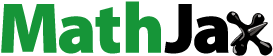
This article is based on paper number OMAE2016-54251 by Professor Q. M. Li which was presented and published in 35th International Conference on Ocean, Offshore and Arctic Engineering (OMAE 2016) held in Busan, South Korea.
Brief citation
This article summarizes Professor Norman Jones FREng’s academic career and his scholarly contributions to impact engineering. In the past 54 years, Professor Jones has performed profound research on a wide range of impact engineering problems, supervised postgraduate students, researchers and academic visitors from all over the world, initiated international research networks and conferences, and has played important roles in consulting government bodies and in generally serving the academic community. Due to his research excellence and achievements, Professor Jones has received numerous prestigious awards and titles including Fellowship of the Royal Academy of Engineering and Foreign Fellowship of the Indian National Academy of Engineering.
Early time (1938-1965)
Norman Jones was born in Farnham, Surrey, England on 18th March, 1938 to parents who hailed from Liverpool. His father was a carpenter and his mother a housewife. They moved back to Liverpool after World War II with their two sons when Norman was 8 years old.
Norman attended Evered High School between 1951 and 1954 and was awarded the school prize in Geography! In 1954, he took up a highly sought-after mechanical engineering apprenticeship sponsored by the government. This provided Norman with an excellent training opportunity and he was introduced to mechanical engineering through technical college lectures and the practical use of various tools and machines. The knowledge that he gained and his improved understanding of engineering acquired during his apprenticeship provided an excellent foundation for Norman’s subsequent research career, particularly for the design of experimental equipment and the interpretation of experimental results.
Norman resigned after four years of his apprenticeship (normally five years long), to pursue a full-time mechanical engineering degree course at the University of Manchester Institute of Science and Technology (UMIST) in 1958 with the aid of a State Scholarship. His graduation with first class honours in 1961 was followed by MSc Tech in 1962 and a PhD in 1965, during which time he also worked as an Assistant Lecturer in Mechanical Engineering at UMIST. Norman’s post-graduate research projects were associated with theoretical and experimental studies on the static elastic behaviour of pressure vessels and pipelines as well as numerical solutions, initially with the aid of the Mercury computer and then soon afterwards with the Atlas computer. It should be noted that Manchester back then led the world-wide development of computers and computer sciences with computer pioneers, such as Turing, Williams, Kilburn, on the academic staff. The first Atlas was commissioned at the University of Manchester in 1962 (the same year when Norman started his Ph.D) and at that time was considered to be the world’s fastest supercomputer!
Period in the United States of America (1965-1979)
Norman married Jenny in 1964. In 1965, they crossed the Atlantic on board the Cunard vessel Sylvania from Liverpool to New York in order to take up a position as an Assistant Professor in the Department of Mechanical Engineering at the Georgia Institute of Technology. Coincidentally, they met Professor Paul S. Symonds and his family during the Atlantic Ocean crossing and, later in the year, Professor Symonds invited Norman to give a seminar at Brown University in Providence, Rhode Island. At that time, Brown University was one of the world’s focal points for static and dynamic plasticity research where Norman met Professors Drucker, Martin, Kolsky and others working in this area. With his knowledge of the pioneering work at UMIST by Professor William Johnson on the plastic methods of analysis for various manufacturing procedures and Professor Gill on the plastic behaviour of pressure vessels and structures, Norman was fascinated with this new area, and particularly, the dynamic plastic behaviour of structures. Norman’s ‘engagement’ with structural impact thus began and soon this became another lifelong ‘marriage’ when Norman took up an Assistant Professor position in the Department of Engineering at Brown University in 1966. The experimental and theoretical studies with Professor Symonds focused on the retention of finite-displacements, or geometry changes, during the dynamic plastic response of beams [Citation1] and circular plates [Citation2] which revealed a considerable increase in the strength and energy absorption capability when compared with the predictions of a classical rigid-plastic theory based on infinitesimal transverse displacements.
In 1968, Norman had an opportunity to become an Assistant Professor in the Department of Naval Architecture and Marine Engineering (soon re-named as Ocean Engineering) at MIT (Massachusetts Institute of Technology) where he was able to develop his research interests further in dynamic plasticity and impact engineering. Experimental studies were undertaken with graduate students on the response of beams, rectangular plates and shells subjected to explosive loadings which produced large deformations and plastic strains (e.g. [Citation3-5]). Reference [Citation3] developed a theoretical method for the dynamic plastic behavior of ductile plates which could have any planar shape and boundary conditions and subjected to dynamic pressure, mass impact or impulsive loadings with various spatial distributions. In fact, the theoretical procedure was general enough to examine the large deflection behavior of rectangular plates subjected to static loads [Citation6] which is a common structural arrangement in naval architecture and ocean engineering. The same method was also used to study the important influence of very small in-plane displacements at the boundaries of beams and plates [Citation7]. This relatively simple method has been used by a number of research groups to study a variety of structures. The theoretical rigid-plastic methods of analysis were observed to predict surprisingly accurate agreement with the corresponding experimental data. As a result, these simple rigid-plastic methods of analysis soon turned out to be quite useful for preliminary design purposes. Many of his research projects were relevant to the field of naval architecture and ocean engineering as summarized in a review paper which was published in the Transactions of SNAME [Citation8]. For example, the theoretical methods developed in the early stages for rectangular plates were used to study the slamming damage on the bottom plating [Citation9] and ice damage [Citation10] on the side plating of ships and marine vehicles, as well as deck and bow loadings [Citation10]. The slamming and ice damage behaviour was studied from the perspective of the new phenomenon of pseudo-shakedown which he developed in [Citation9] to characterize the behaviour of a structure with a permanently deformed configuration which was reached after a sufficient number of repeated impact loadings. The rigid-plastic method of analysis was also used to modify the Minorsky equation for minor ship collisions in order to explain some of the scatter in the original data by recognizing that the collection from actual ship collisions contained plating having a wide range of permanent displacements (damage) [Citation11,Citation12]. It was also used to predict the plastic collapse of a tanker web frame with Mansour [Citation13] and to explore the energy absorbed through higher modal responses in beams with Wierzbicki [Citation14] and Guedes Soares [Citation15]. Around this time, he extended the limit analysis of Caldwell in order to explore the conditions when repeated loads acting on the mid-ship section of a ship hull would cause classical shakedown to a wholly elastic state or cause incremental collapse [Citation16].
The dynamic plastic buckling of rings and shells subjected to explosive loadings was explored both experimentally and theoretically [Citation17], while the phenomenon of creep buckling was studied in [Citation18]. The influence of transverse shear and rotatory inertia on the response of structural members was explored with de Oliveira [Citation19] and later with Q.M. Li [Citation20]. This work provided some design guidance which could be used to estimate the loss of accuracy in design calculations when disregarding these effects.
In fact, it was observed in [Citation21] that the rigid, perfectly plastic method could be used to predict the failure of ductile beams when loaded dynamically. The beam failure was characterised as modes 1, 2 and 3 which were large ductile deformations (damage), large tensile strains causing rupture and transverse shear failure, respectively The predictions were largely in agreement with independent experimental results. Several other research groups have further developed these ideas for the analysis of other structures under static and dynamic loads.
Liverpool period (1979-)
Norman resigned from his position as a tenured full professor at MIT in 1979 in order to return to England and take up a position as Professor of Mechanical Engineering at the University of Liverpool (Head of Department, 1982-90, A.A. Griffith Professor 1993-2005, Emeritus Professor 2005-) and Director of the Impact Research Centre 1985-2005.
He continued with his research interest in naval architecture and ocean engineering and studied the grounding of ships and ship collisions [Citation22,Citation23], aspects of the behavior of ice loadings [Citation24,Citation25], including introducing a dimensionless ice number [Citation26], slamming loads [Citation27], the strength of wire ropes [Citation28-30], and the impact response of pipelines with and without an internal pressure [Citation31-33]. The pseudo-shakedown phenomenon, which he defined in [Citation9], was used to study the response of the bottom plating of a ship’s hold when subjected to repeated impact loadings from cargo [Citation34] and has been explored for stiffened plates under repeated impact loadings [Citation35] and further explored recently to examine the shakedown characteristics of a Suezmax-class double-hull oil tanker with Paik [Citation36]. Some of these studies have been have been summarized in the Transactions of RINA [Citation37]
A wide range of topics associated largely with the dynamic plastic response of structural problems were studied with a succession of outstanding graduate students and visitors over the subsequent 26 years. These included further considerations of the dynamic plastic buckling phenomenon with Karagiozova [Citation38,Citation39], and some theoretical underpinning for the pseudo-shakedown phenomenon for large repeated loadings causing large inelastic deformations [Citation40]. Other studies focused on the perforation of ductile plating struck at relatively low impact velocities [41- 47], transverse shear failure with Q.M. Li [Citation48,Citation49] and with Lili Wang [Citation50]. Further studies on the failure of structures [Citation51-53] and on the applications of damage mechanics in order to predict the failure of structures subjected to dynamic loads causing large inelastic deformations with Alves [Citation54-55]. The post-severance response of beams and plates was also studied [Citation56-58], and other recent investigations have provided some insight into the dynamic plastic response of circular, square and rectangular plates with and without strain-rate sensitivity effects and subjected to mass impact or blast loadings [Citation59,Citation60]. The low velocity axial impact crushing, or dynamic progressive buckling, of circular cylindrical and square thin-walled sections made from mild steel were studied with Abramowicz [Citation61-64]. High strength steels [Citation65-67] were investigated, together with studies on top-hat and double-hat sections [Citation68-71], which are of particular interest for the design of energy absorbing devices in structural safety calculations and structural crashworthiness design.
In contrast to the dynamic progressive buckling of thin-walled structural members, which is often idealised as a quasi-static behaviour, the influence of transverse and axial inertia effects and plastic stress waves are a fundamental aspect of the dynamic plastic buckling phenomenon [Citation72,Citation73]. In some of these studies, a finite-element method of analysis was used to reveal important features of the dynamic response, and comparisons were made with the behaviour of axially impacted thin-walled circular and square tubes, which were examined experimentally by Bell, Florence and others [Citation74]. The simultaneous retention of stress wave propagation and the structural response has led to an increased understanding of the time-dependent changes in the deformation mode and insight into the influence of different material properties and geometrical parameters. These more complete analyses are vital for the higher velocity impact scenarios encountered increasingly in practical designs and for comparing properly the relative merits of employing different ductile materials in energy absorbing systems [Citation73,Citation75,Citation76].
In view of the increasing developments and growing interest in the structural crashworthiness field and in the energy absorption of structures, Norman and Professor Wierzbicki organised the 1st International Symposium on Structural Crashworthiness which was held at the University of Liverpool in 1983. After the success of this conference, it was decided to create an Impact Research Centre at the University of Liverpool in 1985. The 2nd and 3rd International Symposia on Structural Failure and Structural Crashworthiness and Failure were organised by Professors Jones and Wierzbicki and held at MIT (1988) and Liverpool (1993), respectively. This series has been continued in recent years with emphasis on lightweight materials and other developments by Professor M. Alves in Florianopolis, Brazil (2005), Professor M. Langseth in Trondheim, Norway (2008), Professor E. Markiewicz, Valenciennes, France (2011), Professor G.N. Nurick in Cape Town (2014) and by Professor G. Belingardi in Italy (2016).
About the time of the first Symposium, it was decided to institute an international research journal that focused on the response of structures and components subjected to dynamic loadings. These ideas morphed into the International Journal of Impact Engineering (IJIE) with Professor W. Johnson as the Editor-in-Chief and Professor Jones as the Editor in March 1983. This journal has increased from strength to strength under the leadership of Professor Jones (Editor 1983-1988, Editor-in-Chief 1988-2008, Honorary Editor-in–Chief, 2008-) and Professor M. Langseth (Editor-in-Chief since 2008).
Other research areas in the Impact Research Centre at Liverpool included investigations into the constitutive equations for strain rate sensitive materials [Citation77-81]. In particular, the form of the constitutive equation for structures with large strains, as encountered in naval architecture and ocean engineering, was proposed by modifying the well known Cowper Symonds equation as discussed in [Citation79-81] An expression for the influence of material strain rate sensitivity on the rupture strain for ductile materials was also proposed in [Citation79-81].
A study was undertaken into the scaling laws for the dynamic behaviour of structures. Of particular interest was the relationship between the actual behaviour of a full-scale prototype and the predictions for the associated large plastic deformations, energy absorption and failure according to the classical scaling laws based on the scaled-up response of a small-scale model. This topic can be important in the naval architecture and ocean engineering fields because of the large size differences of small-scale laboratory structural models and the corresponding full-scale prototypes. It transpires that large ductile deformations, appear to scale reasonably well when compensating for the influence of material strain-rate sensitivity which does not scale according to the elementary Cauchy scaling laws [Citation82-84]. However, this agreement breaks down when failure intervenes during a dynamic response [Citation83, Citation85]. The key factor appears to be the relative amounts of dynamic energy which are absorbed in shearing type failures that scale approximately with the area (, where
is a scale factor) and that energy which is absorbed in bending and membrane deformations (that scale according to the volume of material,
). If the ratio between these two effects is relatively large, as in the large mass and low velocity impact perforation of thin plating, then most of the impact energy is absorbed in the membrane and bending modes which dominate the amount of energy consumed in the shear deformation and failure modes [Citation86,Citation87]. Thus, scaling is essentially preserved in several geometries, including pipelines, when viewed from an engineering perspective. On the other hand, if the amount of energy absorbed in shear and failure is large, then the classical scaling laws are not satisfied, as observed, for example, in the welded plate structural impact tests reported in [Citation88] and in a series of scaled tests conducted at Liverpool on beams [Citation49,Citation85], and wedge-plate impact tests [Citation83]. However, it was found that the simple scaling laws are essentially satisfied for the low velocity impact loading of fibre-metal laminates [Citation89]. More recently, some studies have shown that the responses of foam-filled sandwich structures do scale when subjected to low velocity transverse impact loadings in a drop hammer rig [Citation90]. It transpires that size effects were observed in the load-displacement relations for the smaller test specimens, which were most likely due to the relative size of the impactor and the weave dimensions of the composite skins. Chapter 11 in [Citation84] which starts with Galileo’s observation of the scaling law for animals’ strength has guided many research studies and applications of the scaling laws and size effects in the field of structural impact.
An Energy Absorbing Effectiveness Factor (EAEF) was defined in [Citation91-94] to overcome some difficulties which were encountered when comparing the efficiency of energy absorbing systems made from different materials and/or having different designs. This factor compares the energy absorbed in an actual system with the maximum amount of external energy that could possibly be absorbed on the assumption that all of the material from which the system is manufactured was available for energy absorption. Thus, comparisons could be made about the effectiveness of devices which are made from different materials, or even between different designs. This factor could also be regarded as an Environmental Factor in the sense that it reveals a system which requires the least amount of material in the active and other essential (inactive) parts of a device in order to absorb a certain amount of external energy. For example, it turns out that the ductile behaviour of a given design (i.e., same dimensions and impact energy) for a class of axially crushed cylindrical thin-walled tubes would be more effective when made from an aluminum alloy than when made from mild steel [Citation91]. This situation occurs because the larger rupture strain of mild steel is not required to absorb a given amount of energy in the class of problems considered. In other words, the energy absorbing capacity, or potential of the material from which a mild steel tube is made, is greater than that required to absorb the given amount of energy when compared to a similar aluminium alloy tube which has a smaller rupture strain that is nevertheless sufficient to avoid failure. On the other hand, when comparing different designs which are required to absorb the same amount of external energy, then the factor reveals that a circular tube is more effective than a square one. Thus, this factor provides some insight into the design of energy absorbing systems, and along with the other usual factors, could be used to improve their design in engineering practice.
Another interesting and recent study undertaken by Norman is on the credibility of predictions from finite-element programmes which are used extensively throughout the engineering industry for design calculations [Citation95]. The finite-element methods are, of course, a powerful method of analysis and are particularly accurate for static and dynamic linear elastic structural designs. However, the accuracy is less certain for some other cases, particularly for complex systems and extreme loading situations which involve large plastic strains, failure and other nonlinearities that arise for safety and security calculations in naval architecture and ocean engineering and in many other industries. As noted by Thornton [Mechanical Engineering, ASME, 2010] that “The shortcomings in FEA usually arise in making the mental jumps between theory and the FEA model, between reality and virtual reality”. For example, there are difficulties in the modeling of riveted joints in thin-walled sections which can suffer tearing and subsequent uncontrolled behaviour in the structure near to the rivets [Citation65]. Even the modeling of boundary conditions can be difficult, as shown by [Citation51,Citation52] for the impact failure of a clamped beam. It turns out that for nominally identical problems a beam can fail either at a support or at the mid-span underneath an impactor depending on the details of a clamped support. The supports of the two kinds of beams studied would be classed as clamped in typical engineering practice so it appears that more effort should be expended in the modelling of boundary conditions. In fact, this can be generalized as an issue of the ‘dynamic’ version of Saint-Venant's Principle. In addition, approximations must be introduced into the constitutive equations which typically ignore strain-rate history effects and are usually obtained from one-dimensional tests but are used for two and three dimensional practical situations. The actual details of a practical dynamic loading and the actual pressure-time history are different issues that require further study. Thus, it is vitally important to assess the accuracy of finite-element predictions.
His research activities on impact engineering are still on-going with studies into new materials [Citation96,Citation97].
Final remarks
This article presents a selection of the 320 publications by Prof. Norman Jones which contributes to the body of knowledge on impact engineering and naval architecture and ocean engineering. These publications have been widely used and cited.
In addition to his research contributions to the field of impact engineering, Norman has served many societies and the research community in various ways. He has delivered numerous public lectures and keynote talks in many countries to disseminate research results and to educate the next generation of researchers. As an example, Norman was invited by the Institution of Mechanical Engineers (I.Mech.E) in 1985 to give the Issac Newton Lecture. The talk was across a large time span, as indicated by the title “Shape and Strength: from dinosaurs to spacecraft”, and the presentation was extremely successful and was repeated about 30 times during 1985-1986 in cities throughout the UK. He has maintained his interest in education as evidenced by his recent paper with Schleyer [Citation98].
Prof. Jones has also served many times as a conference chair, has served on various committees in the US and the UK, journal editorial boards and government committees. In particular, he served as the Associate Editor for Applied Mechanics Reviews (1995-2008) and as an Associate Editor for the Latin America Journal of Solids and Structures despite his full-time management of IJIE during his Editor-in-Chief period. He was secretary (1973-1976) for the International Ship Structure Congress which was held at MIT in 1976.
Norman is a Professional Engineer (Massachusetts), a Charted Engineer (UK), a Fellow of I.Mech.E, a Fellow of the Royal Institution of Naval Architects, a Fellow of ASME and a Fellow of the Wessex Institute of Technology (WIT). He obtained his Doctor of Science from the University of Manchester (1980), received the William Sweet Smith Prize (with R.S. Birch, W.S. Jouri) in 1989, the Ludwig Mond Prize (with W.Q. Shen) in 1992 from I.Mech.E, the Eminent Scientist Medal in 1998 from WIT and a bronze medal from RINA in 1998 for reference [Citation37]. He was honoured as Lifetime Honorary Professors by Huazhong University (1987) and Taiyuan University of Technology (1988). He was elected as a Fellow of the Royal Academy of Engineering (1998) and is a Foreign Fellow of Indian National Academy of Engineering (2005) to acknowledge his international distinctions.
As a pioneer of impact engineering, Professor Jones is greatly acknowledged in association with his contributions to the impact engineering field, among others.
References
- Symonds P.S., Jones N. (1972), Impulsive Loading of Fully Clamped Beams with Finite-Plastic Deflections and Strain Rate Sensitivity, International Journal of Mechanical Sciences, Vol. 14, No. 1, pp. 49-69.
- Jones N. (1968), Impulsive Loading of a Simply Supported Circular Rigid-Plastic Plate, Journal of Applied Mechanics, Vol. 35, No. 1, Transactions A.S.M.E., Series E, pp. 59-65.
- Jones N. (1971), A Theoretical Study of the Dynamic Plastic Behavior of Beams and Plates with Finite-Deflections, International Journal of Solids and Structures, Vol. 7, pp. 1007-1029.
- Jones N., Griffin R.N., Van Duzer R.E. (1971), An Experimental Study Into the Dynamic Plastic Behavior of Wide Beams and Rectangular Plates, International Journal of Mechanical Sciences, Vol. 13, No. 8, pp. 721-735.
- Walters R.M., Jones N. (1972), An Approximate Theoretical Study of the Dynamic Plastic Behavior of Shells, International Journal of Non-Linear Mechanics, Vol. 7, No. 3, pp. 255-273.
- Jones, N., Walters, R. M. (1971), Large Deflections of Rectangular Plates, Journal of Ship Research, Vol. 15, No. 2, pp.164-171 and p.288.
- Jones, N. (1973), Influence of In-Plane Displacements at the Boundaries of Rigid-Plastic Beams and Plates, International Journal of Mechanical Sciences, Vol. 15, No. 7, pp.547-561.
- Jones N. (1976), Plastic Behavior of Ship Structures, Transactions, Society of Naval Architects and Marine Engineers, Vol. 84, pp. 115-145.
- Jones N. (1973), Slamming Damage, Journal of Ship Research, Vol. 17, No. 2, pp.80-86.
- Jones N. (1977), Damage Estimates for Plating of Ships and Marine Vehicles, International Symposium on Practical Design in Shipbuilding, Society of Naval Architects of Japan, Tokyo, pp. 121-128.
- Jones, N. (1979), A Literature Survey on the Collision and Grounding Protection of Ships, Ship Structure Committee Report SSC-283.
- Giannotti, J. G. Van Mater, P. R., Jones, N. (1980), Critical Evaluation of Low-Energy Ship Collision Damage Theories and Design Methodologies, Society of Naval Architects and Marine Engineers, STAR Symposium pp.79-90.
- Mansour A., Jones N. (1973), Elastic and Plastic Analysis of a Tanker Web Frame, Journal of Ship Research, Vol. 17, No. 3, pp. 147-161.
- Jones N., Wierzbicki T. (1976), A Study of the Higher Modal Dynamic Plastic Response of Beams, International Journal of Mechanical Sciences, Vol. 18, pp. 533-542.
- Jones N., Guedes Soares C.A.P. (1978), Higher Modal Dynamic Plastic Behavior of Beams Loaded Impulsively, International Journal of Mechanical Sciences, Vol. 20, No. 3, pp. 135-147.
- Jones N. (1975), On the Shakedown Limit of a Ship's Hull Girder, Journal of Ship Research, Vol. 19, No. 2, pp. 118-121.
- Okawa D.M., Jones, N. (1976), Dynamic Plastic Buckling of Rings and Cylindrical Shells, Nuclear Engineering and Design, Vol. 37, No. 1, pp. 125-147.
- Xirouchakis P.C., Jones N. (1980), Axisymmetric and Bifurcation Creep Buckling of Externally Pressurized Spherical Shells, International Journal of Solids and Structures, Vol. 16, No. 2, pp. 131-148.
- Jones N., de Oliveira J.G. (1979), The Influence of Rotatory Inertia and Transverse Shear on the Dynamic Plastic Behavior of Beams, Trans. ASME Journal of Applied Mechanics, Vol.46, No.2, pp.303-310.
- Li Q.M., Jones N. (2000), Formation of a Shear Localisation in Structural Elements under Transverse Dynamic Loads, International Journal of Solids and Structures, Vol.37, No.45, pp.6683-6704.
- Jones, N. (1976), Plastic Failure of Ductile Beams loaded Dynamically, Trans. ASME, Journal of Engineering for Industry, Vol.98, Series B, No. 1, pp. 131-136.
- Jones, N., Jouri, W. S. (1987), A Study of Plate Tearing for Ship Collision and Grounding Damage, Journal of Ship Research, Vol.31, No.4, pp.253-268.
- Jones N. (1983), Structural Aspects of Ship Collisions, Structural Crashworthiness, Ed. N. Jones and T. Wierzbicki, Butterworths Publishers, London and Boston, pp. 308-337.
- Ashby, M. F. et al (1986), Nonsimultaneous Failure and Ice Loads on Arctic Structures, Proceedings Offshore Technology Conference, Paper No. OTC5127, pp. 399-404.
- Hallam, S. D., Jones, N., Howard, M. W. (1987) The Effect of Sub-Surface Irregularities on the Strength of Multi-Year Ice, Proceedings 6th International Offshore Mechanics and Arctic Engineering Symposium, Ed V. J. Lunardini et al ASME, Vol.4, pp 235-238.
- Jones, N. (1986), A Note on Ice Scaling, Journal of Ship Research, Vol. 30, No. 2, pp.134-135.
- Karagiozova, D., Jones, N. (1996), Dynamic Buckling of Columns Due to Slamming Loads, Transactions on the Built Environment Vol. 22, WIT Press, pp. 311-320.
- Jones, N. (1984), Behaviour of Wire Ropes in Offshore Applications, Wire Industry, Vol. 51, No. 606, pp. 471-473.
- Utting, W. S., Jones, N. (1984), A Survey of Literature on the Behaviour of Wire Ropes, Wire Industry, Vol. 51, No.609, pp. 623-629.
- Utting, W. S., Jones, N. (1987), The Response of Wire Rope Strands to Axial Tensile Loads, Part 1; Experimental Results and Theoretical Predictions, Part 2; Comparison of Experimental Results and Theoretical Predictions, International Journal of Mechanical Sciences, Vol.29, No.9, pp.605-619 and 621-636.
- Jones N., Birch S.E., Birch R.S., Zhu L., Brown M. (1992), An Experimental Study on the Lateral Impact of Fully Clamped Mild Steel Pipes, Proc. I.Mech.E., Vol. 206(E), pp. 111-127.
- Jones N., Birch R.S. (2010), Low-velocity Impact of Pressurised Pipelines, International Journal of Impact Engineering, Vol. 37, No. 2, pp 207-219.
- Zhu L., Liu Q., Jones N., Chen M. (2018), Experimental Study on the Deformation of Fully Clamped Pipes under Lateral Impact, International Journal of Impact Engineering, Vol. 111, pp. 94-105.
- Jones N. (2014), Pseudo-Shakedown Phenomenon for the Mass Impact Loading of Plating, International Journal of Impact Engineering, Volume 65, pp. 33-39.
- Zhu L., Shi S., Jones N. (2018), Dynamic Response of Stiffened Plates under Repeated Impacts, International Journal of Impact Engineering, Vol. 117, pp. 113-122.
- Zhang, X., Paik, J. K., Jones, N. (2016), A New Method for assessing the Shakedown Limit State Associated with the Breakage of a Ship’s Hull Girder, Ships and Offshore Structures, Vol. 11, No.1, pp.92-104.
- Jones N. (1997), Dynamic Plastic Behaviour of Ship and Ocean Structures, Trans. Royal Institution of Naval Architects, Vol. 139, Part A, pp. 65-97.
- Karagiozova D., Jones N. (1992), Dynamic Pulse Buckling of a Simple Elastic-Plastic Model Including Axial Inertia, International Journal of Solids and Structures, Vol.29, No.10, pp.1255-1272.
- Karagiozova D., Jones N. (1996), Dynamic Elastic-Plastic Buckling Phenomena in a Rod Due to Axial Impact, International Journal of Impact Engineering, Vol. 18, No. 7/8, pp. 919-947.
- Shen W.Q., Jones N. (1992), The Pseudo-Shakedown of Beams and Plates When Subjected to Repeated Dynamic Loads, Trans. ASME Journal of Applied Mechanics, Vol.59, No.1, pp.168-175.
- Wen H.M., Jones N. (1994), Experimental Investigation into the Dynamic Plastic Response and Perforation of a Clamped Circular Plate Struck Transversely by a Mass, Proc. I.Mech.E., Vol. 208, No. C2, pp. 113-137.
- Wen H.M., Jones N. (1996), LowVelocity Perforation of Punch-Impact-Loaded Metal Plates, Trans. ASME, Journal of Pressure Vessel Technology, Vol. 118, No. 2, pp. 181-187.
- Jones N., Paik J.K. (2012), Impact Perforation of Aluminium Alloy Plates, International Journal of Impact Engineering, Vol. 48, pp 46-53.
- Jones N., Paik J.K. (2013), Impact Perforation of Steel Plates, Ships and Offshore Structures, Volume 8, Number 5, pp. 579-596, 2013.
- Jones N., Kim S.B. (1997), A Study on the Large Ductile Deformations and Perforation of Mild Steel Plates Struck by a Mass. Part I: Experimental Results, Part 2: Discussion, Trans. ASME, Journal of Pressure Vessel Technology, Vol. 119, No. 2, pp. 178-184 and 185-191.
- Jones N., Birch R.S. (2008), Low Velocity Perforation of Mild Steel Circular Plates with Projectiles having Different Shaped Impact Faces, ASME, Journal of Pressure Vessel Technology, Vol.130, No.3, pp. 031205-1 to 031205-11.
- Jones N., Birch R.S., Duan R. (2008), Low Velocity Perforation of Mild Steel Rectangular Plates with Projectiles having Different Shaped Impact Faces, Trans. ASME, Journal of Pressure Vessel Technology, Vol. 130, No.3, pp. 031206-1 to 031206-8.
- Li Q.M., Jones N. (1994), Blast Loading of Fully Clamped Circular Plates with Transverse Shear Effects, International Journal of Solids and Structures, Vol. 31, No. 14, pp. 1861-1876.
- Li Q.M., Jones N. (2002), Response and Failure of a Double-Shear Beam Subjected to Mass Impact, International Journal of Solids and Structures, Vol.39, No. 7. pp. 1919-1947.
- Wang L.L., Jones N. (1996), An Analysis of the Shear Failure of Rigid-Linear Hardening Beams Under Impulsive Loading, Acta Mechanica Sinica, Vol. 12, No. 4, pp. 338-348.
- Yu J.L., Jones N. (1989), Numerical Simulation of a Clamped Beam Under Impact Loading, Computers and Structures, Vol. 32, No. 2, pp. 281-293.
- Yu J.L., Jones N. (1991), Further Experimental Investigations on the Failure of Clamped Beams Under Impact Loads, International Journal of Solids and Structures, Vol. 27, No. 9, pp. 1113-1137.
- Yu J.L., Jones N. (1997), Numerical Simulation of Impact Loaded Steel Beams and the Failure Criteria, International Journal of Solids and Structures, Vol. 34, No. 30, pp. 3977-4004.
- Alves M., Jones N. (2002), Impact Failure of Beams Using Damage Mechanics: Part I–Analytical Model, International Journal of Impact Engineering, Vol. 27, No. 8, pp. 837-861.
- Alves M., Jones N. (2002), Impact Failure of Beams Using Damage Mechanics: Part II – Application, International Journal of Impact Engineering, Vol. 27, No. 8, pp. 863-890.
- Jones N., Alves M. (2004), Post-Severance Analysis of Impulsively Loaded Beams, International Journal of Solids and Structures, Vol. 41, No. 22/23, pp. 6441-6463.
- Jones N., Alves M. (2006), Post-Failure Response of Impulsively Loaded Clamped Beams, European Journal of Mechanics, A/Solids. Vol. 25, No.5, pp. 707-728.
- Jones N., Alves M. (2010), Post-failure Behaviour of Impulsively Loaded Circular Plates, International Journal of Mechanical Sciences, Vol. 52, No. 5, pp.706-715.
- Jones N. (2012), Impact Loading of Ductile Rectangular Plates, Thin-Walled Structures, Vol. 50, No. 1, pp. 68-75.
- Jones N. (2014), Dynamic Inelastic Response of Strain Rate Sensitive Ductile Plates due to Large Impact, Dynamic Pressure and Explosive Loadings, International Journal of Impact Engineering, Vol. 74, pp. 3-15.
- Abramowicz W., Jones N. (1984), Dynamic Axial Crushing of Square Tubes, International Journal of Impact Engineering, Vol. 2, No. 2, pp. 179-208.
- Abramowicz W., Jones N. (1984), Dynamic Axial Crushing of Circular Tubes, International Journal of Impact Engineering, Vol. 2, No. 3, pp. 263-281.
- Abramowicz W., Jones N. (1986), Dynamic Progressive Buckling of Circular and Square Tubes, International Journal of Impact Engineering, Vol. 4, No. 4, pp. 243-270.
- Abramowicz W., Jones N. (1997), Transition from Initial Global Bending to Progressive Buckling of Tubes Loaded Statically and Dynamically, International Journal of Impact Engineering, Vol. 19, No. 5/6, pp. 415-437.
- Schneider F.D., Jones N. (2003), Influence of Spot-Weld Failure on Crushing of Thin-Walled Structural Sections, International Journal of Mechanical Sciences, Vol. 45, No. 12, pp. 2061-2081.
- Schneider F.D., Jones N. (2004), Impact of Thin-Walled High-Strength Steel Structural Sections, Proc. Institution of Mechanical Engineers, Vol. 218, Part D, Journal of Automobile Engineering, 131-158.
- Peixinho N., Jones N., Pinho A. (2003), Experimental and Numerical Study in Axial Crushing of Thin Walled Sections Made of High-Strength Steels, Journal Physics IV France, Vol. 110, pp. 717-722.
- White M.D., Jones N. (1999), Experimental Quasi-Static Axial Crushing of Top-Hat and Double-Hat Thin-Walled Sections, International Journal of Mechanical Sciences, Vol. 41, pp. 179-208.
- White M.D., Jones N., Abramowicz W. (1999), A Theoretical Analysis for the Quasi-Static Axial Crushing of Top-Hat and Double-Hat Thin-Walled Sections, International Journal of Mechanical Sciences, Vol. 41, pp. 209-233.
- White M.D., Jones N. (1999), Experimental Study into the Energy Absorbing Characteristics of Top-Hat and Double-Hat Sections Subjected to Dynamic Axial Crushing, Proceedings, Institution of Mechanical Engineers, Vol. 213, Part D, pp. 259-278.
- White M.D., Jones N. (1999), A Theoretical Analysis for the Dynamic Axial Crushing of Top-Hat and Double-Hat Thin-Walled Sections, Proceedings, Institution of Mechanical Engineers, Vol. 213, Part D, pp. 307-325.
- Karagiozova D., Jones N. (2001), Influence of Stress Waves on the Dynamic Progressive and Dynamic Plastic Buckling of Cylindrical Shells, International Journal of Solids and Structures, Vol. 38, No. 38/39, pp. 6723-6749.
- Karagiozova D., Jones N. (2004), Dynamic Buckling of Elastic-Plastic Square Tubes Under Axial Impact. Part II – Structural Response, International Journal of Impact Engineering, Vol. 30, No. 2, pp. 167-192.
- Jones N. (2003), Several Phenomena in Structural Impact and Structural Crashworthiness, European Journal of Mechanics A/Solids, Vol. 22, No. 5, pp. 693-707.
- Karagiozova D., Jones N. (2001), Dynamic Effects on Buckling and Energy Absorption of Cylindrical Shells Under Axial Impact, Thin-Walled Structures, Vo. 39, No. 7, pp. 583-610.
- Karagiozova D., Jones N. (2008), On the Mechanics of the Global Bending Collapse of Circular Tubes under Dynamic Axial Load – Dynamic Buckling Transition, International Journal of Impact Engineering, Vol. 35, No. 5, pp 397-424.
- Alves, M., Jones, N, (1999), Influence of Hydrostatic Stress on Failure of Axisymmetric Notched Specimens, Journal of the Mechanics and Physics of Solids, Vol. 47, No. 3, pp.643-667.
- Tanimura, S., Tsuda, T., Abe, A., Hayashi, H., Jones, N. (2014), Comparison of Rate Dependent Constitutive Models with Experimental Data, International Journal of Impact Engineering, Vol. 69, pp. 104-113.
- Jones, N, (2006), Some Recent Developments in the Dynamic Inelastic Behaviour of Structures, Ships and Offshore Structures, Vol. 1, No. 1, pp.37-44.
- Jones, N. (1989), Some Comments on the Modelling of Material Properties for Dynamic Structural Plasticity, Institute of Physics Conference Series, No. 102, Ed J. Harding. pp.435-445.
- Jones, N, (1993), Material Properties for Structural Impact Problems, Advances in Materials and their Applications, Ed. P. Rama Rao, Wiley Eastern Ltd, pp. 151-163.
- Wen H.M., Jones N. (1993), Experimental Investigation of the Scaling Laws for Metal Plates Struck by Large Masses, International Journal of Impact Engineering, Vol. 13, No. 3, pp.485-505.
- Jones N., Jouri W.S. (1987), A Study of Plate Tearing for Ship Collision and Grounding Damage, Journal of Ship Research, Vol. 31, No. 4, pp. 253-268.
- Jones, N., (2012) Structural Impact, 2nd Edition, Cambridge University Press, Cambridge, UK.
- Jouri W.S., Jones N. (1988), The Impact Behaviour of Aluminium Alloy and Mild Steel Double-Shear Specimens, International Journal of Mechanical Sciences, Vol. 30, No. 3/4, 153-172.
- Jones N., Birch R.S. (2008), On the Scaling of Low Velocity Perforation of Mild Steel Plates, Trans. ASME, Journal of Pressure Vessel Technology, Vol. 130, No. 3, pp 031207-1 to 031207-11.
- Jones, N., Jouri, W. S. (1987), Scaling of Ship Collision and Grounding Damage, International Maritime Association of East Mediterranean, 4th Congress, Varna, Bulgaria, Vol. 3, pp.95.1-95.8.
- Booth, E., Collier, D., Miles, J, (1983), Impact Scalability of Plated Steel Structures, Structural Crashworthiness, Ed. N. Jones and T. Wierzbicki, Butterworths Publishers, London, pp. 136-174.
- McKown S., Cantwell W.J., Jones N. (2008), Investigation of Scaling Effects in Fiber – Metal Laminates, Journal of Composite Materials, Vol.42, No. 9, pp. 865-888.
- Yang F.J., Hassan M.Z., Cantwell W.J., Jones N. (2013), Scaling Effects in the Low Velocity Impact Response of Sandwich Structures, Composite Structures, Vol. 99, pp. 97-104.
- Hsu S.S., Jones N. (2004), Quasi-Static and Dynamic Axial Crushing of Thin-Walled Circular Stainless Steel, Mild Steel and Aluminium Alloy Tubes, International Journal of Crashworthiness, Vol. 9, No. 2, pp. 195-217.
- Jones N. (2005), Energy Absorption Effectiveness of Thin-Walled Structures Under Static and Dynamic Axial Crushing Loads, Impact Loading of Lightweight Structures, Ed. M. Alves and N. Jones, WIT Press, Boston and Southampton, pp. 273-287.
- Jones N. (2010), Energy-absorbing Effectiveness Factor, International Journal of Impact Engineering, Vol. 37, No. 6, pp.754-765.
- Jones N. (2018), Some Comments on the Energy-Absorbing Effectiveness Factor, Proceedings Institution of Mechanical Engineers, Journal of Mechanical Engineering Science, Vol. 232, No. 8, pp. 1433-1445.
- Jones N. (2013), The Credibility of Predictions for Structural Designs Subjected to Large Dynamic loadings Causing Inelastic Behaviour, International Journal of Impact Engineering, Vol. 53, pp. 106-114.
- Jones, N. (2017), Note on the Impact Behaviour of Fibre-Metal Laminates, International Journal of Impact Engineering, Vol. 108, pp. 147-152.
- Santiago R.C., Cantwell W.J., Jones N., Alves M. (2018), The Modelling of Impact Loading on Thermoplastic Fibre-Metal Laminates, Composite Structures, Vol. 189, pp.228-238.
- Schleyer G., Jones N. (2014), Undergraduate Laboratory Exercise on Impact Testing of Structures, International Journal of Mechanical Engineering Education, Vol. 42, No. 4, pp. 340-350.