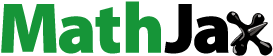
ABSTRACT
The allelopathic effect of Callistemon viminalis’s leaf extracts on seed germination and growth of Triticum aestivum and Cicer arietinum, as well as associated weeds (Anagallis arvensis, Poa annua, Lepidium didymium, and Vicia sativa), was studied. Different concentrations (2–10%) of aqueous extracts were used, and physiological parameters were measured. Higher concentrations (6–10%) significantly inhibited the germination and growth of wheat and chickpea. In pot assay, the extract did not affect shoot length, dry plant mass, chlorophyll, carotenoid, proline, protein content, stomatal conductance, photosynthetic and transpiration rate, and antioxidant enzymes in crop and various parameters of soil properties in comparison to the control. Both crops grew significantly at lower concentrations. This study suggests that extract of C. viminalis can inhibit the growth of A. arvensis and V. sativa without affecting germination, photosynthetic and biochemical properties of C. arietinum and T. aestivum, and soil properties, making it a potential candidate for bioherbicide development.
Introduction
Weeds are plants that grow in unwanted places and can negatively affect crops. They compete with cultivated plants for important resources such as water, nutrients, sunlight, and space. As a result, they can significantly reduce the yield and quality of crops, interfere with harvesting, and affect soil quality. Farmers use various weed control methods such as hand weeding, mechanical cultivation, mulching, crop rotation, and chemical herbicides to reduce the negative impact of weeds on crops. Integrated weed management strategies that combine multiple control methods are often the most effective and sustainable way to control weed populations in agricultural environments. The uncontrolled spread of weeds can significantly hamper crop growth, yield, and quality, posing a major challenge to sustainable agricultural practices in India. In response to the growing weed problem, farmers in India have increasingly used herbicides, chemical agents designed to kill or inhibit the growth of unwanted vegetation. Although herbicides can instantly eliminate weed infestations, their excess and indiscriminate use has caused serious environmental problems. Widespread use of herbicides has caused a number of negative impacts on ecosystems, soil health, water quality, and biodiversity. Despite the critical importance of weed control, there is a lack of effective and integrated weed control strategies in the country. Therefore, scientists and farmers are constantly looking for alternative, environment-friendly weed control methods. One such approach is the use of natural plant products that can inhibit the growth and development of nearby competing plants. The allelopathic effect has received much attention as a promising natural weed control strategy that can potentially reduce reliance on synthetic herbicides and promote a more ecologically balanced agricultural ecosystem.
Natural plant products such as allelochemicals offer a unique solution for weed control. Biologically active compounds have evolved over time in plants and have multiple ecological functions, including defense against competing plants and pests. Harnessing the power of allelochemicals provides an opportunity to develop sustainable weed control methods that minimize environmental impact while maintaining agricultural productivity. Allelochemical weed control is environment friendly, reduces reliance on herbicides, can improve crop productivity by reducing weed competition, improving soil nutrient availability, and is more economically feasible. Bioassay allows the detection of phytotoxicity in various species characterized by delayed seed germination, inhibition of plant growth, or other adverse effects caused by allelochemicals. Unlike chemical herbicides, allelochemicals act at low but constant concentrations over a long period of time (Lopes et al. Citation2022). This fact, together with the increase in global demand for organic agricultural products during the last two decades, makes an urgent investment in research on the use of allelochemicals as natural herbicides to promote more sustainable agriculture and minimize the effect of herbicides environment and human health as most of the allelochemicals are biodegradable and less polluting than traditional chemical herbicides.
C. viminalis, also known as bottle brush, is a Myrtaceae plant. It is a medium-sized tree with evergreen foliage. It can be found worldwide but is most common in humid tropical areas (Oyedeji et al. Citation2009). Some Callistemon species, particularly C. viminalis, are widely used as environmental bioindicators because they contain chemical compounds with insecticidal (Ndomo et al. Citation2010), fungicidal (Badawy and Abdelgaleil Citation2014; Somnuek et al. Citation2020), antibacterial (Uwaya et al. Citation2020) and antimicrobial properties (Silva et al. Citation2010). This plant contains diverse secondary metabolites, including phenolic, triterpenes, monoterpenes, steroids, alkaloids, saponins, and flavonoids (Ashmawy et al. Citation2014; Ahmad and Athar Citation2017). The first study on the phytotoxic effect of leaf extract of C. viminalis showed that the allelochemicals present in the plant could be extracted in water so that they can be used to suppress the growth of other plants (Cheema et al. Citation2013). The phenolics found in the leaf extract of C. viminalis can be related to the inhibitory effects of tested species on seed germination and radicle length. The phenolic acids found in the Leaf extract of C. viminalis, namely gallic acid, ferulic acid, syringic acid, and coumaric acid, are known phytotoxins that inhibit other plant’s growth (Batish et al. Citation2007; Sodaeizadeh et al. Citation2009). A study revealed that the leaf extracts of C. viminalis affected the weeds (Echinochloa crus-galli, Cyperus rotundus, Leptochloa chinensis, and Commelina benghalensis) more significantly than rice (Oryza sativa) in the laboratory bioassays. Based on these findings, it is possible to conclude that C. viminalis leaf extracts have a high potential for weed management, and more research could be conducted to develop them as a viable alternative to synthetic herbicides in sustainable agriculture under field conditions (Bali et al. Citation2017). The phenolic allelochemicals have been shown to inhibit root elongation and interfere with plant growth and development (Li et al. Citation2010). Most of the allelopathic potential studies of the plants mentioned above were conducted on essential oil, which is not a cost-effective option for farmers. So to make a green, economically efficient, and easy-to-use plant-based herbicide, we explored the aqueous extract of the leaves. The plant is easily available in India and can be grown per our needs.
The purpose of this study was to investigate the allelopathic effect of aqueous extract of C. viminalis leaves on two economically important cash crops, Triticum aestivum (wheat) and Cicer arietinum (chickpea), and four common weeds viz., Anagallis arvensi (Scarlet pimpernel), Vicia sativa (Common vetch), Lepidium. didymium (Swine cress), and Poa annua (Meadow grass).
2. Materials and methods
2.1. Experimental site and plant materials
The current study was conducted in Graphic Era Deemed to be university, Clement town, Dehradun, Uttarakhand, India. The coordinates of the city are 30.318°N and 78.029°E. The fundamental requirement for the study was leaves. The leaves of C. viminalis are used to make an aqueous extract for the study. The fresh green leaves of the plants were collected from the residential colony of Clement town, Dehradun, and proceeded forward to the extraction. The Systematic Botany Division of Forest Research Institute, Dehradun, Uttarakhand, India, identified and authenticated the plant.
2.2. Preparation of extract
Firstly, the leaves of C. viminalis were washed with running tap water to remove dust particles from the surface of the leaves. The leaves are then allowed to shade dry for around 2 h. After that, the leaves were shredded into small pieces to increase the surface area so they could contact the solvent, i.e. water. The leaves are then immersed in water in a closed glass vessel to begin the extract preparation. During this 24-hour process, the leaves were heated five times every 4 h. The boiling procedure was periodic, i.e. the leaves were allowed to boil for 10 min and then left to cool for 4 h. The extract was filtered with a double layer of muslin cloth to obtain crude extracts and then centrifuged for 20 min at 1000 rpm to remove particulate matter. The supernatant was collected and stored at 4°c until further use (Zain et al. Citation2013).
2.3. Petri-plate experiment and observation on seed germination-related parameters
Seeds of C. arietinum and T. aestivum were sterilized with 0.2% sodium hypochlorite solution for 10 min and rinsed with distilled water 3 times to avoid pathogen contamination. Two layers of filter paper were fixed in Petri plates (diameter = 90 mm) then ten seeds from the recipient species were placed and treated with 10 ml of C. viminalis aqueous extract (CVAE) in different concentrations (2%, 4%, 6%, 8%, and 10%) independently. Distilled water was used as a control, and 3 replicates of each treatment group were established. The plates were kept at room temperature of 22°C and a controlled 12-hour light and 12-hour dark cycle. 2 ml of the corresponding extract was added every 48 h to maintain the moisture of the filter paper in the Petri plates. The number of germinated seeds was counted from the second day to the last day, i.e. after a week. In addition, the seeds’ plumule and radicle length were recorded (Li et al. Citation2021) ().
2.4. Set up of pot experiments, growth condition, and experimental design
Pot experiments were set up in December 2021 and carried out till February 2022 in loamy soil. The pots were kept under standard weather conditions (10–26°C; 50–60% relative humidity; 14 h light/10 h dark) similar to normal field conditions in which chickpeas and wheat grow in India. The extract treatments were designed according to the observations recorded during the Petri-plate experiment. The 3 kg of agricultural soil was filled, and 4 plants were potted in each pot; the first extract dose was given in soil 2 days before planting plants. The extract treatment was given at different concentrations, i.e. 1%, 2%, 3%, 4%, and 5% (weight/volume). Distilled water was used as a control, and 3 replicates of each treatment group were established. A total of 2 doses of the corresponding extract were given. The plants that died in weed recipient species were counted (Li et al. Citation2021) ().
Figure 2. Triticum aestivum and Cicer arietinum plants treated with Callistemon viminalis aqueous extract (CVAE).
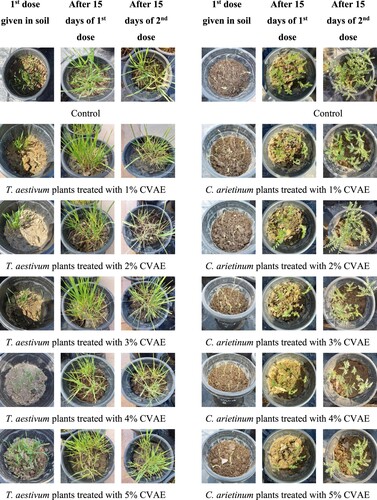
2.5. Photosynthetic parameters measurements
The quantification of photosynthetic pigments (chlorophyll a, chlorophyll b, and total chlorophyll) was performed by Arnon's (Citation1949) method. The fresh and dry weight of root and shoot and chlorophyll content of crop recipient species were calculated. Shoots were separated from the roots at the cotyledonary node, dried for 48 h at 65°C, and then weighed. 250 mg of leaves were ground in 10 ml of 80% acetone using a pestle and mortar. The paste was then centrifuged at 3000 RPM for 5 min. The mixture was then transferred to a 25 ml volumetric flask, and the volume was made up of acetone. The samples were then analyzed on a spectrophotometer (Labtronics instrument LT- 2800) at 663 and 645 nm for chlorophyll A and B, 652 nm for total chlorophyll, and 470 nm for carotenoids (Arnon Citation1949).
Where, A – Absorbance on Spectrophotometer. V – Final volume of supernatant. W – Fresh weight of the sample.
A Li 6400 photosynthetic system (Li-Cor, Inc., Nebraska, USA) was used to measure net photosynthetic rate (Pn), stomatal conductance (gs), and transpiration rate (E). Fully mature and expanded leaves from each replication were measured between 1000 and 1200 h in the morning, under photosynthetic flux density of 1,000 µmol m−2 s−1 provided by an LED light source. The leaf chamber had an air humidity of about 60%, a CO2 concentration of 370–380 µmol mol−1, an ambient air temperature of 26–28°C, and a flow rate of 500 µmol s−1 (Husen Citation2010).
Chlorophyll fluorescence measurements were taken between 1000 and 1100 h in the morning using a portable Hansatech Plant Efficiency Analyzer (Hansatech, King's Lynn, England). Before measuring, the leaves were darkened for 20 min with leaf clips. Red (actinic) radiation stimulated fluorescence with a peak wavelength of 650 nm emission by light-emitting diodes. The irradiance was set to 2000 µmol m−2 s−1. According to Genty et al. (Citation1989), the maximum quantum yield of photosystem II was estimated by the ratio Fv/Fm = (Fm-Fo)/Fm (Husen Citation2010).
2.6. Determination of biochemical parameters
2.6.1. Proline
The proline assay was performed using the Bates method (Citation1973). Firstly, Root tissue (0.2 g) was homogenized with 4 ml of 3% sulphosalicylic acid solution. Then 2 ml of the solution was then mixed with reactive ninhydrin (2 ml) in acetic acid (2 ml) and incubated in boiling water for 1 h. Following that time, samples were immediately transferred to an ice container. After the reaction was completed, the samples were allowed to settle until room temperature was reached. The samples were then mixed with 4 mL of toluene, and the resulting solution was vigorously shaken. A UV-Vis spectrophotometer (Perkin Elmer Lambda 25) was used to measure absorbance at 520 nm using the colored complex. Finally, the proline content was calculated in mol per gram of fresh weight (FW) using the following equation: (Hosseinzadeh et al. Citation2017).
2.6.2. Soluble protein
Protein content measurements in root tissue were done using Lowry et al. (Citation1951). This experiment is based on the hydrolysis of proteins and the release of amino acids, which results in colored complexes with the Fulvin reagent. The protein concentration in each sample was determined using the bovine serum albumin standard curve, and protein was calculated in mg/g DW (Hosseinzadeh et al. Citation2017). Finally, the samples’ absorption at 660 nm was measured using a spectrophotometer (Perkin Elmer Lambda 25).
2.6.3. Antioxidant enzymes assay
Each sample's leaf material (500 mg) was homogenized in an extraction buffer containing 0.5% Triton X-100 [C14H22O(C2H4O) n] and 1% polyvinylpyrrolidone [(C6H9NO) n] in 100 mM potassium phosphate buffer (pH 7.0). The crude extraction was prepared using a chilled mortar and pestle. After centrifuging the homogenate at 15,000 rpm for 20 min at 4°C, the supernatant was used for enzymatic assays.
A cold-temperature enzyme extract for superoxide dismutase (SOD) was prepared by first freezing a weighed amount of leaf sample (1 g) in liquid nitrogen to prevent proteolytic activity, then grinding with 10 ml of extraction buffer (0.1M phosphate buffer, pH 7.5, containing 0.5 mM EDTA and 1 mM ascorbic acid). It was briefly passed through four cheesecloth layers, and the filtrate was centrifuged for 20 min at 15,000xg at 4°C, with the supernatant used as an enzyme (Prochazkova et al. Citation2001). SOD activity was determined by measuring the enzyme's decrease in the optical density of nitroblue tetrazolium dye (Dhindsa et al. Citation1981). The reaction was started by adding 2 mM of riboflavin to the tubes and then placing them under two 15W fluorescent lamps for 15 min. The reaction mixture contained 13 mM methionine, 25 mM nitroblue tetrazolium chloride, 0.1 mM EDTA, 50 mM phosphate buffer (pH 7.8), 50 mM sodium carbonate, and 0.1 ml of the enzyme. As a control, a complete reaction mixture without enzymes produced the most color. The reaction was halted by turning off the light and leaving the tubes in the dark. The absorbance was measured at 560 nm with a Perkin Elmer UV/VIS spectrophotometer Lamda 25, and one unit of enzyme activity was defined as the amount of enzyme that reduced the absorbance reading to 50% when compared to tubes that did not contain an enzyme (Husen Citation2010). As a control, a non-irradiated complete reaction mixture was used.
PER activity was measured with guaiacol as the substrate, as Husen (Citation2008) described. The optical density (OD) was measured at 420 nm using a spectrophotometer (Perkin Elmer Lambda 25), and enzyme activity was expressed as μmoles H2O2 destroyed min−1 mg−1 protein (Husen Citation2008). 0.1 M phosphate buffer (pH 6.1), 4 mM guaiacol, 3 mM H2O2, and 0.4 ml crude enzyme extract were included in the assay mixture. The total volume of the reaction was 1.2 ml.
Chandlee and Scandalios (Citation1984) method was used to measure catalase enzyme activity (CAT). 2.5 ml of potassium phosphate 50 mmol L−1 and 0.3 ml of 3% H2O2 were mixed in an ice container. Then, immediately, 2.0 ml of enzyme extract was added. For 3–4 min, the absorbance variation curve at 240 nm was determined. The change in absorbance was used to calculate enzyme activity, which was expressed as mg protein−1 min−1 (Hosseinzadeh et al. Citation2017).
2.7. High-Performance Thin Layer Chromatography (HPTLC) analysis of allelopathic compounds
An aqueous extract of C. viminalis was analyzed using HPTLC. The extract was qualitatively analyzed for various phytoconstituents using TLC (thin layer chromatography) silica gel G-60 F254 (Merck) plates as stationary and mobile phases of toluene, ethyl acetate, methanol, and formic acid. A Biostep AS-30 applicator and an AS-30 filling syringe were used for the application. HPTLC plates precoated with silica gel G-60 F254 were used for fingerprinting to separate all the compounds in the extract better. Before use, the plates were washed with methanol to remove any impurities that may have accumulated during laboratory storage. The fingerprint of the extract was obtained by scanning the chromatographic plate at 254 nm. C. viminalis dried extract was weighed precisely and dissolved in 100 ml of methanol. The experiment was conducted at a constant temperature (22 ± 1°C) and humidity (47 ± 1%) (Shivarkar et al. Citation2018).
2.8. Physio-chemical analysis of soils
Soil samples were taken from the donor's extract-treated weed pots at the end of the experiment. All soil samples were collected randomly from three points of each pot, mixed uniformly, and kept inside the zipper plastic bags. Each soil sample was air-dried at room temperature after fine roots, stones, and plant organic residues were manually removed. Then, samples were crushed, sieved through a 2 mm mesh sieve, and homogeneously mixed for additional soil physicochemical analysis. The potential of hydrogen ion (pH), water holding capacity (WHC), soil texture, Nitrogen (N), Phosphorus (P), Potassium (K), Organic Carbon (OC), Organic matter (OM), Calcium (Ca), and Magnesium (Mg) of all soil samples were determined.
The pH was determined using a digital meter (Labtronics instrument LT- 49) (Kashyap et al. Citation2021). The hydrometer was used to measure soil texture (Agbeshie et al. Citation2020), while the WHC was used by gravimetric method (Estefan et al. Citation2013). Total Nitrogen was estimated by the Kjeldhal method using the Goyal et al. (Citation2022) method. UV- vis spectrophotometer (Labtronics instrument LT- 2800) was used to calculate phosphorus (McDowell and Condron Citation2004). Systronics flame photometer-128 measured potassium (Doruk and Venkatakrishnan Citation2022). Calcium, magnesium, organic carbon, and organic matter content were measured using an atomic absorption spectrophotometer (Analyst-800) by the APHA (2012) method (Ou-Zine et al. Citation2021; Lalrammawia et al. Citation2022).
The standard range of physio-chemical parameters of soil described by Krishi Vigyan Kendra (KVK) (Delhi) for good agricultural soil is mentioned in .
Table 1. Standard range of Krishi Vigyan Kendra.
2.9. Statistical analysis
The data obtained from the experiments were analyzed using Analysis of Variance (ANOVA). The Level of significance was taken as 0.05. The null hypothesis was rejected if the p-value of the test statistic (F) was less than or equal to 0.05. Whenever the null hypothesis was rejected, Tukey's post hoc test was used for pair-wise comparisons.
3. Results
3.1. Seed germination
The germination percentage (GP) of C. arietinum and T. aestivum was significantly (p ≤ 0.05) affected by different concentrations of C. viminalis aqueous extract (CVAE) (). In the case of C. arietinum, the germination percentage remained quite good (around 70–80%) at all concentrations (2%, 4%, 6%, 8%, and 10%) of the extract,
while for T. aestivum, the germination percentage was high (around 80–100%) at 2% concentration but decreased as the extract concentration increased. It declined to 20–70% at 4% and 6% and further reduced to 0–60% at 8% and 0–10% at 10% concentrations (). The radicle and plumule lengths of C. arietinum and T. aestivum were similar to the control group after exposure to 2% and 4% concentrations of C. viminalis extracts. However, at 6%, 8%, and 10% concentrations, the radicle and plumule lengths were notably reduced, indicating a slowdown in plant growth at higher concentrations, which could have implications for crop yield. The findings of this study revealed that the apparent allelopathic action of CVAE significantly influenced the germination inhibition percentage (GIP) of C. arietinum and T. aestivum (p ≤ 0.01) (). Notably, the controls did not exhibit any GIP. The lowest inhibition was observed at 2% concentration, while the highest inhibition occurred at 6%, 8%, and 10% concentrations (Figure S1).
Table 2. Number of germinated seeds, germination percentage, germination inhibition percentage, radicle and plumule length in two important crop plants (Cicer arietinum and Triticum aestivum) under control and treated situation. In treated condition different concentrations of Callistemon viminalis aqueous extract (CVAE) were used at different temperatures in the petri-dish experiment.
3.2. Cash crop growth and their physiological parameters
The shoot height of C. arietinum ranged from 19.17 cm to 19.63 cm, including the control, while T. aestivum ranged from 14.33 cm to 14.84 cm, including the control. The total plant dry mass ranged from 0.8 gm to 1.4 gm for both crops, including the control, and these morphological growth parameters were not significantly affected (p ≤ 0.05) by the application of the extract (). Chlorophyll a (Chl a) levels showed no significant difference between the control and CVAE treatments. Similarly, the interaction of CVAE treatments on Chlorophyll b (Chl b) levels exhibited no significant difference between all concentration treatments and the control levels. Chlorophyll is essential for light energy absorption and conversion, and its concentration directly impacts the plant's use of light energy and its ability to cope with various stresses. Photosynthetic rate (Pn), stomatal conductance (gs), and transpiration rate (E) also displayed similar trends (). Fv/Fm is a sensitive indicator of plant photosynthetic performance, but lower values can also indicate stress, photoinhibition, or photosynthesis downregulation. Plant chlorophyll fluorescence has been used to predict environmental and temperature stress (Maxwell and Johnson Citation2000). When the stress increases for the plants, the reaction centers close themselves; hence, the Fv/Fm ratio decreases. The Fv/Fm ratio showed no statistically significant difference between control and CVAE-treated crop plants (). Proline, an organic acid aiding protein biosynthesis, helps plants tolerate environmental stresses. However, no significant effect of CVAE treatments on proline content in leaves was observed for either cash crop at lower concentrations (), indicating that the plants were not under stress.
Figure 5. Inhibition percentage of Weed plants after the treatment of Callistemon viminalis aqueous extract.
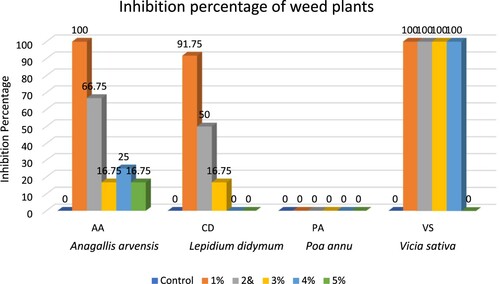
Table 3. Shoot height, dry mass, photosynthetic pigments (Chlorophyll a, b, total chlorophyll, and carotenoids), net photosynthetic rate (Pn), stomatal conductance (gs), transpiration rate (E), and chlorophyll fluorescence (Fv/Fm) in two important crop plants (Cicer arietinum and Triticum aestivum) under control and treated situation. Values followed by the same letter indicate no significant differences at P < 0.05 level according to Tukey’s test. Each value represents the mean of five replicates.
Table 4. Content of proline, and soluble protein in two important crop plants (Cicer arietinum and Triticum aestivum) under control and treated situation. Values followed by the same letter indicate no significant differences at P < 0.05 level according to Tukey’s test. Each value represents the mean of five replicates.
Antioxidant systems in plants protect against cytotoxic effects caused by environmental stresses. Superoxide dismutase (SOD), catalase (CAT), and peroxidase (PER) are critical enzymes involved in the antioxidant protective capacity of plants against free radical attacks. No statistically significant difference in SOD activity was found between control and CVAE-treated plants (). Likewise, the means comparison revealed no significant difference in PER and CAT activity between all CVAE concentrations and the controls (). High extract concentrations reduced the activities of CAT, PER, and SOD in receptor plants, while low concentrations stimulated them. Low concentrations of allelochemicals are likely to enhance the ability of receptor plants to resist oxidative stress by activating their antioxidant enzymes and mitigating the impact of reactive oxygen species (ROS) (Liu et al. Citation2017).
Table 5. Antioxidant enzymes activities in two important crop plants (Cicer arietinum and Triticum aestivum) under control and treated situation. Values followed by the same letter indicate no significant differences at P < 0.05 level according to Tukey’s test. Each value represents the mean of five replicates.
3.3. Number of weed plants died
After fifteen days, the first dose did not affect the A. arvensis plants, which were green, fresh, and healthy at all aqueous extract concentrations. Following the first treatment dose, all weed plants followed the same pattern. The average plant height of A. arvensis was between 14.70–15.27 cm (compared to the control plant height of 15.00 cm), 0.73 cm to 1.27 cm (control 1.63 cm) for L. didymium, 7.87 cm to 9.43 cm including the control for P. annua, and 9.70 cm to 10.17 cm for V. sativa (compared to the control 10.27 cm).
After seven days of the second dose, 91.75% and 66.75% of plants of A. arvensis died at 1% and 2% concentrations, respectively, while there was no impact on 3%, 4%, and 5%. After a fortnight of the second dose, all the plants died at 1% treatment, 66.75% died at 2%, and the leaves turned brownish yellow, and flowers and a few plants drooped at 3%, 4%, and 5% concentrations. All the plants of control were green and healthy with flowers. After a week of the second treatment dose, the plants of L. didymium started drooping at 1% and 2%, with no impact on 3%, 4% and 5%. In contrast, after fifteen days, approximately 75% and 50% of plants died at 1% and 2% treatment, respectively, and a few plants at 3% with no impact of dose two as well on 4% and 5%, whereas all of the plants in control were green and healthy. In the case of P. annua, the mean heights ranged from 9.40–10.30 cm (including the control), and budding started in all of the plants after the second dose of treatment. None of the plants drooped or died, indicating that the second dose had no visual effect. After a week of the second dose of treatment, the plants of Vicia sativa started drooping at 1%, 2%, 3% and 4% concentrations. In comparison, there was no significant impact seen at 5% concentration, while after a fortnight, all the plants died at 1%, 2%, 3% and 4%, while there was no impact at 5% concentration. In the control assay, the plants were green and healthy. Hence, the inhibition percentage (IP) is zero in the control conditions and at all concentrations of P. annua. The IP of A. arvensis was 100% at 1% treatment, 66.75% at 2%, and too low at 3%, 4%, and 5%. In the case of L. didymium, the IP was 91.75% at 1%, 50% at 2%, while very low at 3%, and zero at 4% and 5% treatments. Whereas in V. sativa, the IP was 100% at 1%, 2%, 3%, and 4%, with little impact at 5% treatment.
3.4. HPTLC analysis of allelopathic phytochemicals
HPTLC analysis of the extracts revealed the presence of benzoic acid (4.08%), kaempferol (2.64%), and quercetin (3.90%) compounds in the extract (). As the compounds were reported for their allelopathic activity against various plant species in different forms. In parallel, Kaempferol was found as allelochemical in Solidago canadensis (Li et al. Citation2011), while the allelopathic activity of benzoic acid was also reported by Blum et al. (Citation1991), Ben-Hammouda et al. (Citation1995) and (Sun et al. Citation2010). Quercetin was found responsible for significantly inhibiting the seed germination and seedling length of alfalfa (Ghimire et al. Citation2019).
3.5. Physio-chemical parameters of soil
Soil pH measures the soil's acidity or alkalinity and a ‘negative logarithm’ of hydrogen ion activity in the soil-water cycle. It is an essential component of nutrient availability. The pH of ordinary soil ranges from 3.5–9, in precipitated areas from 5 to 7, and in dry regions from 6.5–9. As a result, among all micronutrients, urea, phosphate, and potassium fertilizers are primarily applied to the soil, which is also pH-dependent (Husnain et al. Citation2021). The pH of the soil ranged from 7.27–7.37 in A. arvensis pots, 7.20–7.33 in L. didymium pots, 7.20–7.40 in P. annua pots, and V. sativa pots (Figure S2a), which was good for plant growth. Moisture availability in soil is critical for various biophysical processes such as seed germination, plant growth, nutrient cycling, and maintaining natural biodiversity in the soil. Soil moisture is also an essential variable in agricultural monitoring because it provides key insights into crop water availability, soil health, and moisture retention, both of which are important indicators of a sustainable agroecosystem (Kashyap et al. Citation2021). The moisture content in the soil of A. arvensis pots was 16.87%–17.07%, 17.03%–17.17% in the soil of L. didymium pots, 16.87%–17.10% in the soil of P. annua pots, and 16.90%–17.17% in the soil of Vicia sativa pots (Figure S2b). The water holding capacity ranged from 46.28–46.41 in A. arvensis pots, 47.20–47.37 in L. didymium pots, 47.27–47.45 in P. annua pots, and 46.33–46.43 in V. sativa pots () (Figure S2c). The pH of control was recorded as 7.21 for A. arvensis, 7.26 for L. didymium, 7.24 for P. annua, and 7.20 for V. sativa. In comparison, moisture content and WHC of control were 17.10% and 46.41% for A. arvensis, 17.11% and 46.39% for L. didymium, 17.09% and 46.40% for P. annua and 17.12% and 46.43% for V. sativa. All of the parameters were within KVK's standard range. Statistical analysis reveals no significant difference between all treatments and the control.
Table 6. The impact of Callistemon viminalis extract on physical parameters of Loam soil.
Soil organic carbon is a critical component of soil functioning and a significant determinant of the soil's ability to hold and release water and other nutrients required for plant and root system growth and thus represents the essence of all life (Ramesh et al. Citation2019). Total organic carbon and organic matter were 0.51%–0.52% (Figure S2d) and 0.88%–0.89% (Figure S2e) in all pots, respectively ().
Table 7. The impact of Callistemon viminalis extract on chemical parameters of soil.
Nitrogen is primarily obtained from pre-crops, green manures, and organic fertilization. On the other hand, plant phosphorus and potassium supply are dominated by soil processes and soil reserves (Løes and Øgaard Citation2001). In the A. arvensis pots, total nitrogen ranges from 319.87–340.78 kg/ha, 321.96–336.60 kg/ha in pots of L. didymium, 321.96–344.96 kg/ha in P. annua pots and 321.96–342.87 kg/ha in V. sativa pots (Figure S2f). The available phosphorus ranges from 42.75–46.67 kg/ha in A. arvensis pots, 42.75–46.85 kg/ha in L. didymium pots, 43.87–46.67 kg/ha in P. annua pots and 44.05–47.04 kg/ha in V. sativa pots (Figure S2 g). The potassium content was 171.77–172.29 kg/ha in A. arvensis pots, 171.96–172.78 kg/ha in L. didymium pots, 171.62–171.99 kg/ha in P. annua pots, and 171.85–172.70 kg/ha in V. sativa pots (Figure S2 h). However, omitting phosphorus supply affects soil phosphorus levels, and low soil phosphorus, potassium, and sulfur availability also limit legumes’ ability to fix atmospheric nitrogen. Because the availability of P, K, and S are significant drivers of overall nitrogen inputs via biological nitrogen fixation into organic farming systems, ensuring an adequate supply of these nutrients is critical to sustaining the system's overall productivity (Cooper et al. Citation2018). The calcium and magnesium were recorded in the range of 6.41–6.57 and 2.24–2.96 kg/ha in pots of A. arvensis, 5.99–6.55 kg/ha, and 2.26–2.96 kg/ha in pots of L. didymium, 6.24–6.53 kg/ha and 2.41–2.91 kg/ha in pots of P. annua, and 6.34–6.53 kg/ha (Figure S2i) and 2.17–2.91 kg/ha (Figure S2j) in pots of V. sativa. Calcium and magnesium are replenished through mineral dissolution and surface exchange reactions. Nutrient mobility in soil influences ion transport to plant roots, nutrient availability evaluation, and, ultimately, nutrient management decisions. The ability of native soils to buffer nutrient loss due to crop removal determines native soil nutrient supply (Havlin and Heiniger Citation2020). All the parameters fell within the standard range of KVK. According to statistical analysis, there was no significant difference between all treatments and the control.
One of the major challenges in organic farming is nutrient availability. Little is known about organic farm nutrient budgets, underlying factors, or effects on soil fertility. While nitrogen can be supplied through biological nitrogen fixation, all other nutrients must be replenished through external inputs to replace off-takes, at least in the long run, to avoid system degradation. The organic farming pioneers’ original concept of soil fertility management included efficiently recycling nutrients within farms through re-distribution and application of animal manure (Reimer et al. Citation2020). A physicochemical study of soil by Dandwate (Citation2020) also supported the results with a pH range from 7.60–8.81 and organic carbon from 0.52–0.72%. Among the nutrients, Nitrogen is from 140.01–252.68 kg/ha, Phosphorous from 15.11–54.13 kg/ha, and Potassium from 125.31–630.15 kg/ha.
4. Discussion
Seed germination is the fundamental process in plant growth, directly influencing the survival and development of the plant. The seed germination test serves as one of the most widely used biological detection methods in allelopathy studies (Wang et al. Citation2019). Numerous studies have demonstrated that allelochemicals released from C. viminalis can significantly hinder seed germination and seedling growth (de Oliveira et al. Citation2014; Pélagie Michelin et al. Citation2016; Bali et al. Citation2017). In this present study, we investigated the allelopathic effects of C. viminalis extracts on the seed germination and growth of cash crops, T. aestivum (wheat), and C. arietinum (chickpea), along with four common weeds, namely A. arvensis (Scarlet pimpernel), V. sativa (Common vetch), L. didymium (Swine cress), and P. annua (Meadow grass).
During the initial set of experiments, the germination indices of both cash crops were significantly inhibited at higher concentrations of the extract. The results revealed that increasing the leaf extract concentration delayed germination and reduced the final germination percentage, which was consistent with a previous study on the allelopathic impact of walnut leaf extract on wheat (T. aestivum cv. VL 719) seed germination and seedling growth (Prasad et al. Citation2020). At 20°C, wheat seeds were germinated in Petri dishes, and the application of walnut leaf extract led to a concentration-dependent reduction in seed germination and seedling growth. Interestingly, many studies have reported a phenomenon of a promotional effect at low concentrations and an inhibitory effect at high concentrations (Wang et al. Citation2018).
The growth and development of the radicle directly affect plant growth, while plumule growth reflects the plant's growth rate at the seedling stage. In this study, both radicle and plumule lengths displayed a decreasing trend with increasing concentrations of aqueous extracts. Similarly, Oraon and Mondal (Citation2022) observed that 5% aqueous leaf extracts had the most significant inhibitory effect on seed germination, root length, and shoot length compared to the control. This suggests that increasing aqueous extract concentrations intensified the inhibitory effect. Allelochemicals can be classified into several categories based on their structures and properties. In our study, we identified the presence of three types of allelochemicals, benzoic acid, kaempferol, and quercetin, in water extracts, which have previously been reported for their allelopathic activity by many researchers in various plant extracts. The HPTLC results enhance our understanding of the allelopathic potential of C. viminalis and provide scientific evidence for its application as a natural herbicide in sustainable weed management. The presence of allelopathic compounds like benzoic acid (4.08%), kaempferol (2.64%), and quercetin (3.90%) further supports the notion that harnessing the power of allelochemicals can offer an environmentally friendly and effective approach to weed control, reducing the reliance on synthetic herbicides and promoting more sustainable agricultural practices. A previous study indicated that reduced germination was significantly associated with increased membrane damage (Bogatek et al. Citation2006). In this study, phenolic compounds increased cell membrane lipid peroxidation and damaged the cell membrane to the greatest extent. Based on these results, it is concluded that phenols may be the primary cause of the observed inhibitory effect of C. viminalis.
Conclusion
India and several other countries actively cultivate wheat and chickpea due to their ability to thrive with less water compared to other comparable crops. Moreover, these crops serve as essential ingredients in a wide range of processed foods highly valued in modern urban life. However, weed infestations in crops can significantly impact crop production by competing for essential resources such as moisture, nutrients, space, and light, as well as providing shelter to harmful insect pests, resulting in yield loss. Weeds reduce yield by up to 15–50% depending on weed density and flora type (Adeux et al. Citation2019). This study revealed that 1% and 2% concentrations of C. viminallis aqueous extract are lethal for A. arvensis and V. sativa without affecting the germination, growth, photosynthetic, and biochemical properties of cash crops (C. arietinum and T. aestivum), and physio-chemical soil properties. It confirms that the C. viminallis extract works in a species-specific manner, and crops are more insistent than weeds. Hence the weed/crop interaction complexities of C. viminallis can be used as a bioherbicide for managing weeds. By selectively targeting weed species while preserving the health and growth of cash crops, this natural bioherbicide offers a sustainable and environmentally friendly approach to weed management in wheat and chickpea cultivation. Further research and field trials will be essential to fully understand its efficacy and practical application in weed control practices.
Author Contributions
Conceptualization: Deepti Singh Vashishth; Data curation: Deepti Singh Vashishth; Formal analysis: Deepti Singh Vashishth; Investigation: Deepti Singh Vashishth and Archana Bachheti; Methodology: Deepti Singh Vashishth, Archana Bachheti and Rakesh Kumar Bachheti; Resources: Archana Bachheti and Rakesh Kumar Bachheti; Software: Deepti Singh Vashishth and Archana Bachheti; Supervision: Archana Bachheti and Rakesh Kumar Bachheti; Validation: Archana Bachheti and Rakesh Kumar Bachheti; Visualization: Deepti Singh Vashishth, Archana Bachheti and Azamal Husen; Writing: Deepti Singh Vashishth and Archana Bachheti; review & editing: Archana Bachheti, Rakesh Kumar Bachheti and Azamal Husen; All authors have read and agreed to the published version of the manuscript.
Availability of data and material
The data that support the findings of this study are available on request from the corresponding author. The data are not publicly available due to privacy or ethical restrictions.
Supplemental Material
Download MS Word (505.3 KB)Acknowledgments
The infrastructural support provided by the Head of the Department of Environmental Science, Graphic Era (Deemed to be University), Dehradun, India is gratefully acknowledged. The author wishes to thank Manoj Singh for the support.
Disclosure statement
No potential conflict of interest was reported by the author(s).
Additional information
Notes on contributors
Deepti Singh Vashishth
Deepti Singh Vashishth is an aspiring scholar currently pursuing her Ph.D. at Graphic Era Deemed to be University since June 2019. She holds a Master of Science degree in Environmental Sciences, which she earned from MJPRU in 2017. Her academic journey commenced with a Bachelor of Science in Biology from MJPRU in 2015, setting the foundation for her research pursuits. Her research focus revolves around the intriguing realms of weed management, biocontrol, and phytochemistry. Her dedication to these fields is evident through her impressive track record, boasting a total of 15 publications and a multitude of presentations at both national and international conferences.
Archana Bachheti
Dr. Archana Bachheti is a prominent and accomplished researcher who has embarked on a remarkable academic journey spanning over 18 years. Her scholarly focus encompasses a range of subjects including eco-restoration, wasteland development, ecology, sustainable agriculture, and biodiversity. This is evident through her substantial body of work, comprising approximately 85 publications and numerous presentations at conferences. Dr. Archana Bachheti's efforts and impact extend beyond her field, as she has earned acknowledgement and esteem from fellow researchers and associates. Her achievements underscore her resolute dedication to academic distinction and her unyielding quest for the progression of knowledge.
Rakesh Kumar Bachheti
Dr. Rakesh Kumar Bachheti is a distinguished researcher with a prolific academic journey, encompassing 20 years of research and teaching experience. His research expertise spans across natural products, nanoparticles, and pulp and paper technology, evident through his 110 publications and extensive conference presentations. He has effectively mentored 40 MSc and 5 Ph.D. students, while also making substantial contributions to curriculum development and editorial work. Dr. Bachheti's dedication to advancing knowledge, promoting sustainable technology, and fostering interdisciplinary collaboration establishes him as a valuable asset within academia and the scientific community.
Azamal Husen
Azamal Husen served as Professor and Head of the Department of Biology, University of Gondar, Ethiopia, and is a Foreign Delegate at Wolaita Sodo University, Wolaita, Ethiopia. Previously, he was a Visiting Faculty of the Forest Research Institute, and the Doon College of Agriculture and Forest at Dehra Dun, India. Husen's research and teaching experience of 20 years encompasses the biogenic nanomaterial fabrication and application, plant responses to nanomaterials, plant adaptation to harsh environments at the physiological, biochemical, and molecular levels, herbal medicine, and clonal propagation for improvement of tree species. He has conducted research sponsored by the World Bank, the National Agricultural Technology Project, the Indian Council of Agriculture Research, the Indian Council of Forest Research Education, and the Japan Bank for International Cooperation. Husen has published extensively (< 250), and served on the Editorial Board and as reviewer of reputed journals. He is also working as Series Editor of ‘Exploring Medicinal Plants', published by Taylor & Francis Group, USA; ‘Plant Biology, Sustainability, and Climate Change', published by Elsevier, USA; ‘Smart Nanomaterials Technology’, published by Springer Nature Singapore Pte Ltd. Singapore; and is Editor-in-Chief of the American Journal of Plant Physiology.
References
- Adeux G, Munier-Jolain N, Meunier D, Farcy P, Carlesi S, Barberi P, Cordeau S. 2019. Diversified grain-based cropping systems provide long-term weed control while limiting herbicide use and yield losses. Agron Sustain Dev. 39:1–13. doi:10.1007/s13593-019-0587-x.
- Agbeshie AA, Adjei R, Anokye J, Banunle A. 2020. Municipal waste dumpsite: impact on soil properties and heavy metal concentrations, Sunyani, Ghana. Sci Afr. 8:e00390. doi:10.1016/j.sciaf.2020.e00390.
- Ahmad K, Athar F. 2017. Phytochemistry and pharmacology of Callistemon viminalis (Myrtaceae): a review. Nat Prod J. 7(3):166–175. doi:10.2174/2210315507666161216100323.
- Arnon DI. 1949. Copper enzymes in isolated chloroplasts. Polyphenol-oxidase in Beta vulgaris. Plant Physiol. 24(1):1–15. doi:10.1104/pp.24.1.1.
- Ashmawy NA, Behiry SI, Ali HM, Salem MZ. 2014. Evaluation of Tecoma stans and Callistemon viminalis extracts against potato soft rot bacteria in vitro. J Pure Appl Microbio. 8:667–673.
- Badawy ME, Abdelgaleil SA. 2014. Composition and antimicrobial activity of essential oils isolated from Egyptian plants against plant pathogenic bacteria and fungi. Ind Crops Prod. 52:776–782. doi:10.1016/j.indcrop.2013.12.003.
- Bali AS, Batish DR, Singh HP, Kaur S, Kohli RK. 2017. Phytotoxicity and weed management potential of leaf extracts of Callistemon viminalis against the weeds of rice. Acta Physiol Plant. 39(1):1–9. doi:10.1007/s11738-016-2300-x.
- Bates LS, Waldren RP, Teare ID. 1973. Rapid determination of free proline for water stress studies. Plant Soil. 39:205–207. doi:10.1007/BF00018060.
- Batish DR, Lavanya K, Singh HP, Kohli RK. 2007. Phenolic allelochemicals released by Chenopodium murale affect the growth, nodulation and macromolecule content in chickpea and pea. Plant Growth Regul. 51:119–128. doi:10.1007/s10725-006-9153-z.
- Ben-Hammouda M, Kremer RJ, Minor HC, Sarwar M. 1995. A chemical basis for the differential allelopathic potential of sorghum hybrids on wheat. J Chem Ecol. 21:775–786. doi:10.1007/BF02033460.
- Blum U, Wentworth TR, Klein K, Worsham AD, King LD, Gerig TM, Lyu SW. 1991. Phenolic acid content of soils from wheat-no till, wheat-conventional till, and fallow-conventional till soybean cropping systems. J Chem Ecol. 17:1045–1068. doi:10.1007/BF01402933.
- Bogatek R, Gniazdowska A, Zakrzewska W, Oracz K, Gawronski SW. 2006. Allelopathic effects of sunflower extracts on mustard seed germination and seedling growth. Biol Plantarum. 50(1):156–158. doi:10.1007/s10535-005-0094-6.
- Chandlee JM, Scandalios JG. 1984. Analysis of variants affecting the catalase development program in maize scutellum. Theor Appl Genet. 69:71–77. doi:10.1007/BF00262543.
- Cheema ZA, Farooq M, Khaliq A. 2013. Application of allelopathy in crop production: success story from Pakistan. Allelopathy: Curr Trends Future Appl. 113–143. doi:10.1007/978-3-642-30595-5_6.
- Cooper J, Reed EY, Hörtenhuber S, Lindenthal T, Løes AK, Mäder P, Magid J, Oberson A, Kolbe H, Möller K. 2018. Phosphorus availability on many organically managed farms in Europe. Nutrient Cycling Agroecosystems. 110:227–239. doi:10.1007/s10705-017-9894-2.
- Dandwate SC. 2020. Analysis of soil samples for its physicochemical parameters from Sangamner city. GSC Biol Pharma Sci. 12(2):123–128. doi:10.30574/gscbps.2020.12.2.0243.
- de Oliveira CM, das Graças Cardoso M, da Silva Figueiredo AC, de Carvalho MLM, de Miranda CASF, Albuquerque LRM, Brandão RM. 2014. Chemical composition and allelopathic activity of the essential oil from Callistemon viminalis (myrtaceae) blossoms on lettuce (Lactuca sativa L.) seedlings. Am J Plant Sci. 5(24):3551. doi:10.4236/ajps.2014.524371.
- Dhindsa RA, Dhindsa PP, Thorpe TA. 1981. Leaf senescence: correlated with increased permeability and lipid peroxidation, and decreased levels of superoxide dismutase and catalase. J Exp Bot. 126:93–101. doi:10.1093/jxb/32.1.93.
- Doruk K, Venkatakrishnan D. 2022. Evaluation of nutritional quality attributes and their inter relationship in maize. J Pharmacogn Phytochem. 11(2):296–297.
- Estefan G, Sommer R, Ryan J. 2013. Methods of soil, plant, and water analysis. Manual West Asia North Africa Reg. 3:65–119.
- Genty B, Briantais JM, Baker NR. 1989. The relationship between the quantum yield of photosynthetic electron transport and quenching of chlorophyll fluorescence. Biochim Biophys Acta. 990(1):87–92.
- Ghimire BK, Ghimire B, Yu CY, Chung IM. 2019. Allelopathic and auto-toxic effects of Medicago sativa—Derived allelochemicals. Plants. 8(7):233. doi:10.3390/plants8070233.
- Goyal K, Singh N, Jindal S, Kaur R, Goyal A, Awasthi R. 2022. Kjeldahl method. Advan Tech Anal Chem. 1(1):105. doi:10.2174/9789815050233122010011.
- Havlin J, Heiniger R. 2020. Soil fertility management for better crop production. Agronomy. 10(9):1349. doi:10.3390/agronomy10091349.
- Hosseinzadeh SR, Amiri H, Ismaili A. 2017. Nutrition and biochemical responses of chickpea (Cicer arietinum L.) to vermicompost fertilizer and water deficit stress. J Plant Nutr. 40:2259–2268. doi:10.1080/01904167.2016.1262412.
- Husen A. 2008. Clonal propagation of Dalbergia sissoo Roxb. and associated metabolic changes during adventitious root primordium development. New For. 36:13–27. doi:10.1007/s11056-007-9079-y.
- Husen A. 2010. Growth characteristics, physiological and metabolic responses of teak (Tectona grandis Linn. f.) clones differing in rejuvenation capacity subjected to drought stress. Silvae Genet. 59(1-6):124–136. doi:10.1515/sg-2010-0015.
- Husnain A, Farooq Q, Sohail S, Shasang S, Toor D, Zafar A, Rehman B. 2021. Adaptability of soil pH through innovative microbial approach. Curr Res Agr Sci. 8(2):71–79. doi:10.18488/journal.68.2021.82.71.79.
- Kashyap T, Athokpam HS, Devi NS, Venu N. 2021. Forms and status of phosphorus in acid soils of Kakching district, Manipur (India). Pharma Inno J. 10(9):407–410.
- Lalrammawia K, Buragohain A, Kakki B, Zote L, Marak NK, Lalmuanpuii R, Kumar NS, Jahau L, Sudarshan M, Chaligava O, Yushin N. 2022. Determination of multi elements in tobacco plant of northeast India by neutron activation analysis and atomic absorption spectrometry. Biol Trace Elem Res. 200(10):4534–4549. doi:10.1007/s12011-021-03040-2.
- Li J, Chen L, Chen Q, Miao Y, Peng Z, Huang B, Guo L, Liu D, Du H. 2021. Allelopathic effect of Artemisia argyi on the germination and growth of various weeds. Scientifuc Rep. 11(1):1–15. doi:10.1038/s41598-020-79139-8.
- Li J, Ye Y, Huang H, Dong L. 2011. Kaempferol-3-O-β-D-glucoside, a potential allelochemical isolated from Solidago Canadensis. Allelopath J. 28:259–266.
- Li ZH, Wang Q, Ruan X, Pan CD, Jiang DA. 2010. Phenolics and plant allelopathy. Molecules. 15:8933–8952. doi:10.3390/molecules15128933.
- Liu J, Li D, Wang D, Liu Y, Song H. 2017. Allelopathic effects, physiological responses and phenolic compounds in litter extracts of Juniperus rigida. Chem Biodiversity. 14:e1700088. doi:10.1002/cbdv.201700088.
- Løes AK, Øgaard AF. 2001. Long-term changes in extractable soil phosphorus (P) in organic dairy farming systems. Plant Soil. 237:321–332. doi:10.1023/A:1013328228904.
- Lopes AD, Nunes MGIF, Francisco JP, dos Santos EH. 2022. Potential allelopathic effect of species of the asteraceae family and its use in agriculture in vegetation dynamics, changing ecosystems and human responsibility.
- Lowry OH, Rosebrough NJ, Farr AL, Randapp RJ. 1951. Protein measurement with the folin phenol reagent. J Biol Chem. 191:265–275. doi:10.1016/S0021-9258(19)52451-6.
- Maxwell K, Johnson GN. 2000. Chlorophyll fluorescence––A practical guide. J Exp Bot. 51:659–668. doi:10.1093/jexbot/51.345.659.
- McDowell RW, Condron LM. 2004. Estimating phosphorus loss from New Zealand grassland soils. N Z J Agric Res. 47(2):137–145. doi:10.1080/00288233.2004.9513581.
- Ndomo AF, Tapondjou LA, Ngamo LT, Hance T. 2010. Insecticidal activities of essential oil of callistemon viminalis applied as fumigant and powder against two bruchids. J Appl Entomol. 134(4):333–341. doi:10.1111/j.1439-0418.2009.01475.x.
- Oraon S, Mondal S. 2022. Allelopathic impacts of an agroforestry tree species (Streblus asper lour.) on seed germination and seedling growth of chickpea. Legume Res Int J. 45(10):1295–1300.
- Ou-Zine M, Symanczik S, Rachidi F, Fagroud M, Aziz L, Abidar A, Mäder P, Achbani EH, Haggoud A, Abdellaoui M, Bouamri R. 2021. Effect of organic amendment on soil fertility, mineral nutrition, and yield of majhoul date palm cultivar in Drâa-tafilalet region, Morocco. J Soil Sci Plant Nutr. 21(2):1745–1758. doi:10.1007/s42729-021-00476-2.
- Oyedeji OO, Lawal OA, Shode FO, Oyedeji AO. 2009. Chemical composition and antibacterial activity of the essential oils of Callistemon citrinus and Callistemon viminalis from South Africa. Molecules. 14:1990–1998. doi:10.3390/molecules14061990.
- Pélagie Michelin KT, Jean AN, Donatien G, Paul Keilah L, Stephen LT, Jules-Roger K. 2016. In vitro allelopathic effects of extracts and fractions of five plants on tomato seed germination and vigor index. Cogent Biol. 2(1):1220661. doi:10.1080/23312025.2016.1220661.
- Prasad B, Lavania SK, Sah VK. 2020. Allelopathic effects of walnut leaf extract on seed germination and seedling growth of wheat (Triticum aestivum L.). Indian J Agroforest. 13(2):87–90.
- Prochazkova D, Sairam RK, Srivastava GC, Singh DV. 2001. Oxidative stress and antioxidant activity as the basis of senescence in maize leaves. Plant Sci. 161:765–771. doi:10.1016/S0168-9452(01)00462-9.
- Ramesh T, Bolan NS, Kirkham MB, Wijesekara H, Kanchikerimath M, Rao CS, Sandeep S, Rinklebe J, Ok YS, Choudhury BU, Wang H. 2019. Soil organic carbon dynamics: impact of land use changes and management practices: a review. Adv Agron. 156:1–107. doi:10.1016/bs.agron.2019.02.001.
- Reimer M, Hartmann TE, Oelofse M, Magid J, Bünemann EK, Möller K. 2020. Reliance on biological nitrogen fixation depletes soil phosphorus and potassium reserves. Nutr Cycling Agroecosyst. 118(3):273–291. doi:10.1007/s10705-020-10101-w.
- Shivarkar R, Bhise S, Gupta RM. 2018. HPTLC fingerprinting and antimicrobial activity of Azadirachta indica leaf extracts. Int j biomed adv res. 9(6):235–240.
- Silva CJ, Barbosa LCA, Pinheiro AL, Andrade NJ. 2010. Chemical composition and antibacterial activities from the essential oils of Myrtaceae species planted in Brazil. Química Nova'. 33:104–108. doi:10.1590/S0100-40422010000100019.
- Sodaeizadeh H, Rafieiolhossaini M, Havlı ´k J, Van Damme P. 2009. Allelopathic activity of different plant parts of Peganum harmala L. and identification of their growth inhibitors sub-stances. Plant Growth Regul. 59:227–236. doi:10.1007/s10725-009-9408-6.
- Somnuek S, Jaenaksorn T, Laosinwattana C. 2020. Effect of crude ethanolic extracts from bottle brush (Callistemon viminalis) against leaf spot fungi and their phytotoxicity on lettuce (Lactuca sativa L.). Curr Appl Sci Technol. 20(1):1–14.
- Sun YY, Liu XX, Yan BL, Wang CH. 2010. Effects of p-hydroxybenzoic acid on the growth of two species of red tide microalgae. Environ Sci Technol. 33(7):36–39.
- Uwaya GE, Fayemi OE, Sherif ESM, Junaedi H, Ebenso EE. 2020. Synthesis, electrochemical studies, and antimicrobial properties of Fe3O4 nanoparticles from Callistemon viminalis plant extracts. Materials. 13(21):4894. doi:10.3390/ma13214894.
- Wang C, Wu B, Jiang K. 2019. Allelopathic effects of Canada goldenrod leaf extracts on the seed germination and seedling growth of lettuce reinforced under salt stress. Ecotoxicology. 28:103–116. doi:10.1007/s10646-018-2004-7.
- Wang X, Wang J, Zhang R, Huang Y, Feng S, Ma X, Roy R. 2018. Allelopathic effects of aqueous leaf extracts from four shrub species on seed germination and initial growth of Amygdalus pedunculata Pall. Forests. 9(11):711. doi:10.3390/f9110711.
- Zain NM, Yew OH, Sahid I, Seng C. 2013. Potential of napier grass (Pennisetum purpureum) extracts as a natural herbicide. Pak J Bot. 45(6):2095–2100.