Abstract
Nanozyme has been one of the most promising artificial enzymes for substitute natural enzymes. The main research focus in this field is developing the nanozymes with high activity, sensitivity and stability. In this paper, prussian blue/polyacrylonitrile (PB/PAN) composite nanofiber was prepared by electrospinning which was calcined to obtain iron oxide/carbon (FeOx/C) composite nanozyme. The structure of the prepared FeOx/C nanozyme was characterized by scanning electron microscopy (SEM), X-ray diffractometer (XRD), Energy dispersive spectrometer (EDS) and X-ray photoelectron spectra (XPS). The results revealed that FeOx/C nanozyme containing the catalytic active species of γ-Fe2O3 possessed peroxidase-like catalytic activity. The catalytic activity of prepared nanozyme which was influenced by different content of PB and different calcination temperatures was investigated. The optimal PB content in the PB/PAN nanofiber and calcination temperature were 41.5% and 600 °C in this research. The prepared FeOx/C nanozyme possessed highly sensitive detection of ascorbic acid. This research provides a new approach to fabricate iron oxide/carbon composite nanozyme with highly catalytic activity and sensitive for colorimetric sensor.
1. Introduction
Enzymes with the characteristic of high specificity and highly catalytic efficiency are considered as green catalysts which are widely used in many fields[Citation1,Citation2]. However, the limitations of stringent using conditions, high cost and hard to storage restrict their applications[Citation3,Citation4]. In recent years, artificial enzymes have received more and more attentions which were used to mimic the catalytic properties of natural enzymes[Citation5,Citation6]. Among the artificial enzymes, nanozymes which are prepared by nanomaterials with enzyme-mimic activity has been one of the most promising substitutes of natural enzymes since the unexpected discovery of peroxidase-like activities of magnetic Fe3O4 nanoparticles[Citation7]. In recent years, nanozyme has been a focus of research in the field of high-performance enzyme mimic catalysts due to its simpler structure than natural enzymes, stronger environmental tolerance, lower cost in preparation, preservation and use, and easier recovery[Citation8,Citation9]. Until now, four types of redox enzymes including peroxidase, oxidase, catalase, and superoxide dismutase have been reported to be mimicked by nanomaterials[Citation10–13].
Numerous artificial electron mediators have been reported to prepare artificial enzymes. Prussian blue (PB) has been used to construct the first generation of electrochemical enzyme biosensor due to its excellent electrochemical and catalytic properties[Citation14,Citation15]. PB contains redox binding sites in its molecular structure, a reversible redox transformation that often occurs at these active sites. Karyakin et al. [Citation16] compared the catalytic activity of PB and horseradish peroxidase (HRP) in the construction of electrochemical glucose biosensor. And they proposed that PB was “artificial peroxidase”[Citation17]. Gu et al. [Citation18] reported that PB could improve the catalytic activity of γ-Fe2O3 peroxidase. In further studies, they found that PB nanoparticles themselves also had peroxidase-like activity under acidic conditions. The catalytic constant of PB nanoparticles is 4 times larger than that of Fe3O4 nanoparticles when 3,3′,5,5′-tetramethylbenzidine (TMB) was used as the substrate. However, PB nanoparticles are easy to aggregate which influences their catalytic activity. And they are not easy to recycle and reuse limiting their applications. Thus, it is important to avoid aggregation and improve the reusability of PB nanoparticles by suitable carriers[Citation19,Citation20]. Electrospun nanofibrous membranes have the advantages of small diameter, large specific surface area, porous structure, low mass transfer resistance and reusability[Citation21], making them as the excellent catalyst carrier. Electrospun nanofibrous membranes have been extensively used for enzyme immobilization such as hydrogen peroxide [Citation22], lipase [Citation23,Citation24], and glucose [Citation25]. Numerous polymers have been used to be electrospun the nanofiber as the catalyst carrier, such as polypyrrole, poly(vinylpyrrolidone) and polyacrylonitrile (PAN)[Citation26–28]. Among them, PAN possesses the advantages of excellent mechanical strength and high thermal resistance, which has been extensively studied to be electrospun nanofibrous membranes as the support for enzyme immobilization. PAN has also been reported as a precursor of carbon fiber, which is widely used in various research fields due to its large specific surface area, easy machining, strong toughness and good electrical conductivity.
In this paper, the PB/PAN composite nanofiber was prepared by electrospinning which was calcined at different temperatures under the N2 atmosphere to obtain FeOx/C nanozyme. The catalytic activity of the prepared FeOx/C nanozyme was determined by the catalytic chromogenic reaction of 3,3′,5,5′-tetramethylbenzidine (TMB). The structure of the prepared FeOx/C nanozyme was characterized by scanning electron microscopy (SEM), X-ray diffractometer (XRD), Energy Dispersive Spectrometer (EDS) and X-ray photoelectron spectra (XPS). The prepared FeOx/C nanozyme was also used for ascorbic acid detection. This research provides a new approach to fabricate iron oxide/carbon composite nanozyme with high catalytic activity and sensitive for colorimetric sensor.
2. Experimental
2.1. Materials
Polyacrylonitrile (PAN, Mw = 105g/mol) was purchased from Courtaulds Co., Ltd (UK). Prussian blue (PB), dimethylformaide (DMF), absolute ethanol and sodium hydroxide were purchased from Sinopharm Chemical Reagent Co., Ltd (Shanghai, China). ascorbic acid, 3,3′,5,5′-tetramethylbenzidine (TMB), phosphoric acid, glacial acetic acid, and hydrogen peroxide solution (30%) were purchased from Aladdin reagent (Shanghai) Co., Ltd (Shanghai, China).
2.2. Prepartion of FeOx/C nanozyme
20 mL DMF was added into the 50 mL conical flask, and then a certain amount of PB particles were added into DMF which were dissolved by ultrasonic oscillation 2 ∼ 3 h. 2.5 g PAN powder was added to the solution which was magnetic stirred for 24 h to obtain the uniform spinning solution. After then the spinning solution was electrospun under the temperature of 20 °C and the humidity of 65% with the electrospinning process parameters setting at applied voltage of 20 kV, the flow rate of the spinning solution of 0.3 mL/h and the distance between needle tip and collector of 18 cm. After a electrospinning time over 12 h, the PB/PAN nanofibrous membrane was peeled from the aluminum foil and kept into the desiccator.
PB/PAN nanofibrous membrane was calcined using the quartz tubular furnace which was heated to the target temperature with the heating rate of 2 °C/min and kept for 6 h at this temperature under the protection of N2 gas. After that the FeOx/C nanozyme was obtained.
2.3. Colorimetric detection of catalytic activity and ascorbic acid
The peroxidase-like catalytic activity of FeOx/C nanozyme was determined by the catalytic oxidation of TMB in the TMB/H2O2 mixed solution. 40 μL TMB solution (250 μM TMB in ethanol) and 40 μL H2O2 solution (100 μM H2O2 in ethanol) were added into the 6 mL acetate buffer solution (CH3COOH-CH3COONa buffer solution, pH = 3.5) to construct the TMB/H2O2 mixed solution. And then 1 mg nanozyme was added into the TMB/H2O2 mixed solution which reacted in the 30 °C water bath thermostatic oscillator for 10 min. After that the absorbance at the wavelength of 652 nm of reaction solution was determined by the ultraviolet-visible spectrophotometer.
The detection of ascorbic acid was also by the colorimetric method using the above TMB/H2O2 mixed solution. And then 0.4 mL of different concentration ascorbic acid solution was added into the TMB/H2O2 mixed solution, respectively. And then 1 mg nanozyme was added into the above mixed solution which reacted in the 30 °C water bath thermostatic oscillator for 10 min. After that the absorbance at the wavelength of 652 nm of reaction solution was determined by the UV-5500 PC ultraviolet-visible (UV-vis) spectrophotometer (Shanghai Metash Instrument Co., Ltd). The surface area and pore diameter of FeOx/C nanozyme was detemined by Brunner Emmet Teller (BET) method using the NOVA 2000e specific surface and porosity analyzer (Quantachrome, USA). The apparent kinetic parameters were obtained from the Michaelis-Menten equation and the derived Lineweaver-Burk plot, which exhibited as 1/ν = Km/Vmax﹒(1/[S] + 1/Km), where ν is the initial reaction velocity, Km is the Michaelis constant, Vmax is maximal initial reaction velocity, [S] is the substrate concentration.
2.4. Characterization of FeOx/C nanozyme structure
The morphology of PB/PAN nanofiber and nanozyme was recorded by a Hitachi S-4800 scanning electron microscopy (SEM, Hitachi, Japan) after being sputtered with gold for 20 s. The crystalline phase of PB/PAN nanofiber and nanozyme was performed on a D8 X-ray diffractometer (XRD, Brucker, Germany) with Cu-Kα radiation. Energy Dispersive Spectrometer (EDS) was used to determine element content by the Scanning Electron Microscope-Energy Spectrometer (SEM-EDS). X-ray photoelectron spectra (XPS) was conducted on a thermo ESCALAB 250 spectrometer.
3. Results and discussion
3.1. Influence of the catalytic activity of FeOx/C nanozyme
The catalytic activity of FeOx/C nanozyme would be influenced by PB content in the composite nanofiber. Therefore, the effect of PB content on the nanozyme activity was investigated. The mass percent of PB in the PB/PAN nanofiber was 17%, 29%, 38%, 41.5%, 44%, 47% and 50%. And the catalytic activities of FeOx/C nanozymes with different content PB in PB/PAN nanofiber were shown in .
Figure 1. (a) Catalytic activities of FeOx/C nanozymes with different content of PB, (b) Effect of calcination temperature on catalytic activity of FeOx/C nanozyme, (c) Effect of pH on catalytic activity of FeOx/C nanozyme.
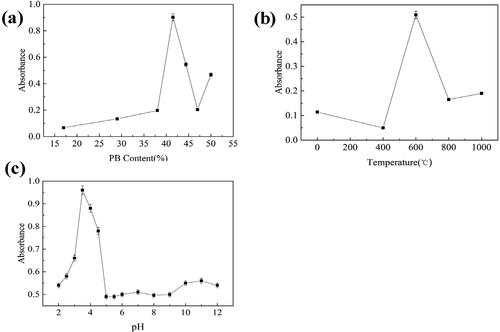
As can be seen from , the catalytic activities of FeOx/C nanozymes increased with the increasing of PB content from 17% to 41.5%, after that the activity decreased with the increasing of PB content. The FeOx/C nanozymes achieved the highest catalytic activity when the PB content was 41.5%. Therefore, the optimal PB content in the PB/PAN nanofiber was 41.5% for the subsequent experiment.
shows the different calcination temperature effect on catalytic activities of FeOx/C nanozymes. The calcination temperatures were chosen 400 °C, 600 °C, 800 °C and 1000 °C. As can be seen from , the catalytic activity of FeOx/C nanozyme increased with the increasing of calcination temperature below 600 °C, after that it decreased when the calcination temperature was over 600 °C. The highest catalytic activity of FeOx/C nanozyme was obtained when the calcination temperature was 600 °C. The reason about this maybe due to the main catalytic active species was magnetic γ-Fe2O3. The PB particles would convert to magnetic γ-Fe2O3 when they were heated close to the Curie point of 585 °C of the ferromagnetic material Fe2+/3+. Further increasing the calcination temperature higher than 650 °C, they would convert to non-magnetic α-Fe2O3 with no catalytic property. Therefore, the optimal calcination temperature of PB/PAN nanofiber was 600 °C in this research.
shows the pH of catalytic system effect on catalytic activities of FeOx/C nanozymes. The catalytic activity of FeOx/C nanozyme increased with the increase of pH value from 2 to 3.5. When the pH was higher than 3.5, the catalytic activity of nanozyme decreased with the pH value. The FeOx/C nanozyme showed negligible activity when the pH was higher than 5. There also exist the interesting phenomenon, the FeOx/C nanozyme showed a certain catalase-like catalytic activity when the pH value was higher than 10. Therefore, the FeOx/C nanozyme exhibited the high peroxidase-like activity under acidic condition, while it exhibited the weak catalase-like activity under the alkaline condition. The optimal pH value of the FeOx/C nanozyme catalytic system was chosen 3.5 for the subsequent experiment in this research.
3.2. Characterization of the FeOx/C nanozyme structure
shows the SEM images of PB/PAN nanofiber and nanozyme. As can be seen from , the electrospun PB/PAN nanofiber possessed uniform size with the diameter in the nanometer scale, and the electrospun PB/PAN nanofibers were generally arranged in one directional order. As for the magnification images of the PB/PAN nanofiber, it showed the rough surface possessing fishbone structure with porous, and there exist the nanoparticles on its surface. The reason may be due to the PB nanoparticles dispersed on the PAN nanofiber carrier which would prevent the solvent to volatilize, thus forming a porous structure. shows the morphology of the horizontal and vertical direction of PB/PAN nanofiber after the calcination. The FeOx/C nanozyme preserved the original fishbone structure similar with PB/PAN nanofiber. It believes that this porous structure is benefit for the catalytic active species to expose on the surface of FeOx/C nanozyme improving the catalytic efficiency.
shows the XRD spectra of PB/PAN nanofiber and nanozyme. As can be seen from , there existed the peaks at 15.10°, 17.25°, 22.54°, 35.11°, 54.14° of PB/PAN nanofiber corresponding to the diffraction peaks of (110), (211), (210) and (422) lattice planes of PB. As for FeOx/C nanozyme, the characteristic peaks of PB at 15.23°, 17.28°, 22.85° still exist, while the peak intensities decreased. There are the peaks at 30.71° and 36.07° which belong to the characteristic diffraction peaks of γ-Fe2O3 as the main catalytic species. And there also exist the weak peaks of 43.72°, 65.40°, 81.09° which are assigned to the (110), (220) and (211) lattice planes of α-Fe2O3. These results revealed that the calcined treatment of PB/PAN nanofiber at 600 °C would make the most of PB convert to γ-Fe2O3 and a certain amount of α-Fe2O3, while there still a small amount of PB kept it origin state.
EDS was used to determine element content of PB/PAN nanofiber and nanozyme which as shown in . As can be seen from , four elements of C, N, O and Fe were detected in PB/PAN nanofiber, and C and N element accounted for the main proportion which were from the PAN substrate. A certain amount of Fe element was detected in PB/PAN nanofiber which was from the PB. As for FeOx/C nanozyme, the main element types haven’t changed, while their contents were different from PB/PAN nanofiber. The contents of C and N decreased while the content of Fe element increased. The reason about this maybe due to the calcination of PB/PAN nanofiber at 600 °C would make the carbonization of PAN to consume C and N elements. The content of O elements increased greatly comparing PB/PAN nanofiber, this is because the Fe element in PB would convert γ-Fe2O3 and α-Fe2O3. These results could also demonstrate the iron oxides were existence in FeOx/C nanozyme. The surface area and pore diameter of FeOx/C nanozyme were shown in . As shown in , the surface area of FeOx/C nanozyme was 78.28 m2/g, and its pore diameter was 3.435 nm showing FeOx/C nanozyme possessed high surface area.
Table 1. The surface area and pore diameter of FeOx/C nanozyme.
XPS was used to determine the chemical composition of PB/PAN nanofiber and nanozyme which as shown in . As can be seen from , the full survey spectrum of PB/PAN nanofiber and nanozyme show that there exist the C, N, O, Fe elements. show the Fe 2p spectrum of them, there exist two major peaks at 711.0 and 724.6 eV corresponding to Fe 2p3/2 and Fe 2p1/2, respectively. The peaks intensity of Fe 2p of FeOx/C nanozyem was higher than that of PB/PAN nanofiber indicating there are more Fe content in nanozyme than nanofiber after the calcination at 600 °C. Base on the above analysis results, the PB/PAN nanofiber would convert to PB/PAN nanozyme containing the catalytic active species of γ-Fe2O3.
3.3. Catalytic activity of FeOx/C nanozyme
The peroxidase catalytic activity of FeOx/C nanozyme was determined by the catalytic oxidation reaction of TMB and the photographs of TMB or H2O2 solution with or without nanozyme which were shown in . As can be seen from , the color of TMB solution in presence of H2O2 turns blue after 10 min by the catalytic active FeOx/C nanozyem. As for its UV-vis adsorption spectra curve, there exist the absorption peak at 652 nm belonging to the characteristic absorption peak of oxidation state of TMB. This result revealed that FeOx/C nanozyme possessed the peroxidase catalytic activity. It is worth to note that, the TMB solution also could turn light blue when O2 was used to instead of H2O2. There was the weak absorption peak at 652 nm with lower absorbance comparing with the FeOx/C nanozyme, TMB and H2O2 system. This result revealed that FeOx/C nanozyme also showed a certain oxidase-like catalytic activity.
Figure 6. (a) UV-vis spectra of different systems which was determined after reaction for 10 min (b)The photographs of TMB, H2O2 or their mixed solution with nanozyme which reacted in the 30 °C water bath thermostatic oscillator for 10 min. (c) The absorbance changes at 652 nm of the TMB system with different concentration of ascorbic acid.
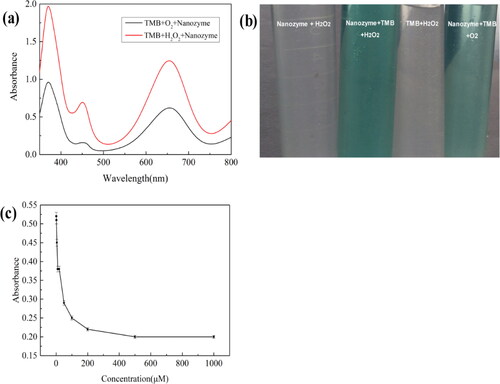
Ascorbic acid is a highly efficient antioxidant which plays a important role in protection our human body[Citation29]. Its concentration also should be precise detection. Ascorbic acid possesses excellent reducing property which could inhibit the catalytic oxidation of TMB. shows the absorbance changes at 652 nm of the TMB system with different concentration of ascorbic acid under the condition of the constant amount of TMB (250 μM), H2O2 (100 μM) and nanozyme (1 mg). As can be seen from , the absorbance at 652 nm decreased with the increasing of ascorbic acid concentration in the range from 0 to 200 μM. The detection of ascorbic acid in this range exhibits high sensitivity. Therefore, FeOx/C nanozyme could be used to precisely detect the concentration of ascorbic acid.
The Lineweaver-Burke method was used to study the kinetic parameters of FeOx/C nanozyme by investigating the relationship between the initial rate of nanozyme reaction (V0) and the different concentration of TMB or H2O2 ([S]), which were shown in . The Km and Vmax of FeOx/C nanozyme from double reciprocal plots were showed in . The Km of horse radish peroxidise (HRP), Fe3O4 nanoparticles and APTES-Fe3O4 which were reported by previous researches were also listed in .
Figure 7. The Lineweaver-Burke plots of FeOx/C toward (a) the TMB concentration and (b) the H2O2 concentration.
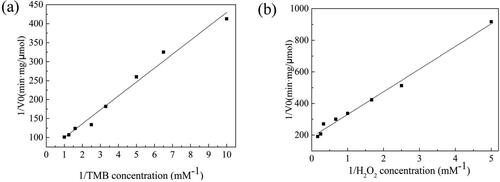
Table 2. The apparent kinetic constant Km and Vmax of the prepared FeOx/C nanozyme.
The Km value is usually to characterize the affinity of enzyme to substrate, and the lower Km value represents the stronger affinity of the catalyst towards the substrate. As shown in , the Km value of FeOx/C nanozyme with TMB as substrate was 0.582 mM, which was higher than that of previously reported HRP and Fe3O4 nanoparticle, while it was lower than APTES-Fe3O4, revealing that FeOx/C nanozyme possess a higher affinity to TMB than APTES-Fe3O4. The Km value of FeOx/C nanozyme with H2O2 as substrate was 0.855 mM which was lower than that of HRP, Fe3O4 nanoparticles, and APTES-Fe3O4 suggesting that FeOx/C nanozyme possessed higher affinity to H2O2. These results demonstrated that the FeOx/C nanozyme possessed higher affinity to TMB and H2O2 as well as excellent catalytic activity.
4. Conclusion
In this paper, FeOx/C composite nanozyme was prepared by electrospinning the PB and PAN composite spinning solution which was then calcined at different temperatures. The structure of the prepared nanozyme was characterized by SEM, XRD, EDS and XPS. The results revealed that FeOx/C nanozyme containing the catalytic active species of γ-Fe2O3 possessed peroxidase-like catalytic activity. The catalytic activity of the prepared nanozyme was determined by the catalytic chromogenic reaction of TMB. The catalytic activity of prepared nanozyme which was influenced by different content of PB and different calcination temperatures. The optimal PB content in the PB/PAN nanofiber and calcination temperature were 41.5% and 600 °C in this research. The prepared nanozyme was also used for ascorbic acid detection. The results showed that this prepared FeOx/C nanozyme possessed high sensitive detection of ascorbic acid. This research would provide a new approach to fabricate nanofiber-based nanozyme with high catalytic activity for colorimetric sensor.
Disclosure statement
No potential conflict of interest was reported by the author(s).
Data availability statement
All data generated or analysed during this study are included in this submitted article. The raw data shall be made available upon request to the corresponding author.
Additional information
Funding
References
- Itoh T, Hanefeld U. Enzyme catalysis in organic synthesis. Green Chem. 2017;19(2):331–332.
- Shoda S, Uyama H, Kadokawa J, et al. Enzymes as green catalysts for precision macromolecular synthesis. Chem Rev. 2016;116(4):2307–2413.
- Yang HQ, Li JH, Shin HD, et al. Molecular engineering of industrial enzymes: recent advances and future prospects. Appl Microbiol Biotechnol. 2014;98(1):23–29.
- Lin YH, Ren JS, Qu XG. Catalytically active nanomaterials: a promising candidate for artificial enzymes. Acc Chem Res. 2014;47(4):1097–1105.
- Schafer JW, Zoi I, Antoniou D, et al. Optimization of the turnover in artificial enzymes via directed evolution results in the coupling of protein dynamics to chemistry. J Am Chem Soc. 2019;141(26):10431–10439.
- Breslow R. Biomimetic chemistry and artificial enzymes: catalysis by design. Acc Chem Res. 1995;28(3):146–153.
- Gao L, Zhuang J, Nie L, et al. Intrinsic peroxidase-like activity of ferromagnetic nanoparticles. Nat Nanotechnol. 2007;2(9):577–583.
- Huang Y, Ren J, Qu X. Nanozymes: classification, catalytic mechanisms, activity regulation, and applications. Chem Rev. 2019;119(6):4357–4412.
- Zhang J, Liu J. Light-activated nanozymes: catalytic mechanisms and applications. Nanoscale. 2020;12(5):2914–2923.
- Wu J, Wang X, Wang Q, et al. Nanomaterials with enzyme-like characteristics (nanozymes): next-generation artificial enzymes (II). Chem Soc Rev. 2019;48(4):1004–1076.
- Wei H, Wang E. Nanomaterials with enzyme-like characteristics (nanozymes): next-generation artificial enzymes. Chem Soc Rev. 2013;42(14):6060–6093.
- Wang X, Gao XJ, Qin L, et al. eg occupancy as an effective descriptor for the catalytic activity of perovskite oxide-based peroxidase mimics. Nat Commun. 2019;10(1):704.
- Shen X, Wang Z, Gao X, et al. Density functional Theory-Based method to predict the activities of nanomaterials as peroxidase mimics. ACS Catal. 2020;10(21):12657–12665.
- Zeng J, Wei W, Liu X, et al. A simple method to fabricate a prussian blue nanoparticles/carbon nanotubes/poly (1, 2-diaminobenzene) based glucose biosensor. Microchim Acta. 2008;160(1–2):261–267.
- Chen J, Wang Q, Huang L, et al. Prussian blue with intrinsic heme-like structure as peroxidase mimic. Nano Res. 2018;11(9):4905–4913.
- Karyakin AA, Gitelmacher OV, Karyakina EE. Prussian blue-based first-generation biosensor. A sensitive amperometric electrode for glucose. Anal Chem. 1995;67(14):2419–2423.
- Karyakin AA, Karyakina EE, Gorton L. Amperometric biosensor for glutamate using Prussian blue-based “artificial peroxidase” as a transducer for hydrogen peroxide. Anal Chem. 2000;72(7):1720–1723.
- Zhang XQ, Gong SW, Zhang Y, et al. Prussian blue modified iron oxide magnetic nanoparticles and their high peroxidase-like activity. J Mater Chem. 2010;20(24):5110–5116.
- Wu J, Yin F. Sensitive enzymatic glucose biosensor fabricated by electrospinning composite nanofibers and electrodepositing Prussian blue film. Electroanal Chem. 2013;694:1–5.
- Komkova MA, Karyakina EE, Karyakin AA. Catalytically synthesized Prussian blue nanoparticles defeating natural enzyme peroxidase. J Am Chem Soc. 2018;140(36):11302–11307.
- Song W, Zhao B, Wang C, et al. Electrospun nanofibrous materials: a versatile platform for enzyme mimicking and their sensing applications. Compos Commun. 2019;12:1–13.
- Miao YE, He S, Zhong Y, et al. A novel hydrogen peroxide sensor based on Ag/SnO2 composite nanotubes by electrospinning. Electrochim Acta. 2013;99:117–123.
- Liu X, Fang Y, Yang X, et al. Electrospun nanofifibrous membranes containing epoxy groups and hydrophilic polyethylene oxide chain for highly active and stable covalent immobilization of lipase. Chem Engin J. 2018;336:456–464.
- Liu X, Fang Y, Yang X, et al. Electrospun epoxy-based nanofibrous membrane containing biocompatible feather polypeptide for highly stable and active covalent immobilization of lipase. Colloids Surf B Biointerfaces. 2018;166:277–285.
- Ren G, Xu X, Liu Q, et al. Electrospun poly (vinyl alcohol)/glucose oxidase biocomposite membranes for biosensor applications. React Funct Polym. 2006;66(12):1559–1564.
- Jiang Y, Nie G, Chi M, et al. Synergistic effect of ternary electrospun TiO2/Fe2O3/PPy composite nanofibers on peroxidase-like mimics with enhanced absorbance performance. RSC Adv. 2016;6(37):31107–31113.
- Song W, Yang Z, Ma F, et al. Electrospun magnetic CoFe2O4/Ag hybrid nanotubes for sensitive SERS detection and monitoring of the catalytic degradation of organic pollutants. RSC Adv. 2017;7(64):40334–40341.
- Zhang Z, Jiang Y, Chi M, et al. Electrospun polyacrylonitrile nanofibers supported alloyed Pd-Pt nanoparticles as recyclable catalysts for hydrogen generation from the hydrolysis of ammonia borane. RSC Adv. 2015;5(114):94456–94461.
- Lou Z, Zhao S, Wang Q, et al. N-doped carbon as peroxidase-like nanozymes for total antioxidant capacity assay. Anal Chem. 2019;91(23):15267–15274.
- Lu N, Zhang M, Ding L, et al. Yolk-shell nanostructured Fe3O4@C magnetic nanoparticles with enhanced peroxidase-like activity for label-free colorimetric detection of H2O2 and glucose. Nanoscale. 2017;9(13):4508–4515.
- Zhu H, Hu Y, Jiang G, et al. Peroxidase-like activity of aminopropyltriethoxysilane-modified iron oxide magnetic nanoparticles and its application to clenbuterol detection. Eur Food Res Technol. 2011;233(5):881–887.