ABSTRACT
Papain-like cysteine proteases (PLCPs) play important roles in plant-pathogen interactions. In citrus, PLCPs are targeted by effectors from Candidatus Liberibacter asiaticus (CLas), the causal agent of Huanglongbing (HLB). To study interactions between Citrus sinensis and CLas, we identified 33 candidate CsPLCP genes by HMMsearch with the Peptidase_C1 domain (PF00112), and further confirmed them using local BLAST with PLCP sequences from Arabidopsis thaliana. The 33 CsPLCPs were classified into 9 subfamilies. Analysis of the cis-acting elements in their promoters indicated that CsPLCPs are responsive to various phytohormones and stressors. Further qPCR analysis indicated that several CsPLCPs were up-regulated during CLas infection. Subcellular localization analysis indicated that five CsPLCPs were localized to the cytoplasm and nucleus, while CsXBCP2 was localized only to the cytoplasm. In summary, this study provides a sound basis for understanding the roles of CsPLCPs in the Citrus-CLas interaction.
Introduction
Cysteine proteases are important proteolytic enzymes, and are distributed widely across the plant kingdom. Papain-like cysteine proteases (PLCPs) belong to the C1A protease family, which is part of the large CA clan of cysteine peptidases (Rawlings et al. Citation2014; Rawlings et al. Citation2018). PLCPs are classified into nine subfamilies based on their structures and functions: RD21A, CEP1, XCP2, XBCP3, THI1, SAG12, RD19A, ALP, and CTB3 (Richau et al. Citation2012). The PLCP family has been identified in Arabidopsis thaliana (Cannon et al. Citation2004), soybean (Van Wyk et al. Citation2014), papaya (Liu et al. Citation2018), cotton (Zhang et al. Citation2019), rice (Niño et al. Citation2020), fig (Zhai et al. Citation2021), and grape (Kang et al. Citation2021).
PLCPs exhibit a papain-like fold composed of an α-helix domain and a β-sheet domain, which form a deep cleft to bind the substrate (Turk et al. Citation1998; Wu et al. Citation2015). An active catalytic site containing a Cys-His-Asn catalytic triad is located within the cleft (Wu et al. Citation2020). PLCPs are typically found in proteolytically harsh subcellular locations and are often encoded as preproproteins containing various targeting signal peptides, autoinhibitory prodomains, and mature protease domains (Richau et al. Citation2012). Removal of the autoinhibitory prodomain activates the enzymes (Coulombe et al. Citation1996). The majority of PLCPs weigh between 20 and 35 kDa, with a few weighing between 50 and 75 kDa. The optimal pH for PLCP catalytic activity is between 5.0 and 8.0 (Dubey et al. Citation2007).
In plants, PLCPs participate in various physiological processes (Liu et al. Citation2018). For example, autumn leaves contain abundant cysteine proteases (Bhalerao et al. Citation2003). In wheat, vacuolar-targeted cysteine proteases are involved in leaf senescence under different inducing conditions (Thoenen et al. Citation2007). PLCPs also regulate the bulk degradation of stromal proteins during leaf senescence in barley (Parrott et al. Citation2010). In soybean, 18 cysteine proteases were found to be actively transcribed in nodules during development and senescence (Van Wyk et al. Citation2014). In addition, PLCPs are associated with germination and programmed cell death (PCD). In A. thaliana, anther-specific CP51 is a critical mediator of tapetum stability and pollen exine formation (Yang et al. Citation2014), and RD21 is a negative regulator of cell death induced by mycotoxin fumonisin B1 (Ormancey et al. Citation2019). Proteases also play important roles in different types and stages of innate immunity (Balakireva and Zamyatnin Citation2018). In plants, PLCPs act as central immunity hubs and induce a wide range of defense responses (Van der Hoorn Citation2008; Misas-Villamil et al. Citation2016). PLCPs are also targeted by pathogen-secreted effectors. For example, the Phytophthora infestans effector AVRblb2 significantly enhances the susceptibility of host potato plants by targeting the PLCP C14 (Bozkurt et al. Citation2011). Moreover, effectors secreted by Pseudomonas syringae selectively target PLCPs in tomato (Shindo et al. Citation2016). In maize, leaf tissues infested by fall armyworm accumulate cysteine proteases (Castano-Duque et al. Citation2017). PLCPs were found to be activated in the leaf apoplast of the tolerant tomato variety ‘Hawaii 7996’ during Ralstonia solanacearum infection (Planas-Marquès et al. Citation2018). The clubroot effector SSPbP53 targets cruciferous PLCPs to suppress immunity (Pérez-López et al. Citation2021). Finally, both PLCPs and effectors contribute to the coevolutionary arms race between hosts and pathogens (Misas-Villamil et al. Citation2016).
Citrus (Citrus spp.) is one of the most economically-important fruits worldwide, and the emergence of Huanglongbing (HLB) has become a disaster for the citrus industry (Bové Citation2006; Bassanezi et al. Citation2009). Previous studies suggest that the Candidatus Liberibacter asiaticus (CLas) effector SDE1 promotes colonization by inhibiting the activity of PLCPs in citrus (Clark et al. Citation2018). However, to date, there have been no comprehensive studies of the PLCP genes in citrus.
To systematically evaluate the PLCP gene family in Citrus sinensis, we performed a genome-wide identification and analyzed the phylogenetic relationships, chromosomal distributions, collinearity, cis-acting elements, and expression profiles of the identified CsPLCPs. In addition, we studied the dynamic expression profiles and subcellular localizations of 6 CsPLCPs representing different PLCP subfamilies. Our results provide a comprehensive survey of the CsPLCP gene family, which will aid future functional studies of PLCPs in C. sinensis.
Materials and methods
Identification of CsPLCP gene family
The whole genome sequence of C. sinensis was acquired from the Orange Genome Annotation Project database (OGAPd) (http://citrus.hzau.edu.cn/orange/) (Xu et al. Citation2013). Candidate PLCP proteins were identified with HMMsearch using the Peptidase_C1 domain (PF00112) acquired from the Pfam database (Mistry et al. Citation2021). A local BLAST search was carried out on the C. sinensis genome using 31 PLCP sequences from A. thaliana (E-value ≤ 1e−10). Candidate CsPLCP protein sequences were uploaded to the Conserved Domain Database (CDD) using default parameters (E-value ≤ 0.01) (Marchler-Bauer et al. Citation2015). The chromosomal locations of candidate CsPLCP genes were predicted using the CPBD database (Liu et al. Citation2022). All CsPLCP genes were renamed according to their physical chromosomal location and subfamily. The isoelectric points and molecular weights of CsPLCP proteins were calculated with ExPASy (https://web.expasy.org/compute_pi/) using ‘Average’ resolution (Duvaud et al. Citation2021).
Gene structure, synteny, and conserved motif analysis of CsPLCP gene family
The exon and intron structures of CsPLCP genes were determined by extracting the corresponding gene loci from an annotated GFF3 file acquired from the CPBD database (Liu et al. Citation2022). The syntenic blocks between the genomes of C. sinensis and A. thaliana were identified using MCScanX (Wang et al. Citation2012). The conserved motifs present in the CsPLCP protein sequences were identified using MEME suite (Bailey et al. Citation2009). The identified motifs are listed in Figure S1.
Sequence alignment and phylogenetic analysis of CsPLCP gene family
The PLCP protein sequences from C. sinensis, A. thaliana, and Vitis vinifera were used for multiple sequence alignment in MAFFT (Katoh and Standley Citation2013). ModelFinder was used to select the best model for phylogenetic estimation (Kalyaanamoorthy et al. Citation2017). A maximum-likelihood (M-L) phylogenetic tree was constructed using IQ-TREE (Nguyen et al. Citation2015), and visualized using FigTree v1.4.3. In addition, the CsPLCP protein sequences were used to generate another M-L phylogenetic tree ((A)).
Cis-acting element analysis of CsPLCP gene family
The upstream regions (2,000 bp) of CsPLCP genes were extracted manually using a self-written Perl script and submitted to PlantCARE to search for cis-acting elements (Lescot et al. Citation2002). TBtools was used to display the distribution of abiotic- and biotic-responsive cis-acting elements (Chen et al. Citation2020).
Expression profiles of CsPLCPs in public database
Raw RNA-seq data (Chin et al. Citation2020; Tang et al. Citation2021) were acquired from NCBI using SRA Toolkit 2.11.0 and mapped to the reference genome using Hisat2 (Kim et al. Citation2019). The FPKM values of transcripts were calculated using StringTie (Pertea et al. Citation2016). Expression heatmaps were visualized using the R package ‘Pheatmap’ (Kolde and Kolde Citation2018).
RNA isolation and qPCR analysis
Genomic DNA was extracted from the midribs of CLas-infected and uninfected ‘Newhall’ navel orange leaves using the CTAB method (Clarke Citation2009). The presence of CLas was detected by quantitative polymerase chain reaction (qPCR) using the specific CQULA03F/CQULA03R primers (Wang et al. Citation2006). The three CLas-positive samples had Ct values of 25.5, 24.6, and 26.3, respectively, while Ct values were higher than 40 in uninfected samples. Total RNA was extracted from leaves of CLas-infected and uninfected ‘Newhall’ navel oranges using a TaKaRa MiniBEST Universal RNA Extraction Kit (TaKaRa, Dalian). First strand cDNA was synthesized using a PrimeScript RT reagent Kit with gDNA Eraser (Perfect Real Time) (TaKaRa, Dalian) and diluted 5 times for qPCR detection of CsPLCP gene expression profiles. All primers used in this experiment can be found in Table S1.
Subcellular localization analysis
The coding sequences of CsALP1, CsCTB1, CsSAG12-14, CsXBCP2, CsRD21-2, and CsRD19-3 were amplified and inserted into the pCAMBIA2300-GFP binary vector at Sac I and Xba I restriction endonuclease sites using a pEASY-Basic Seamless Cloning and Assembly Kit (Transgen, Beijing). All primers used in this experiment can be found in Table S1. The recombinant vectors were transferred into Agrobacterium tumefaciens strain ‘GV3101.’ Monoclonal colonies were cultured overnight in LB medium containing 20 μg ml−1 rifampicin and 50 μg ml−1 kanamycin, and then resuspended using MES buffer (10 mM MES, 10 mM MgCl2, pH 5.6) containing 200 μM acetosyringone. The resuspended cultures were incubated at 25℃ for 2 hours and diluted to OD600 = 0.4, followed by infiltration into Nicotiana benthamiana leaves (Huang et al. Citation2019). The subcellular localizations of the six proteins were observed using a LEICA SP8 confocal laser microscope at 3 days post infiltration.
Statistical analysis
Statistically significant differences among the qPCR expression data were determined by one way ANOVA using SPSS 25.0 software.
Results
Identification and phylogenetic analysis of the CsPLCP gene family
A total of 33 CsPLCP genes were identified across the C. sinensis genome using HMMsearch and local BLAST ( and Table S2), and were renamed according to their subfamilies and chromosomal locations. The CsPLCP proteins ranged in length from 218 to 686 amino acids, with isoelectric points ranging from 4.39 to 9.97 and molecular weights ranging from 24.46 to 76.38 KDa (Table S2). To elucidate the phylogenetic relationships among PLCP genes from A. thaliana, C. sinensis, and V. vinifera, an M-L tree was constructed using 87 AtPLCP, CsPLCP, and VvPLCP protein sequences. The PLCPs clustered into nine subfamilies, of which the SAG12 subfamily was the largest (29 members) and the THI subfamily was the smallest (3 members) (). The SAG12 subfamily was significantly expanded in C. sinensis (17 members), while the other 8 subfamilies consisted of only 16 members. In C. sinensis, there were 3 members each in the RD19, RD21, and XCP subfamilies; 2 members each in the CEP and XBCP subfamilies; and one member each in the ALP, CTB, and THI subfamilies. This was similar to V. vinifera, wherein SAG12 was the largest subfamily, and the ALP, CTB, and THI subfamilies contained one member each (Kang et al. Citation2021). In A. thaliana, the largest subfamily was RD21, while the smallest subfamilies were XBCP and THI (one member each) (). The results of our phylogenetic analysis indicate that functional diversification events occurred in the PLCP gene family.
Distribution and collinearity analysis of the CsPLCP gene family
The CsPLCP genes exhibited heterogenous distribution across chromosomes 1, 2, 3, 4, 5, 7, 8, and 9 ((A)). Collinearity analysis between C. sinensis and A. thaliana was carried out to reveal homology between CsPLCPs and AtPLCPs, and 18 pairs of genes exhibited credible linkages ((B)).
Gene structure and conserved motif analysis of the CsPLCP gene family
To further study the phylogenetic relationships among the conserved motifs and domains of the CsPLCP proteins, we constructed an M-L tree with 9 clades using CsPLCP protein sequences ((A)). In addition, the exon-intron structures of CsPLCP genes were analyzed to understand the structural diversity in this gene family. The CsPLCP genes contained between 1 and 8 exons (1 with one exon, 1 with two exons, 4 with seven exons, 4 with eight exons, and 1 with nine exons) ((B)). Using the MEME tool, we found that motifs 1 and 6 were present in all CsPLCP proteins ((C)). Additionally, most of the CsPLCP proteins contained motifs 3, 4, and 5, with the exception of CsCTB1 ((C)).
Analysis of cis-acting elements of the CsPLCP gene family
Promoter cis-acting elements regulate gene expression in response to an array of biotic and abiotic factors. To identify cis-acting elements in the promoters of the CsPLCP genes, we used the PlantCare database to analyze promoter sequences (2000 bp) upstream of the coding sequences. A total of 6 categories of cis-acting elements related to stress and phytohormone response were identified (). Of the 33 CsPLCPs, 29 contained abscisic acid-responsive elements, 8 contained defence- and stress-responsive elements, 17 contained gibberellin-responsive elements, 24 contained low-temperature-responsive elements, 29 contained methyl jasmonate (MeJA)-responsive elements, and 1 (CsXCP1) contained a wound-responsive element ().
Expression profile analysis of CsPLCP genes in response to HLB infection
To evaluate the expression profiles of CsPLCP genes in response to CLas and Xcc infection, the expression profiles of CsPLCP genes were analyzed using RNA-seq data generated in a previous study. The expression profiles of CsPLCP genes were clearly altered upon CLas infection ((A)). Several CsPLCPs (CsSAG12-7, CsSAG12-8, CsSAG12-9, CsSAG12-10, CsSAG12-14, CsSAG12-15, CsSAG12-17, and CsXCP) were down-regulated at 8 weeks post-infection. However, these same genes were up-regulated at 26- and 46-weeks post-infection ((A)). Several other genes (CsALP1, CsTHI1, CsSAG12-12, CsSAG12-13, CsCEP2, and CsXBCP1) were also up-regulated at 26 weeks post-infection, compared to the control samples ((A)). Interestingly, more genes were up-regulated at 26 weeks or 46 weeks post-infection. In addition, the dynamic expression of CsPLCP genes in response to Xcc infection was analyzed. The expression levels of many CsPLCP genes were up-regulated at 24- or 48-hours post-infection, with the exception of CsXBCP1, CsRD21-3, CsCEP1, CsXBCP2, CsRD19-2, and CsXCP2 ((B)). These results suggest that the majority of CsPLCP genes are involved in the response to pathogen infection.
Figure 5. Expression profile analysis of CsPLCP genes. (A) Huanglongbing samples; (B) Citrus bacterial canker disease samples.
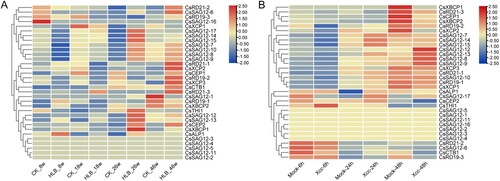
In order to investigate the expression patterns of CsPLCP genes during CLas infection, both mature and young leaves from uninfected and CLas-infected Newhall navel orange trees were subjected to qPCR analysis. Based on their phylogenetic relationships and expression profiles, the expression patterns of CsALP1, CsCTB1, CsSAG12-14, and CsXBCP2 (representing four PLCP subfamilies) were analyzed further. The four CsPLCP genes were all up-regulated in young and mature CLas-infected leaves. For example, CsALP1 expression was 15 times higher in mature CLas-infected leaves than in mature uninfected leaves, and 6 times higher in young CLas-infected leaves than in young uninfected leaves. Moreover, the expression of CsALP1 and CsSAG12-14 was significantly higher in mature leaves than in young leaves after CLas-infection ((A)). Overall, the qPCR-validated expression patterns of CsALP1, CsCTB1, and CsSAG12-14 were consistent with the RNA-seq data, while CsXBCP2 was up-regulated at 8 weeks post-infection and down-regulated at 18 weeks post-infection according to the RNA-seq results ((A)).
Figure 6. Expression profile analysis of CsPLCP genes in response to CLas infection. Expression patterns of CsALP1 (A), CsCTB1 (B), CsSAG12-14 (C), and CsXBCP2 (D), as determined by qPCR. NB1: young CLas-infected leaves of ‘Newhall’ navel orange; NH1: young uninfected leaves; NB2: mature CLas-infected leaves; NH1: mature uninfected leaves. The CsGAPDH gene was used as the internal control, and the expression level of each PLCP gene in sample NH1 was set as 1. Error bars represent the standard deviation (SD) of three independent biological replicates. Different letters indicate statistically significant differences (one way ANOVA).
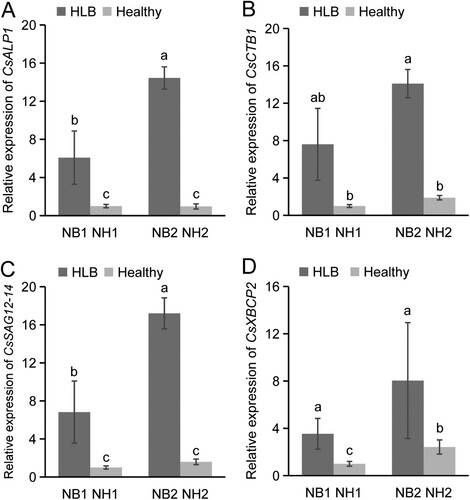
Subcellular localization analysis of CsPLCP proteins
The subcellular locations of proteins are associated with their biological functions. To understand the roles played by CsPLCP proteins, CsALP1, CsCTB1, CsSAG12-14, CsXBCP2, CsRD21-2, and CsRD19-3 were transiently expressed in N. benthamiana leaves. CsALP1, CsCTB1, CsSAG12-14, CsRD21-2, and CsRD19-3 were localized to the cytoplasm and nucleus, while CsXBCP2 was localized only to the cytoplasm ().
Discussion
The PLCP family has been documented in various plants, including A. thaliana (Cannon et al. Citation2004), soybean (Van Wyk et al. Citation2014), papaya (Liu et al. Citation2018), cotton (Zhang et al. Citation2019), rice (Niño et al. Citation2020), fig (Zhai et al. Citation2021), and grape (Kang et al. Citation2021). However, the number of PLCP members varies greatly among different plants. For example, 33 PLCP genes were identified in papaya (Liu et al. Citation2018), while 23 PLCP genes were identified in grape (Kang et al. Citation2021). In the present study, 33 CsPLCP genes were identified across the C. sinensis genome. Interestingly, C. sinensis contained the same number of PLCP members as papaya, and their genome sizes are nearly the same (Ming et al. Citation2008; Xu et al. Citation2013). Although grape has a larger genome than either C. sinensis or papaya, it contains fewer PLCP members. Such disparities may be due to differences in the frequency of gene duplication or genome duplication events in different species.
In plants, PLCPs are divided into nine subfamilies and each subfamily contains different numbers of PLCPs. In C. sinensis, the SAG12 subfamily is the largest, containing 17 members (), which is similar to grape (Kang et al. Citation2021). In A. thaliana, the RD21 subfamily is the largest and contains 9 members (Cannon et al. Citation2004). In C. sinensis, the THI subfamily is the smallest, with only one member (). Likewise, this subfamily contains only one member in fig (Zhai et al. Citation2021), grape (Kang et al. Citation2021), and papaya (Liu et al. Citation2018). Howerver, there are 12 members in the THI subfamily in rice (Liu et al. Citation2018; Niño et al. Citation2020). It is likely that differences in the number of members in each subfamily correspond to the functional differentiation of PLCPs in different species.
The functions of PLCP family members were evaluated by studying their protein domains, motifs, and gene structrues. In fig, PLCP members within each subfamily are characterized by similar motifs and gene structures (Zhai et al. Citation2021). The same phenomenon was observed in C. sinensis, indicating that PLCP members within the same subfamily contain conserved domains (). For example, most of the SAG12 subfamily members contain one intron, while members of the RD19 subfamily contain three introns, in C. sinensis. Similar exon-intron gene structures have been reported for the PLCP family in castor bean and physic nut (Zou et al. Citation2018). Motif analysis indicated that motifs 1, 2, and 6 were conserved throughout the PLCP family in C. sinensis. The key amino acids (Cys, Asn, and His) responsible for the catalytic activity of PLCPs (Richau et al. Citation2012) were identified in motifs 1, 2, and 8, respectivily.
The cis-acting elements distributed on gene promoters regulate gene expression in response to an array of biotic and abiotic factors. Unlike the similarity observed for motifs and exon-intron structures, the distribution of cis-acting elements was irregular and polymorphic. For example, the promoter of CsCTB1 contained a MeJA-responsive element (), while the promoter of FcCTB1 in fig contained a drought-inducibility element. However, the promoter of FcCTB2 contained 18 cis-acting elements, including auxin-responsive, abscisic acid-responsive, MeJA-responsive, gibberellin-responsive, salicylic acid-responsive, and low-temperature-responsive elements (Zhai et al. Citation2021). In the SAG12 subfamily, the promoter of CsSAG12-11 contained 7 abscisic acid-responsive elements, while the promoters of CsSAG12-1 and CsSAG12-6 did not (). Although PLCP members in the same subfamily may perform the same or similar functions, different expression profiles and regulatory mechanisms may be the cause of functional differentiation among the PLCP gene family (Clark et al. Citation2018).
Previous studies report that PLCP family members exhibit dynamic expression patterns during seed germination, programmed cell death, leaf senescence, and immune response (Zhao et al. Citation2013 Liu et al. Citation2018;). Knockout of the tomato PLCP genes Rcr3 and Pip1 enhanced susceptibility to P. infestans (Song et al. Citation2009; Ilyas et al. Citation2015), while overexpression of the PLCP genes C14 and C14pep in N. benthaminana enhanced resistance to P. infestans (Bozkurt et al. Citation2011). The activity of PLCPs have been found to be intensively induced by salicylic acid treatment in plants such as maize and citrus (van der Linde et al. Citation2012 Clark et al. Citation2018;). In a recent study, CsPLCP gene expression was significantly up-regulated during salicylic acid treatment and CLas infection (Clark et al. Citation2018). We also found that CsPLCPs were induced by CLas infection, further demonstrating that the PLCP family plays an important role in plant-pathogen interactions.
Determining the precise subcellular localization of PLCPs helps clarify their functions, as well as interactions between PCLPs and pathogen effectors. In this study, we found that CsALP1, CsCTB1, CsSAG12-14, CsRD21-2, and CsRD19-3 were localized to the cytoplasm and nucleus, while CsXBCP2 was localized only to the cytoplasm (). In A. thaliana, RD21 was shown to be localized to ER bodies and vacuoles (Lampl et al. Citation2013), RD19 was targeted to mobile vacuole-associated compartments but could be relocalized to the nucleus by effector PopP2 (Bernoux et al. Citation2008), and RD19c exhibited intramembrane localization in protoplasts (Dervisi et al. Citation2022). Such disparities in subcellular localization may be due to the functional differentiation of different family members.
Conclusion
In summary, a total of 33 PLCP genes were identified across the genome of C. sinensis. The CsPLCP genes were heterogeneously distributed on 8 chromosomes and exhibited diverse gene structures and conserved motifs. Dynamic expression analysis indicated that several CsPLCPs were induced by CLas infection. Moreover, the subcellular localizations of 6 selected PLCPs were in coordination with their functions. Our identification and comprehensive analysis of CsPLCPs provides a foundation for further study of their functions in the Citrus-CLas interaction.
Supplemental Material
Download Zip (16.9 KB)Acknowledgements
The authors would like to thank TopEdit (www.topeditsci.com) for improving the grammar in this manuscript.
Disclosure statement
No potential conflict of interest was reported by the author(s).
Data availability statement
All data generated or analysed during this study are included in this manuscript.
Additional information
Funding
Notes on contributors
Tianyu Li
Tianyu Li Graduate student, College of Life Science, Gannan Normal University, is engaged in Citrus-Huanglongbing interaction analysis.
Chang Liu
Chang Liu Graduate student, College of Life Science, Gannan Normal University.
Xiaopeng Chang
Xiaopeng Chang Graduate student, College of Life Science, Gannan Normal University.
Fuxuan Li
Fuxuan Li Graduate student, College of Life Science, Gannan Normal University.
Tao Wang
Tao Wang Undergraduate student, College of Life Science, Gannan Normal University.
Guiyan Huang
Guiyan Huang Associate professor, College of Life Science, Gannan Normal University.
Ruimin Li
Ruimin Li Associate professor, College of Life Science, Gannan Normal University, is engaged in Citrus-Huanglongbing interaction analysis.
References
- Bailey TL, Boden M, Buske FA, Frith M, Grant CE, Clementi L, Ren J, Li WW, Noble WS. 2009. MEME SUITE: tools for Motif discovery and searching. Nucleic Acids Res. 37:W202–W208. doi:10.1093/nar/gkp335.
- Balakireva AV, Zamyatnin A. 2018. Indispensable role of proteases in plant innate immunity. Int J Mol Sci. 19:629. doi:10.3390/ijms19020629.
- Bassanezi RB, Montesino LH, Stuchi ES. 2009. Effects of Huanglongbing on fruit quality of sweet orange cultivars in Brazil. Eur J Plant Pathol. 125:565–572. doi:10.1007/s10658-009-9506-3.
- Bernoux M, Timmers T, Jauneau A, Brière C, de Wit PJGM, Marco Y, Deslandes L. 2008. RD19, an Arabidopsis cysteine protease required for RRS1-R–mediated resistance, is relocalized to the nucleus by the Ralstonia solanacearum PopP2 effector. Plant Cell. 20:2252–2264. doi:10.1105/tpc.108.058685.
- Bhalerao R, Keskitalo J, Sterky F, Erlandsson R, Bjorkbacka H, Birve SJ, Karlsson J, Gardestrom P, Gustafsson P, Lundeberg J. 2003. Gene expression in autumn leaves. Plant Physiol. 131:430–442. doi:10.1104/pp.012732.
- Bové JM. 2006. Huanglongbing: a destructive, newly-emerging, century-old disease of citrus. J Plant Pathol. 88(1):7–37. doi:10.4454/jpp.v88i1.828.
- Bozkurt TO, Schornack S, Win J, Shindo T, Ilyas M, Oliva R, Cano LM, Jones AME, Huitema E, van der Hoorn RAL. 2011. Phytophthora infestans effector AVRblb2 prevents secretion of a plant immune protease at the Haustorial interface. Proc Natl Acad Sci USA. 108:20832–20837. doi:10.1073/pnas.1112708109.
- Cannon SB, Mitra A, Baumgarten A, Young ND, May G. 2004. The roles of segmental and tandem gene duplication in the evolution of large gene families in Arabidopsis thaliana. BMC Plant Biol. 4:1–21. doi:10.1186/1471-2229-4-10.
- Castano-Duque L, Loades KW, Tooker JF, Brown KM, Paul Williams W, Luthe DS. 2017. A maize inbred exhibits resistance against western corn rootwoorm, Diabrotica virgifera virgifera. J Chem Ecol. 43:1109–1123. doi:10.1007/s10886-017-0904-2.
- Chen Q-j, Deng B-h, Gao J, Zhao Z-y, Chen Z-l, Song S-r, Wang L, Zhao L-p, Xu W-p, Zhang C-X, et al. 2020. A miRNA-encoded small peptide, vvi-miPEP171d1, regulates adventitious root formation. Plant Physiol. 183:656. doi:10.1104/pp.20.00197.
- Chin EL, Ramsey JS, Mishchuk DO, Saha S, Foster E, Chavez JD, Howe K, Zhong X, Polek M, Godfrey KE, et al. 2020. Longitudinal transcriptomic, proteomic, and metabolomic analyses of Citrus sinensis (L.) Osbeck graft-inoculated with “Candidatus Liberibacter asiaticus”. J Proteom Res. 19:719–732. doi:10.1021/acs.jproteome.9b00616.
- Clark K, Franco JY, Schwizer S, Pang Z, Hawara E, Liebrand TWH, Pagliaccia D, Zeng L, Gurung FB, Wang P, et al. 2018. An effector from the Huanglongbing-associated pathogen targets citrus proteases. Nat Commun. 9:1718. doi:10.1038/s41467-018-04140-9.
- Clarke JD. 2009. Cetyltrimethyl ammonium bromide (CTAB) DNA miniprep for plant DNA isolation. Cold Spring Harb Protoc. 2009(3):pdb.prot5177. doi:10.1101/pdb.prot5177.
- Coulombe R, Grochulski P, Sivaraman J, Menard R, Mort JS, Cygler M. 1996. Structure of human procathepsin L reveals the molecular basis of inhibition by the prosegment. EMBO J. 15:5492–5503. doi:10.1002/j.1460-2075.1996.tb00934.x.
- Dervisi I, Haralampidis K, Roussis A. 2022. Investigation of the interaction of a papain-like cysteine protease (RD19c) with selenium-binding protein 1 (SBP1) in Arabidopsis thaliana. Plant Sci. 315:111157. doi:10.1016/j.plantsci.2021.111157.
- Dubey VK, Pande M, Singh BK, Jagannadham MV. 2007. Papain-like proteases: applications of their inhibitors. Afr J Biotechnol. 6(9):1077–1086. doi:10.5897/AJB2007.000-2139.
- Duvaud S, Gabella C, Lisacek F, Stockinger H, Ioannidis V, Durinx C. 2021. Expasy, the Swiss bioinformatics resource portal, as designed by its users. Nucleic Acids Res. 49:W216–W227. doi:10.1093/nar/gkab225.
- Huang G, Liu Z, Gu B, Zhao H, Jia J, Fan G, Meng Y, Du Y, Shan W. 2019. An RXLR effector secreted by Phytophthora parasitica is a virulence factor and triggers cell death in various plants. Mol Plant Pathol. 20:356–371. doi:10.1111/mpp.12760.
- Ilyas M, Hörger AC, Bozkurt TO, Van Den Burg HA, Kaschani F, Kaiser M, Belhaj K, Smoker M, Joosten MHAJ, Kamoun S. 2015. Functional divergence of two secreted immune proteases of tomato. Curr Biol. 25:2300–2306. doi:10.1016/j.cub.2015.07.030.
- Kalyaanamoorthy S, Minh BQ, Wong TKF, Haeseler V, Jermiin A, S L. 2017. ModelFinder: fast model selection for accurate phylogenetic estimates. Nat Methods. 14:587–589. doi:10.1038/nmeth.4285.
- Kang J, Gong P, Ge M, Sadeghnezhad E, Liu Z, Zhang M, Shangguan L, Fang J. 2021. The PLCP gene family of grapevine (Vitis vinifera L.): characterization and differential expression in response to Plasmopara viticola. BMC Plant Biol. 21:1–14. doi:10.1186/s12870-020-02777-7.
- Katoh K, Standley DM. 2013. MAFFT multiple sequence alignment software version 7: improvements in performance and usability. Mol Biol Evol. 30:772–780. doi:10.1093/molbev/mst010.
- Kim D, Paggi JM, Park C, Bennett C, Salzberg SL. 2019. Graph-based genome alignment and genotyping with HISAT2 and HISAT-genotype. Nat Biotechnol. 37:907–915. doi:10.1038/s41587-019-0201-4.
- Kolde R, Kolde MR. 2018. Package ‘pheatmap’. R package.1.
- Lampl N, Alkan N, Davydov O, Fluhr R. 2013. Set-point control of RD21 protease activity by AtSerpin1 controls cell death in Arabidopsis. Plant J. 74:498–510. doi:10.1111/tpj.12141.
- Lescot M, Déhais P, Thijs G, Marchal K, Moreau Y, Van de Peer Y, Rouzé P, Rombauts S. 2002. PlantCARE, a database of plant cis-acting regulatory elements and a portal to tools for in silico analysis of promoter sequences. Nucleic Acids Res. 30:325–327. doi:10.1093/nar/30.1.325.
- Liu H, Hu M, Wang Q, Cheng L, Zhang Z. 2018a. Role of papain-like cysteine proteases in plant development. Front Plant Sci. 9:1717. doi:10.3389/fpls.2018.01717.
- Liu H, Wang X, Liu S, Huang Y, Guo Y-X, Xie W-Z, Liu H, ul Qamar MT, Xu Q, Chen L-L. 2022. Citrus Pan-genome to breeding database (CPBD): a comprehensive genome database for citrus breeding. Mol Plant. 15:1503–1505. doi:10.1016/j.molp.2022.08.006.
- Liu J, Sharma A, Niewiara MJ, Singh R, Ming R, Yu Q. 2018b. Papain-like cysteine proteases in Carica papaya: lineage-specific gene duplication and expansion. BMC Genom. 19:1–12. doi:10.1186/s12864-017-4368-0.
- Marchler-Bauer A, Derbyshire MK, Gonzales NR, Lu S, Chitsaz F, Geer LY, Geer RC, He J, Gwadz M, Hurwitz DI. 2015. CDD: NCBI's conserved domain database. Nucleic Acids Res. 43:D222–D226. doi:10.1093/nar/gku1221.
- Ming R, Hou S, Feng Y, Yu Q, Dionne-Laporte A, Saw JH, Senin P, Wang W, Ly BV, Lewis KLT, et al. 2008. The draft genome of the transgenic tropical fruit tree papaya (Carica papaya Linnaeus). Nature. 452:991–996. doi:10.1038/nature06856.
- Misas-Villamil JC, van der Hoorn RAL, Doehlemann G. 2016. Papain-like cysteine proteases as hubs in plant immunity. New Phytol. 212:902–907. doi:10.1111/nph.14117.
- Mistry J, Chuguransky S, Williams L, Qureshi M, Salazar GA, Sonnhammer ELL, Tosatto SCE, Paladin L, Raj S, Richardson LJ. 2021. Pfam: the protein families database in 2021. Nucleic Acids Res. 49:D412–D419. doi:10.1093/nar/gkaa913.
- Nguyen L-T, Schmidt HA, Haeseler V, Minh A, Q B. 2015. IQ-TREE: a fast and effective stochastic algorithm for estimating maximum-likelihood phylogenies. Mol Biol Evol. 32:268–274. doi:10.1093/molbev/msu300.
- Niño MC, Kang KK, Cho Y-G. 2020. Genome-wide transcriptional response of papain-like cysteine protease-mediated resistance against Xanthomonas oryzae pv. oryzae in rice. Plant Cell Rep. 39:457–472. doi:10.1007/s00299-019-02502-1.
- Ormancey M, Thuleau P, van Der Hoorn RAL, Grat S, Testard A, Kamal KY, Boudsocq M, Cotelle V, Mazars C. 2019. Sphingolipid-induced cell death in Arabidopsis is negatively regulated by the papain-like cysteine protease RD21. Plant Sci. 280:12–17. doi:10.1016/j.plantsci.2018.10.028.
- Parrott DL, Martin JM, Fischer AM. 2010. Analysis of barley (Hordeum vulgare) leaf senescence and protease gene expression: a family C1A cysteine protease is specifically induced under conditions characterized by high carbohydrate, but low to moderate nitrogen levels. New Phytol. 187:313–331. doi:10.1111/j.1469-8137.2010.03278.x.
- Pérez-López E, Hossain MM, Wei Y, Todd CD, Bonham-Smith PC. 2021. A clubroot pathogen effector targets cruciferous cysteine proteases to suppress plant immunity. Virulence. 12:2327–2340. doi:10.1080/21505594.2021.1968684.
- Pertea M, Kim D, Pertea GM, Leek JT, Salzberg SL. 2016. Transcript-level expression analysis of RNA-seq experiments with HISAT, StringTie and Ballgown. Nat Protoc. 11:1650–1667. doi:10.1038/nprot.2016.095.
- Planas-Marquès M, Bernardo-Faura M, Paulus J, Kaschani F, Kaiser M, Valls M, van der Hoorn RAL, Coll NS. 2018. Protease activities triggered by Ralstonia solanacearum infection in susceptible and tolerant tomato lines. Mol Cell Proteom. 17:1112–1125. doi:10.1074/mcp.RA117.000052.
- Rawlings ND, Barrett AJ, Thomas PD, Huang X, Bateman A, Finn RD. 2018. The MEROPS database of proteolytic enzymes, their substrates and inhibitors in 2017 and a comparison with peptidases in the PANTHER database. Nucleic Acids Res. 46:D624–D632. doi:10.1093/nar/gkx1134.
- Rawlings ND, Waller M, Barrett AJ, Bateman A. 2014. MEROPS: the database of proteolytic enzymes, their substrates and inhibitors. Nucleic Acids Res. 42:D503–D509. doi:10.1093/nar/gkt953.
- Richau KH, Kaschani F, Verdoes M, Pansuriya TC, Niessen S, Stüber K, Colby T, Overkleeft HS, Bogyo M, Van der Hoorn RAL. 2012. Subclassification and biochemical analysis of plant papain-like cysteine proteases displays subfamily-specific characteristics. Plant Physiol. 158:1583. doi:10.1104/pp.112.194001.
- Shindo T, Kaschani F, Yang F, Kovács J, Tian F, Kourelis J, Hong TN, Colby T, Shabab M, Chawla R. 2016. Screen of non-annotated small secreted proteins of Pseudomonas syringae reveals a virulence factor that inhibits tomato immune proteases. PLoS Pathog. 12:e1005874. doi:10.1371/journal.ppat.1005874.
- Song J, Win J, Tian M, Schornack S, Kaschani F, Ilyas M, van der Hoorn RA, Kamoun S. 2009. Apoplastic effectors secreted by two unrelated eukaryotic plant pathogens target the tomato defense protease Rcr3. Proc Natl Acad Sci U S A. 106:1654–1659. doi:10.1073/pnas.0809201106.
- Tang X, Wang X, Huang Y, Ma L, Jiang X, Rao MJ, Xu Y, Yin P, Yuan M, Deng X, et al. 2021. Natural variations of TFIIAγ gene and LOB1 promoter contribute to citrus canker disease resistance in Atalantia buxifolia. PLoS Genet. 17:e1009316. doi:10.1371/journal.pgen.1009316.
- Thoenen M, Herrmann B, Feller U. 2007. Senescence in wheat leaves: is a cysteine endopeptidase involved in the degradation of the large subunit of Rubisco? Acta Physiol Plant. 29:339–350. doi:10.1007/s11738-007-0043-4.
- Turk D, Guncar G, Podobnik M, Turk B. 1998. Revised definition of substrate binding sites of papain-like cysteine proteases. Biol Chem. 379:137–147. doi:10.1515/bchm.1998.379.2.137.
- Van der Hoorn RAL. 2008. Plant proteases: from phenotypes to molecular mechanisms. Annu Rev Plant Biol. 59:191–223. doi:10.1146/annurev.arplant.59.032607.092835.
- van der Linde K, Hemetsberger C, Kastner C, Kaschani F, van der Hoorn RAL, Kumlehn J, Doehlemann G. 2012. A maize cystatin suppresses host immunity by inhibiting apoplastic cysteine proteases. Plant Cell. 24:1285–1300. doi:10.1105/tpc.111.093732.
- Van Wyk SG, Du Plessis M, Cullis CA, Kunert KJ, Vorster BJ. 2014. Cysteine protease and cystatin expression and activity during soybean nodule development and senescence. BMC Plant Biol. 14:1–13. doi:10.1186/s12870-014-0294-3.
- Wang Y, Tang H, Debarry JD, Tan X, Li J, Wang X, Lee T-H, Jin H, Marler B, Guo H. 2012. MCScanX: a toolkit for detection and evolutionary analysis of gene synteny and collinearity. Nucleic Acids Res. 40:e49. doi:10.1093/nar/gkr1293.
- Wang Z, Yin Y, Hu H, Yuan Q, Peng G, Xia Y. 2006. Development and application of molecular-based diagnosis for ‘Candidatus Liberibacter asiaticus’, the causal pathogen of citrus huanglongbing. Plant Pathol. 55:630–638. doi:10.1111/j.1365-3059.2006.01438.x.
- Wu F, Cen Y, Deng X, Chen J, Xia Y, Liang G. 2015. Movement of Diaphorina citri (Hemiptera: Liviidae) adults between huanglongbing-infected and healthy citrus. Fla Entomol. 98:410–416. doi:10.1653/024.098.0203.
- Wu H, Hu Y, Fu S, Zhou C, Wang X. 2020. Coordination of multiple regulation pathways contributes to the tolerance of a wild citrus species (Citrus ichangensis ‘2586’) against Huanglongbing. Physiol Mol Plant Pathol. 109:101457. doi:10.1016/j.pmpp.2019.101457.
- Xu Q, Chen L-L, Ruan X, Chen D, Zhu A, Chen C, Bertrand D, Jiao W-B, Hao B-H, Lyon MP, et al. 2013. The draft genome of sweet orange (Citrus sinensis). Nat Genet. 45:59–66. doi:10.1038/ng.2472.
- Yang Y, Dong C, Yu J, Shi L, Tong C, Li Z, Huang J, Liu S. 2014. Cysteine Protease 51 (CP51), an anther-specific cysteine protease gene, is essential for pollen exine formation in Arabidopsis. Plant Cell Tissue Organ Culture. 119:383–397. doi:10.1007/s11240-014-0542-0.
- Zhai Y, Cui Y, Song M, Vainstein A, Chen S, Ma H. 2021. Papain-like cysteine protease gene family in fig (Ficus carica L.): genome-wide analysis and expression patterns. Front Plant Sci. 12:681801. doi:10.3389/fpls.2021.681801.
- Zhang S, Xu Z, Sun H, Sun L, Shaban M, Yang X, Zhu L. 2019. Genome-wide identification of papain-like cysteine proteases in Gossypium hirsutum and functional characterization in response to Verticillium dahliae. Front Plant Sci. 10:134. doi:10.3389/fpls.2019.00134.
- Zhao P, Zhou X-M, Zhang L-Y, Wang W, Ma L-G, Yang L-B, Peng X-B, Bozhkov PV, Sun M-x. 2013. A bipartite molecular module controls cell death activation in the basal cell lineage of plant embryos. PLoS Biol. 11:e1001655. doi:10.1371/journal.pbio.1001655.
- Zou Z, Huang Q, Xie G, Yang L. 2018. Genome-wide comparative analysis of papain-like cysteine protease family genes in castor bean and physic nut. Sci Rep. 8(331):1–13. doi:10.1038/s41598-017-18760-6.