ABSTRACT
Background
Given the range of subjective experiences reported by patients with chronic pain, Spinal Cord Stimulation (SCS) systems designed for tailored delivery of analgesic therapy may help improve treatment effectiveness and satisfaction.
Research design and methods
This case-series evaluated 420 patients with chronic back and/or leg pain implanted with an SCS device capable of sequential or simultaneous delivery of neurostimulation (i.e. combination therapy) as well as multiple waveforms and/or field shapes. Following implantation, an array of standard programs (e.g. paresthesia-based SCS), and a custom set of sub-perception programs were provided per patient feedback. Pain scores (Numeric Rating Scale, NRS) were collected at baseline and during follow-up.
Results
In this multicenter, observational series (n = 420, 53.1% female; Age: 64.2 ± 13.4 years), a mean overall pain score of 7.2 ± 1.8 (SD) was reported pre-trial (Baseline). At a mean follow-up duration of 208 ± 200 (SD) days, the mean overall pain score reduced to 2.4 (p < 0.0001). Overall pain was reduced by 5.1 ± 2.4 and 4.5 ± 2.4 points (NRS) at 3-months (N = 256) and at 12-months post-implant (N = 122) respectively (p < 0.0001).
Conclusions
These results suggest that highly ‘customizable’ SCS approaches may allow for highly effective pain relief within the real-world clinical setting.
1. Introduction
A growing body of evidence suggests that optimal pain relief achieved in one Spinal Cord Stimulation (SCS) patient using a specific paradigm of stimulation may fail to render the same outcome in another patient with the identical diagnosis and symptom complaint [Citation1,Citation2,Citation3,Citation6]. This includes the use of SCS at higher frequencies (e.g. 1.2–10 kHz) for chronic pain as strikingly different preferences and/or variable treatment satisfaction data have been reported by various studies [Citation1,Citation4–7]. Moreover, pain relief outcomes described by real-world, observational studies assessing 10 kHz stimulation have not consistently reproduced results that match those reported by a large, multicenter randomized controlled trial using 10 kHz [Citation7,Citation8,Citation9,Citation10,Citation11]. In addition, burst stimulation has also been reported to provide added benefit versus other conventional SCS approaches in implanted patients [Citation12,Citation13,Citation14]. However, as with studies investigating high-frequency SCS, varying levels of clinical success as measured by pain relief and/or patient preference/satisfaction have been reported across different studies including those assessing dissimilar patient sub-populations [Citation1,Citation5,Citation12–14]. The integration of various burst parameters (e.g. pulses per burst, intra-burst frequency, inter-burst frequency, pulse-width, others) underlying the clinical application of this waveform allows for optimization of device energy usage and possibly patient-to-patient variability [Citation15]. This aspect of the burst waveform thus serves to reinforce the need for systems engineered for flexible manipulation of neurostimulation programming to provide each patient with the opportunity to uncover the best mode of treatment for their own needs and preferences. Finally, a randomized controlled trial (RCT) using 1 kHz in de novo subjects demonstrated no difference in pain relief among four different rates (1, 4, 7, and 10 kHz) [Citation16]. An important learning from this study was that nearly a quarter of subjects obtained effective pain relief from only one stimulation mechanism – either paresthesia-based or paresthesia-free (i.e. sub-perception SCS) – demonstrating the anticipated need to provide implanted patients with multiple neurostimulation approaches. In sum, the highly varied nature of the chronic pain experience in the SCS-implanted population provides rationale for SCS devices engineered to provide a range of customizable waveforms as well as personalization of stimulation field targeting. In this regard, we now report here a multicenter, observational clinical assessment that set out to evaluate real-world pain relief outcomes in patients who used an SCS system designed to provide multiple waveforms that can be delivered in a simultaneous or sequential manner while also equipped with an algorithm enabling precise control of neurostimulation field shape.
2. Patients and methods
Data evaluated in this study were collected from 420 consecutive patients permanently implanted with an SCS system (Spectra WaveWriter, Boston Scientific, Marlborough, MA USA) to treat chronic pain at seven pain management centers in the United States. All patients previously underwent a trial implantation, but data collection was only limited to those who received a permanent implant (with a positive trial as determined by standard-of-care typically, 50% improvement in pain following a 3–7 day trial, compared with baseline, where leads were temporarily implanted to determine if a patient may be a responder to SCS). Institutional Review Board (IRB) approval was obtained from each site and the study was conducted in accordance with GCP (ISO14155) guidelines and the Declaration of Helsinki. IRB approval was obtained on 21 March 2012 (Reference Number: WIRB/20120371). Inclusion criteria consisted of the following: previously treated with or eligible for use with commercially approved SCS systems (Boston Scientific) and 18 years of age or older. There were no exclusion criteria per study protocol.
2.1. Device description
The SCS system utilized in this study (Spectra WaveWriter, Boston Scientific, Marlborough, MA USA) possesses the capability for anatomically guided neural targeting so as to deliver paresthesia-based SCS therapy using a three-dimensional finite element model of the spinal cord [Citation17]. Incorporating the location of implanted SCS leads, the algorithm calculates precisely how much current is needed at each contact to best target a user-specified central point of stimulation on the dorsal column. Additionally, the system enables delivery of multiple SCS waveforms, such that higher-frequency (i.e. up to 1200 Hz) and lower-frequency stimulation timing channels can be run in the same program (delivered in a simultaneous manner), thereby enabling higher frequency (i.e. up to 1200 Hz) sub-perception SCS and lower-frequency paresthesia SCS as a combination therapy. The system also uses a three-dimensional finite element model to place a constant electrical field (dV/dy) along the dorsal horn spanning one or more vertebral levels. A constant electrical field along the rostrocaudal plane of the spinal cord is hypothesized to selectively engage the synaptic terminals of rostrocaudally oriented inhibitory interneurons in the superficial dorsal horn [Citation18,Citation19]. Supporting this possibility are computational studies and experimental observations demonstrating that terminals may be preferentially polarized over axons of passage or cell bodies with near-field electrical stimulation using geometries designed to maximize the local electric field [Citation20,Citation21,Citation22,Citation23]. It is also possible to directly excite presynaptic inhibitory terminals that produce postsynaptic inhibition at amplitudes below the excitation threshold of the postsynaptic cell, as demonstrated with deep brain stimulation [Citation24]. The elongated bipole configuration was therefore designed to generate a broader electrical field in the rostrocaudal plane to increase the likelihood of engaging similarly aligned inhibitory interneurons and thereby reduce variability in the search for the optimal sub-perception sweet spot [Citation16]. Additionally, by employing tightly spaced contacts and multiple independent current control, multiple potential sub-perception targets can be captured with a contoured or shaped electric field specifically customized to the individual. Furthermore, the system includes the capability of running different waveforms and/or stimulation settings (e.g. amplitude, pulse-width, and frequency) that on the basis of known parameter relationships can be modulated to adjust the total amount of charge delivered to neural tissue per unit time (i.e. neural dosing) in an automated or sequentially scheduled manner (i.e. waveform automation). This capability provides for multiple programs to be automatically evaluated during the optimization process without conventional manual intervention by the physician, programmer, and/or patient, which can be beneficial when using current sub-perception waveforms due to the reported latency period of analgesic onset (typically a 1–3-day ‘wash-in’ or pain relief) [Citation16,Citation25,Citation26]. Finally, to facilitate identification of the most efficient and/or effective waveform, the patient controller allows the patient to enter time-stamped assessments of the quality of each program (1 to 5 stars), thereby allowing the physician to assess both the efficacy and efficiency of each provided waveform ().
Figure 1. Finite element models were used to estimate compare the voltage (a) and the first derivative of the voltage in the rostral-caudal direction (b) of a standard 8 mm Bipole and a Novel Field with elongated span, both at unit current (1 mA). The polarity assignments for each are shown in the two panels of a (left – standard Bipole; right – Novel Field with elongated span). Note that for polarity assignments, darker color denotes more cathodic or anodic current from the current sources. Consistent with the intended design, the elongated field (dV/dy) is more uniform and has longer span than the standard bipole (b) (spatial units in mm). The system is capable of combination programs, i.e. delivering multiple frequencies in the same program. In panel (c) an example of a program delivering 1000 Hz (pulses beneath pink bars) and 40 Hz (pulses demarcated by green diamonds) in combination. Blue arrowheads identify charge recovery phases for the 40 Hz pulses. The oscilloscope trace (c) shows the two frequencies delivered from a common electrode, although this is not required and the frequencies can be assigned to be delivered from independent fields within the program
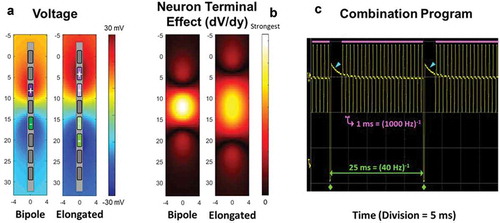
2.2. Data collection and analysis
This is a multicenter, consecutive, retrospective, observational case series that assesses pain relief outcomes in patients implanted with a commercially available SCS system for use in the treatment of chronic pain. This study is part of an ongoing assessment of real-world outcomes of SCS for chronic pain based on retrospective chart review (Clinicaltrials.gov identifier: NCT01550575). Stimulation parameters including waveform and electric field shapes were optimized based on physician and patient preferences as part of standard-of-care.
Due to the retrospective nature of this assessment, data reported were limited to only those patients who had completed follow-up at the time of the data snapshot. Hence, sample size varies across time, with fewer patients evaluated at later follow-up timepoints, as data could not be obtained from those who had not yet reached subsequent follow-up visits.
To minimize potential bias, data collection from patients was performed directly by clinical site personnel without any sponsor involvement. Demographic information, pain location, surgical history, medical history were collected for all subjects. Numeric Rating Scale (NRS) scores and Percent Pain relief (PPR) were collected as part of the chart review. Due to the nature of this evaluation, patient-specific device programming information and adverse events were not collected.
Mean, median, and standard deviations were calculated for demographic data and NRS scores. Score distribution was recorded for NRS and percent pain relief. A paired t-test with two-sided 0.05 significance level was used to determine whether the mean reduction in baseline pain was greater than 0.
3. Results
A total of 420 permanently implanted patients were analyzed in this study. Of those, the number of patients for which data were available at the completion of trial implantation, 3 months after permanent implant, and 12 months after permanent implant was 391, 256, and 122, respectively (). Among all patients with gender information available (n = 409), 217 of them were female. On average, patients were 64.2 ± 13.4 years of age (n = 417), with 67.9% reporting low back pain and 85.7% reporting low back and leg pain and were diagnosed with one or more of the following: Lumbosacral Radiculopathy 83.3% (350/420), Failed Back Surgery Syndrome 47.9% (201/420), and Osteoarthritis 44.5% (187/420). Baseline NRS scores were available for all patients, with a mean of 7.2 ± 1.8 (n = 420). The mean follow-up duration was found to be 208 ± 200 days (n = 420) ().
Table 1. Baseline and demographic characteristics in analyzed patients (n = 420)
In patients assessed at 3 and 12 months following implantation, a 5.1 ± 2.4-point improvement (n = 256), and a 4.5 ± 2.4 point improvement (n = 122) were noted, respectively (p < 0.0001) (). The varying sample size is due to the fact that some subjects had not reached these specified timepoints at the time of the data analysis. This level of pain reduction at these timepoints correlated with an 84% (3 months) and 80% (12 months) responder rate, respectively, as defined by the proportion of subjects with a ≥ 50% improvement in overall pain compared with Baseline using NRS scores. displays the distribution of achieved percent pain score reduction in patients who were assessed at 12 months post-implantation.
Figure 3. Mean overall NRS pain scores at baseline, end of trial, and 3 and 12 months post-implant. error bars denote standard error (SE). p < 0.0001. Median scores at baseline, end of trial, 3 and 12 months post-implant were 7.0, 2.0, 2.0, 2.0
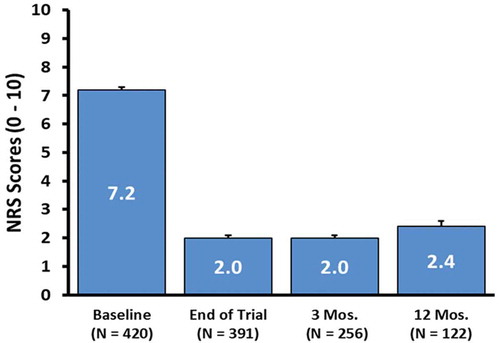
Figure 4. Distribution of percent NRS score reduction in evaluated patients at 12 months post-implantation (n = 122)
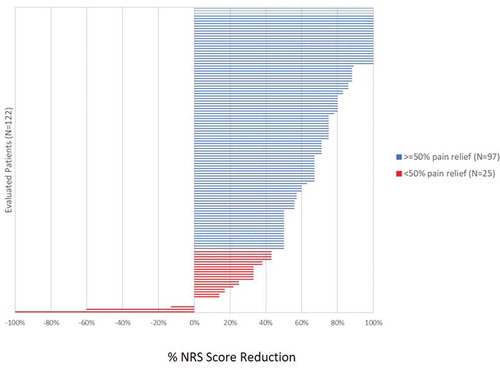
The distribution of mean overall pain at baseline trended toward higher NRS scores ≥7, thereby indicating that a majority of patients (>65%) reported severe chronic pain prior to SCS device implantation ()). Based on the distribution of mean NRS pain scores collected from all 420 evaluated patients at last follow-up, a 4.9 ± 2.5-point improvement in overall pain was observed (mean last follow-up = 208 days). Twenty-three percent (95 of 420) of all patients reported no pain, while 61% (256 of 420) reported NRS scores of 2 or less ()). These results were likewise reflected by Percent Pain Relief (PPR) data in which 41% (160 of 393) of all patients had 81–100% improvement and 89% (349 of 393) had greater than 50% improvement as compared with Baseline (PPR data for 27 patients was not available for analysis).
4. Discussion
This real-world, large, multicenter, observational study reported the outcomes of patients who used an SCS system capable of providing multiple modalities for the treatment of chronic low back/leg pain. Clinically significant reduction of pain on average was reported, with mean NRS scores decreasing over time (out to 1-year follow-up) and NRS scores at last follow-up orientated toward the lower end of the pain scale. These results suggest that SCS patients may benefit when provided with a single device that can deliver multiple waveform types, delivered in a sequential or simultaneous manner, and with capability for high precision targeting of neurostimulation fields.
The results of this study align with previous studies suggesting that the use of various stimulation paradigms among different patients may be necessary for achieving the most effective pain relief outcomes, even within a select population displaying the same or similar pain conditions or symptoms [Citation1–6]. For example, a crossover RCT (WHISPER) assessing ≤1.2 kHz sub-perception SCS versus supra-perception in previously implanted subjects recently provided evidence supporting the clinical benefit of sub-perceptive neurostimulation when provided as an option(s) for selective use, even in those who were implanted with and previously utilized tonic-based SCS for several years prior [Citation6]. Additionally, the SUNBURST RCT showed that 12-weeks of either burst or tonic stimulation reduced Visual Analog Scale scores versus Baseline, and that notable proportions of study participants preferred one paradigm over the other, though most preferred burst [Citation27]. Further, the SENZA study, although carried out without a crossover design, examined study subjects out to 2 years after implantation and found both responders (10 kHz SCS group: 76%; Standard SCS group: 49%) and non-responders in groups randomized to either 10 kHz SCS or standard, paresthesia-based SCS [Citation7]. Notably, a similar total proportion of randomized subjects were either very satisfied or satisfied with their assigned treatment at 2 years post-implant (10 kHz SCS group: 86.3%; Standard SCS group: 86%). However, it is not clear whether those who benefited from one treatment may have benefited more from the other or whether non-responders to one treatment may have responded to the other treatment, though interestingly, one published study has shown the possibility for this to be the case [Citation28].
Overall, the collective results reported here and from other clinical studies, such as those described above, provide ancillary support for the implications of other previous investigations demonstrating that a variety of patient characteristics can affect (at least in part) the experience and perception of chronic pain (e.g. gender, cognitive and emotional factors, genetic/physiological heterogeneity, central sensitization or changes in pain experience over time, neural tolerance or loss of clinical efficacy over time, and anatomical variation or inter-patient differences in structural anatomy) [Citation29,Citation30,Citation31,Citation32,Citation33]. Thus, conceptually, it is our view that the notion that use of a single, fixed SCS waveform can be an all-encompassing solution which provides optimal short term and long-term pain relief for all SCS-implanted patients alike is not supported by the consolidation of reported evidence . Accordingly, treatment paradigms integrating multiple therapeutic options may be now particularly well suited to improve and provide sustained benefit to the widest cross-section of patients with chronic pain. Additionally, as with any treatment and as observed in this real-world evaluation, not all patients report being pain-free despite all measures taken from a programming standpoint. This may be attributed to varying factors including (but not limited to) underlying etiology and pain patterns.
The current study has several limitations due to the nature of retrospective data collection and limited data available in patient medical charts. Hence, the data reported here do not include functional outcomes such as assessment of disability and treatment satisfaction. Knowing whether outcomes would be less favorable with other devices or if some patients require more than one stimulation paradigm for effective pain relief could not be determined. In addition, the specific utilization and outcomes associated with the various modes of SCS treatment that were available to patients assessed in this study could not be discerned. Future clinical investigations will be required to evaluate what proportion of SCS-implanted patients require more than one waveform for optimal pain relief and what correlates may exist with the use of any particular neurostimulation approach as well as assess other endpoints besides reduction in reported pain alone.
5. Conclusions
This large, real-world, multicenter observational study demonstrates that an SCS device engineered to enable patient-specific implementation of combination therapy, multiple waveform options, and enhanced targeting capabilities can allow for highly effective pain relief. In addition, the variable diagnoses for chronic low back/leg pain that are represented within the patients in this cohort, in conjunction with the pain relief outcomes ultimately obtained, suggest that the benefits of a ‘customizable’ system may be broadly applicable to a range of diverse candidates for SCS for use in the treatment of chronic pain.
Authorship contributions
Drs. Metzger, Hammond, Pyles, Washabaugh, Waghmarae, Berg, and North carried out the study including collecting patient data. Mrs. Jain and Dr. Metzger contributed to study design and helped prepare the manuscript. Statistical support in analyzing the data was carried out by Ms. Pei with additional input from Mrs. Jain. All authors critically reviewed and approved the submitted manuscript.
Data sharing
The data, analytic methods, and study materials for this clinical trial will be made available to other researchers in accordance with the Boston Scientific Data Sharing Policy (https://www.bostonscientific.com/).
Declaration of interest
MB Hammond reports personal fees from Orthomicrospine and Boston Scientific. ST Pyles has received speaker honoraria from and is a consultant for Boston Scientific. R Waghmarae, AP Berg, and JM North are consultants for Boston Scientific. Y Pei and R Jain are employees of Boston Scientific. The authors have no other relevant affiliations or financial involvement with any organization or entity with a financial interest in or financial conflict with the subject matter or materials discussed in the manuscript apart from those disclosed.
Reviewer disclosures
One peer reviewer has received honoraria for their role as a consultant and for faculty at sponsored events and their institution’s R&D department has received research payments for investigator-sponsored and manufacturer-sponsored research from Boston Scientific. Another peer reviewer has performed research on spinal cord stimulation sponsored by Boston Scientific Corporation and has licensed patents on the novel temporal pattern of spinal cord stimulation from which they receive royalty payments. Another peer reviewer served in the past as a consultant to Boston Scientific, Nevro, and Saint Jude medical. Peer reviewers on this manuscript have no other relevant financial relationships or otherwise to disclose.
Acknowledgments
The authors would like to thank Daniel Halperin, PhD for writing and editorial assistance during the preparation of this manuscript.
Additional information
Funding
References
- Kriek N, Groeneweg JG, Stronks DL, et al. Preferred frequencies and waveforms for spinal cord stimulation in patients with complex regional pain syndrome: a multicentre, double-blind, randomized and placebo-controlled crossover trial. Eur J Pain. 2017 Mar;21(3):507–519.
- Berg AP, Mekel-Bobrov N, Goldberg E, et al. Utilization of multiple spinal cord stimulation (SCS) waveforms in chronic pain patients. Expert Rev Med Devices. 2017 Aug;14(8):663–668.
- Provenzano DA, Rebman J, Kuhel C, et al. The efficacy of high-density spinal cord stimulation among trial, implant, and conversion patients: a retrospective case series. Neuromodulation. 2017 Oct;20(7):654–660.
- Kumar V, Prusik J, Lin Y, et al. Efficacy of alternating conventional stimulation and high frequency stimulation in improving spinal cord stimulation outcomes: a pilot study. Neuromodulation. 2018 July;21(5):466–471.
- Duse G, Reverberi C, Dario A. Effects of multiple waveforms on patient preferences and clinical outcomes in patients treated with spinal cord stimulation for leg and/or back pain. Neuromodulation. 2019 Feb;22(2):200–207.
- North J, Loudermilk E, Lee A, et al. Outcomes of a multicenter, prospective, crossover, randomized controlled trial evaluating subperception spinal cord Stimulation at ≤1.2 kHz in previously implanted subjects. Neuromodulation. 2019 July 2. [Epub ahead of print]. DOI:10.1111/ner.13015
- Kapural L, Yu C, Doust MW, et al. Comparison of 10-kHz high-frequency and traditional low-frequency spinal cord stimulation for the treatment of chronic back and leg pain: 24-month results from a multicenter, randomized, controlled pivotal trial. Neurosurgery. 2016 Nov;79(5):667–677.
- Kapural L, Yu C, Doust MW, et al. Novel 10-kHz high-frequency therapy (HF10 Therapy) is superior to traditional low-frequency spinal cord stimulation for the treatment of chronic back and leg pain: the SENZA-RCT randomized controlled trial. Anesthesiology. 2015;123:851–860.
- Russo M, Verrills P, Mitchell B, et al. High frequency spinal cord stimulation at 10 kHz for the treatment of chronic pain: 6-month Australian clinical experience. Pain Physician. 2016;19:267–280.
- De Andres J, Monsalve-Dolz V, Fabregat-Cid G, et al. Prospective, randomized blind effect-on-outcome study of conventional vs high-frequency spinal cord stimulation in patients with pain and disability due to failed back surgery syndrome. Pain Med. 2017 Dec 1;18(12):2401–2421.
- DiBenedetto DJ, Wawrzyniak KM, Schatman ME, et al. 10 kHz spinal cord stimulation: a retrospective analysis of real-world data from a community-based, interdisciplinary pain facility. J Pain Res. 2018 Nov 20;11:2929–2941.
- Muhammad S, Roeske S, Chaudhry SR, et al. Burst or high-frequency (10 kHz) spinal cord stimulation in failed back surgery syndrome patients with predominant back pain: one year comparative data. Neuromodulation. 2017 Oct;20(7):661–667.
- Courtney P, Espinet A, Mitchell B, et al. Improved pain relief with burst spinal cord stimulation for two weeks in patients using tonic stimulation: results from a small clinical study. Neuromodulation. 2015 July;18(5):361–366.
- de Vos CC, Bom MJ, Vanneste S, et al. Burst spinal cord stimulation evaluated in patients with failed back surgery syndrome and painful diabetic neuropathy. Neuromodulation. 2014 Feb;17(2):152–159.
- Vesper J, Slotty P, Schu S, et al. Burst SCS Microdosing is as efficacious as standard burst SCS in treating chronic back and leg pain: results from a randomized controlled trial. Neuromodulation. 2019 Feb;22(2):190–193.
- Thomson SJ, Tavakkolizadeh M, Love-Jones S, et al. Effects of rate on analgesia in kilohertz frequency spinal cord stimulation: results of the PROCO randomized controlled trial. Neuromodulation. 2018;21:67–76.
- Veizi E, Hayek SM, North J, et al. Spinal cord stimulation (SCS) with anatomically guided (3D) neural targeting shows superior chronic axial low back pain relief compared to traditional SCS-LUMINA study. Pain Med. 2017;18:1534–1548.
- Szucs P, Luz LL, Pinho R, et al. Axon diversity of lamina I local-circuit neurons in the lumbar spinal cord. J Comp Neurol. 2013;521(12):2719–2741.
- Jensen MP, Brownstone RM. Mechanisms of spinal cord stimulation for the treatment of pain: still in the dark after 50 years. Eur J Pain. 2019;23(4):652–659.
- Rubinstein JT. Axon termination conditions for electrical stimulation. IEEE Trans Biomed Eng. 1993;40(7):654–663.
- McIntyre CC, Grill WM. Excitation of central nervous system neurons by nonuniform electric fields. Biophys J. 1999;76(2):878–888.
- Gustafsson B, Jankowska E. Direct and indirect activation of nerve cells by electrical pulses applied extracellularly. J Physiol. 1976;258(1):33–61.
- Radman T, Ramos RL, Brumberg JC, et al. Role of cortical cell type and morphology in subthreshold and suprathreshold uniform electric field stimulation in vitro. Brain Stimul. 2009;2(4):215–228.e2283.
- Dostrovsky JO, Levy R, Wu JP, et al. Microstimulation-induced inhibition of neuronal firing in human globus pallidus. J Neurophysiol. 2000;84(1):570–574.
- Tiede J, Brown L, Gekht G, et al. Novel spinal cord stimulation parameters in patients with predominant back pain. Neuromodulation. 2013 July–Aug;16(4):370–375.
- Al-Kaisy A, Van Buyten JP, Smet I, et al. Sustained effectiveness of 10 kHz high-frequency spinal cord stimulation for patients with chronic, low back pain: 24-month results of a prospective multicenter study. Pain Med. 2014 Mar;15(3):347–354.
- Deer T, Slavin KV, Amirdelfan K, et al. Success using neuromodulation with BURST (SUNBURST) study: results from a prospective, randomized controlled trial using a novel burst waveform. Neuromodulation. 2018 Jan;21(1):56–66.
- Haider N, Ligham D, Quave B, et al. Spinal cord stimulation (SCS) trial outcomes after conversion to a multiple waveform SCS system. Neuromodulation. 2018 July;21(5):504–507.
- Melchior M, Poisbeau P, Gaumond I, et al. Insights into the mechanisms and the emergence of sex-differences in pain. Neuroscience. 2016 Dec;3(338):63–80.
- Zorina-Lichtenwalter K, Meloto CB, Khoury S, et al. Genetic predictors of human chronic pain conditions. Neuroscience. 2016 Dec;3(338):36–62.
- Pergolizzi J, Ahlbeck K, Aldington D, et al. The development of chronic pain: physiological CHANGE necessitates a multidisciplinary approach to treatment. Curr Med Res Opin. 2013;29(9):1127–1135.
- Kumar K, Hunter G, Demeria D. Spinal cord stimulation in treatment of chronic benign pain: challenges in treatment planning and present status, a 22-year experience. Neurosurgery. 2006 Mar;58(3):481–496.
- Holsheimer J, Khan YN, Raza SS, et al. Effects of electrode positioning on perception threshold and paresthesia coverage in spinal cord stimulation. Neuromodulation. 2007 Jan;10(1):34–41.