ABSTRACT
The Aladağlar is a mountain range located in the central Taurus Mountains (37°45′ N, 35°15′ E) along the Mediterranean coast of Turkey, dominated by geomorphic features intervened by karstic and glacial landscapes. The Aladağlar, also known as The Alps of Turkey, is one of the most popular places for mountaineering. Here, we present a comprehensive glacial geomorphological map of the mountain. Despite increased recent research, understanding of the spatial distribution of glacial landforms is still poorly known. To provide a framework for future research, we present a 1:50,000-scale glacial geomorphological map, covering an area of ∼1200 km2. Our map is based on remote sensing and detailed field observations on geomorphological imprints of glacial erosional and depositional landforms, such as glacial valleys, cirques, arêtes, horns, debris-covered glaciers, moraines and outwash fans. Our glacial geomorphological map could be used as a base for future dating and glacier reconstructions efforts.
1. Introduction
Geomorphological mapping of glacial landforms is an ingrained technique in earth sciences to analyse earth surface processes and landscape evolution especially in high altitudes (>2500 m) at mid-latitude regions (CitationHubbard & Glasser, Citation2005; CitationSmith et al., Citation2011; CitationStroeven et al., Citation2013; CitationChandler et al., Citation2018, Citation2019). Today, the presence of open-source high-resolution remote sensing data and their integration with Geographical Information System (GIS) techniques provide a unique insight to map large areas with high accuracy. On the other hand, traditional field mapping methods in glacial geomorphology still have a significant role in terms of robustness and ground-truthing of remote mapping (CitationChandler et al., Citation2018).
Although glacial processes are well-known in high-latitudes and alpine environments, in mid-latitude environments where karstic landforms largely dominated, these processes are poorly constrained. The glacial valleys in the Aladağlar were formed by the interaction between glacial, fluvial, and karstic processes (CitationAltın, Citation2003a). Initially, the gradual collapse of underground karst rivers created many such valleys. The continuing roof collapses originated gorges that later turned into V-shaped valleys by ongoing fluvial and karstic erosion. Because the Taurus Mountains are mainly composed of carbonate rocks, the glacial landforms observed in the study area are the result of both glacial and karstic processes (Bayarı et al., Citation2019; CitationNazik et al., Citation2019; CitationÖztürk et al., Citation2018). These landscapes provide a unique environment to understand the interaction between glacial, post-glacial, and karstic processes.
Increasing applications of cosmogenic nuclides (i.e. 36Cl, 10Be) for dating glacial deposits and landforms gave rise to several publications related to glacial geochronology of the Turkish mountains (e.g. CitationAkçar et al., Citation2007; CitationDede et al., Citation2017; CitationSarıkaya et al., Citation2008, Citation2009, Citation2011, Citation2014; CitationKöse et al., Citation2019). During the Late Pleistocene, glaciers occupied the Taurus and Black Sea Mountains and high dormant volcanoes in the Anatolia (CitationBayarı, 2003; CitationÇiner, Citation2004; CitationKurter, Citation1991; Sarıkaya et al., Citation2014; CitationAzzoni et al., Citation2017). The glacial geomorphological maps of these studied areas have not always been constructed in detail. For further development of the geochronology and the reconstruction of paleoglaciers, detailed maps in smaller scales are needed (Altınay et al., Citation2020).
The purpose of our work is to develop a framework for the glacial erosional and depositional landforms that are keys to palaeoglacier studies in Turkey. Therefore, we created a high-resolution glacial geomorphological map of the Aladağlar, to provide a more detailed and accurate distribution of glacial and periglacial features. While earlier published maps focused mainly on the selected parts of the Aladağlar (e.g. CitationAltın, Citation2003a; Citation2010a), in this study we present the first comprehensive glacial geomorphological map covering a whole mountain range in Turkey (). Such a map is invaluable for efforts to locate target sites of strategic importance for palaeoglaciological reconstruction, to build robust glacial chronologies, and subsequently to infer palaeoclimate.
Figure 1. Study area and main topography of the Aladağlar, Turkey. (b) Western and Central Taurus Mountain Range topography derived from a spatial resolution of 1 arc second ASTER GDEM data, particular mountains above 2000m a.s.l. are labelled. The black rectangle in (b) indicates the Main Map (c). Glaciated valleys and main ridges in the Aladağlar and the area covered by Main Map are shown in (c).
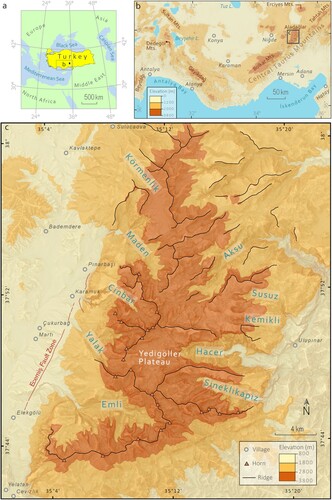
1.1. Study area
The Aladağlar is one of the Turkish National Parks and hosts the highest mountains of the central Taurus Range. The name ‘ala-dağlar’ (crimson-mountains) evolves from the rusty (or pink) appearance of its hills in the sunset (). It is also known as The Alps of Turkey, one of the most popular places for mountaineering, hiking, and trekking. The highest part of the mountain consists of Mesozoic carbonates with extensive karst that limits surface drainage (CitationTekeli et al., Citation1984; CitationAltın, Citation2003c; CitationKlimchouk et al., Citation2006). The modern climate is a mixture of Mediterranean and continental type climates, with hot and dry summers and wet and cold winters.
Figure 2. Panoramic photographs of valleys and outwash deposits in the western Aladağlar (a) and Main Map preview (b). In order to cover the highest peaks and whole outwash deposits, 12 UAV (an Unmanned Aerial Vehicle) photographs were merged to produce this panoramic view (a). The 1: 50.000 scale geomorphological map of the Aladağlar previewed in (b), records glacial landforms.
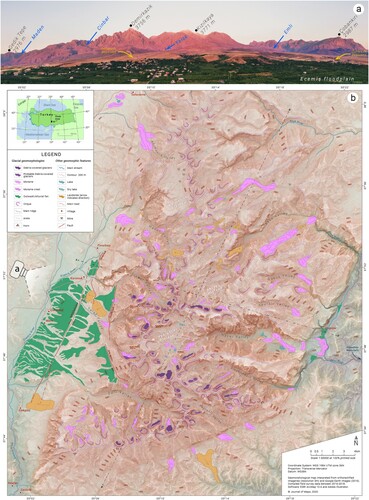
The Aladağlar extends from 37°41′ N to 38°01′ N and from 35°01′ E to 35°23′ E, in the south-central Anatolia. It is a broad carbonate massif that envelops a SW to NE trending mountain belt, covering c. 1200 km2. This massif is delimited by the sinistral strike-slip Ecemiş Fault corridor on the west (CitationKoçyiğit & Beyhan, Citation1998; CitationSarıkaya et al., Citation2015a, Citation2015b; CitationYıldırım et al., Citation2016), Zamantı River on the east, Erciyes Volcano to the north and Karsantı basin to the south. The topographic relief from 400 m a.s.l. (above sea level) to above 3750 m a.s.l. makes the Aladağlar one of the deepest karst systems in the world (Bayarı et al., Citation2019).
During the Late Pleistocene, the Aladağlar was extensively glaciated with the development of an ice cap on Yedigöller Plateau from which several outlet glaciers descended via U-shaped valleys. As a result, numerous glacial erosional and depositional landforms developed in the study area (CitationBayarı et al., Citation2003; CitationKöse et al., Citation2018). In Aladağlar, cirques, U-shaped valleys, striated/polished surfaces, roches moutonnées, moraines, periglacial features, and debris-covered glaciers are common (CitationOliva et al., Citation2018) (Main Map). The landforms shaped by the glacial processes created a polygenic topography together with the fluvial-karstic processes. The Mesozoic carbonate country rocks have played an essential role in protecting many of the glacial landforms, such as moraines and sharp cirques as deeply permeable karstic bedrock has produced poorly developed fluvial systems.
Several researchers previously investigated the glacial deposits of the Taurus Mountains (e.g. CitationErinç, Citation1952, Citation1978; CitationSpreitzer, Citation1969, Citation1971; Sarıkaya & Çiner, Citation2015, Citation2017 and references therein). During the Late Pleistocene at least two different glacial stages (MIS 4 and 2) occurred in the Aladağlar (CitationAltın, Citation2003b; CitationSarıkaya & Çiner, Citation2017). An ice cap covering nearly 40 km2, located in Yedigöller Plateau (3200 m), was flowing mainly into the eastern Hacer valley (Bayarı, Citation2003; CitationZreda et al., Citation2011), in which deglaciation occurred in Late-glacial (14.0 ± 1.5 ka). The c. 15 km long Hacer Valley glacier rapidly melted on the onset of the Holocene (11.5 ± 1.3 ka ago). Little Ice Age moraines have also observed in limited narrow cirques which covers upper parts of the Emli Valley (CitationAltın, Citation2003b). Today, debris-covered glaciers in Emli Valley and Yedigöller Plateau were mapped by Gürgen et al. (Citation2010a).
2. Methods
We prepared a thematic geomorphology map (CitationRobinson & Kimerling, Citation1995; CitationKraak & Ormeling, Citation2013), which focuses only the glacial geomorphology of the Aladağlar. We did not prefer the more general approach, i.e classical mapping, which may consist of less relevant multi-layered data (CitationKlimaszewski, Citation1990; CitationGustavsson et al., Citation2006). This way, we tried to show more relevant data on specific landforms with increased specialization.
The 1:50,000 scale glacial geomorphological map of the mountain (Main Map) was carried out at on-screen scales ranging from 1:15,000–1:30,000. Four stages were employed to produce the main map: (1) Basemap production, (2) literature review, (3) remote and field mapping, and (4) final map production (CitationChandler et al., Citation2018).
First, the DEM obtained from the Shuttle Radar Topography Mission (SRTM) was converted to a hypsometric colored DEM. Later, the hill-shade and slope raster images were prepared by using spatial analyst tools in ArcGIS Pro. The Red Relief Image Map (RRIM) and topographic openness were computed by SAGA 7.1. (CitationConrad et al., Citation2015) software. Topographic openness represents the surface concavities and convexities observed from a given Zenith (CitationYokoyama et al., Citation2002; CitationChiba et al., Citation2007). The RRIM images are very useful to recognize many landforms such as landslides (Görüm, Citation2019) or fluvial terraces (CitationÖzpolat et al., Citation2020). By using this multi-layered illumination-free images in ArcMap, the openness was produced as a combination of positive openness (Op) and negative openness. At the end of this initial stage, a unique basemap, named ‘u-basemap’, was produced by stacking the four layers: colored DEM, slope, hill-shade, and topographic openness, respectively ( and ).
Figure 3. The u-basemap (a unique basemap) illustration summarising the datasets to produce the final topographic basemap used in the study area (Main Map). Coloured DEM (Digital Elevation Model), slope, hillshade and topographic openness (CitationChiba et al., Citation2008) layers used in particular transparency.
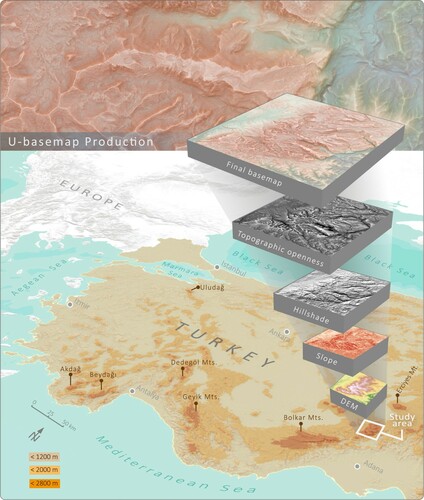
Figure 4. Flowchart diagram summarising the methods and datasets used in glacial geomorphological map of the Aladağlar (Main Map). Four main stages carried out with the help of remote and field mapping.
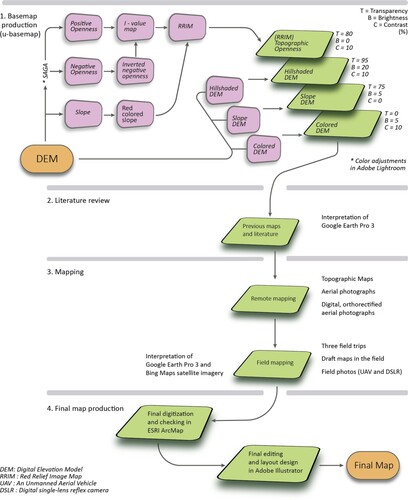
In the literature review stage, a GIS database was created including the prominent glacial landforms. Main glacial features were adapted from previous glacial geomorphology maps (CitationAltın, Citation2003b) which covers only Emli and Cinbar valleys on the eastern side of the mountain. Debris-covered glaciers in Emli Valley and Yedigöller Plateau were digitized from earlier studies (Gürgen et al., Citation2010a). All these features were overlaid on the u-basemap and then interpreted with Google Earth Pro®.
Later, glacial landforms were mapped using a combination of remotely sensed data and field surveys. A Digital Elevation Model (12 m resolution in horizontal) as a ‘bare earth’ landscape, digital color aerial photographs and hard-copy topographic maps were used as primary remotely sensed datasets. With the help of the digital rectified aerial photographs and Google Earth Pro®, we digitized the main arêtes, horns, cirques and moraines. Unfortunately, DEM and aerial photographs were not always able to differentiate individual moraine boundaries. To minimize any errors related to interpretation of features, we performed extensive field mapping alongside remote mapping. In addition to our long-lasting (since 2001) exploration of the glacial landforms of the area, we also carried out field campaigns in the summers of 2016, 2017 and 2018, specifically designed for mapping of the Aladağlar. Shape, size, orientation and positions of glacial features were noted during the fieldworks. Sketch maps of each valley were created in field studies These field investigations allowed us to identify landforms and glaciogenic deposits, which are not always easily detectable from remotely sensed data.
The final map production was conducted on GIS (ESRI ArcMap 10.6) with additional processing using graphic design software (Adobe® Illustrator CC™). During the map production, we used Google Earth Pro’s three-dimensional capability to solve the complex landform assemblages. The final map layout was initially designed in the GIS software before exporting to Adobe® Illustrator CC™, along with all individual vector layers (geomorphological features and contours). The focus at this stage was on the final map design and ensuring optimal map presentation.
3. Geomorphological features
Five landform categories; (1) glacial valleys, (2) cirques, arêtes and horns, (3) debris-covered glaciers, (4) moraines, and (5) outwash deposits were recorded in the 1:50,000-scale glacial geomorphological map of the Main Map summarized below. Our landform definitions used here are based mainly on previous paleoglaciological maps (e.g. CitationAltın, Citation2003b; Gürgen et al., Citation2010a). However, some adjustments have been made based on their paleoglaciological significance to deliver the purpose of this map accurately.
3.1. Glacial valleys
The parabolic-shaped valleys are one of the most well-known products of alpine-type glaciation, yet relatively little is known about how ice flow and glacial erosion interact with karst development. The post-glacial erosion in this area makes the identification of trimlines on the valley sides problematic. Therefore, we focused on two criteria to identify glacial valleys; U-shaped cross-section of a valley, and smoothness of valley sides (CitationGraf, Citation1970; CitationLi et al., Citation2001; CitationBenn & Evans, Citation2010).
The identification of glacial valleys was primarily based on the RRIM (CitationChiba et al., Citation2008) and slope model derived from DEM (). Together with this u-basemap and Google Earth Pro® we delineated glacial valleys. The ArcGIS Pro 2 topographic profile tool was used to differentiate the transition from glacial to fluvial/tectonic control on valley cross-section. First, transect lines for surface profiles were created at variable positions. Then, DEM derived data were used to analyse surface profile morphology whether they are reflecting glacial erosion or not. As a result of surface profile analyses, we have confirmed the general notion that many glaciated valleys in the Aladağlar have approximately parabolic-shape cross sections ().
Figure 5. Field photo plate of Yedigöller Plateau and Hacer glacial valley. (a) The Google Earth Pro® imagery of Yedigöller Plateau, and (b) U-shaped form of Hacer Valley. (c) LLR (left lateral moraine) and (d, e) the terminal moraine complex reaches 150-200 m in height. (f) Large boulders up to 7-10 m in diameter observed in the field.
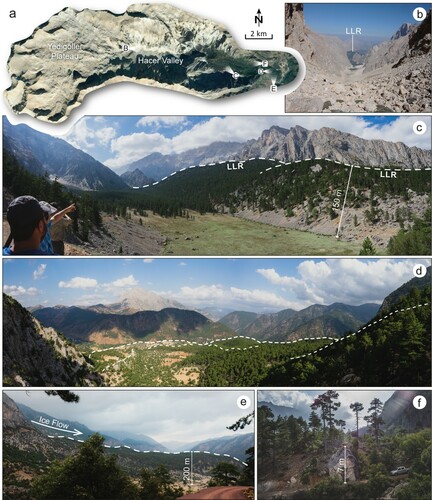
Surface profiles demonstrate that U-shaped valleys in the eastern part of the study area have parabolic-shape, however, evolved into V-shape valleys towards the north due to the decrease in glaciation influence (). Although, Sineklikapız, Hacer, Kemikli and Susuz valleys present a clear parabolic-shape, the valleys in the northeastern part of the Aladağlar (i.e. Aksu Valley) have V-shaped forms (). When glaciers advanced during the Late Pleistocene, the valleys in the east (Sineklikapız, Hacer, Kemikli, Susuz and Aksu valleys), and west (Emli, Cinbar, Maden and Körmenlik valleys) of the Aladağlar became straighter and smoother (, and ). The valley walls in the eastern and western part of the study area are almost vertical due to the chemical weathering in the less resistant karstic bedrock. After deglaciation, snow and ice melt formed streams and rivers within parabolic-shaped valleys. As a result, talus cones have been deposited at the bottom of the valley walls (CitationUtku et al., Citation2020).
3.1.1. Hacer Valley – the deepest glacial valley in the eastern Aladağlar
The Hacer Valley, the most prominent glacial valley in the eastern part of Aladağlar was originated from the paleo-ice cap in Yedigöller Plateau (3300 m a.s.l.) (CitationBayarı et al., Citation2003) ((a)). The ice cap in this plateau was c. 200 m thick and had an area of c. 22 km2. The glacier tongue that originated at the eastern part of this ice cap occupied the Hacer Valley and descended to the east at an elevation of 1100 m a.s.l., reaching c. 15 km in length (Bayarı et al., Citation2019). Several terminal and lateral moraines were deposited in the lower end of the valley ((d and e)). Additionally, dissected outwash deposits are observed in the lowest part of the valley down to an elevation of 850 m a.s.l. On the eastern flank of the Aladağlar to the north of Hacer Valley, at c. 1800m a.s.l., moraines overly the dolines ((d)). At higher elevations, mainly in eastern Aladağlar, glaciers have scoured all pre-glacial karstic landforms, and several of them were covered by glacial deposits (). For example, a series of dolines at 2200 m a.s.l. were covered by lateral moraines in Kemikli Valley.
Figure 6. Cross-section profiles showing interactions between glacial and fluvial processes in the eastern Aladağlar. (a) Yedigöller Plateau, Sineklikapız and Hacer valleys have A, B, C profiles, and (b) Aksu and Susuz valleys have D and E profiles with a dashed black line (see Figure 1 for location). A, B, C and E profiles show a U-shaped form, while (b) profile D shows a clear V-shaped form.
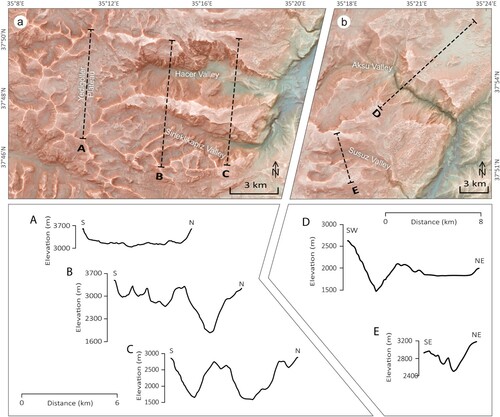
Figure 7. Field photo plate of eastern Aladağlar. (a) Yedigöller Plateau, (b) debris-covered glaciers, and (c) surface girlands above 3000 m a.s.l. (d) A well-preserved right-lateral moraine covers the dolines in Kemikli Valley. (f) An example of typical U-shaped imprint from Sineklikapız Valley. (e) The Kapuzbaşı Waterfalls located in the far east edge of the study area follow an ophiolite and limestone contact zone.
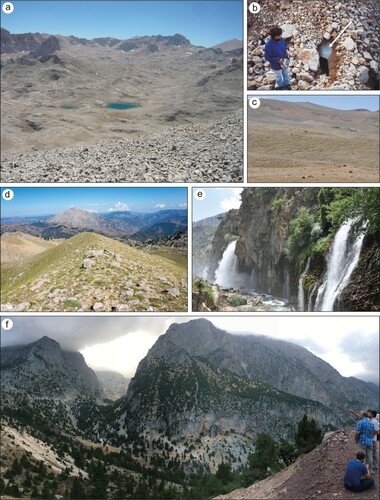
3.2. Cirques and arêtes
Cirques are glacial erosional hollows in mountainous terrain with concave slopes bounded upstream by a sharp arcuate headwall and sharp valley side ridges (arêtes) (CitationEvans et al., Citation2006) and are flat-floored and open at the downstream end (CitationEvans & Cox, Citation1974, p. 1995; Benn & Evans, Citation2010, Citation2014). One hundred and sixty-five individual cirques were identified in the Aladağlar; clusters of cirques were formed in the vicinities of Yedigöller Plateau, and Emli and Maden valleys ((c)).
After delineating the main ridges, most of the arêtes were commonly digitized with those ridges. Both ridges and arêtes have the same symbology types on the Main Map. However, the essential criteria to differentiate the arêtes are that they have a thinner line compared to the ridges. At the pyramidal peaks between arêtes, we have also defined the horns as a point feature. Then cirques have been digitized along the ridges, horns and arêtes. They have amphitheatre shaped basins bounded upstream by a headwall. We digitized the sharp (knife-edge) serrated ridges as aretes in between cirques. Cirques are the most easily identified features because they have more evident morphology. Most of the cirques, arêtes, and horns developed at >3000 m a.s.l. in the study area.
3.3. Moraines
There are different types of moraines in almost all valleys in the Aladağlar such as lateral, hummocky, ground and terminal moraines (). Although most of the terminal moraines have been partially dissected and washed away by the post-glacial processes, most of the moraines in valleys still present a clear morphology. In particular, lateral moraines are well preserved. They have easily recognizable crestlines due to almost inexistent surface flow on karstic bedrock. On the other hand, the morphology of these moraines might be asymmetric in cross-sections. Moraine sizes also vary from small (10 m2) to much larger complexes >1 km2. Furthermore, different subtypes of moraines can be deposited in the same small area. Hence, different moraine types were classified as ‘moraines’. Therefore, we did not separate between sub-types of moraines to avoid complexities.
Moraines were mainly identified during field studies and using the topographic basemap. The identification criteria were firstly based on landform characteristics of a moraine. The moraine’s shape and size were noted during the field trips. Moraine positions in valleys and crest orientations were also drawn into draft maps in the field. Furthermore, we analysed the boulders on the accessible moraine crests. For inaccessible areas, remote mapping and relative relief of topographic base has been interpreted from Google Earth Pro®. Ninety-seven moraine polygons were manually digitised for this regional study.
The vast majority of moraines in the eastern part of the Aladağlar were found in the Hacer Valley (). They mainly include several lateral and terminal moraine complexes. In the lower parts of the Hacer Valley, moraines reach c. 200 m in height and contain several limestones blocks up to 7-10 m in diameter ((f)). The Kemikli and Susuz valleys also contain large lateral moraines up to c. 80 m in height ((d)). However, the moraines in Sineklikapız Valley do not display clear crestlines; i.e. they have been dissected and partially washed away (Main Map). We observed some ground moraines in the upper parts of the Sineklikapız Valley.
In the western side of the Aladağlar, the most conspicuous similarity in four valleys is the absence of terminal moraines. Some remnants of terminal moraines were observed in Emli, Yalak, Cinbar and Maden valleys, however they are heavily dissected and modified by colluviums. Therefore, mapped moraines include mainly lateral and hummocky moraine morphologies. Most of the moraines are preserved on the upper parts of the Aladağlar, above 2200 meters. In contrast, Körmenlik Valley is the only valley which has well-preserved lateral moraines between 2000–2400 meters. These c. 2 km long lateral moraines consist of unstratified and unsorted limestone blocks up to 1 m in diameter.
3.4. Debris-covered glaciers
Although small recent glaciers are encountered in the Aladağlar, they are mostly covered by debris (Gürgen et al., Citation2010a, Citation2010b; CitationÇalışkan et al., Citation2014). We define debris-covered glaciers distinctively as having no clean ice is visible. Correct identification of debris-covered glaciers is difficult because they have continuous variations with the bed topography. In some cirques, they visually resemble rock glaciers. Therefore, we used previous maps and the topographic base interpreted with Google Earth Pro®. During the fieldwork, we observed the glaciers and their crescent-shaped imprints and noted several small ponds occupied the surface of debris-covered glaciers.
Debris-covered glaciers in the Aladağlar are predominantly settled in the north-facing cirques around the Yedigöller Plateau, and the upper parts of Emli Valley (>2800 m a.s.l.) ((b)). These cirques act as a shield preserving debris-covered glaciers with quite steep walls. (300-500 m in height and close to vertical). Most of the glaciers are in between 2800 and 3200 m a.s.l., and cover an area of approximately 1.43 km2 in total (calculated from Table 2 in Gürgen et al., Citation2010a).
3.5. Outwash deposits
Remote mapping combined with ground checking were applied to determine the boundary of glacial outwash deposits in Aladağlar. Only large, flat to slightly inclined areas (6–8°) that consist predominantly of sand and gravel-sized glaciofluvial deposits have been identified in the Main Map.
The western mountain front of the Aladağlar is covered by very extensive outwash and alluvial fan deposits, mostly composed of well-lithified limestone cobbles (5–25 cm in size) that contain crudely stratified and few meters thick beds reaching several tens of meters in total thickness ((a and c)). The Emli Valley on the southwestern part of the mountain seems to be the major feeding area, although much smaller Yalak River Valley also contributed to the formation of these fans (CitationSarıkaya et al., Citation2015a) (). These fan complexes are among the best-preserved successions observed in Turkey, which were later cut and offset by the major strike-slip Ecemiş Fault () (CitationKoçyiğit & Beyhan, Citation1998; CitationSarıkaya et al., Citation2015a, Citation2015b; CitationYıldırım et al., Citation2016).
Figure 8. Field photo plate of western Aladağlar. (a) Emli and (c) Yalak glacio-fluvial outwash deposits. (b) Maden and (d) Cinbar are deeply incised canyon valleys. An example of (e) moraine dammed glacial lake in the upper parts of Maden Valley. (f) Panoramic view of the lateral moraines in Körmenlik Valley (see for location).
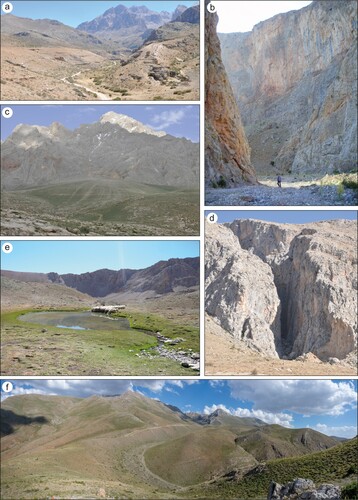
Cosmogenic surface exposure dating of the oldest alluvial fan surface (Yalak Fan) indicates a fan abandonment age that coincides mainly on the transition from the Penultimate Glaciation (Marine Isotope Stage 6; MIS 6) to the Last Interglacial (MIS 5) (i.e. Termination II) (CitationSarıkaya et al., Citation2015a). The second set of alluvial fan (Emli Fan) was possibly developed during the Last Interglacial (MIS 5), and was incised twice between c. 97–81 ka ago. A younger alluvial fan deposit developed on the relatively older erosional terraces of the Emli Fan during the Last Glacial Cycle (MIS 2) (CitationSarıkaya et al., Citation2015a). However, it is challenging to understand past glacial events, distributions of landforms, and reconstruct the glacial chronology in overall the Aladağlar due to the scarcity of detailed geomorphological mapping in these areas.
3.6. Landslides
Several landslides have been identified in the Aladağlar, with most of them clustered in the northwestern parts of the mountain. We observed 16 individual landslides in the study area. They usually have well-defined forms, which are easily recognized by remote mapping.
In the western part of the mountain, several large landslide bodies were mapped. The Kartal Landslide overlaid the alluvial fans near the Ecemiş and the Kartal Faults (CitationYıldırım et al., Citation2016). The rest of the landslides in the western Aladağlar are shallower and lie between the Kartal and Cevizlik Faults (CitationYıldırım et al., Citation2016). On the northern side of the mountain, we mapped eleven landslides, especially in the upper parts of Maden and Aksu valleys. These landslides mainly originated from the cirques. Their forms indicate rock avalanches, which are different from Kartal Landslides in terms of origin and morphology. Unlike Kartal Landslides, they are not controlled by faults. On the other hand, these landslides display a very local distribution over 3000 m a.s.l.
4. Conclusions
We present a new glacial geomorphology map of the Aladağlar, a mountain range that covers an area of c. 1200 km2 in the central Taurus Mountains of Turkey. Based on remote sensing and field mapping studies glacial landforms were divided into five different categories using 1:50,000 scale geomorphological map: (1) glacial valleys, (2) cirques, arêtes and horns, (3) debris-covered glaciers, (4) moraines, and (5) outwash deposits. In total, we identified 97 moraine polygons, which cover a total area of c. 30 km2 (2.5% of the study area). Today, the Yedigöller Plateau still has some debris-covered glaciers that cover an area of c. 1.43 km2 in total. Although all glacial valleys have typical parabolic-shaped valley profiles developed on carbonate bedrock, V-shaped fluvial valley profiles are also observed on the north-eastern side. Furthermore, prominent glacial landforms have survived until today due to the highly permeable carbonate rocks. The distribution of moraines and glacial valleys indicates that the Aladağlar experienced several alpine-style glaciations during the Late Pleistocene.
The map presented here is the most comprehensive inventory of glacial geomorphology for the Aladağlar. A detailed record of the glacial landforms in the Aladağlar provides a better understanding of glacial events and interactions on karstic bedrock assemblages by using a unique visualization of topographic basemap (u-basemap). Consequently, future studies will contribute to establishing a glacial history, reconstructing the last glacial ice masses, and predicting paleoclimatic conditions in the Aladağlar.
Software
ESRI ArcGIS 10.6 was used for data processing and mapping. Additional on-screen digitization was performed using Google Earth Pro®. The final map was designed in Adobe Illustrator CC 2018.
Main Map
Download PDF (106.5 MB)Acknowledgements
This paper is the result of several long-lasting financial supports by The Scientific and Technological Research Council of Turkey (TÜBİTAK grants no: 116Y155, 112Y087 and 101Y002). We thank for the field support provided by Emrah Özpolat and Adem Candaş. The first author thanks to the PhD scholarships provided by YÖK 100/2000 and TÜBİTAK BİDEB 2211-A.
Disclosure statement
No potential conflict of interest was reported by the author(s).
Additional information
Funding
References
- Akçar, N., Yavuz, V., Ivy-Ochs, S., Kubik, P. W., Vardar, M., & Schlüchter, C. (2007). Paleoglacial records from Kavron Valley, NE Turkey: Field and cosmogenic exposure dating evidence. Quaternary International, 164-165, 170–183. https://doi.org/https://doi.org/10.1016/j.quaint.2006.12.020
- Altın, B. N. (2003a). Aladağlarda Pleistosen buzullaşması ve jeomorfolojik sonuçları. TURQUA IV, İTÜ, Avrasya Yerbilimleri Enstitüsü Kuvaterner Çalıştayı Bildiri Özetleri, İstanbul, 15–16.
- Altın, B. N. (2003b). Aladağlar’da Preglasiyal dönem manzarası, İstanbul Üniversitesi Edebiyat Fakültesi Coğrafya Bölümü, Sırrı Erinç Sempozyumu, Genişletilmiş Bildiri Özetleri, 16–21.
- Altın, T. (2003c). “Aladağlar (Ecemiş çayı aklanı) üzerinde buzul ve karst jeomorfolojisi”, yayınlanmamış doktora tezi, İstanbul Üniversitesi, Sosyal Bilimler Enstitüsü.
- Altınay, O., Sarıkaya, M. A., & Çiner, A. (2020). Late-Glacial to Holocene glaciers in the Turkish Mountains. Mediterranean Geoscience Reviews. https://doi.org/https://doi.org/10.1007/s42990-020-00024-7
- Azzoni, R. S., Zerboni, A., Pelfini, M., Garzonio, C. A., Cioni, R., Meraldi, E., Smiraglia, C., & Diolaiuti, C. A. (2017). Geomorphology of Mount Ararat/Ağri Daği (Ağri Daği Milli Parki, eastern Anatolia, Turkey). Journal of Maps, 13(2), 182–190. https://doi.org/https://doi.org/10.1080/17445647.2017.1279084
- Bayarı, C. S., Klimchouk, A., Sarıkaya, M. A., & Nazik, L. (2019). Aladağlar mountain range: A landscape-shaped by the Interplay of glacial, karstic, and fluvial erosion. In C. Kuzucuoğlu, A. Çiner, & N. Kazancı (Eds.), Landscapes and landforms of Turkey (pp. 423–435). Springer.
- Bayarı, S., Zreda, M., Çiner, A., Nazik, L., Törk, K., Özyurt, N., Klimchouk, A., & Sarıkaya, M. A. (2003). The Extent of Pleistocene Ice Cap, glacial deposits and Glaciokarst in the Aladağlar massif: Central Taurids range, Southern Turkey. The XVI INQUA Congress: “shaping the Earth: A Quaternary Perspective” Reno, Nevada USA. Geological Society America Abstracts, 144–145.
- Benn, D. I., & Evans, D. J. A. (2010). Glaciers and glaciation (2nd ed., p. 802). Routledge.
- Benn, D., & Evans, D. J. (2014). Glaciers and glaciation. Routledge.
- Çalışkan, O., Gürgen, G., Yılmaz, E., & Yeşilyurt, S. (2014). Debris-covered glaciers during glacial and interglacial periods on the Taurus Mountains (Turkey). Procedia - Social and Behavioral Sciences, 120, 716–721. https://doi.org/https://doi.org/10.1016/j.sbspro.2014.02.154
- Chandler, B. M. P., Lovell, H., Boston, C. M., Lukas, S., Barr, I. D., Benediktsson, Í. Ö., Benn, D. I., Clark, C. D., Darvill, C. M., Evans, D. J. A., Ewertowski, M. W., Loibl, D., Margold, M., Otto, J.-C., Roberts, D. H., Stokes, C. R., Storrar, R. D., & Stroeven, A. P. (2018). Glacial geomorphological mapping: A review of approaches and frameworks for best practice. Earth-Science Reviews, 185, 806–846. https://doi.org/https://doi.org/10.1016/j.earscirev.2018.07.015
- Chandler, B. M., Lukas, S., Boston, C. M., & Merritt, J. W. (2019). Glacial geomorphology of the Gaick, central Grampians, Scotland. Journal of Maps, 15(2), 60–78. https://doi.org/https://doi.org/10.1080/17445647.2018.1546235
- Chiba, T., Kaneta, S. I., & Suzuki, Y. (2008). Red relief image map: New visualization method for three-dimensional data. The International Archives of the Photogrammetry, Remote Sensing and Spatial Information Sciences, 37(B2), 1071–1076.
- Chiba, T., Suzuki, Y., & Hiramatsu, T. (2007). Digital terrain representation methods and Red relief image Map. 45, 27–36. In Japanese with English abstract. https://doi.org/https://doi.org/10.11212/jjca1963.45.27.
- Çiner, A. (2004). Turkish glaciers and glacial deposits. In J. Ehlers, & P. L. Gibbard (Eds.), Quaternary glaciations: Extent and chronology, part I: Europe (pp. 419–429). Elsevier.
- Conrad, O., Bechtel, B., Bock, M., Dietrich, H., Fischer, E., Gerlitz, L., Wehberg, J., Wichmann, V., & Böhner, J. (2015). System for Automated Geoscientific analyses (SAGA) v. 2.1.4. Geoscientific Model Development, 8(7), 1991–2007. https://doi.org/https://doi.org/10.5194/gmd-8-1991-2015
- Dede, V., Çiçek, İ, Sarıkaya, M. A., Çiner, A., & Uncu, L. (2017). First cosmogenic geochronology from the Lesser Caucasus: Late Pleistocene glaciation and rock glacier development in the Karçal Valley, NE Turkey. Quaternary Science Reviews, 164, 54–67. https://doi.org/https://doi.org/10.1016/j.quascirev.2017.03.025
- Erinç, S. (1952). Glacial evidences of the climatic variations in Turkey. Geografiska Annaler, 34(1?2), 89–98.
- Erinç, S. (1978). Changes in the physical environment in Turkey since the end of last glacial. In W. C. Brice (Ed.), The environmental history of the near and Middle East since the Last Ice Age (pp. 87–110). Academic Press.
- Evans, I. S., & Cox, N. (1974). Geomorphometry and the operational definition of cirques. Area, 150–153.
- Evans, D. J., Twigg, D. R., & Shand, M. (2006). Surficial geology and geomorphology of the þórisjökull plateau icefield, west-central Iceland. Journal of Maps, 2(1), 17–29. https://doi.org/https://doi.org/10.4113/jom.2006.52
- Görüm, T. (2019). Landslide recognition and mapping in a mixed forest environment from airborne LiDAR data. Engineering Geology, 258, 105155. https://doi.org/https://doi.org/10.1016/j.enggeo.2019.105155
- Graf, W. L. (1970). The geomorphology of the glacial valley cross section. Arctic and Alpine Research, 2(4), 303–312. https://doi.org/https://doi.org/10.2307/1550243
- Gürgen, G., Çalışkan, O., Yılmaz, E., & Yeşilyurt, S. (2010a). Yedigöller platosu ve Emli vadisinde (Aladağlar) döküntü örtülü Buzullar, E-Journal of New world Sciences Academy. NEWSSA, 5(2), 98–116.
- Gürgen, G., Çalışkan, O., Yılmaz, E., & Yeşilyurt, S. (2010b). Döküntü örtülü Buzullar ve kaya Buzulları. NWSA, (www.Newwsa.com), 5(1), 32–45.
- Gustavsson, M., Kolstrup, E., & Seijmonsbergen, A. C. (2006). A new symbol-and-GIS based detailed geomorphological mapping system: Renewal of a scientific discipline for understanding landscape development. Geomorphology, 77(1-2), 90–111. https://doi.org/https://doi.org/10.1016/j.geomorph.2006.01.026
- Hubbard, B., & Glasser, N. F. (2005). Field techniques in glaciology and glacial geomorphology. John Wiley & Sons.
- Klimaszewski, M. (1990). Thirty years of detailed geomorphological mapping. Geographia Polonica, 58, 11–18.
- Klimchouk, A., Bayari, S., Nazik, L., & Törk, K. (2006). Glacial destruction of cave systems in high mountains, with a special reference to the Aladaglar massif, central Taurus, Turkey. Acta Carsologica, 35(2), 111–121.
- Koçyiğit, A., & Beyhan, A. (1998). A new intracontinental transcurrent structure: The central Anatolian Fault zone, Turkey. Tectonophysics, 284(3-4), 317–336. https://doi.org/https://doi.org/10.1016/S0040-1951(97)00176-5
- Köse, O., Çiner, A., Sarıkaya, M. A., Yıldırım, C., Candaş, A., & Wilcken, K. M. (2018). Late Pleistocene cosmogenic 36Cl glacial chronology of the Mount Karanfil and Aladağlar, Central Taurus Range. II. Kapadokya Yerbilimleri Sempozyumu, 24-26 Ekim 2018, Niğde, 114-115.
- Köse, O., Sarıkaya, M. A., Çiner, A., & Candaş, A. (2019). Late Quaternary glaciations and cosmogenic 36Cl geochronology of Mount Dedegöl, Taurus Mountains. Journal of Quaternary Science, 34(1), 51–63. https://doi.org/https://doi.org/10.1002/jqs.3080
- Kraak, M. J., & Ormeling, F. J. (2013). Cartography: Visualization of spatial data. Routledge.
- Kurter, A. (1991). Glaciers of Middle East and Africa - glaciers of Turkey. In R. S. Williams, & J. G. Ferrigno (Eds.), Satellite Image Atlas of the world. USGS Professional Paper. 1386–G–1, 1–30.
- Li, Y., Liu, G., & Cui, Z. (2001). Glacial valley cross-profile morphology, Tian Shan Mountains, China. Geomorphology, 38(1-2), 153–166. https://doi.org/https://doi.org/10.1016/s0169-555x(00)00078-7
- NASA/METI/AIST/Japan Spacesystems, and U.S./Japan ASTER Science Team. (2019). ASTER Global Digital Elevation Model V003 [Data set]. NASA EOSDIS Land Processes DAAC. Retrieved March 18, 2020, from https://doi.org/https://doi.org/10.5067/ASTER/ASTGTM.003
- Nazik, L., Poyraz, M., & Karabıyıkoğlu, M. (2019). Karstic landscapes and landforms in Turkey. In Landscapes and landforms of Turkey (pp. 181–196). Springer. https://doi.org/https://doi.org/10.1007/978-3-030-03515-0_5
- Oliva, M., Žebre, M., Guglielmin, M. M., Hughes, P., Çiner, A., Vieria, G., Bodin, X., Andrés, N., Colucci, R. R., García-Hernández, C., Mora, C., Nofre, J., Palacios, D., Pérez-Alberti, Ribolini, A., Ruiz-Fernández, J., Sar��kaya, M. A., Serrano, E., Urdea, P., Valcárcel, M., Woodward, J. & Yıldırım, C. (2018). Permafrost conditions in the Mediterranean basin since the Last glaciation. Earth-Science Reviews, 185, 397–436. https://doi.org/https://doi.org/10.1016/j.earscirev.2018.06.018.
- Özpolat, E., Yıldırım, C., & Görüm, T. (2020). The Quaternary landforms of the büyük Menderes Graben System: The southern Menderes massif, western Anatolia, Turkey. Journal of Maps, 16(2), 405–419. https://doi.org/https://doi.org/10.1080/17445647.2020.1764874
- Öztürk, M. Z., Şimşek, M., Şener, M. F., & Utlu, M. (2018). GIS based analysis of doline density on Taurus Mountains, Turkey. Environmental Earth Sciences, 77(14), 536. https://doi.org/https://doi.org/10.1007/s12665-018-7717-7
- Robinson, A. H., & Kimerling, A. (1995). Elements of cartography (No. 526 E4).
- Sarıkaya, M. A., & Çiner, A. (2015). Late Pleistocene glaciations and paleoclimate of Turkey. Maden Tetkik ve Arama Dergisi, 151(151), 107–127.
- Sarıkaya, M. A., & Çiner, A. (2017). The late Quaternary glaciation in the eastern Mediterranean. In Quaternary glaciation in the Mediterranean Mountains (Vol. 433, pp. 289–305). P. Hughes. & J. Woodward. (Eds.), Geological Society of London Special Publication. https://doi.org/https://doi.org/10.1144/SP433.4
- Sarıkaya, M. A., Çiner, A., Haybat, H., & Zreda, M. (2014). An early advance of glaciers on Mount Akdağ, SW Turkey, before the global Last glacial Maximum; insights from cosmogenic nuclides and glacier modeling. Quaternary Science Reviews, 88, 96–109. https://doi.org/https://doi.org/10.1016/j.quascirev.2014.01.016
- Sarıkaya, M. A., Çiner, A., & Zreda, M. (2011). Quaternary glaciations of Turkey. In J. Ehlers, P. L. Gibbard, & P. D. Hughes (Eds.), Quaternary glaciations-extent and chronology; a closer look (Vol. 15, pp. 393–403). Elsevier pub., Developments in Quaternary Science.
- Sarıkaya, M. A., & Tekeli, A. E. (2014). Satellite inventory of glaciers in Turkey. In J. S. Kargel, G. J. Leonard, M. P. Bishop, A. Kääb, & B. Raup (Eds.), Global Land Ice Measurements from Space (pp. 876). Praxis-Springer (Publisher). ISBN: 978-3-540-79817-0. 465-480.
- Sarıkaya, M. A., Yıldırım, C., & Çiner, A. (2015a). Late Quaternary alluvial fans of Emli Valley in the Ecemiş Fault zone, south central Turkey: Insights from cosmogenic nuclides. Geomorphology, 228, 512–525. https://doi.org/https://doi.org/10.1016/j.geomorph.2014.10.008
- Sarıkaya, M. A., Yıldırım, C., & Çiner, A. (2015b). No surface breaking on Ecemiş Fault, central Turkey, since Late Pleistocene (64.5 ka); new geomorphic and geochronologic data from cosmogenic dating of offset alluvial fans. Tectonophysics, https://doi.org/https://doi.org/10.1016/j.tecto.2015.02.022
- Sarıkaya, M. A., Zreda, M., & Çiner, A. (2009). Glaciations and palaeoclimate of Mount Erciyes, central Turkey, since the Last glacial Maximum, inferred from 36Cl cosmogenic dating and glacier modeling. Quaternary Science Reviews, 28(23–24), 2326–2341. https://doi.org/https://doi.org/10.1016/j.quascirev.2009.04.015
- Sarıkaya, M. A., Zreda, M., Çiner, A., & Zweck, C. (2008). Cold and wet Last glacial Maximum on Mount sandıras, SW Turkey, inferred from cosmogenic dating and glacier modeling. Quaternary Science Reviews, 27(7–8), 769–780. https://doi.org/https://doi.org/10.1016/j.quascirev.2008.01.002
- Smith, M. J., Griffiths, J., & Paron, P. (2011). Geomorphological mapping: Methods and applications, Developments in Earth surface processes, Volume, 15. Elsevier.
- Spreitzer, H. (1969). Die eiszeitliche und gegenwaertige Vergletscherung des kikilschen Ala Dag im Taurus (Pleistocene geology and glaciation of Ala Dag, Taurus Mountains, Turkey). Actas - Congreso Internacional del INQUA International Union for Quaternary Research, 5(1), 339–347.
- Spreitzer, H. (1971). Rezente und eiszeitlische Grenzen der glazialen und periglazielen höhenstufen im Zentralen Taurus (vornehmlich am Beispiel des Kilikischen Ala Dag). Mitteilungen des Naturwissenschaftlichen Vereines für Steiermark, 101, 139–162.
- Stroeven, A. P., Hättestrand, C., Heyman, J., Kleman, J., & Morén, B. M. (2013). Glacial geomorphology of the Tian Shan. Journal of Maps, 9(4), 505–512. https://doi.org/https://doi.org/10.1080/17445647.2013.820879
- Tekeli, O., Aksay, A., Urgun, B. M., & Isik, A. (1984). Geology of the Aladag mountains. Geology of the Taurus belt (pp. 143–158). MTA Publications.
- Utku, M., Öztürk, M. Z., & Şimşek, M. (2020). Emli Vadisi’ndeki (Aladağlar) Talus Depolarının Kantitatif Analizlere Göre İncelenmesi. COĞRAFİ PERSPEKTİFLE DAĞ VE DAĞLIK ALANLAR (Sürdürülebilirlik-Yönetim-Örnek Alan İncelemeleri), 978-625-7130-71-4.
- Yıldırım, C., Sarıkaya, M. A., & Çiner, A. (2016). Late Pleistocene intraplate extension of the central Anatolian Plateau, Turkey: Inferences from cosmogenic exposure dating of alluvial fan, landslide and moraine surfaces along the Ecemiş Fault zone. Tectonics, 35(6), https://doi.org/https://doi.org/10.1002/2015TC004038
- Yokoyama, R., Shirasawa, M., & Pike, R. J. (2002). Visualizing topography by openness: A new application of image processing to digital elevation models. Photogrammetric Engineering and Remote Sensing, 68(3), 257–266.
- Zreda, M., Çiner, A., Sarıkaya, M. A., Zweck, C., & Bayarı, S. (2011). Remarkably extensive glaciation and fast deglaciation and climate change in Turkey near the Pleistocene-Holocene boundary. Geology, 39(11), 1051–1054. https://doi.org/https://doi.org/10.1130/G32097.1