ABSTRACT
We present the results of the geomorphological mapping of a region of the Dhofar (Sultanate of Oman) including two contrasting physiographic units sharing a common drainage system into the Arabian Sea: the Jebel Qara limestone massif and the coastal plain of Salalah. Neogene to Quaternary tectonic activity controlled the formation of an extensive system of faults and caused the uplift of the Jebel Qara, forming structural escarpments. The massif underwent karstification and subsequent linear erosion. Today the Jebel is cut by a dendritic net of dry valleys, occasionally dammed by calcareous tufa dams. The transition between the southern escarpment of the Jebel and the plain below displays flat alluvial fans, bordered by a strip of beachrock, coastal dunes, and coastal lagoons, located in correspondence to estuaries. Dramatic soil erosion is evident, linked to intense human-triggered zoogeomorphological processes started in the Mid-Late Holocene after the introduction of pastoral land-use.
1. Introduction
The landscape of Earth’s deserts is generally inherited from a variety of geomorphological processes acting since the Neogene (CitationEl-Baz, 1988; CitationGoudie, 2002; CitationKnight & Zerboni, 2018; CitationParsons & Abrahams, 2009). During the Quaternary, present-day arid lands underwent drastic environmental modifications caused by major shifts in rainfall regime (CitationNicholson, 2011). Subtropical arid regions of the Northern hemisphere, such as the Sahara, the deserts’ belt between the Levant and the Indus Valley, and the Arabian Peninsula, shifted many times from hyperarid deserts to savannah environments, and back to hyperaridity (CitationGoudie, 2002, Citation2013). Changes in precipitations rates influenced the activation and deactivation of geomorphological processes as well as their intensity (CitationGutiérrez, 2005; CitationThomas, 1997). Therefore, in present arid lands each physiographic unit bears the mark left by such variations. Sometimes the response to new climatic conditions was abrupt, sometimes was instead delayed due to specific geomorphological or hydrogeological settings (CitationCremaschi & Zerboni, 2009). Moreover, human adaptation and exploitation of desert regions also changed in response to new environmental changes (CitationNicoll, 2004; CitationNicoll & Zerboni, in press), further influencing landscape modifications.
Most of the deserts of the Old World occur in continental and tectonically stable regions. The intensity of major processes shaping the landscape (weathering as well as water and slope dynamics) is controlled mainly by local water availability. In contrast, the southern Arabian Peninsula is tectonically dynamic, and where local deserts meet the sea their evolution is controlled by the interplay between climate change, tectonics (uplift, faulting, and folding), and relative sea level change (CitationAl-Kindi, 2018; CitationEdgell, 2006). The coastal deserts of the Sultanate of Oman are therefore suitable places to investigate the evolution of landscapes as a response to endogenous and exogenous geomorphological processes, combined with long-lasting human exploitation. For these reasons, we selected an area in the Dhofar region of the southern Sultanate of Oman, which includes (i) the mountain massif of the Jebel Qara, and (ii) the coastal plain of Salalah (). The two physiographic units belong to the same hydrographic system draining into the Arabian Sea, and their multi-stratified landscape represents the result of natural and anthropogenic processes acting across the Quaternary under changing tectonic and climatic settings.
2. The study area: geography, geology, and climate
The Dhofar (south-western Sultanate of Oman) lies on the shores of the Arabian Sea, bordering eastern Yemen. The study area consists of two distinct physiographic settings: (i) the large coastal plain of Salalah abruptly ending against (ii) the step escarpment of the Jebel Qara limestone plateau (). The massif is the natural watershed separating the coastal plain and the Arabian Sea from the Nejd Desert.
Late Cretaceous to Neogene limestone strata form the Jebel Qara (Ministry of Petroleum and Minerals, Citation1987, Citation1988, Citation1992; Platel, Qidwai, & Khalifa, Citation1987; CitationKhalifa, 1988); they slope gently to the North and are crossed by a system of transtensional faults, mostly ENE–WSW and WNW–ESE oriented. The fault system has probably contributed to the lowering of the Salalah plain relative to the plateau and supported intensive erosion in the area; as a consequence, stepped escarpments border the Jebel to the North and to the South (CitationPlatel, Berthiaux, Le Metour, Beurier, & Roger, 1992). The escarpment reaches the maximum height of ∼850 m above sea level. The massif is dissected by a dendritic system of valleys cut into the bedrock. Intense soil loss left large outcrops of bare rock in the area. The coastal plain is flat in the surrounds of Salalah as a result of the coalescence of multiple alluvial fans into a mostly uniform surface, whereas in the eastern part of the region several terraces are present.
Climate data for the Pleistocene are scanty and mainly inferred by geomorphological observations. Several high- to medium-resolution stable isotope palaeoclimatic records from stalagmites and calcareous tufa have provided information on Holocene climatic fluctuations in southern Arabia (CitationBurns, Matter, Frank, & Mangini, 1998; CitationCremaschi et al., 2015, Citation2018; CitationFleitmann et al., 2003, Citation2004, Citation2007; Zerboni et al., Citationin press). In the Early-Middle Holocene, a rapid northward displacement in the latitudinal position of the summer Intertropical Convergence Zone (ITCZ) and the associated monsoonal belt promoted a large amount of rainfall. Conversely, since the Middle-Late Holocene monsoon precipitation decreased gradually in response to the lowering of the northern hemisphere summer insolation (CitationFleitmann et al., 2004). Today, the Dhofar is a semi-arid land with approximately 100–400 mm of rainfall per year (CitationKwarteng, Dorvlo, & Kumar, 2009). It is reached by the outer margin of the Indian summer monsoon that brings rainfall from the Indian Ocean between July and September (CitationFleitmann et al., 2004); most of the annual rainfall arrives in this season – locally called Kharīf. Due to the orographic effect, the southern part of the Jebel Qara receives heavier summer rainfall than the coastal plain of Salalah (CitationKhalifa, 1988; CitationRogers, 1980). Rainfall supports the groundwater supply to oases along the coast, and to shrubs and trees on the southern coastal side of the mountain, whereas its northern part is an arid steppe (CitationGalletti, Turner, & Myint, 2016; CitationRogers, 1980; CitationSale, 1980).
3. Human occupation
Evidence of Pleistocene human occupation in the coastal Dhofar is sparse. The Jebel and the Arabian seashore and coastline were more intensely settled since the Early Holocene by late Palaeolithic groups (CitationCharpentier, 2008; CitationCharpentier, Berger, Crassard, Borgi, & Béarez, 2014; CitationCremaschi & Negrino, 2002, Citation2005; CitationHilbert, 2014; CitationHilbert et al., 2015; CitationZarins, 2001). Geoarchaeological research in the Jebel Qara has brought to light an entire series of rock shelters, settled by the last Palaeolithic hunter-gatherers between ∼9500–8700 BCE (CitationCremaschi et al., 2015). Archaeological sites on the coastline of Hasik Bay (e.g. the Natif 2 Cave), were settled between ∼8700–7600 BCE by maritime hunter-gatherers (CitationCharpentier et al., 2014). The Neolithic period (∼8650–3500 BCE) is not represented in the Jebel Qara, and by contrast no occupation belonging to the Late Palaeolithic has yet been found in the Salalah Plain. This large coastal plain was regarded as unsettled during the Neolithic, but recent investigations discovered many Neolithic sites on the lower escarpments of the Dhofar hills (CitationNewton & Zarins, 2010; CitationZarins, 2013) and along the Arabian Sea shoreline, often appearing as impressive shell-middens (CitationMaiorano et al., 2018). Along the Salalah coast, at least two Neolithic sites associated with ancient lagoon deposits are preserved (CitationCharpentier et al., 2014).
In the region, the spreading of pastoralism started in the Bronze Age (∼3500–1000 BCE), and included the Jebel Qara and the Salalah coastal plain. Pastoral land-use is attested also in the Iron Age and Islamic times, up to recent years. Thanks to the trade of frankincense the city of Khor Rorī (also known as Sumhuram) flourished in the first centuries BCE and CE along the shoreline, becoming one of the main maritime centres of that time (CitationAvanzini, 2016). In Medieval times, the Dhofarian shoreline was a crucial node in the maritime commercial routes across the Indian Ocean between the Horn of Africa, the Arabian Peninsula, and India. In this phase, the city of Al-Balīd (ancient Salalah) grew and became one of the main harbours of southern Arabia (CitationZarins, 2013).
4. Methods
The geomorphological mapping of the region is based on data retrieved and interpreted from both remote sensing imaging and field observations, according to the approach proposed by several authors for mapping arid and semi-arid regions (CitationAzzoni et al., 2017; CitationHüneburg et al., 2019; CitationPerego, Zerboni, & Cremaschi, 2011; CitationZerboni, Perego, & Cremaschi, 2015). Validation of the desk-compiled map was done during several field surveys, which included a few kilometres of buffer beyond the northern watershed of the Jebel Qara. This was included in the map to guarantee a non-abrupt termination.
Observations from remote sensing came from several available datasets. Recent (08-02-2018, 26-09-2018, 15-12-2018) multispectral images (visible and infrared) with up to 10-metre spatial resolution from ESA Copernicus Satellite 2A (tiles T39QZU and T39QZV, source: https://scihub.copernicus.eu/) were combined in false colours (visible + near infrared). Images were then reprojected to UTM Zone 40 reference system and used as the reference background for remote observations in QGIS software.
An AW3D30 Digital Surface Model (DSM) with 1 arcsec horizontal resolution (∼30 m at the equator) released by the Japan Aerospace Exploration Agency (JAXA; source: https://www.eorc.jaxa.jp/ALOS/en/aw3d30/) was reprojected to UTM Zone 40 with 25-metre spatial resolution. Contour lines at 100 metres derived from this model were also used for landform interpretation. From the same model, auxiliary 10-metre contour lines for elevations between 0 and 100 m a.s.l. were calculated, as well as hillshade and slope models. We applied to the Digital Elevation Model (DEM) imported in QGIS an elevation dependent colour scale and superimposed it over a hillshade, in order to easily observe specific landforms such as escarpments and alluvial fans. The channel network was automatically extracted from the DEM using SAGA software. The results were reliable in the mountainous area, while the automatically-rendered model of the alluvial plain was manually corrected using satellite and field data.
Other than topographical and satellite visual information, 1:100,000 and 1:250,000 sheets of the Geological Map of the Sultanate of Oman (Ministry of Petroleum and Minerals, Citation1987, Citation1988, Citation1992) were used in the recognition and interpretation of landforms. High-resolution (0.5–1 m) natural colour satellite images provided from Google Earth and Microsoft Bing and visualised in QGIS and Google Earth Pro were used to identify and validate small-scale landforms.
5. Geomorphological setting
The region consists of two main physiographic units (Main Map): the plateau of the Jebel Qara to the north and, at the foot of its escarpment to the south, a plain which includes several very flat apron fans and the coastal plain. The escarpment separating the two units is complex, including high steps controlled by the geological structure (mostly faults) and in some cases related to downfaulted blocks (Platel, Qidwai et al., Citation1987; Platel et al., 1992).
The Jebel Qara massif is a limestone plateau deeply cut by an intricate system of dry valleys (wadis) mostly draining into the Arabian Sea. To the north, outside the limit of the Main Map, only a few minor wadis in the north-western part of the massive drain into the Empty Quarter/Rubi al-Khālī lowlands. The massif gently slopes towards the south with elevations ranging from between ∼400-500 m a.s.l. near the southern escarpment, and up to ∼1000 m a.s.l. at its northern watershed. Most of the top of the Jebel is heavily affected by surface erosion and soil loss; as a consequence, exposed outcrops of limestone and dolomitic limestone are common. Residual soil strips are present in the northern part of the region, where Early Holocene residual loess strata are also preserved (CitationCremaschi & Negrino, 2005). Colluvial accumulations of soil material are present in the most depressed inter-wadi areas of the top of the Jebel, often associated with sinkholes. The latter are related to ancient karst processes that shaped the underground karst system and likely influenced the evolution of the surface drainage network. Tufa dams and cascades are often located along the wadis’ bottom, where morphological steps of several tens of metres are present.
The southern margin of the Jebel Qara is confined by a system of escarpments between ∼300–400 m a.s.l., likely formed since the Neogene due to uplift (). Escarpments are more evident and continuous in the eastern part of the massif, whereas in its western part they are often interrupted and segmented by deep fluvial cuts. At the bottom of the escarpment, the transition belt toward the coastal plain, between ∼100–300 m a.s.l., is ∼1–2 km wide and consists of residual pediments and a narrow belt of talus. Large-scale slope movements are also present.
Figure 2. Field pictures of the (A) northern and (B) southern escarpments of the Jebel Qara, in (B) a residual portion of the pediment is visible on the left. (C) DEM and (D) GoogleEarth™ image of the DSGSD (arrows indicate unloading valleys and dotted line mark the trenches separating DSGSD from the massif).
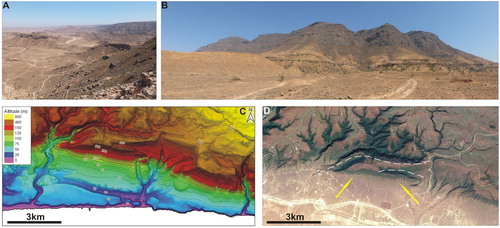
The alluvial/coastal plain extends from East to West, reaching a maximum width of ∼13 km in the vicinity of Salalah and decreasing at its sides to a minimum width of ∼3 km. The maximum elevation is 100 m a.s.l.. Several wadis originating in the Jebel Qara cross the plain. The lowlands surrounding Salalah can be divided into two main geomorphological units. The western and central parts of the area constitute an alluvial plain. Observations from the DEM allow the detection of at least two major alluvial fans accumulating from Wadi Jarsis and Wadi Nahiz (West and North of Salalah), and of a more complex system of coalescent apron fans () descending from minor wadis. The DEM also suggests the existence of several abandoned palaeochannels. Close to the escarpment and inside the plain, alluvial fans enclose parts of older pediments dated to the Miocene–Pliocene (CitationKhalifa, 1988; Platel, Rogers et al., Citation1987). To the west, the coastal belt is narrower and many rocky terraces are present. They possibly represent flattened surfaces cut at their front by later linear erosion; in some cases, flat surfaces are related to marine erosion and correspond to Pleistocene marine terraces. A ∼10 m high escarpment marks the transition from the terraces to the shoreline. A sandy beach forms the outer part of the coastal plain, sometimes with alignments of coastal dunes up to a few metres high. On the back of the coastal dunes, discontinuous outcrops of beachrock are present (CitationHoorn & Cremaschi, 2004). Several lagoons often related to estuaries are also present along the back-dune depressions.
6. Main geomorphological features
6.1. Structural landforms and escarpments
The low-angle monocline setting of the Jebel Qara is a result of intensive uplift in the area most likely since the late Palaeogene, in relation to the opening of the Gulf of Aden and the formation of parallel and conjugate systems of extensional and transtensional faults. Faults have also been mapped along the coastal plain. Related landforms are several structural escarpments that border the massif to the north and to the south (). Faults have a strong influence on the present day setting of the southern escarpment of the Jebel and downfaulted blocks have been mapped in the eastern part of the massif (Ministry of Petroleum and Minerals, Citation1987). Possibly, some of the bedrock blocks sliding down along the slope correspond to deep-seated gravitational slope deformations (DSGSD) (). In fact, they share some distinctive features with DSGSDs (CitationCrosta, Frattini, & Agliardi, 2013; CitationMariani, Cremaschi, Zerboni, Zuccoli, & Trombino, 2018): the presence of graben-like counterscarps and trenches at their top, and the formation of unloading valleys ot both sides.
6.2. Fluvial landforms
A dense network of sinuous to meandering, deeply incised wadis dissects the Jebel Qara in a moderately developed dendritic pattern (). The main channels are oriented towards the centre of the Salalah plain: NW–SE in the western part of the massif, N–S in the central part, and NE–SW or ENE–WSW in the eastern part. The structural control on the orientation and evolution of the branches of the wadis is evident from their prevailing ∼E–W orientation, the same of the fault and fracture systems indicated in geological maps (Ministry of Petroleum and Minerals, Citation1987, Citation1988) and detectable in the field. The drainage network is generally inactive aside from the rainfall season and immediately after. Occasionally, ponds can last for several months and extreme precipitations can cause huge flooding episodes (). The tectonic uplift of the Jebel Qara favoured the formation of deeply incised valleys, likely originated from the underground karst network and deepened by linear erosion after their collapse (see: CitationHill & Polyak, 2014; CitationNicod, 1997). The hypothesis of a karst-related evolution of the hydrographic net is confirmed by the occurrence of several large sinkholes along wadis; in a few cases, wadis drain into or are captured by sinkholes (). Also, the occurrence of tufa dams () along the main wadis in correspondence of deep steps is related to a tectono-karstic control over the drainage system (CitationBosak, Ford, Glazek, & Horacek, 1989). The wadi banks are deeply incised into the bedrock, forming narrow to wide canyons. Their bed is generally free of sediments apart from sparse gravel accumulations with the exception of the areas behind tufa dams, where the wadi bottom is flat and occupied by fluvial sediments and freshwater carbonate deposits ().
Figure 4. (A) GoogleEarth™ satellite image illustrating the dendritic and tectonic-controlled pattern of Wadi Darbat and its influents. (B) DEM and (C) GoogleEarth™ image of wadis flowing into the Teyq sinkhole. (D) Field pictures of the Teyq sinkhole.
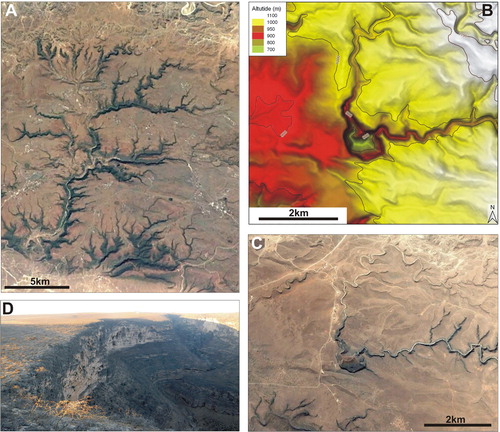
Figure 5. Examples of karst features: (A–C) rock shelters along Wadi Jinkarmat and Wadi Darbat; (D) a sinkhole on the top of the Jebel; (E) GoogleEarth™ image of two sinkholes; (F) aerial photo of the entrance of a sinkhole; (G) surface karst pits; (H) cone bearing limestone outcrops in planar layers; (I) silicified egg-shaped nodules on a residual surface of the massif.
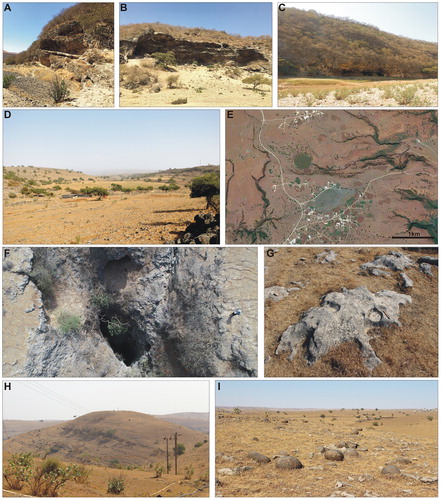
Figure 6. Examples of calcareous tufa: (A) the wadi Darbat tufa dam in the dry season and (B) after the Kharīf; (C) backfill of the Wadi Darbat dam; (D) GoogleEarth™ image of the Wadi Darbat dam (the dashed lines indicate the rim); (E-F) tufa dams and backfill along Wadi Ghidht and Wadi Arzat; (G–I) outcrops of Holocene calcareous tufa in rockshelters.
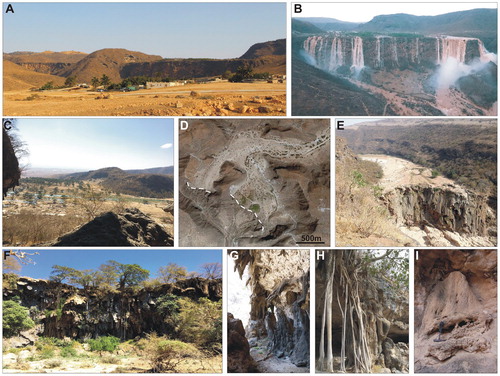
In the inner part of the coastal plain, wadis built a flat alluvial apron made of multiple alluvial fans (). They consist of gravel-dominated sediments and likely mostly formed during the Pleistocene, with only the oldest – nowadays dissected into pediments – dating to the late Neogene (CitationHoorn & Cremaschi, 2004; CitationPlatel et al., 1992). Today, wadis are cutting into these fans forming fluvial terraces: this evidence suggests that the lowering of their basal level occurred after their formation. The major wadis enter the Arabian Sea forming narrow to wide estuaries often related to lagoons or colonised by mangroves. In the case of Wadi Darbat – at Khor Rorī – two 30 m-elevated, flat top, rock promontories border the estuary.
6.3. Karst landforms and calcareous tufa
As mentioned in Section 6.2., karst is one of the main processes that acted on the Jebel Qara, deeply influencing the drainage network. Carbonate dissolution acting on the limestone massif triggered the formation of a complex endokarst system with many large- to small-scale surface landforms. The behaviour of the surface drainage network, which activates only after rainfall, confirms the existence of a karst hydrology, as well as the presence of many springs active throughout the year at the foot of the southern escarpment (CitationEdgell, 2006). Surface karst-related landforms include sinkholes and cones; bare rock surfaces often display the formation of a variety of forms of karren and dissolution pits. A peculiar karst feature is evident on nodular limestone surfaces: there, numerous spherical to egg-shaped siliceous nodules up to 1 m in diameter lay on the residual surface due to differential weathering ().
Many rock shelters and caves are located along the steep slopes in the southern part of the Jebel (CitationCremaschi et al., 2015); they are the result of underground dissolution and many of them can be interpreted as inland notches (CitationShtober-Zisu, Amasha, & Frumkin, 2015). Rock shelters are especially common along the Jenikermat and Darbat Wadis (), where they host characteristic natural and anthropogenic deposits dating to the Early and Middle Holocene and described in CitationCremaschi et al. (2015). Thick and complex deposits of calcareous tufa (flowstones, columns, stalactites, cement) occur within caves, overlying and cementing anthropogenic strata ().
The deposition of tufa occurred throughout the Quaternary (Platel, Qidwai et al. Citation1987): cascades and dams are among the most important features in the region. The latter act as barrages along wadis forming tufa-dammed lakes (CitationGoudie, 2013; CitationHoorn & Cremaschi, 2004). Tufa dams in this area possibly grew during subsequent phases of the Pleistocene, reaching remarkable dimensions: one of the largest, closing Wadi Darbat, is a ∼130 m-high, ∼700 m-long cliff ().
6.4. Coastal and marine landforms
A large belt of beachrock is located up to 3–4 m above the present-day sandy shoreline and separates the distal part of the alluvial apron from the coastline (CitationHoorn & Cremaschi, 2004). Khalifa (Citation1988) indicates a Pleistocene age for this formation, whereas on the basis of radiocarbon dating CitationPlatel et al. (1992) suggested that beachrock formed ∼35,000–32,000 years BCE. Beachrock are a crucial tool to reconstruct sea level changes (CitationMauz, Vacchi, Green, Hoffmann, & Cooper, 2015), and the available chronology needs to be confirmed by further investigation. A belt of coastal dunes forms a sand barrier between the beachrock and the Arabian Sea, allowing the formation of an elongated depression, cut by estuaries and mostly occupied by wetlands (). Estuarine wetlands are known as khor (without mangroves) or qurms (with mangroves) and are fed by fresh water from the mountains (); the beachrock is deeply incised by erosional valleys. The formation of sand barriers is controlled by seasonal winds. The interplay between SW summer monsoonal winds and NW winter winds leads to the destruction and reconstruction of the sand barrier, which in turn can close the estuaries at their mouths, thus controlling the input of saline water to wetlands (CitationHoorn & Cremaschi, 2004).
Figure 7. Examples of coastal and marine landforms: (A) GoogleEarth™ image and (B) field picture of the Khor Rori estuary and sand barrage, with marine terraces in the background; (C) coastal dunes; (D) DEM image illustrating a staircase of flat surfaces including marine terraces.
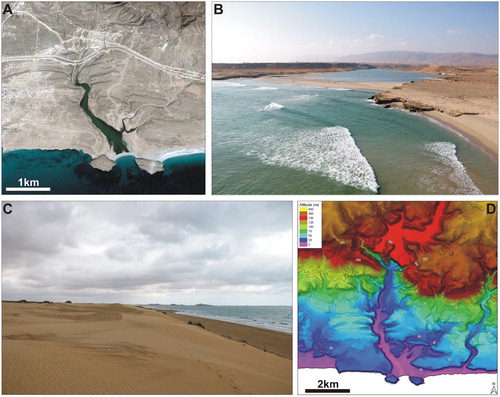
In the eastern part of the region, staircases of flat erosional surfaces connect the shore to the escarpment of the Jebel Qara. The DEM suggests the existence of several of these surfaces; a few of them can correspond to marine terraces (). The investigation of their geometry and age is still on-going, but their origin is certainly related to relative sea level change (CitationRovere, Stocchi, & Vacchi, 2016), thus to the combination of eustatic changes and recent uplift, as evident in other regions of the Sultanate of Oman (CitationHoffmann et al., 2020; CitationKusky, Robinson, & El-Baz, 2005).
6.5. Zoogeomorphological and anthropogenic landforms
Several small-scale landforms related to human (anthropogenic) and animal activities are present in the area. The latter are a result of zoogeomorphological processes (CitationButler, 1995) that in arid regions are particularly evident (CitationZerboni & Nicoll, 2019). Anthropogenic features include archaeological remains of ancient settlements on top of the Jebel Qara and along its wadis, and, more commonly, stone enclosures to corral stock. These features are very visible in high-resolution satellite imagery and consist of sub-rounded to complex areas surrounded by stonewalls in flat areas, with dark coprolite-bearing sediments accumulated inside (). Trails related to animal trampling are another common landform on the top of the plateau, as well as along talus and alluvial fans (). Enclosures and game trails are the consequence of a prolonged pastoral exploitation of the region. Termite mounds are a further and more striking evidence of zoogeomorphological processes (). 1–2 m high mounds are clustered in extensive fields distributed at the northern margin and in the eastern part of the Jebel, as well as along its escarpment and on top of alluvial fans.
Figure 8. Examples of zoogeomorphological and anthropogenic features: (A) GoogleEarth™ image of livestock enclosures on top of the Jebel Qara (notice the dark, dung-rich layer at the bottom of the stone structure); (B) enclosures along the slope of a cone; (C) GoogleEarth™ image of animal trails on the top of the Jebel; (D) field picture of animal trails along the northern margin of the Jebel; (E) field picture and (F) GoogleEarth™ image of a field of termite mounds (each withish spot is a mound) on the pediment at the southern margin of the Jebel Qara; (G) detail of the termite mounds in (F).
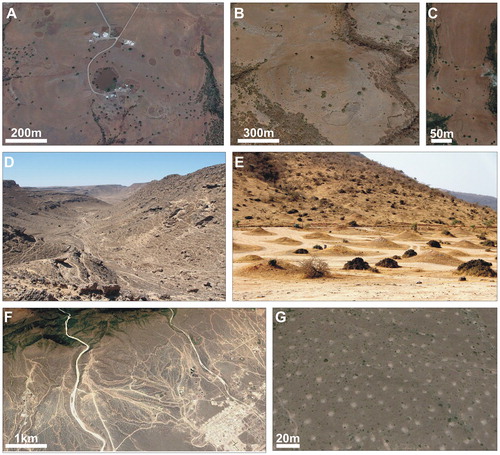
Pastoral features and termite mounds testify the influence of surface processes on soil loss in the region. Since the late prehistory human-animal agency (intense trampling) has enhanced soil loss processes, whereas enclosures have preserved residual strips of soil. Yet, termite mounds preserve at their base the pristine soil cover of the massif, thus representing animal agency contrasting soil erosion.
7. Conclusion: Quaternary evolution of the landscape
The geomorphological mapping of the area of the Jebel Qara and the coastal plain of Salalah highlights that their landscape is the result of geomorphological processes acting since the Neogene under different climatic conditions. The main drivers of landscape evolution are tectonics, which triggered the uplift of the Jebel and the formation of faults, and climate. Rainfall promoted extensive and deep limestone dissolution, linear erosion, the formation of large tufa deposits and the deposition of alluvial fans. The interplay between regional uplift and sea level change (CitationRovere et al., 2016) also played an important role in shaping the region along the shoreline of the Arabian Sea as in the case of marine terraces. Along the coastline, geomorphological and archaeological data (CitationHoorn & Cremaschi, 2004) suggest that lagoons formed and disappeared during at least the Late Quaternary, testifying significant changes in coastal environments and their exploitation.
From a geoarchaeological point of view, our work offers a key to interpret human exploitation of this part of Dhofar. The complexity of the landscape and the availability of natural resources of the Jebel Qara and Salalah plain attracted human communities since at least the Pleistocene/Holocene transition. Human occupation of the area applied over time a stronger pressure on the landscape and the transition to pastoral land-use, possibly under progressively more arid environmental conditions, enhanced zoogeomorphological processes and soil loss. As elsewhere (CitationArchaeoGLOBE Project, 2019; Regattieri et al., Citation2019), in the mid-late Holocene the human influence on the environment increased, and the human-induced overgrazing became a major surface process controlling the evolution of the landscape.
Software
QGIS 3.4 was used for the management of the mapping project in all phases. Specific terrain analysis tools were carried out in SAGA 2.3. Satellite images observation was done with the help of Google Earth Pro 7.3.
zerboni_et_al_Main_Map_revised.pdf
Download PDF (28.6 MB)Acknowledgments
We wish to thank the archaeological authorities of the Sultanate of Oman, the Salalah MHC branch, and its welcoming staff. We wish to thank Ali al-Ma’ashani for his help in the field and the people of Jebel Qara. We also thank A. Pavan for fruitful discussions. C. Pain and G. Hoffmann are kindly thanked for constructive comments on a previous version of the manuscript. This research was carried out as part of the French Archaeological Mission of the MEAE – Archaeology of the Arabian seashores.
Disclosure statement
No potential conflict of interest was reported by the authors.
Additional information
Funding
References
- Al Kindi, M. H. (2018). Land and life evolution in Oman: An 800 milion years story. Springer International Publishing.
- ArchaeoGLOBE Project . (2019). Archaeological assessment reveals Earth’s early transformation through land use. Science , 365 , 897–902. doi: 10.1126/science.aax1192
- Avanzini, A. (2016). By land and by sea. A history of South Arabia before Islam recounted from inscription . Roma : L’Erma di Bretschneider.
- Azzoni, R. S. , Zerboni, A. , Pelfini, M. , Garzonio, C. A. , Cioni, R. , Meraldi, E. , … Diolaiuti, G. A. (2017). Geomorphological map of Mount Ararat/Ağri Daği (Ağri Daği Milli Parki, Eastern Anatolia, Turkey). Journal of Maps , 13 , 182–190. doi: 10.1080/17445647.2017.1279084
- Bosak, P. , Ford, D. , Glazek, J. , & Horacek, I. (1989). Paleokarst – a systematic and regional review . Development in Earth Surface Processes 1. New York : Elsevier.
- Burns, S. J. , Matter, A. , Frank, N. , & Mangini, A. (1998). Speleothem-based paleoclimate record from northern Oman. Geology , 26 , 499–502. doi: 10.1130/0091-7613(1998)026<0499:SBPRFN>2.3.CO;2
- Butler, D. R. (1995). Zoogeomorphology: Animals as Geomorphic Agents . Cambridge, UK : Cambridge University Press.
- Charpentier, V. (2008). Hunter-gatherers of the ‘empty quarter of the early Holocene’ to the last Neolithic societies: Chronology of the late prehistory of south-eastern Arabia (8000–3100 BC). Proceedings of the Seminar for Arabian Studies , 38 , 59–82.
- Charpentier, V. , Berger, J. F. , Crassard, R. , Borgi, F. , & Béarez, P. (2014). Les premiers chasseurs-collecteurs maritimes d’Arabie (IXe-IVe millénaires avant notre ère). Archéologie des chasseurs-cueilleurs maritimes. De la fonction des habitats à l’organisation de l’espace littoral. Société Préhistorique Française , 6 , 345–365.
- Cremaschi, M. , Degli Esposti, M. , Fleitmann, D. , Perego, A. , Sibilia, E. , & Zerboni, A. (2018). Late Holocene onset of intensive cultivation and introduction of the Falaj irrigation system in the Salut oasis (Sultanate of Oman). Quaternary Science Reviews , 200 , 123–140. doi: 10.1016/j.quascirev.2018.09.029
- Cremaschi, M. , & Negrino, F. (2002). The frankincense road of Sumhuram: Palaeoenvironmental and prehistorical background. Khor Rori Report , 1 , 325–363.
- Cremaschi, M. , & Negrino, F. (2005). Evidence for an abrupt climatic change at 8700 14C yr B.P. in rockshelters and caves of Gebel Qara (Dhofar-Oman): Palaeoenvironmental implications. Geoarchaeology: An International Journal , 20 , 559–579. doi: 10.1002/gea.20068
- Cremaschi, M. , & Zerboni, A. (2009). Early to middle Holocene landscape exploitation in a drying environment: Two case studies compared from the central Sahara (SW Fezzan, Libya). Comptes Rendus Géoscience , 341 , 689–702. doi: 10.1016/j.crte.2009.05.001
- Cremaschi, M. , Zerboni, A. , Charpentier, V. , Crassard, R. , Isola, I. , Regattieri, E. , & Zanchetta, G. (2015). Early Holocene climate changes and pre-Neolithic human occupations as recorded in the cavities of Jebel Qara (Dhofar, southern Sultanate of Oman). Quaternary International , 382 , 264–276. doi: 10.1016/j.quaint.2014.12.058
- Crosta, G. B. , Frattini, P. , & Agliardi, F. (2013). Deep seated gravitational slope deformations in the European Alps. Tectonophysics , 605 , 13–33. doi: 10.1016/j.tecto.2013.04.028
- Edgell, H. S. (2006). Arabian deserts: Nature, origin and evolution . Dordrecht, The Netherlands : Springer.
- El-Baz, F. (1988). Origin and evolution of the desert. Interdisciplinary Science Reviews , 13 , 331–347. doi: 10.1179/isr.1988.13.4.331
- Fleitmann, D. , Burns, S. J. , Mangini, A. , Mudelsee, M. , Kramers, J. , Villa, I. , … Matter, A. (2007). Holocene ITCZ and Indian monsoon dynamics recorded in stalagmites from Oman and Yemen (Socotra). Quaternary Science Reviews , 26 , 170–188. doi: 10.1016/j.quascirev.2006.04.012
- Fleitmann, D. , Burns, S. J. , Mudelsee, M. , Neff, U. , Kramers, J. , Mangini, A. , & Matter, A. (2003). Holocene forcing of the Indian monsoon recorded in a stalagmite from southern Oman. Science , 300 , 1737–1739. doi: 10.1126/science.1083130
- Fleitmann, D. , Burns, S. J. , Neff, U. , Mudelsee, M. , Mangini, A. , & Matter, A. (2004). Palaeoclimatic interpretation of high-resolution oxygen isotope profiles derived from annually laminated speleothems from southern Oman. Quaternary Science Reviews , 23 , 935–945. doi: 10.1016/j.quascirev.2003.06.019
- Galletti, C. S. , Turner II B. L. , & Myint, S. W. (2016). Land changes and their drivers in the cloud forest and coastal zone of Dhofar, Oman, between 1988 and 2013. Regional Environmental Change , 16 , 2141–2153. doi: 10.1007/s10113-016-0942-2
- Goudie, A. S. (2002). Great warm deserts of the world. Landscapes and evolution . Oxford : Oxford University Press.
- Goudie, A. S. (2013). Arid and semi-arid geomorphology . Cambridge: Cambridge University Press.
- Gutiérrez, M. (2005). Climatic geomorphology . Amsterdam, The Netherlands : Elsevier.
- Hilbert, Y. H. (2014). Khashabian: A late Paleolithic industry from Dhofar, southern Oman . Oxford: Archaeopress.
- Hilbert, Y. H. , Parton, A. , Morley, M. W. , Linnenlucke, L. P. , Jacobs, Z. , Clark-Balzan, L. , … Rose, J. I. (2015). Terminal Pleistocene and Early Holocene archaeology and stratigraphy of the southern Nejd, Oman. Quaternary International , 382 , 250–263. doi: 10.1016/j.quaint.2015.02.053
- Hill, C. A. , & Polyak, V. J. (2014). Karst piracy: A mechanism for integrating the Colorado River across the Kaibab uplift Grand Canyon, Arizona, USA. Geosphere , 10 , 627–640. doi: 10.1130/GES00940.1
- Hoffmann, G. , Schneider, B. , Mechernich, S. , Falkenroth, M. , Dunai, T. , & Preusser, F. (2020). Quaternary uplift along a passive continental margin (Oman, Indian Ocean). Geomorphology , doi: 10.1016/j.geomorph.2019.106870
- Hoorn, C. , & Cremaschi, M. (2004). Late Holocene palaeoenvironmental history of Khawr Rawri and Khawr Al Balid (Dhofar, Sultanate of Oman). Palaeogeography, Palaeoclimatology, Palaeoecology , 213 , 1–36. doi: 10.1016/j.palaeo.2004.03.014
- Hüneburg, L. , Hoelzmann, P. , Knitter, D. , Teichert, B. , Richter, C. , Lüthgens, C. , … Luciani, M. (2019). Living at the wadi – integrating geomorphology and archaeology at the oasis of Qurayyah (NW Arabia). Journal of Maps , 15 , 215–226. doi: 10.1080/17445647.2019.1576068
- Khalifa, M. I. (1988). Geological map of Oman, 1:100,000 Sheet NE-40-9D Salalah – explanatory notes. Ministry of Petroleum and Minerals – Directorate General of Minerals, Muscat, Sultanate of Oman.
- Knight, J. , & Zerboni, A. (2018). Formation of desert pavements and the interpretation of lithic-strewn landscapes of the central Sahara. Journal of Arid Environments , 153 , 39–51. doi: 10.1016/j.jaridenv.2018.01.007
- Kusky, T. , Robinson, C. , & El-Baz, F. (2005). Tertiary–Quaternary faulting and uplift in the northern Oman Hajar mountains. Journal of the Geological Society of London , 162 , 871–888. doi: 10.1144/0016-764904-122
- Kwarteng, A. , Dorvlo, A. , & Kumar, G. T. (2009). Analysis of a 27-year rainfall data (1977–2003) in the Sultanate of Oman. International Journal of Climatology , 29 , 605–617. doi: 10.1002/joc.1727
- Maiorano, M. P. , Marchand, G. , Vosges, J. , Berger, J.-F. , Borgi, F. , & Charpentier, V. (2018). The Neolithic of Sharbithāt (Dhofar, Sultanate of Oman): Typological, technological, and experimental approaches. Proceedings of the Seminar for Arabian Studies , 48 , 219–233.
- Mariani, G. S. , Cremaschi, M. , Zerboni, A. , Zuccoli, L. , & Trombino, L. (2018). Geomorphological map of the Mt. Cusna Ridge (northern Apennines, Italy): Evolution of a Holocene landscape. Journal of Maps , 14 , 392–401. doi: 10.1080/17445647.2018.1480976
- Mauz, B. , Vacchi, M. , Green, A. , Hoffmann, G. , & Cooper, A. (2015). Beachrock: A tool for reconstructing relative sea level in the far-field. Marine Geology , 362 , 1–16. doi: 10.1016/j.margeo.2015.01.009
- Ministry of Petroleum and Minerals . (1987). Geological map of Oman, 1:100,000 Sheet NE-40-9E Marbat. Ministry of Petroleum and Minerals – Directorate General of Minerals, Muscat, Sultanate of Oman.
- Ministry of Petroleum and Minerals . (1988). Geological map of Oman, 1:100,000 Sheet NE-40-9D Salalah. Ministry of Petroleum and Minerals – Directorate General of Minerals, Muscat, Sultanate of Oman.
- Ministry of Petroleum and Minerals . (1992). Geological Map of Oman, 1:250,000 Sheet NE-40-9 Salalah. Ministry of Petroleum and Minerals – Directorate General of Minerals, Muscat, Sultanate of Oman.
- Newton, L. S. , & Zarins, J. (2010). Preliminary results of the Dhofar archaeological survey. Proceedings of the Seminar for Arabian Studies , 40 , 247–265.
- Nicholson, S. E. (2011). Dryland climatology . Cambridge : Cambridge University Press.
- Nicod, J. (1997). Les canyons karstiques ‘Nouvelles approches de problèmes géomorphologiques classiques’ (spécialement dans les domaines méditerranéens et tropicaux). Quaternaire , 8 , 71–89. doi: 10.3406/quate.1997.1563
- Nicoll, K. (2004). Records of recent environmental change and prehistoric human activity in Egypt and northern Sudan. Quat. Sci. Rev , 23 , 561–580. doi: 10.1016/j.quascirev.2003.10.004
- Nicoll, K. , & Zerboni, A. (in press). Is the past key to the present? Observations of cultural continuity and resilience reconstructed from geoarchaeological records. Quaternary International , doi: 10.1016/j.quaint.2019.02.012
- Parsons, A. J. , & Abrahams, A. D. (2009). Geomorphology of desert environments . Dordrecht, The Netherlands : Springer.
- Perego, A. , Zerboni, A. , & Cremaschi, M. (2011). The geomorphological map of the Messak Settafet and Mellet (central Sahara, SW Libya). Journal of Maps , 2011 , 464–475. doi: 10.4113/jom.2011.1207
- Platel, J. P. , Berthiaux, A. , Le Metour, J. , Beurier, M. , & Roger, J. (1992). Geological map of Juzor Al Halaaniyaat. Sheet NE 10-10. Ministry of Petroleum and Minerals – Directorate General of Minerals, Muscat, Sultanate of Oman.
- Regattieri, E. , Zanchetta, G. , Isola, I. , Zanella, E. , Drysdale, R. N. , Hellstrom, J. C. ,… Magrì, F. (2019). Holocene Critical Zone dynamics in an Alpine catchment inferred from a speleothem multiproxy record: Disentangling climate and human influences. Scientific Reports , 9 , 17829. doi: 10.1038/s41598-019-53583-7
- Platel, J. P. , Qidwai, H. A. , & Khalifa, M. I. (1987). Geological map of Oman, 1:100,000 Sheet NE-40-9E Marbat – Explanatory notes. Ministry of Petroleum and Minerals – Directorate General of Minerals, Muscat, Sultanate of Oman.
- Platel, J. P. , Rogers, J. , Peters, T. J. , Mercolli, I. , Kramers, J. D. , & Le Metour, J. (1987). Geological map of Salalah, 1:250,000 Sheet NE-40-9. Explanatory notes. Ministry of Petroleum and Minerals – Directorate General of Minerals, Muscat, Sultanate of Oman.
- Rogers, T. D. (1980). Meteorological records from the mountain region of Dhofar. The scientific results of the Oman flora and fauna survey 1977 (Dhofar). Journal of Oman Studies , Special Report 2 , 55–58.
- Rovere, A. , Stocchi, P. , & Vacchi, M. (2016). Eustatic and relative sea level changes. Current Climate Change Reports , 2 (4), 221–231. doi: 10.1007/s40641-016-0045-7
- Sale, J. B. (1980). The environment of the mountain region of the Dhofar. The scientific results of the Oman flora and fauna survey 1977 (Dhofar). Journal of Oman Studies , Special Report 2 , 17–24.
- Shtober-Zisu, N. , Amasha, H. , & Frumkin, A. (2015). Inland notches: Implications for subaerial formation of karstic landforms – an example from the carbonate slopes of Mt. Carmel, Israel. Geomorphology , 229 , 85–99. doi: 10.1016/j.geomorph.2014.09.004
- Thomas, D. S. G. (1997). Arid zone geomorphology . Chichester, UK : Wiley.
- Zarins, J. (2001). Dhofar: Land of incense. Archaeological work in the Governorate of Dhofar, Sultanate of Oman 1990. Archaeology and Cultural Heritage Series, Sultan Qaboos University Publications, Muscat.
- Zarins, J. (2013). Hailat Araka and the south Arabian Neolithic. Arabian Archaeology and Epigraphy , 24 , 109–117. doi: 10.1111/aae.12009
- Zerboni, A. , Degli Esposti, M. , Wu, Y.-I. , Brandolini, F. , Mariani, G. S. , Villa, F. , … Cremaschi, M. (in press). Age, palaeoenvironment, and preservation of prehistoric petroglyphs on a boulder in the oasis of Salut (northern Sultanate of Oman). Quaternary International , doi:10.1016/j.quaint.2019.06.040
- Zerboni, A. , & Nicoll, K. (2019). Enhanced zoogeomorphological processes in arid North Africa on the human-impacted landscape of the Anthropocene. Geomorphology , 331 , 22–35. doi: 10.1016/j.geomorph.2018.10.011
- Zerboni, A. , Perego, A. , & Cremaschi, M. (2015). Geomorphological map of the Tadrart Acacus massif and the Erg Uan Kasa (Libyan central Sahara). Journal of Maps , 11 , 772–787. doi: 10.1080/17445647.2014.955891