ABSTRACT
Gold nanoparticles (AuN) are one of the most investigated nanomaterials, finding numerous applications from medicine to industry. AuN were obtained by reduction of gold salts using tannic acid as a reducing agent. To detach the impact of AuN onto cells of two lines human monocytic cells (U-937) and human promyelocytic leukaemia cells (HL-60), the effects of postreaction residues (of effluent from sol cleaning) and gold ions were also examined. It was demonstrated that resistance of cells to the toxic action of AuN is dependent not only on incubation time and dosage, but also on stages of cell differentiation. It was found that after incubation of promonocytes U-937 with 25 ppm AuN, the cell viability decreased by 25% and of macrophages by 50%. Differentiated cells U-937 showed a significantly lower resistance than the not-differentiated cells. For HL-60 cells, regardless of differentiation, cell viability decreased by approximately 20% after treatment with 25 ppm AuN sol. It was noticed that U-937 exhibited higher vulnerability to AuN than HL-60 cells. It was proved that AuN induced over a 15-fold increase in nitric oxide and a decrease of intracellular glutathione levels indicating the inflammatory response of cells. Even though gold ions did not show a significant effect on cell viability, they caused fivefold increase of nitric oxide level. The results show a higher cytotoxicity of AuN than gold ions. An overall picture of the interaction of AuN with human cells of first defence line was obtained. The results indicate that AuN may cause changes in the response of phagocytes in inflammatory conditions.
1. Introduction
The huge development of nanotechnology has contributed to the ever-growing demand for nanoparticles with an extremely wide range of applications, from medicine and cosmetics to industry. This is also related to the unacknowledged risk of exposure of living organisms to nanoparticles.
Nanotoxicology as a new branch of science is trying to find an answer to the question whether the nanoparticles or nanomaterials are toxic to living organisms. The ‘foreign’ nanoparticles at first meet cells of the immune system. ‘The intruders’ use all possible ways of penetration into the body (skin, respiratory, digestive and circulatory systems). Nanoparticles are generally defined as particles between 1 and 50 nm in size. Studies have shown that the nanoscale objects smaller than 12 nm may cross the blood-brain barrier [Citation1,Citation2] and objects smaller than or equal to 30 nm may be endocytosed into cells.[Citation3] It is not surprising that intensive research is carried out on biomedical applications of nanoparticles. The action of the nanoparticles on living organisms depends on such parameters as physical and chemical properties of the particles, cell type, dose and duration of action. Unfortunately, the clear establishing whether the nanomaterials acts as a good or bad is still a matter of dispute.[Citation4] Gold nanoparticles (AuN) became an object of great attention due to their interesting electronic, optical and thermal properties and potential applications in the fields of medicine, biology, chemistry, physics and material sciences.[Citation5] The AuN have been reported as both toxic and non-toxic.[Citation6,Citation7] AuN were used as markers for tracking signal transduction in cells, which also suggests their non-toxic nature.[Citation8,Citation9] The AuN of diameter of 13 nm, covered by oligonucleotides were used for gene regulation.[Citation10]
Bulk gold is widely regarded as ‘safe’ and chemically inert, and, produced as drugs, have been used, inter alia, in the treatment of rheumatic diseases already in the seventies of the twentieth century.[Citation11] AuN are also applied in the local radionuclide therapy of cancer.[Citation12] Nanogold is also used in photothermal intravital therapy as imaging agents. AuN have some special optical properties, such as plasmon resonance. It was also shown that the AuN are selectively accumulated in tumour cells exhibiting bright scattering.[Citation13] Toxicological studies suggest the adverse effects of nanoparticles on health, but a full explanation of their action at the molecular level is still missing. The AuN used in medicine are in contact with body fluids or cells. Analysis of cytotoxicity in vivo is difficult and the results are often contradictory. That was the case of the study of multi-walled carbon nanotubes acting in a mouse model, where no induction of inflammation was showed, while macrophage activation and development of the inflammatory response was observed for single-walled carbon nanotubes.[Citation14,Citation15]
In the last decade, a lot of methods for the synthesis of AuN with the defined parameters, such as size or shape was developed.[Citation16–19] The preparation of AuN generally involves the chemical reduction of gold salt in aqueous, organic or simultaneously in two phases. Chemical reduction of metal salts is carried out in the presence of stabiliser which binding to particle surface imparts high stability and provides the desired charge and solubility.[Citation20] Great progress has been made in the synthesis of AuN. The surface chemistry plays a key role in future AuN applications as nanosensors, biosensors, catalysts, nanodevices and in nanoelectrochemistry.
Purification of gold sols for biomedical applications, understood as the removal of the residue after synthesis is crucial as improperly cleaned reaction mixture can itself act proinflammatory. The immune response includes humoral and cellular partners, where the phagocytes like macrophages and granulocytes are the first line of defence. For this reason, human granulocytes, monocytes and macrophages were chosen as a model system for detection of the action of AuN. In this paper, the effects of AuN, gold salts and the residue after the synthesis on model cells: a human cell line U-937 and HL-60 were presented. The human cell line U-937 was isolated from the pleural fluid of a patient with diffuse histiocytic lymphoma.[Citation21] HL-60 originated from the peripheral blood of a patient with acute promyelocytic leukaemia.[Citation22] These stable lines are capable to differentiate into granulocytes, monocytes and macrophages.
2. Materials and methods
2.1. Materials
Gold (III) chloride trihydrate and tannic acid were purchased from Sigma-Aldrich. Ultrapure water used during the AuN synthesis was obtained from the Milli-Q Elix&Simplicity 185 purification system (Millipore SA Molsheim, France). Dimethyl sulfoxide (DMSO) and 12-myristate-13-acetate (PMA) and all standard chemicals were purchased from Sigma (USA). RPMI-1640 medium, foetal bovine serum (FBS) and antibiotics were from CytoGen GmbH (Germany).
2.2. Preparation of gold nanoparticles
Aqueous solution of 0.1 mM tannic acid (93.6 ml) was heated to temperature of 50 °C in three-necked round-bottomed flask for 20 minutes under vigorous stirring. Afterwards, 5 ml of HAuCl4 (1 mM) was rapidly added to the reaction mixture. In 10 minutes, the colour of the reaction mixture changed from yellow to dark red. Nonetheless heating and stirring was continued for 30 minutes. After this period, the obtained gold suspension was cooled to room temperature. In order to remove the excess of unreacted substrates, suspension was washed with deionised water using a stirred filtration cell (Millipore, model 8400) with a regenerated cellulose membrane (Millipore, nominal molecular weight limit: 100 kDa). The washing procedure was repeated until the conductivity of the supernatant solution stabilised at 10–15 μS/cm and pH at 5.5–5.8.
2.3. Nanoparticle characterisation techniques
The AuN concentration in the suspension was determined using Anton Paar densitometer of high-precision (type DMA5000M) according to the procedure described previously.[Citation23]
UV–vis spectra of gold suspension were recorded using the Shimadzu UV-1800 spectrometer. Ultrapure water was used as the reference sample to take the ‘blank’ spectrum for all measurements.
The morphology and size of particles were determined using scanning electron microscope JEOL JSM-7500F working in a transmission mode. Samples for this examination were prepared by dispersing a drop of the gold colloid on a copper grid which was covered by a carbon film.
The diffusion coefficients and the electrophoretic mobility of obtained nanoparticles were measured by the dynamic light scattering (DLS) and microelectrophoresis, respectively, using the Zetasizer Nano ZS Malvern instrument.
2.4. Cell cultures and nanoparticles treatment
Human promyelocytic cells of the HL-60 line (ATCC) were cultured in suspension in RPMI-1640 medium containing 10% FBS and 0.01% penicillin–streptomycin. Stock solution of AuN (214 ppm) and their effluent were diluted in RPMI-1640 medium to the required concentration. Human promyelocytic leukaemia cells (HL-60) were induced to differentiate into macrophage-like cells by application of the phorbol-12-myristate-13-acetate (PMA) at a dose of 3 nM in RPMI-1640 medium with 10% FBS and 0.01% penicillin–streptomycin for 6 days.[Citation24] The differentiation into granulocytes was achieved by using 1.5% DMSO in RPMI-1640 medium with 10% FBS and 0.01% penicillin–streptomycin applied for 6 days.[Citation24,Citation25]
Human histiocytic lymphoma cell line U-937 (ATCC) was cultured in suspension in RPMI-1640 containing 5% FBS and 0.01% penicillin–streptomycin. The activated macrophages were obtained by inducting the U-937 cells with 1 μM PMA for 24 h in the full medium. The solution of appropriate concentration of nanoparticles was added to the cultures to obtain respective concentration of nanoparticles and such prepared cultures were incubated for 24, 48 and 72 h.
2.5. Cell viability assay
The tetrazolium salt colorimetric assay (MTT) described by Mosmann (Citation1983) was used to measure cytotoxicity of nanoparticles.[Citation26] Cells were cultured in 24-well plates in an amount of 0.25 mln cells HL-60 per well or 0.5 mln cells U-937 in a volume of 0.3 ml/well. After incubation with nanoparticles, gold salt (HAuCl4) or effluent from sol purification the 50 μl MTT solution (5mg/ml) in sterile water was added to each well and incubated for 2 h in 37 °C. Then, 0.3 ml of DMSO was added to each well and left for 5 minutes. After centrifugation, the supernatant optical density at 570 nm was read. Data were expressed as mean ± SD. The viability of the treated group was expressed as a percentage of the control group. Data points are means ± standard deviations (three determinations). The statistical analysis was performed by Duncan's multiple range test, taking p < 0.05 using PC SAS 8.0.
2.6. Nitric oxide production
Cells (1×106 cells/well) were treated for 24, 48 or 72 h with AuN or effluent of final volume of 0.5 ml. After treatment, the supernatants were collected, centrifuged (1000 × g, 5 minutes) and stored at –20 °C. Nitric oxide (NO) production from AuN, effluent or HAuCl4-treated cells was quantified spectrophotometrically using the Griess reagent (modified) (Sigma). The absorbance was measured at 540 nm and the nitrite concentration was determined using a calibration curve prepared with sodium nitrite.[Citation27] Data were expressed as mean ± SD. The statistical analysis was performed by Duncan's multiple range test, taking p < 0.05 using PC SAS 8.0.
2.7. Measurements of GSH
The intracellular reduced glutathione (GSH) level was determined by the reaction with 5,5′-dithiobis(2-nitrobenzoic acid) (DTNB) – the method of Ellman.[Citation28] Cells were differentiated as described above and then cells (3 × 106 cells/well) were treated with AuN or effluent for 24 h on 24-well plate (of 1 ml final volume). To 1 ml of samples, 75 μl of 50% trichloroethanoic acid was added and mixed for 30 s. The samples were centrifuged at 12,000 × g for 10 minutes at 4 °C and the supernatants were transferred to a tube on ice. The reaction mixture contained 0.8 ml of phosphate buffer (pH = 8.2), 0.1 ml of 6 mM DTNB and 0.1 ml of samples. Control samples without supernatant were also prepared. The absorbance was measured at 412 nm and the GSH concentration was read from the calibration curve. Results are presented in terms of protein content determined by the Bradford method. Data were expressed as mean ± SD. The statistical analysis was performed by Duncan's multiple range test, taking p < 0.05 using PC SAS 8.0.
3. Results
3.1. Characteristics of gold nanoparticle sol
At the first step of studies, the UV–vis analysis of gold suspension was performed. The absorption spectrum was presented in . As can be seen, the three characteristic absorption bands appear in the spectrum. The bands at 214 and 271 nm come from the oxidised form of tannic acid. The band at 530 nm is a result of plasmon resonance of AuN. As can be noticed, this peak is narrow suggesting that the suspension should be fairly monodisperse.
Figure 1. Ultraviolet–visible spectrum of gold nanoparticle suspension obtained with the use of tannic acid.
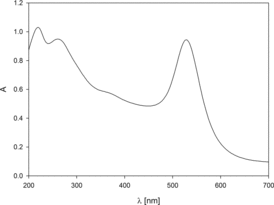
After filtration, the weight concentration of the AuN in aqueous suspension was precisely determined. The density of the stock gold suspension and the effluent solution acquired by membrane filtration was measured using densitometer DMA 5000M. Then, the concentration of gold suspension was calculated using formula described in detail in previous work.[Citation23] These measurements revealed that the concentration of AuN in stock sol was equal to 214 ppm.
To obtain the particle size distribution and information about the shape, the micrographs from transmission electron microscopy (TEM) were studied. The size of a single nanoparticle was defined as an average of the two axes perpendicular to each other and from the surface area of particles by using MultiScan Base software. Based on the obtained histograms (), it was found that the average size of nanoparticles was 20.9 ±10 nm.
Figure 2. Geometrical characteristics of obtained nanoparticles: main plot – the size distribution of nanoparticles, insets: TEM micrograph of nanoparticles deposited on copper grid.
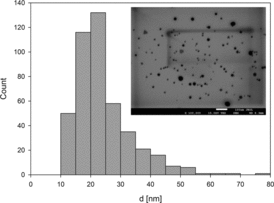
Furthermore, the DLS method was used to determine the hydrodynamic diameter of AuN at varying pH of the medium. Particle diffusion coefficients were measured by DLS and the hydrodynamic diameters were calculated using the Stokes–Einstein relationship. The results obtained at ionic strengths 10−3 and 10−2 M NaCl and pH range 3.5–9.3 are presented in . As can be noticed, the particle hydrodynamic diameters remain practically constant and equal to 20 nm in pH range 4.0–8.0. A significant increase in nanoparticle size was only observed at pH below 4.0 which indicates some instability (aggregation) of the gold particle suspension under such conditions.
Figure 3. The dependence of the hydrodynamic diameter of nanoparticles on pH determined for ionic strength: (•)10−3 M NaCl and (○) 10−2 M NaCl. Measurement conditions: suspension concentration cp = 100 mg/l, T = 298 K. The solid line is a guide to the eye.
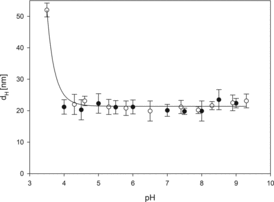
The measurements of electrophoretic mobility (μe) of AuN at controlled pH were carried out at constant ionic strength. From electrophoretic mobility, the zeta potential of nanoparticles was calculated using Henry's equation analogously as it was described previously.[Citation29] As can be seen in , the zeta potential of gold particles remains negative for the entire range of pH, indicating that the particles acquired a net negative surface charge. With increasing pH, zeta potential attains more negative values. Thus, the zeta potential equals –43 mV at pH 4.3 and –65 mV at pH 9.2.
3.2. Cytotoxicity of AuN, effluent and gold ions against cultured U-937 and HL-60 cells
Representative plots of cell viability after AuN treatment are shown in . For both lines, cell survival changes pretty much the same after 24 and 48 h of incubation, but being, however, dependent on nanoparticles' doses. At 25 ppm AuN, the concentration survival of U-937 cells decreased by approx. 25% and for the HL-60 cells by about 20%. Significant changes were observed after 72 h contact. Extending the incubation time to 72 h resulted in a decrease in viability by 50% for U-937 cells and only by 25% for HL-60 cells.
Figure 5. Changes of cell viability due to contact with AuN. Cell viability was measured by the MTT assay and the viability of the treated group was expressed as a percentage of the control group (cells not treated). (a) U-937 cells or (b) HL-60. Cells were incubated with AuN for 24, 48 and 72 h. Data points are means ± standard deviations (three determinations).
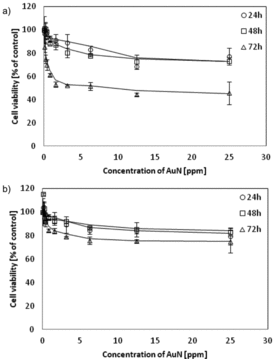
Cytotoxicity of AuN was studied as a function of the stage of cell differentiation (). U-937 cells after treatment with phorbol ester showed lower survival after contact with AuN than undifferentiated ones. After differentiation into macrophages, 20% decrease in survival was observed when comparing to cells not treated with PMA. For HL-60, cells differentiated into macrophages or granulocytes and no significant changes in survival were found.
Figure 6. The dependence of cell viability on AuN concentration. (a) U-937 cells were activated in 1 day with 1 μM PMA and (b) HL-60 cells were differentiated during 6 days: to macrophages using 3 nM PMA or to granulocytes with 1.5% DMSO. Cell viability was measured by the MTT assay and the viability of the treated group was expressed as a percentage of the control group (cells not treated by AuN). After that cells were incubated with AuN for 24 h. Data points are means ± standard deviations (three determinations).
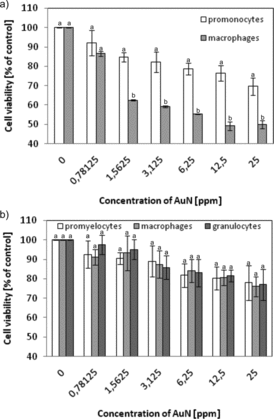
To study a possible cytotoxicity of the residue after the AuN synthesis, the effluent was added to both cells lines (). It was found that the effluent does not affect cell viability. Only 5% decrease in viability of the cell line HL-60 was observed. The tannin content in the effluent was also determined (results not shown). Tannin content in stock effluent was 2.1 ppm, which, after dilution in the same way as gold sol with 25 ppm AuN, corresponds to 0.14 ppm.
Figure 7. Cell viability after effluent (remains of the synthesis of AuN) treatment. U-937 and HL-60 cells were incubated for 24 h with effluent (diluted the same way as gold sol). Cell viability was measured by the MTT assay and the viability of the treated group was expressed as a percentage of the control group. Data points are means ± standard deviations (three determinations).
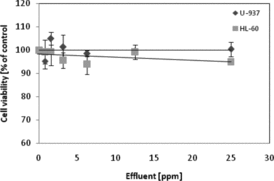
The effect of gold ions on U-937 and HL-60 cells was tested (). We observed that incubation of the cells with gold ions (originated from a salt constituting a substrate in the nanoparticle synthesis) has little influence on the cell viability. At the highest concentration of gold salt (25 ppm), U-937 promonocytes showed a 9% reduction in survival (relative to the control), while macrophages survival decreased by 15%. In the case of HL-60, promyelocytes viability decreased to 88% at Au2+ concentration equal to 25 ppm, whereas the survival of macrophages decreased by 4% and survival of granulocytes remained unchanged.
Figure 8. Effect of gold ions on the survival of original and differentiated U-937 and HL-60 cells. Cell viability was measured by the MTT assay and the viability of the treated group was expressed as a percentage of the control group (cells not treated). (a) U-937 cells were activated in 1 day with 1 μM PMA and (b) HL-60 cells were differentiated in 6 days: to macrophages using 3 nM PMA or to granulocytes with 1.5% DMSO. After that cells were incubated with HAuCl4 for 24 h. Data points are means ± standard deviations (three determinations).
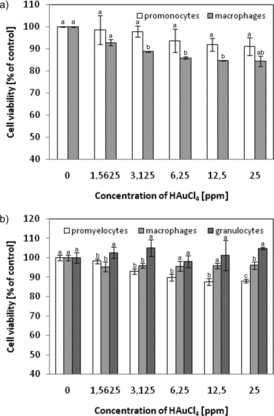
3.3. Increased NO level
To investigate the relationship between the development of the inflammatory response and cytotoxicity caused by AuN or gold ions, the release of NO (acting as a second messenger in inflammatory signalling) was measured. As shown in , secretion of NO increased significantly after contacting the cells with AuN. For promonocytes U-937, as a result of cell treatment by AuN at a concentration of 25 ppm, 17-fold increase in the NO content in the tested cell medium was found. For U-937, macrophages treated by AuN at the same concentration NO level increased 15 times. In contrast to the U-937 line, promyelocytes, granulocytes and macrophages of the HL-60 line did not differ among themselves in NO production. For HL-60 cells, a fivefold increase in NO concentration after treatment with 25 ppm AuN was found.
Figure 9. Levels of NO secretion due to AuN treatment of (a) U-937 cells or (b) HL-60 cells differentiated or not. NO production was quantified spectrophotometrically using the Griess reagent. Cells were treated with AuN with the indicated concentrations for 24 h. Data points are means ± standard deviations (three determinations).
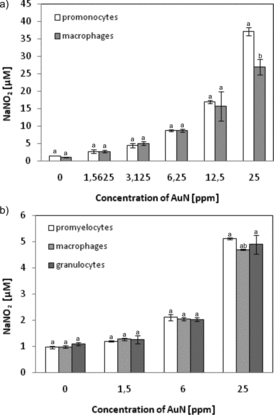
Figure 10. NO production by cells treated with gold salt (HAuCl4). (a) U-937 cells or (b) HL-60 cells differentiated or not. NO level was quantified spectrophotometrically using the Griess reagent. Cells were treated with HAuCl4 with the indicated concentrations for 24 h. Data points are means ± standard deviations (three determinations).
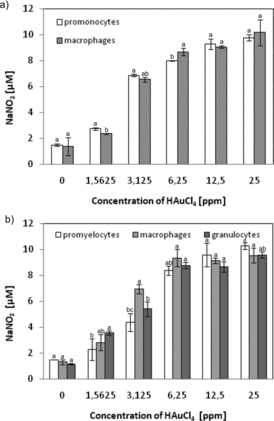
To study the possible induction of inflammation through the gold ions, the NO content in the culture medium was examined (). For all tested cell types, incubation with the highest concentration of HAuCl4 (25 ppm) resulted in a fivefold increase in the content of NO in the medium (relative to the control). No relationship between the degree of cell differentiation and production of NO was found in this study.
3.4. Decreased intracellular GSH level
To explore the correlation between cytotoxicity and the induction of oxidative stress induced by the presence of AuN, the level of intracellular GSH (acting as an antioxidant scavenger of reactive oxygen species) was measured. Concentration of intracellular GSH in promonocytes U-937 decreased to 75.8%, 75.5% and 36.2% of the control (untreated cells) at AuN concentrations of 1.56, 6.25 and 25 ppm, respectively, while in U-937 macrophages, the GSH level decreased by approx. 40% ().
Figure 11. Concentration of intracellular GSH in (a) U-937 or (b) HL-60 cells treated for 24 h by AuN at various doses. Data points are means ± standard deviations (three determinations).
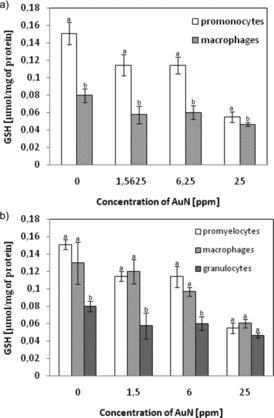
Reduction of intracellular GSH content was also observed for the cells of the HL-60 line. The GSH level in promyelocytes HL-60 treated with AuN at 25 ppm concentration for 24 h diminished by 60%, in macrophages HL-60 by 55% and in granulocytes by 42% ().
4. Discussion
The nanoparticles may cause a variety of effects on the cellular response of the components, such as cell membranes, mitochondria and nucleus. They may have adverse effects on organelles, DNA and regulation of protein biosynthesis, and may cause oxidative stress, apoptosis and mutagenesis.[Citation30–32] Nanotoxicological studies of the model cell lines facilitate evaluation of the effects of particles exerted on the cells; nonetheless, the results obtained for model systems not always coincide with the in vivo studies.
Based on numerous reports, AuN were hailed as non-toxic. Studies on human leukaemia cell line treated with gold nanospheres of different sizes (4, 12, 18 nm in diameter) and covered by various capping agents have shown that they are non-toxic.[Citation6] Similar results were obtained with AuN of 3.5 nm diameter. These nanoparticles penetrated the cells by endocytosis without inducing toxicity.[Citation33] For example, AuN did not exhibit cytotoxic activity against dendritic cells.[Citation34] In contrast to these findings, other research showed that the AuN are toxic. Thus, cationic gold nanospheres (2 nm in diameter) were found to be toxic, whereas anionic nanoparticles were not toxic to the same cell line.[Citation35] Equally important seems to be their size, for example, 1.4 nm gold nanospheres resulted in necrosis, damage to mitochondria and induction of oxidative stress, while 15 nm gold nanospheres did not harm the cells.[Citation32]
Many authors focused on the impact of physical and chemical parameters, such as particle size, shape or charge, while the effect of a degree of nanoparticle purification on the biological model was not tested. Today, many preparations of gold sols are available in commercial sales, but they are not equipped with information on the degree of purification from the residues after synthesis. Particle purification was defined by the conductivity of the sol solution. Gold sol used in presented studies underwent a very thorough cleaning and reliable physicochemical characterisation. Among many described physical, physicochemical and biological methods for the AuN synthesis, the chemical reduction of gold ions by tannic acid was chosen. Tannic acid is a natural polyphenolic reducer belonging to the group of hydrolysable tannins, which contain glucose esterified by gallic acid in the central core.[Citation36] Due to reducing and stabilising properties, tannic acid is often used for the synthesis of nanoparticles of gold,[Citation37,Citation38] silver [Citation39,Citation40] and nickel.[Citation41] The additional advantage of tannic acid is that it is a natural antioxidant exhibiting also antiviral activity.[Citation42–47]
Toxicity of AuN was tested using two human cell lines of the immune system which are capable to differentiate into macrophages or granulocytes after treatment by the inductive factors. The role of these cells is to defend organisms against ‘strangers’: microbial or inorganic particles that can penetrate the body. Human monocytic U-937 cells are non-adherent under standard culture conditions. These cells when treated with phorbol ester differentiate into macrophages.[Citation48,Citation49] It has been shown that these macrophages contain non-specific esterase, β-glucuronidase, elastase and lysozyme. At the surface of U-937 cells, Fc receptor, C3 and chemotactic peptides for monocytes were identified. After activation, U-937 macrophages are capable of producing reactive oxygen species.[Citation49] Formation of H2O2 and O2•− by U-937 has been studied in great detail. In this case, the ability for reactive oxygen species production is a measure of the cell maturity. The percentage of nitro blue tetrazolium chloride (NBT)-positive cells increases significantly after treatment with agents, such as TPA, DMSO, LK, RA, VD3 and PMA.[Citation49–51] Promielocytes HL-60 are also non-adherent under standard culture conditions. The characteristic feature of this cell line is the ability to differentiate into several types of cells of the myelocytic line, such as granulocytes, monocytes, macrophages and eosinophils.[Citation25] In these studies, two ways of differentiation were used: to macrophages (using 6-day incubation with 3 nM PMA) and to granulocytes (6-day incubation with 1.5% DMSO).[Citation24] The application of DMSO results in significant changes of morphological, functional, enzymatic and surface membrane antigens’ characteristics of cells with consequent formation of mature granulocytes.[Citation25] Cells of the HL-60 line, differentiated to neutrophils-like cells, possess granulocytes’ nuclei disintegrating into lobes. These cells exhibit an increased expression of the CD11b antigen, and are capable to reduce NBT to phagocytosis.[Citation52] Differentiation, in vitro, beside changes in cell functions, causes significant alterations in the composition of membrane lipids and in cell membrane fluidity.[Citation53]
The experimental system included two types of cells: the human cell lines U-937 and HL-60. Selected cell types that after differentiation become monocytes, macrophages and granulocytes constitute the first line of defence. Being widely distributed in blood and tissues, these cells come into contact with ‘foreign’ agents, such as metal nanoparticles. ‘Response’ of the body to a toxic agent depends on the cell types, which reaches the place of contact with the toxic agent as the first. Their activation promotes and modulates the course of the immune response of the body. Getting the best purified and defined AuN is the goal of many laboratories around the world. Using highly purified AuN enables one to observe only the activity of gold (not of the postsynthesis residue which often can be more toxic than analysed nanoparticles).
The plasticity of selected cell lines allowed us to carry out tests on the human immune system cells that are most exposed to contact with toxic agents. The results provide a tentative picture of the possible effects of AuN on the human body and induce further research on this issue.
In this work, the cell viability as a function of incubation time and AuN was determined. Viability of the cells treated with 25 ppm AuN was reduced by 20% and was practically the same for 24 and 48 h of incubation. Increasing the incubation time to 72 h led to a decrease in the survival of the cell line U-937 to 50% only. On the basis of these results, 24 h incubation was chosen for studying the effect of AuN on cells. Macrophages have a nearly twice lower survival compared to the U-937 promonocytes. Probably it is connected with many changes taking place during cell differentiation. Viability of the HL-60 cells did not change with differentiation. An interestingly opposite effect, i.e. differentiated cells showing a significantly higher resistance to the toxic effect of silver nanoparticles than the non-differentiated cells, has been described in our previous work.[Citation54]
The mechanism of nanogold seems to be based on a different mode of action. For example, in contrast to the well-soluble nanoparticles of silver or copper, gold does not dissolve so easily. Increased toxicity to the cells differentiated in the forfeiture of macrophages may be related to, among others, mechanical damaging of the U-937 cells.
Treatment of U-937 cells by PMA causes the changes in the production of reactive oxygen species and specific cell surface receptors, and cells acquire the ability to phagocytosis and chemotaxis.[Citation55] This might explain such a significant decrease in cell viability.
To ensure that the observed changes are not due to the possible presence of residues from the sol gold postprocessing, the influence of the effluent and the substrate used for the nanoparticle synthesis was examined. The effluent caused no alterations to studied cell viability. Tannin used for the synthesis arouses interest as a factor which itself can influence the cell viability. Tannic acid is a highly water-soluble polyphenolic antioxidant that exhibits antiviral, anti-inflammatory and anticancer activity.[Citation44,Citation55–65] However, there are literature reports indicating the induction of apoptosis by the action of TA on cells. Chen et al. showed pro-apoptotic activity of TA on the cells of the HL-60 line depending on the time of exposition and TA dose.[Citation63] The authors noticed 50% reduction in cell viability after 24 h incubation at a TA concentration equal to 17 μM. Experiments described in our publication were performed after subsequent purification steps aimed at removing the postsynthesis remains (including TA). The TA concentration in the collected effluent (determined by two analytical methods) appeared to be equal to 2.1 ppm TA (1.2 μM), while the TA content in the gold sol was below the detection limit. Therefore, it can be excluded that the observed effects were caused by the postreaction remnants.
In addition, a study was conducted on the impact of gold ions used for the synthesis on cell viability. It appeared that gold ions have less effect on cell viability than AuN. So far, it has been suggested that gold can inhibit the activity of lysosomal enzymes in phagocytic cells.[Citation66] Knowledge about the influence of gold ions on cells of the immune system is still modest. It was recognised that gold ions are powerful inhibitors of macrophages and polymorphonuclear leucocytes.[Citation67] Most reports are focused on the release of gold ions from implants and their accumulation in tissues and cells.[Citation68] The immuno-modulatory effects of gold ions have been exploited in the treatment of rheumatic arthritis for more than 50 years. Even though the underlying mechanisms have never been fully revealed.
NO acts as a mediator of inflammation state and has many biological functions, both in physiological and pathophysiological processes. Depending on the concentration, NO may be involved in neurotransmission, regulation of blood pressure and vascular permeability, as well as in the body's immune response. More than 15-fold increase in NO production by U-937 cells incubated with AuN found in the studies indicates a strong stimulation of the cells to induce an immune response. This effect is not as strong as in the case of HL-60 cells, where the production of NO increases only five times. Gold ions did not cause such a strong response, as for both types of cells, fivefold increase in the NO concentration in the culture medium was observed. Undoubtedly, the presence of AuN is responsible for induction of the inflammatory processes.
As is well known, metal nanoparticles induce oxidative stress through the generation of reactive oxygen species in the cells. The relationship between oxidative stress and AuN cytotoxicity can be represented by intracellular GSH. GSH acts as an antioxidant scavenging of reactive oxygen species formed under stress conditions. In this study, the concentration of GSH was determined. A significant decrease of GSH levels after treatment by AuN was found in all examined cell types. A similar effect, i.e. a decrease in the GSH content, was observed in murine macrophages treated with silver nanoparticles.[Citation69]
The increasing potential benefits from the use of AuN in biomedicine and industry to human health and to the environment are widely studied.
For more than 50 years, gold is used in medicine for dental implants. The radioactive gold is used in cancer therapy.[Citation70,Citation71] Cytotoxicity – a term of biological and medical importance – depends on the context. It can be understood either as a universal cytotoxic activity or as toxic only to a particular cell type. AuN have been found to be ‘non-toxic’ according to many authors. Our research shows that the synthesised 20 nm AuN, of high purity and physicochemically defined, exhibit cell-type selective ability of death induction. Furthermore, AuN activate a signalling cascade leading to induction of inflammation, especially through promonocytes and human macrophages, as well as the silver nanoparticles, as described previously.[Citation54] In this regard, it seems that very less importance has been paid to gold ions as inductors of the inflammatory response. The synthesis procedure and accurate purification of AuN contributes to their biocompatibility. It seems that it is possible to work out an acceptable compromise between the good and bad influence of AuN on the human body.
Disclosure statement
No potential conflict of interest was reported by the authors.
References
- Oberdörster G, Sharp Z, Atudorei V, et al. Translocation of inhaled ultrafine particles to the brain. Inhalation Toxicol. 2004;16(6–7):437–445. doi:10.1080/08958370490439597
- Sonavane G, Tomoda K, Makino K. Biodistribution of colloidal gold nanoparticles after intravenous administration: effect of particle size. Colloids Surf B. 2008;66(2):274–280. doi:10.1016/j.colsurfb.2008.07.004
- Conner SD, Schmid SL. Regulated portals of entry into the cell. Nature. 2003;422(6927):37–44. doi:10.1038/nature01451
- Alkilany AM, Murphy C J. Toxicity and cellular uptake of gold nanoparticles: what we have learned so far? J Nanoparticle Res. 2010;12(7):2313–2333. doi:10.1007/s11051-010-9911-8
- Rashid MH, Bhattacharjee RR, Kotal A, et al. Synthesis of spongy gold nanocrystals with pronounced catalytic activities. Langmuir. 2006;22(17):7141–7143. doi:10.1021/la060939j
- Connor EE, Mwamuka J, Gole A, et al. Gold nanoparticles are taken up by human cells but do not cause acute cytotoxicity. Small. 2005;1(3):325–327. doi:10.1002/smll.200400093
- Pernodet N, Fang X, Sun Y, et al. Adverse effects of citrate/gold nanoparticles on human dermal fibroblasts. Small. 2006;2(6):766–773. doi:10.1002/smll.200500492
- Yi H, Leunissen JL, Shi GM, et al. A novel procedure for pre-embedding double immunogold–silver labeling at the ultrastructural level. J Histochem Cytochem. 2001;49(3):279–283. doi:10.1177/002215540104900301
- Tkachenko AG, Xie H, Liu Y, et al. Cellular trajectories of peptide-modified gold particle complexes: comparison of nuclear localization signals and peptide transduction domains. Biocon Chem. 2004;15(3):482–490. doi:10.1021/bc034189q
- Rosi NL, Giljohann DA, Thaxton CS, et al. Oligonucleotide-modified gold nanoparticles for intracellular gene regulation. Science. 2006;312(5776):1027–1030. doi:10.1126/science.1125559
- Finkelstein AE, Walz DT, Batista V, et al. Auranofin. New oral gold compound for treatment of rheumatoid arthritis. Ann Rheumatic Diseases. 1976;35(3):251–257. doi:10.1136/ard.35.3.251
- Metz O, Stoll W, Plenert W. Meningosis prophylaxis with intrathecal 198Au‐colloid and methotrexate in childhood acute lymphocytic leukemia. Cancer. 1982;49(2):224–228. doi:10.1002/1097-0142(19820115)49:2<224
- El-Sayed IH, Huang X, El-Sayed MA. Surface plasmon resonance scattering and absorption of anti-EGFR antibody conjugated gold nanoparticles in cancer diagnostics: applications in oral cancer. Nano Lett. 2005;5(5):829–834. doi:10.1021/nl050074e
- Mitchell LA, Gao J, Vander Wal R, et al. Pulmonary and systemic immune response to inhaled multiwalled carbon nanotubes. Toxicol Sci. 2007;100(1):203–214. doi:10.1093/toxsci/kfm196
- Chou CC, Hsiao HY, Hong QS, et al. Single-walled carbon nanotubes can induce pulmonary injury in mouse model. Nano Lett. 2008;8(2):437–445. doi:10.1021/nl0723634
- Murphy CJ, Sau TK, Gole AM, et al. Anisotropic metal nanoparticles: synthesis, assembly, and optical applications. J Phys Chem B. 2005;109(29):13857–13870. doi:10.1021/jp0516846
- Grzelczak M, Pérez-Juste J, Mulvaney P, et al. Shape control in gold nanoparticle synthesis. Chem Soc Rev. 2008;37(9):1783–1791. doi:10.1039/B711490G
- Jain PK, Huang X, El-Sayed IH, et al. Noble metals on the nanoscale: optical and photothermal properties and some applications in imaging, sensing, biology, and medicine. Acc Chem Res. 2008;41(12):1578–1586. doi:10.1021/ar7002804
- Skrabalak SE, Chen J, Sun Y, et al. Gold nanocages: synthesis, properties, and applications. Acc Chem Res. 2008;41(12):1587–1595. doi:10.1021/ar800018v
- Guo S, Wang E. Synthesis and electrochemical applications of gold nanoparticles. Anal Chim Acta. 2007;598(2):181–192. doi:10.1016/j.aca.2007.07.054
- Sundström C, Nilsson K. Establishment and characterization of a human histiocytic lymphoma cell line (U‐937). Int J Cancer. 1976;17(5):565–577. doi:10.1002/ijc.2910170504
- Gallagher R, Collins S, Trujillo J, et al. Characterization of the continuous, differentiating myeloid cell line (HL-60) from a patient with acute promyelocytic leukemia. Blood. 1979;54(3):713–733.
- Oćwieja M, Adamczyk Z, Morga M, et al. High density silver nanoparticle monolayers produced by colloid self-assembly on polyelectrolyte supporting layers. J Colloid Interface Sci. 2011;364:39–48. doi:10.1016/j.jcis.2011.07.059
- Murao SI, Gemmell MA, Callaham MF, et al. Control of macrophage cell differentiation in human promyelocytic HL-60 leukemia cells by 1, 25-dihydroxyvitamin D3 and phorbol-12-myristate-13-acetate. Cancer Res. 1983;43(10):4989–4996.
- Collins SJ. The HL-60 promyelocytic leukemia cell line: proliferation, differentiation, and cellular oncogene expression. Blood. 1987;70(5):1233–1244.
- Mosmann T. Rapid colorimetric assay for cellular growth and survival: application to proliferation and cytotoxicity assay. J Immunol Methods. 1983;65:55–63. doi:10.1016/0022-1759(83)90303-4
- Chen F, Kuhn DC, Sun SC, et al. Dependence and reversal of nitric oxide production on NF-κ-B in silica and lipopolysaccharide induced macrophages. Biochem Biophys Res Commun. 1995;214(3):839–846. doi:10.1006/bbrc.1995.2363
- Ellman GL. A colorimetric method for determining low concentrations of mercaptans. Arch Biochem Biophys. 1958;74(2):443–450. doi:10.1016/0003-9861(58)90014-6
- Bubniene U, Oćwieja M, Bugelyte B, et al. Deposition of gold nanoparticles on mica modified by poly(allylamine hydrochloride) monolayers. Colloids Surf A. 2014;441:204–210. doi:10.1016/j.colsurfa.2013.08.058
- Unfried K, Albrecht C, Klotz LO, et al. Cellular responses to nanoparticles: target structures and mechanisms. Nanotoxicology. 2007;1(1):52–71. doi:10.1080/00222930701314932
- Aillon KL, Xie Y, El-Gendy N, et al. Effects of nanomaterial physicochemical properties on in vivo toxicity. Adv Drug Deliv Rev. 2009;61(6):457–466. doi:10.1016/j.addr.2009.03.010
- Pan Y, Leifert A, Ruau D, et al. Gold nanoparticles of diameter 1.4 nm trigger necrosis by oxidative stress and mitochondrial damage. Small. 2009;5(18):2067–2076. doi:10.1002/smll.200900466
- Shukla R, Bansal V, Chaudhary M, et al. Biocompatibility of gold nanoparticles and their endocytotic fate inside the cellular compartment: a microscopic overview. Langmuir. 2005;21(23):10644–10654. doi:10.1021/la0513712
- Villiers CL, Freitas H, Couderc R, et al. Analysis of the toxicity of gold nano particles on the immune system: effect on dendritic cell functions. J Nanoparticle Res. 2010;12(1):55–60. doi:10.1007/s11051-009-9692-0
- Goodman CM, McCusker CD, Yilmaz T, et al. Toxicity of gold nanoparticles functionalized with cationic and anionic side chains. Biocon Chem. 2004;15(4):897–900. doi:10.1021/bc049951i
- Hemingway RW, Laks PE, Branham SJ. Plant polyphenols: synthesis, properties. Significance. 1992;59:429–432. doi:10.1007/978-1-4615-3476-1
- Liao J, Zhang Y, Yu W, et al. Linear aggregation of gold nanoparticles in ethanol. Colloids Surf A. 2003;223(1):177–183. doi:10.1016/S0927-7757(03)00156-0
- Sivaraman SK, Kumar S, Santhanam V. Room-temperature synthesis of gold nanoparticles – size-control by slow addition. Gold Bull. 2010;43(4):275–286. doi:10.1007/BF03214997
- Sivaraman SK, Elango I, Kumar S, et al. A green protocol for room temperature synthesis of silver nanoparticles in seconds. Curr Sci. 2009;97(7):1055–1059.
- Dadosh T. Synthesis of uniform silver nanoparticles with a controllable size. Mat Lett. 2009;63(26):2236–2238. doi:10.1016/j.matlet.2009.07.042
- Dutta A, Dolui SK. Tannic acid assisted one step synthesis route for stable colloidal dispersion of nickel nanostructures. Appl Surf Sci. 2011;257(15):6889–6896. doi:10.1016/j.apsusc.2011.03.025
- Sánchez-Moreno C, Larrauri JA, Saura-Calixto F. Free radical scavenging capacity and inhibition of lipid oxidation of wines, grape juices and related polyphenolic constituents. Food Res Int. 1999;32(6):407–412. doi:10.1016/S0963-9969(99)00097-6
- Khan NS, Ahmad A, Hadi SM. Anti-oxidant, pro-oxidant properties of tannic acid and its binding to DNA. Chem Biol Interact. 2000;125:177–189. doi:10.1016/S0009-2797(00)00143-5
- Chung KT, Wong TY, Wei CI, et al. Tannins and human health: a review. Crit Rev Food Sci Nutr. 1998;38:421–464. doi:10.1080/10408699891274273
- Akiyama H, Fujii K, Yamasaki O, et al. Antibacterial action of several tannins against Staphylococcus aureus. J Antimicrobial Chemother. 2001;48(4):487–491. doi:10.1093/jac/48.4.487
- Kim TJ, Silva JL, Kim MK, et al. Enhanced antioxidant capacity and antimicrobial activity of tannic acid by thermal processing. Food Chem. 2010;118(3):740–746. doi:10.1016/j.foodchem.2009.05.060
- Kim TJ, Silva JL, Jung YS. Enhanced functional properties of tannic acid after thermal hydrolysis. Food Chem. 2011;126(1):116–120. doi:10.1016/j.foodchem.2010.10.086
- Ralph P, Moore MA, Nilsson K. Lysozyme synthesis by established human and murine histiocytic lymphoma cell lines. J Exp Med. 1976;143(6):1528–1533.
- Harris P, Ralph P. Human leukemic models of myelomonocytic development: a review of the HL-60 and U937 cell lines. J Leukocyte Biol. 1985;37(4):407–422.
- Minta JO, Pambrun L. In vitro induction of cytologic and functional differentiation of the immature human monocytelike cell line U-937 with phorbol myristate acetate. Am J Pathol. 1985;119(1):111–126.
- Olsson I, Gullberg U, Ivhed I, et al. Induction of differentiation of the human histiocytic lymphoma cell line U-937 by 1α, 25-dihydroxycholecalciferol. Cancer Res. 1983;43(12):5862–5867.
- Olins AL, Herrmann H, Lichter P, et al. Retinoic acid differentiation of HL-60 cells promotes cytoskeletal polarization. Exp Cell Res. 2000;254(1):130–142. doi:10.1006/excr.1999.4727
- Ip SH, Cooper RA. Decreased membrane fluidity during differentiation of human promyelocytic leukemia cells in culture. Blood. 1980;56(2):227–232.
- Barbasz A, Oćwieja M. Cytotoxic activity of highly purified silver nanoparticles sol against cells of human immune system. Appl Biochem Biotechnol. 2015;176(3):817–834. doi:10.1007/s12010-015-1613-3
- Hamiza OO, Rehman MU, Tahir M, et al. Amelioration of 1,2 dimethylhydrazine (DMH) induced colon oxidative stress, inflammation and tumor promotion response by tannic acid in Wistar rats. Asian Pac J Cancer Prev. 2012;13:4393–4402. doi:10.7314/APJCP.2012.13.9.4393
- Khan NS, Ahmad A, Hadi SM. Anti-oxidant, pro-oxidant properties of tannic acid and its binding to DNA. Chemico-Biol Interac. 2000;125(3):177–189. doi:10.1016/S0009-2797(00)00143-5
- Koleckar V, Kubikova K, Rehakova Z, et al. Condensed and hydrolysable tannins as antioxidants influencing the health. Mini Rev Med Chem. 2008;8:436–447. doi:10.2174/138955708784223486
- Serrano J, Puupponen-Pimia R, Dauer A, et al. Tannins: current knowledge of food sources, intake, bioavailability and biological effects. Mol Nutr Food Res. 2009;53:S310–S329. doi:10.1002/mnfr.200900039
- Buzzini P, Arapitsas P, Goretti M, et al. Antimicrobial and antiviral activity of hydrolysable tannins. Mini Rev Med Chem. 2008;8:1179–1187. doi:10.2174/138955708786140990
- Payne DE, Martin NR, Parzych KR, et al. Tannic acid inhibits Staphylococcus aureus surface colonization in an IsaA dependent manner. Infect Immun. 2013;81:496–504. doi:10.1128/IAI.00877-12
- Holderness J, Hedges JF, Daughenbaugh K, et al. Response of gammadelta T Cells to plant-derived tannins. Crit Rev Immunol. 2008;28:377–402. doi:10.1615/CritRevImmunol.v28.i5.20
- Chang TL, Wang CH. Combination of quercetin and tannic acid in inhibiting 26S proteasome affects S5a and 20S expression and accumulation of ubiquitin resulted in apoptosis in cancer chemoprevention. Biol Chem. 2012;5(11 Supplement):A59–A59. doi:10.1515/hsz-2012-0277
- Chen KS, Hsiao YC, Kuo DY, et al. Tannic acid-induced apoptosis and -enhanced sensitivity to arsenic trioxide in human leukemia HL-60 cells. Leuk Res. 2009;33:297–307. doi:10.1016/j.leukres.2008.08.006
- Khan NS, Hadi SM. Structural features of tannic acid important for DNA degradation in the presence of Cu(II). Mutagenesis. 1998;13:271–274. doi:10.1093/mutage/13.3.271
- Sun Y, Zhang T, Wang B, et al. Tannic acid, an inhibitor of poly(ADP-ribose) glycohydrolase, sensitizes ovarian carcinoma cells to cisplatin. Anticancer Drugs. 2012;23:979–990. doi:10.1097/CAD.0b013e328356359f
- Persillin RM, Ziff M. The effect of gold salt on lysosomal enzymes of the peritoneal macrophage. Arthritis Rheum. 1966;9,57–65. doi:10.1002/art.1780090107
- Hostynek JJ. Gold: an allergen of growing significance. Food Chem Toxicol. 1997;35(8):839–844. doi:10.1016/S0278-6915(97)00058-6
- Danscher G. In vivo liberation of gold ions from gold implants. Autometallographic tracing of gold in cells adjacent to metallic gold. Histochem Cell Biol. 2002;117(5):447–452. doi:10.1007/s00418-002-0400-8
- Park EJ, Yi J, Kim Y, et al. Silver nanoparticles induce cytotoxicity by a Trojan-horse type mechanism. Toxicol Vitro. 2010;24(3):872–878. doi:10.1016/j.tiv.2009.12.001
- Möhlen KH, Beller FK. Use of radioactive gold in the treatment of pleural effusions caused by metastatic cancer. J Cancer Res Clin Oncol. 1979;94(1):81–85. doi:10.1007/BF00405352
- Rosenberg SJ, Loening SA, Hawtrey CE, et al. Radical prostatectomy with adjuvant radioactive gold for prostatic cancer: a preliminary report. J Urol. 1985;133(2):225–227.