cancer agents view project. Int J Pharm Sci Res. 8:1677–1688.
- Varacallo MA, Fox EJ. 2014. Osteoporosis and its complications. Med Clin North Amer. 98.
- Wang RN, Green J, Wang Z, Deng Y, Qiao M, Peabody M, Zhang Q, Ye J, Yan Z, Denduluri S, et al. 2014. Bone morphogenetic protein (BMP) signaling in development and human diseases. Genes Dis. 1.
- Wang X, Li Z, Wang Z, Liu H, Cui Y, Liu Y, Ren M, Zhan H, Li Z, Wu M, Wang J. 2021. Incorporation of bone morphogenetic protein-2 and osteoprotegerin in 3D-printed Ti6Al4 V scaffolds enhances osseointegration under osteoporotic conditions. Front Bioeng Biotechnol. 9.
- Wang Z, Bao HW, Xu YJ. 2020. Cnidium lactone prevents bone loss in an ovariectomized rat model through the estrogen-α/BMP-2/Smad signaling pathway. J Gene Med. 22.
- Wu AM, Bisignano C, James SL, Abady GG, Abedi A, Abu-Gharbieh E, Alhassan RK, Alipour V, Arabloo J, Asaad M, et al. 2021. Global, regional, and national burden of bone fractures in 204 countries and territories, 1990–2019: a systematic analysis from the Global Burden of Disease Study 2019. Lancet Healthy Longev. 2.
- Yang J, Shi P, Tu M, Wang Y, Liu M, Fan F, Du M. 2014. Bone morphogenetic proteins: relationship between molecular structure and their osteogenic activity. Food Sci Hum Wellness. 3.
- Zhang A, Chen Z, Yu X, Zhang L, Song Q. 2021. Rational derivation of osteogenic peptides from bone morphogenetic protein-2 knuckle epitope by integrating in silico analysis and In vitro assay. Int J Pept Res Ther. 27.
- Zhao YH, Abraham MH, Le J, Hersey A, Luscombe CN, Beck G, Sherborne B, Cooper I. 2002. Rate-limited steps of human oral absorption and QSAR studies. Pharm Res. 19:1446–1457.