ABSTRACT
A 1:1,000,000 map of the Irish Sea, within the Irish Economic Exclusion Zone, is presented highlighting the spatial distribution of potential geological and geotechnical constraints to offshore wind energy development. In this mapping exercise we incorporated existing multibeam echosounder bathymetric and backscatter data collected by the Integrated Mapping for the Sustainable Development of Ireland’s Marine Resource programme. ArcGIS was used to interrogate the bathymetric data and produce maps for seabed morphological characteristics. Backscatter data and QTC Multiview derived sediment classification was used in conjunction with data from the literature to link sediment distribution with sediment transport pathways and to assess the possible impact on infrastructure. The result is a spatial constraints map, which may be used by developers, consultants and marine spatial planning authorities alike to help site projects and plan de-risking site investigations.
1. Introduction
With an energetic wind regime and relatively shallow water depths, the Irish Economic Exclusion Zone (EEZ) within the Irish Sea and its approaches are considered suitable for the economical development of offshore wind generation. The Irish EEZ of the Irish Sea has been technically recognised as being able to support up to 4.8 gigawatts (GW) of fixed offshore wind ‘without any likely significant adverse effects on the environment’ (CitationDepartment of Communications Energy and Natural Resources [DCENR], 2014). More recently, under Action 25 of its Climate Action Plan 2019, the Irish Government has set a target of 3.5 GW of electricity from offshore wind sources by 2030 (CitationDepartment of Communications Climate Action and Environment [DCCAE], 2019). Moreover, Under Action 26 of the Plan, the Irish Government has promised to support emerging marine technologies, including exploring for test locations (CitationDCCAE, 2019). In this context, however, to date only one offshore windfarm has been developed in the Irish sector of the Irish Sea; Arklow Bank, a 25 megawatt (MW) project constructed in 2004 on a sand bank. Correspondingly, almost 2.7 GW of offshore wind has been successfully installed in the UK sector of the Irish Sea. However, a number of offshore wind projects in the UK sector have encountered adverse geological ground conditions which have resulted in their discontinuation (e.g. Celtic Array: CitationMellet et al., 2015). Therefore, as Ireland looks to further develop its offshore wind capacity, understanding the seabed sediments and sub-surface structure regarding siting of offshore wind energy devices is part of the first stage assessment towards a sustainable, national marine energy development strategy.
Water depth and seabed geology are key considerations for the feasibility of offshore wind installations (CitationBarrie & Conway, 2014; CitationBosch et al., 2018; CitationCavazzi & Dutton, 2016; CitationCradden et al., 2016; CitationLe et al., 2014). Since 2003, the Integrated Mapping for the Sustainable Development of Ireland’s Marine Resource (INFOMAR) programme, a joint venture between the Geological Survey of Ireland (GSI) and The Irish Marine Institute (MI), has been collecting a variety of geophysical and groundtruthing data in the Irish Sea. This high-resolution and spatially extensive dataset allows for a regional assessment of seabed conditions pertinent to offshore renewable energy development.
The objective of this study is to spatially map the key seabed characteristics and sedimentary processes affecting potential offshore wind deployment in the Irish EEZ of the Irish Sea. To do so, we utilise the existing, high-quality geological and geophysical dataset gathered by the INFOMAR programme and apply detailed analysis to spatially constrain the geological and geotechnical constraints that need to be considered as part of any future site evaluation for offshore renewable energy development.
2. Materials and methods
Approximately 8200 km2 of multibeam echosounder (MBES) data used in this study were acquired during 36 separate surveys in the Irish Sea and its approaches from 2003 to 2014 (; Inset, Main Map). The Kongsberg Simrad 3002D system was predominately used. The Simrad 3002D system allowed the acquisiton of bathymetric and backscatter data in the 300 kHz range using dynamically focused beams. The horizontal accuracy (x, y) was usually <50 cm with a vertical accuracy (z) of <15 cm obtained for the processed bathymetry data. The Simrad EM1002 system was also used for multibeam echosounder data acquisition, operating at frequencies between 93 and 95 kHz. Data processing was typically performed on board with the CARIS HIPS and SIPS software package to remove erroneous pings and correcting for tides. Data were accessed and downloaded through the INFOMAR Interactive Web Data Delivery System (IWDDS).
Figure 1. Extent of INFOMAR MBES bathymetry data used in this study (A) and same data gradated to 0–40 m (shallow), 40–60 m (transitional) and >60 (deep) representing preferred ranges for technology types (B).
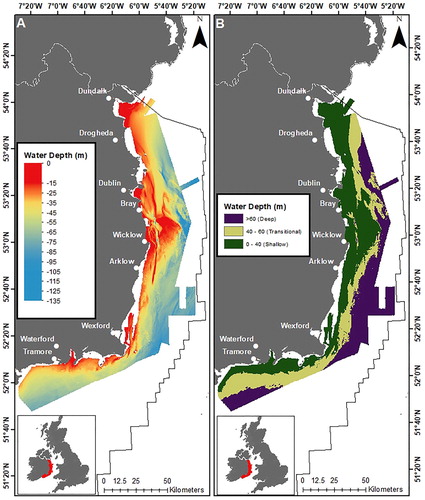
Whilst the bathymetric datasets derived from MBES are easily manged and merged to form a coherent dataset, the inherent differences in collection between backscatter datasets (e.g. different vessels, different MBES equipment, different MBES settings) meant that generating coherent backscatter reflectivity (db) value levels is difficult (F. Sachetti, pers. Comm.). Similarly, given the large area involved in this study, it was unrealistic to use individual data grids in any meaningful way. However, it is possible to apply static db value shifts to backscatter layers in order to match one another and thus help standardise them, making the various surveys as similar as possible and produce a dataset that is useable. The Marine Institute have done this using Geocoder software and the resulting dataset was requested from them and used in this study. Backscatter and sediment classification data were accessed and downloaded through the INFOMAR Web Map Service (WMS) using the ArcGIS REST Services Directory.
2.1. Multibeam echosounder data
A total of 36 raster tiles were used to build a MBES bathymetry mosaic. Given that data were collected by different vessels and multibeam echosounder acquisition systems and gridded to different resolutions, data needed to be at a common resolution (cell size) before it could be combined. Therefore, raster tiles were re-sampled to a 5 m cell size using the ‘Resample Tool’ in the ArcGIS Data Management Toolbox. Once all raster tiles had been re-sampled, they could then be combined into a single, seamless file using the ‘Mosaic to New Raster’ tool in the ArcGIS Data Management Toolbox ((A)). A gradated dataset was also generated to assess areas for suitable foundations with respect to wind turbine installations based on water depth categories from CitationBosch et al. (2018) ((B)). Water depths of 0–40 m were considered ‘shallow’ and suitable for monopile, mono-bucket (suction bucket) and multi-pile (tripod and jacket). Depths of 40–60 m were classified as ‘transitional’ and preferential for multi-pile and gravity base structures (GBS). Water depths greater than 60 m were considered ‘deep’ and likely to be more suited to floating technologies.
2.2. Bathymetric derivatives
The bathymetry mosaic grid was used to generate several additional parameters and gridded datasets. Using IVS Fledermaus rugosity was derived to measure surface roughness and terrain variability (). Within ArcGIS a slope angle dataset was derived using the Spatial Analyst Tools. Slope data were used to and assess where areas may exceed critical thresholds for certain foundation types, such as gravity bases. In this study greater than 5° was considered a significant seabed gradient (CitationGavin and Doherty Geo-Solutions, 2012; ).
Figure 2. Parameters derived from bathymetric data: (A) rugosity and (B) slope greater than significant gradient threshold (i.e. > 5°).
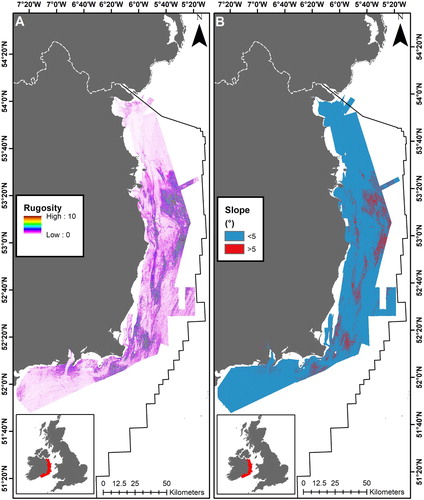
The Benthic Terrain Modeler (BTM) is a plug-in extension for ArcGIS that be used to calculate fine- and broad-scale Bathymetric Position Indices (BPI) (CitationWalbridge et al., 2018). BPI can be used to define the elevation of a particular location relative to the overall grid area. Therefore, it is a useful tool in defining positive topographic features like banks, as well as negative topographic features (e.g. troughs and channels). In this study, the broad-scale BPI was calculated with an inner search radius of 25 m and an outer search radius of 250 m giving a scaling factor of 1000 (). The fine-scale BPI was calculated using an inner search radius of 3 m and an outer search radius of 25 m to give a scaling factor of 100 (). These datasets were then standardised to allow for easier comparison of outputs.
2.3. Backscatter and sediment classification
Backscatter was used in conjunction with bathymetry data and automated sediment classification to map sediment distribution based on acoustic response, seabed morphology and sediment samples. With backscatter data, the intensity of the return signal can be indicative of a soft (low response and, therefore, a light colour) or a hard (high return, dark colour) substrate (). It can be used to infer sediment type and distribution between sample points.
Figure 4. Backscatter (A) and sediment classification (B) data available from INFOMAR used in this study.
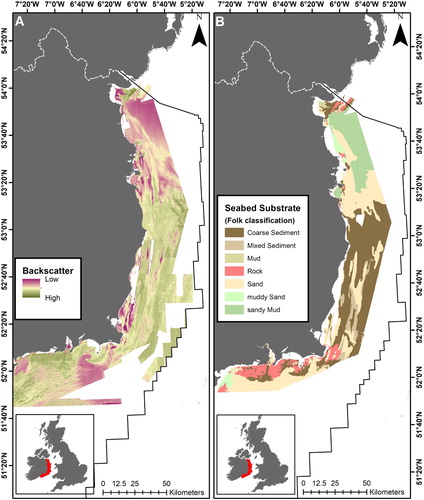
Acoustic classes corresponding to different sediment types were extracted from the backscatter data using various methods: image segmentation, isoclustering and manual tracings. Acoustic classes were classified into seabed substrate types using sediment sample data. A modified classification scheme was used for the sediment classification based on CitationFolk (1954) ().
3. Results and discussion
3.1. Seabed Morphology
The BPI for the Irish Sea proved useful in defining areas of flatness, elevation and depression (). These areas could then be classified as morphological features according to the classification system of CitationDove et al. (2016). Areas of flatness are generally found in the northern part of the Irish Sea and correspond with the end of the dominant transport pathways where fine sediment is deposited (, and ). Contrasting areas of elevation and depression are found in the southern part of the Irish Sea, and its approaches, where there are positive features, such as banks and rock outcrops, as well as negative features including scour pits and channels (or tunnel valleys). The crests and troughs of individual sediment waves form positive and negative features respectively. This proved useful in mapping geomorphic features which may act as constraining factors for offshore wind energy deployment due to sediment migration or adverse ground conditions ( and ). Offshore wind farms are often sited on stable sand banks where water depth is shallow.
3.2. Sediment distribution and processes
Where bed-stresses at or near the seafloor interact with unconsolidated sediments they can be mobilised when critical sediment thresholds, controlled primarily by sediment grain-size, are exceeded. These bed-stresses are driven by a combination of water depth and the hydrographic regime. In the Irish Sea the hydrographic regime is controlled by tidal energy, along with seasonal stratification of the water column and strong winds. Tides enter the Irish Sea from the North Channel and St. Georges Channel to the south and where they meet a degenerate amphidrome is formed in the south Irish Sea, off Wicklow. Tidal ranges increase in opposite directions, north and south, from this point and bedload parting zones have been observed where there are divergent patterns in sediment transport direction (CitationHolmes & Tappin, 2005; CitationPingree & Griffiths, 1979; CitationRobinson, 1979; CitationVan Landeghem et al., 2009) (). The magnitude of migration tends to dissipate away from these bedload zones with the highest bedform migrations rates (up to 35 m/a−1 on average), recorded in the central part of the Irish Sea in an area dominated by coarse-lag deposits and sediment wave fields (CitationVan Landeghem et al., 2012, Citation2009; CitationWard et al., 2015) (). These areas correspond with areas of high rugosity (). There are well established pathways that transport sediment northward where it is deposited in an area of low bed-stress, forming a large sediment patch of sandy Mud to Sand grade material (). This area of fine sediment accumulation forms a relatively flat area of elevation referred to as the Western Irish Sea Mud Belt (WISMB) (CitationBelderson, 1964; CitationHolmes & Tappin, 2005; CitationJackson et al., 1995; CitationWard et al., 2015) (). As a result, Irish Sea sediments consists of a mosaic of reworked glacial and post-glacial sediments which form a range of grain-size classes (CitationDobson et al., 1971; CitationJackson et al., 1995; CitationWard et al., 2015).
Figure 6. Seabed sediment distribution in the Irish Sea (modified CitationFolk (1954) classification) with superimposed morphological features (according to CitationDove et al., 2016), sediment wave direction (CitationVan Landeghem et al., 2009), bedload parting zones (CitationVan Landeghem et al., 2009) and indicative, average bedform migration rates (CitationVan Landeghem et al., 2012).
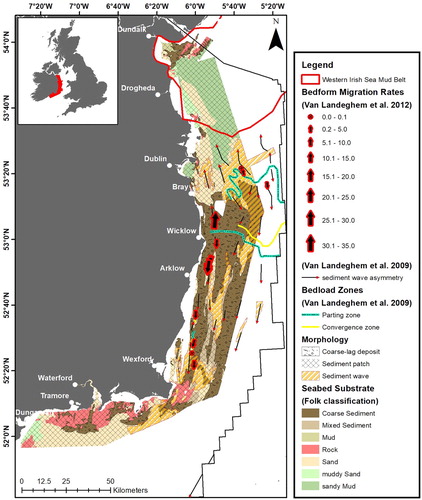
3.3. Geological and geotechnical constraints
The shallow geology of the seabed can offer significant constraining factors to the installation and subsequent stability of offshore infrastructure, like wind turbine foundations (CitationCotterill et al., 2017; CitationMellet et al., 2015). In addition, active seabed processes can adversely impact these structures, such as the removal of sediment from around foundations causing instability (i.e. scour). To determine the primary constraints, and so inform mapping, a comprehensive review of literature related to Irish Sea Quaternary geology and industry best-practice related to siting offshore infrastructure was undertaken. Subsequently, a summary checklist of geological characteristics, processes and their potential constraining properties was adopted from CitationMellet et al. (2015) ().
Table 1. Geological features creating possible geotechnical constraints in the Irish Sea (after CitationMellet et al., 2015)
Previously, as part of the Offshore Renewable Energy Development Plan (CitationDCENR, 2014), the Irish Sea and its approaches was classified into three assessment areas: East Coast (North), East Coast (South) and South Coast. Each one was technically assessed for its potential to support offshore wind development in terms of gigawatts (GW) that could be deployed. Using , geological and geotechnical constraint areas in the Irish Sea were delineated, digitised and overlain with the OREDP assessment zones to indicate the main engineering and siting challenges in each zone ( and Main Map).
Figure 7. Geological and geotechnical constraints mapped in the Irish Sea superimposed on OREDP assessment areas.
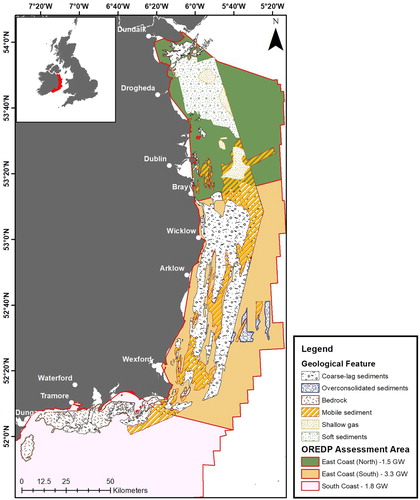
3.3.1. Soft sediments
Soft sediments are predominately found in the area in the north Irish Sea within the Western Irish Sea Mud Belt (CitationBelderson, 1964; CitationJackson et al., 1995) ( and ). Here, fine-grained sediments, referred to as the Mud Facies by CitationJackson et al. (1995), have been accumulating since the end of the last glaciation and form thicknesses of up to 40 m overlying glacial deposits (CitationCoughlan et al., 2019; CitationJackson et al., 1995; CitationWoods et al., 2019). These sediments are typically normally-consolidated, with low undrained shear strengths of up to 20 kPa (CitationCoughlan et al., 2019). Such sediments, in significant thickness, are unlikely to support traditional monopile solutions. The Mud Facies has also been known to host gas, often leading to surface pockmark formation generating seabed instability (CitationBelderson, 1964; CitationCoughlan et al., 2019; CitationCroker et al., 2005; CitationYuan et al., 1992).
3.3.2. Coarse-lag sediments
Coarse-lag sediments can represent areas where glacial sediments, exposed at the surface, have been reworked by modern wave and current processes removing finer material through winnowing. Often, the upper part of these deposits can be mobile with underlying layers forming a hard substrate that is difficult to penetrate. These upper sediments are Holocene in age, but the underlying units are typically classified as the Upper Till member and the Chaotic Facies of CitationJackson et al. (1995). The former is a glacial till that comprises sediment up to boulder class, whilst the Chaotic Facies is interpreted as glacial outwash sediments, predominately gravels, with muds, sands and cobbles (CitationCoughlan et al., 2019; CitationJackson et al., 1995).
3.3.3. Mobile sediment
The mobilisation of seabed sediments can affect the stability of offshore wind turbines and associated infrastructure in several ways. Sediment that is mobile in the form of migrating bedforms, such as sediment waves or sediment banks, can significantly change seabed-level. This change in seabed-level can either bury infrastructure or, in the case of connecting electrical cables, cause ‘free-spanning’ whereby seabed-level is lowered, and cables hang critically un-supported. Similarly, the removal of sediment in the immediate vicinity of wind turbine foundations by seabed erosion, or ‘scour’, can cause instability of the structure. Such issues were recorded at Ireland’s only offshore wind farm; Arklow Bank (CitationWhitehouse et al., 2011). Therefore, understanding seabed sediment and bedform migration patterns are is important to selecting sites that are not adversely affected over the lifetime of a project (CitationMellet et al., 2015)
3.3.4. Shallow gas
Gas in unconsolidated sediments is readily identified on seismic profiles as acoustic turbidity, acoustic blanking and enhanced reflection (CitationJudd & Hovland, 1992). Examples of these have been recorded throughout the Irish Sea (CitationBelderson, 1964; CitationCoughlan et al., 2019; CitationCroker, 1995; CitationCroker et al., 2005; CitationJackson et al., 1995; CitationJones et al., 1986; CitationPantin, 1977; CitationVan Landeghem et al., 2015; CitationYuan et al., 1992). Often gas migrates to the seabed from deeper sources (up to km below the surface) where it can form gas escape structures (e.g. pockmarks) which destabilise the seabed (CitationYuan et al., 1992). Alternatively, in sandier conditions, seeping gas can precipitate carbonate minerals at the seabed forming methane-derived authigenic carbonate which cements the seafloor forming hard substrates (CitationJudd et al., 2019; CitationO’Reilly et al., 2014; CitationVan Landeghem et al., 2015).
3.3.5. Over-consolidated sediments
The advance of the Irish Sea Ice Sheet during the last glaciation deposited a layer of glaciogenic sediments, or till, typically directly on to bedrock (CitationJackson et al., 1995; CitationÓ Cofaigh & Evans, 2001). This unit, referred to as the Upper Till member by CitationJackson et al. (1995), has been overridden by ice-loading and is often over-consolidated exhibiting undrained shear strength values of, on average, 185 kPa in the eastern Irish Sea (CitationMellet et al., 2015) and 148 kPa in the north Irish Sea (CitationCoughlan et al., 2019). The heterogeneous nature of the Upper Till, comprising clasts from sand to boulder-size, and its over-consolidated nature would prove difficult for foundation piling.
3.3.6. Bedrock
The implications of variable bedrock levels have already been demonstrated at existing projects, such as Celtic Array, which was ultimately cancelled. Where bedrock is exposed at the surface it can form a surface which is difficult to pile (CitationMellet et al., 2015). In this study, bedrock was typically found exposed close to shore, in shallow water depths () The presence of a hard, stable substrate like bedrock may favour wind turbine foundation types such as gravity base (CitationOh et al., 2018)
4. Summary
Previous technical assessments of the Irish EEZ in the Irish Sea as part of the OREDP have suggested the strong potential for offshore wind. The Main Map outlines the spatial distribution of some of the key geological features and processes which may constrain the future deployment of offshore wind energy in these areas. The East Coast (North) area is at the end of an active sediment transport route, and is an area where sediment is predominately being deposited rather than eroded (). As a result, there are significant (40 m) accumulations of soft sediments that offer a potential geotechnical constraint (). These sediments can also host gas ().
The East Coast (South) has a variable morphology consisting of sediment banks and bathymetric deeps (). Banks offer potentially shallow sites for construction, but are subject to morphodynamic change, whereas the deeps may offer future sites, previously unconsidered, for floating technology that are close to shore. However, the area is highly dynamic with mobile sediments, migrating bedforms and coarse-lag deposits which will need to be assessed for scour potential and seabed-level change ().
Figure 8. Geomorphological classification of the Irish Sea INFOMAR data using the classification scheme of CitationDove et al. (2016).
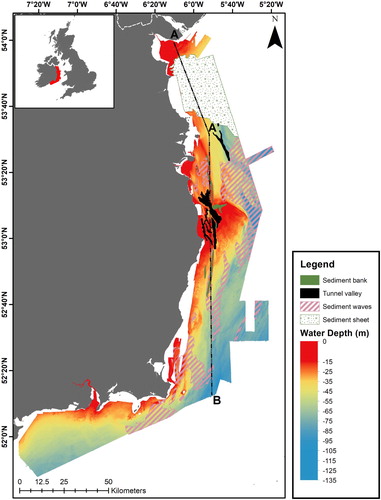
The South Coast area assessed here is constrained by exposed bedrock close to shore and mobile sediment adjacent (). Water depth drops off significantly here, making it more conducive to floating wind technology ().
Seabed characterisation is a critical component of engineering design of offshore wind turbines, as well as environmental impact studies. A full geological and geotechnical classification of the Irish Sea is difficult to establish since this will vary significantly according to the type of renewable energy structure being considered and the localised seabed conditions. The data used in this study provides a snapshot of the seabed at the time of collection and shows a highly dynamic environment with a significant degree of lateral and vertical heterogeneity in terms of seabed structure. It highlights the current understanding of geological conditions in the Irish EEZ in the Irish Sea and is recommended for use as a guide and should not replace a detailed site investigation. Overall, the water depths and seabed conditions in the Irish Sea are conducive to all of the various fixed substructure currently available for wind turbines as well as floating wind technology (CitationBosch et al., 2018; CitationOh et al., 2018). It is intended that this study and map may be used as part of any seabed zonation exercise carried out by the Irish Government in the marine spatial planning process. By doing so, it can significantly reduce the cost of future offshore wind deployment by helping to de-risk site selection and development.
Software
Bathymetric data were interrogated and interpreted using ESRI ArcGIS v10.6. Slope data were generated using the ESRI ArcGIS Spatial Analyst toolbox. Rugosity data were generated using IVS Fledermaus. Bathymetry Position index (BPI) data were generated using the ESRI ArcGIS toolbox ‘Benthic Terrain Modeler’. Digitisation and final data integration and map production were carried out using ESRI ArcGIS v10.6.
Supplemental Material
Download PDF (1.7 MB)Acknowledgements
This paper has emanated from research supported in part by a research grant from Science Foundation Ireland (SFI) under Grant Number 13/RC/2092 and is co-funded under the European Regional Development Fund and by iCRAG industry partners. The data used were collected as Irish Public Sector Data (INFOMAR) by the Geological Survey of Ireland (GSI) and the Marine Institute and licensed under a Creative Commons Attribution 4.0 International (CC BY 4.0) licence. The authors would like to thank Dr. Fabio Sacchetti (Marine Institute) for useful discussions on the data. The authors would like to thank reviewers Sophie Ward and John Abraham, as well as Associate Editor Jasper Knight, for constructive criticism and useful comments which improved the manuscript and map presentation.
Disclosure statement
No potential conflict of interest was reported by the author(s).
Additional information
Funding
References
- Barrie, J. V. , & Conway, K. W. (2014). Seabed characterization for the development of marine renewable energy on the Pacific margin of Canada. Continental Shelf Research , 83 , 45–52. https://doi.org/10.1016/j.csr.2013.10.016
- Belderson, R. H. (1964). Holocene sedimentation in the western half of the Irish Sea. Marine Geology , 2 (1-2), 147–163. https://doi.org/ 10.1016/0025-3227(64)90032-5
- Bosch, J. , Staffell, I. , & Hawkes, A. D. (2018). Temporally explicit and spatially resolved global offshore wind energy potentials. Energy , 163 , 766–781. https://doi.org/10.1016/j.energy.2018.08.153
- Cavazzi, S. , & Dutton, A. G. (2016). An offshore wind energy geographic information system (OWE-GIS) for assessment of the UK’s offshore wind energy potential. Renewable Energy , 87 , 212–228. https://doi.org/10.1016/j.renene.2015.09.021
- Cotterill, C. , Phillips, E. , James, L. , Forsberg, C. F. , & Tjelta, T. I. (2017). How understanding past landscapes might inform present-day site investigations: A case study from Dogger Bank, southern central North Sea. Near Surface Geophysics , 15 (4), 403–414. https://doi.org/10.3997/1873-0604.2017032
- Coughlan, M. , Wheeler, A. J. , Dorschel, B. , Long, M. , Doherty, P. , & Mörz, T. (2019). Stratigraphic model of the quaternary sediments of the Western Irish Sea Mud Belt from core, geotechnical and acoustic data. Geo-Marine Letters , 39 (3), 223–237. https://doi.org/ 10.1007/s00367-019-00569-z
- Cradden, L. , Kalogeri, C. , Barrios, I. M. , Galanis, G. , Ingram, D. , & Kallos, G. (2016). Multi-criteria site selection for offshore renewable energy platforms. Renewable Energy , 87 , 791–806. https://doi.org/10.1016/j.renene.2015.10.035
- Croker, P. F. (1995). Shallow gas accumulation and migration in the western Irish Sea. In P. Croker , & P. Shannon (Eds.), The petroleum geology of Ireland’s offshore basins (pp. 41–58). Geological Society Special Publication No. 93. https://doi.org/10.1144/GSL.SP.1995.093.01.07
- Croker, P. F. , Kozachenko, M. , & Wheeler, A. J. (2005). Gas-related seabed structures in the Western Irish Sea. January 2005 Tech Rep Strategic Environmental Assessment of the Irish Sea (SEA6), UK Department of Trade and Industry.
- Department of Communications Climate Action and Environment . (2019). Climate Action Plan 2019: To tackle climate breakdown. https://doi.org/10.5860/choice.46-0890
- Department of Communications Energy and Natural Resources (DCENR) . (2014). Offshore Renewable Energy Development Plan (Issue February). https://www.dccae.gov.ie/en-ie/energy/topics/Renewable-Energy/electricity/offshore/offshore-renewable-energy-development-plan-/Pages/Offshore-Renewable-Energy-Development-Plan.aspx .
- Dobson, M. R. , Evans, W. E. , & James, K. H. (1971). The sediment on the floor of the southern Irish Sea. Marine Geology , 11 (1), 27–69. https://doi.org/ 10.1016/0025-3227(71)90083-1
- Dove, D. , Bradwell, T. , Carter, G. , Cotterill, C. , Gaferia, J. , Green, S. , Krabbendam, M. , Mellet, C. , Stevenson, A. , Stewart, H. , Westhead, K. , Scott, G. , Guinan, J. , Judge, M. , Monteys, X. , Elvenes, S. , Baeten, N. , Dolan, M. , Thorsnes, T. , … Ottesen, D. (2016). Seabed Geomorphology: A two-part classification system. https://doi.org/10.1111/j.1365-2656.2009.01617.x
- Folk, R. L. (1954). The Distinction between grain size and mineral composition in sedimentary-rock nomenclature. The Journal of Geology , 62 (4), 344–359. http://www.jstor.org/stable/30065016 doi: 10.1086/626171
- Gavin and Doherty Geo-Solutions . (2012). Foundation risk & geotechnical uncertainty mapping for future Offshore Wind Farm Developments “D3: Final Report”. https://maps.marine.ie/infomarData/researchmap/reports/2011/INF-11-24-DOH.pdf
- Holmes, R. , & Tappin, D. R. (2005). DTI Strategic Environmental Assessment Area 6, Irish Sea, seabed and surficial geology and processes, British Geological Survey Commissioned Report, CR/05/057.
- Jackson, D. I. , Jackson, A. A. , Evans, D. , Wingfield, R. T. R. , Barnes, R. P. , & Arthur, M. J. (1995). United Kingdom offshore regional report: The geology of the Irish Sea . British Geological Survey.
- Jones, G. B. , Floodgate, G. D. , & Bennell, J. D. (1986). Chemical and microbiological aspects of acoustically turbid sediments: Preliminary investigations. Marine Geotechnology , 6 (3), 315–332. https://doi.org/10.1080/10641198609388193
- Judd, A. , Noble-James, T. , Golding, N. , Eggett, A. , Diesing, M. , Clare, D. , Silburn, B. , Duncan, G. , Field, L. , & Milodowski, A. (2019). The Croker carbonate slabs: Extensive methane-derived authigenic carbonate in the Irish Sea—nature, origin, longevity and environmental significance. Geo-Marine Letters , https://doi.org/10.1007/s00367-019-00584-0
- Judd, A. G. , & Hovland, M. (1992). The evidence of shallow gas in marine sediments. Continental Shelf Research , 12 (10), 1081–1095. https://doi.org/ 10.1016/0278-4343(92)90070-Z
- Le, T. M. H. , Eiksund, G. R. , Strøm, P. J. , & Saue, M. (2014). Geological and geotechnical characterisation for offshore wind turbine foundations: A case study of the Sheringham Shoal wind farm. Engineering Geology , 177 , 40–53. https://doi.org/10.1016/j.enggeo.2014.05.005
- Mellet, C. , Long, D. , Carter, G. , Chiverell, R. , & Van Landeghem, K. (2015). Geology of the seabed and shallow subsurface: The Irish Sea. British Geological Survey Commissioned Report, CR/15/057. 52pp.
- Ó Cofaigh, C. , & Evans, D. J. A. (2001). Sedimentary evidence for deforming bed conditions associated with a grounded Irish Sea glacier, southern Ireland. Journal of Quaternary Science , 16 (5), 435–454. https://doi.org/10.1002/jqs.631
- Oh, K. Y. , Nam, W. , Ryu, M. S. , Kim, J. Y. , & Epureanu, B. I. (2018). A review of foundations of offshore wind energy convertors: Current status and future perspectives. Renewable and Sustainable Energy Reviews , 88 , 16–36. https://doi.org/10.1016/j.rser.2018.02.005
- O’Reilly, S. S. , Hryniewicz, K. , Little, C. T. S. , Monteys, X. , Szpak, M. T. , Murphy, B. T. , Jordan, S. F. , Allen, C. C. R. , & Kelleher, B. P. (2014). Shallow water methane-derived authigenic carbonate mounds at the Codling Fault Zone, western Irish Sea. Marine Geology , 357 , 139–150. https://doi.org/10.1016/j.margeo.2014.08.007
- Pantin, H. M. (1977). Quaternary sediments of the northern Irish Sea. In C. Kidson , & M. Tooley (Eds.), The quaternary history of the Irish Sea (pp. 27–54). Seel House.
- Pingree, R. D. , & Griffiths, D. K. (1979). Sand transport paths around the British Isles resulting from M2 and M4 tidal interactions. Journal of the Marine Biological Association of the United Kingdom , 59 (2), 497–513. https://doi.org/ 10.1017/S0025315400042806
- Robinson, I. S. (1979). The tidal dynamics of the Irish and Celtic Seas. Geophysical Journal of the Royal Astronomical Society , 56 , 159–197. https://orcid.org/10.1111/j.1365-246X.1979.tb04774.x
- Van Landeghem, K. J. J. , Baas, J. H. , Mitchell, N. C. , Wilcockson, D. , & Wheeler, A. J. (2012). Reversed sediment wave migration in the Irish Sea, NW Europe : A reappraisal of the validity of geometry-based predictive modelling and assumptions. Marine Geology , 295–298 , 95–112. https://doi.org/10.1016/j.margeo.2011.12.004
- Van Landeghem, K. J. J. , Niemann, H. , Steinle, L. I. , O’Reilly, S. S. , Huws, D. G. , & Croker, P. F. (2015). Geological settings and seafloor morphodynamic evolution linked to methane seepage. Geo-Marine Letters , 35 (4), 289–304. https://doi.org/10.1007/s00367-015-0407-5
- Van Landeghem, K. J. J. , Uehara, K. , Wheeler, A. J. , Mitchell, N. C. , & Scourse, J. D. (2009). Post-glacial sediment dynamics in the Irish Sea and sediment wave morphology: Data–model comparisons. Continental Shelf Research , 29 (14), 1723–1736. https://doi.org/10.1016/j.csr.2009.05.014
- Walbridge, S. , Slocum, N. , Pobuda, M. , & Wright, D. J. (2018). Unified geomorphological analysis workflows with Benthic Terrain modeler. Geosciences , 8 (3), 94. https://doi.org/10.3390/geosciences8030094
- Ward, S. L. , Neill, S. P. , Van Landeghem, KJJ, , & Scourse, J. D. (2015). Classifying seabed sediment type using simulated tidal-induced bed shear stress. Marine Geology , 367 , 94–104. https://doi.org/10.1016/j.margeo.2015.05.010
- Whitehouse, R. J. S. , Harris, J. M. , Sutherland, J. , & Rees, J. (2011). The nature of scour development and scour protection at offshore windfarm foundations. Marine Pollution Bulletin , 62 (1), 73–88. https://doi.org/10.1016/j.marpolbul.2010.09.007
- Woods, M. A. , Wilkinson, I. P. , Leng, M. J. , Riding, J. B. , Vane, C. H. , Lopes dos Santos, R. A. , Kender, S. , De Schepper, S. , Hennissen, J. A. I. , Ward, S. L. , Gowing, C. J. B. , Wilby, P. R. , Nichols, M. D. , & Rochelle, C. A. (2019). Tracking Holocene palaeostratification and productivity changes in the Western Irish Sea: A multi-proxy record. Palaeogeography, Palaeoclimatology, Palaeoecology , 532 , 109231. https://doi.org/10.1016/j.palaeo.2019.06.004
- Yuan, F. , Bennell, J. D. , & Davis, A. M. (1992). Acoustic and physical characteristics of gassy sediments in the western Irish Sea. Continental Shelf Research , 12 (10), 1121–1134. https://doi.org/ 10.1016/0278-4343(92)90073-S