Abstract
Regorafenib (Reg) inhibits the growth of liver cancer cells in vitro and animal model. However, due to its poor bioavailability, its potential as a chemopreventive or therapeutic drug is severely restricted. In this work, we developed two environmentally friendly delivery systems by synthesizing Regorafenib-gold nanoparticles conjugates Reg@GNPs1 and Reg@GNPs2, employing a dual role of Reg to reduce Au3+ and stabilize the synthesized GNPs. UV-Vis’s spectroscopy, Fourier transform infrared spectroscopy, and Powder-XRD verified the fabrication of Reg@GNPs. Reg@GNPs1 and Reg@GNPs2 were both found to be spherical and uniform in size (10 ± 2 and 2 ± 33 nm, respectively) using transmission electron microscopy. Similar negative zeta potential (−35.0 ± 2.5 and −37.0 ± 1.6 mV) was observed by dynamic light scattering analysis, even though the hydrodynamic diameter of the nanoconjugates ranged from 65.0 ± 1.7 to 153.0 ± 2.2 nm. Reg@GNPs1 and Reg@GNPs2 were calculated to have a Reg loading of 46% and 48%, respectively. Selectivity towards the non-cancerous cell line (L929) cells, whereas the MTT assay in vitro showed the antiproliferative effects of Reg@GNPs on three liver carcinoma (Hep3B, BEL7402, and HepG2) cell lines. Several fluorescent staining techniques were used to examine liver cancer cell morphology. Flow cytometric analysis confirmed that the effects of the superior Reg@GNPs nanoconjugate on cell proliferation than free Reg. In conclusion, the acquired results show that the novel synthesized GNPs loaded with Reg are stable as an anticancer agent, with minimal toxicity against non-cancerous cells, as determined by cytotoxicity and IC50 evaluations.
1. Introduction
Cancer is widely recognized as a significant global mortality; millions of new cases are recorded yearly. Liver cancer is a challenging disease, ranking the sixth most common cancer diagnosed (906,000 cases) and the third leading cause of cancer fatalities (830,000 deaths) globally [Citation1]. This malignancy has a disproportionately high fatality rate in Asian and North African nations. The most prevalent form of liver cancer, hepatocellular carcinoma (HCC), is notoriously challenging to treat because it is sometimes hard to diagnose before tumours have formed [Citation2]. A 5-year survival rate of around 20% may be expected for those diagnosed with liver cancer (34% for localized cases and 3% for distant cases) [Citation3]. In several instances, it is not possible to do surgery or transplant the liver, which would dramatically improve survival statistics. There are a few therapeutic alternatives when resection is not possible, and while they may delay cancer’s progression, they seldom cause it to go into remission [Citation4]. Patients with HCC get chemotherapy by infusion into the hepatic artery, intended to reduce systemic adverse effects. The healthy liver cells will break down a significant amount of the dosage, lowering the effective dose that reaches the tumour cells when cancer medicines are administered in this manner. Treatment-related adverse symptoms, including hair loss, nausea, and fatigue, persist despite this lowering of the chemotherapeutic in the remainder of the body. Recent years have shown promise for targeted treatment, which includes blocking kinases that promote tumour growth or blood flow [Citation5]. Sorafenib and Lenvatinib are two targeted medications that have gained approval in recent years for treating liver cancer. In addition to surgery and radiation, immunotherapy has shown promise in treating liver cancer. The capacity of malignancy to elude the immune response can be compromised by targeting a protein (such as programmed cell death protein 1) on T cells or the equivalent binder on cancer cells [Citation6]. Compared to standard treatments like chemotherapy and radiation, targeted and immunological therapies have enhanced therapeutic efficacy, but their effects take time to manifest, and not all patients react to them. Patients with liver cancer who are not candidates for surgery have a pressing need for improved therapeutic choices [Citation7].
Though most cancer drug nanoparticle (NP) formulations include a nanocarrier and an active agent, specific drug-only NP formulations have been developed. Because of their increased metabolic stability, membrane permeability, bioavailability, and sustained action, nano-drug systems are favourable [Citation8–10]. Anti-cancer drugs may now be delivered to both the tissue and the cellular level using nano transporters, improving their efficacy. Whether a drug is absorbed through the mucosa or the skin depends on its size, surface charge, and hydrophobicity [Citation11–13]. Transcellular uptake is enhanced by particle size, with smaller NPs eliciting more absorption than their bigger counterparts. However, more significant than 300 nm NPs were not absorbed by intestinal cells. Only NPs with a size of less than 500 nm may go through the body’s circulatory system [Citation14–16]. Due to their exceptional optical characteristics, high chemical stability, and biocompatibility, gold nanoparticles (GNPs) as nano-materials have found widespread application in many industries. As a result, it has promising future uses in several fields, including nano-electronics, nano-photonics, catalysis, sensors, biomarkers, and many more. The high surface area and uniform sphericity of GNPs make them a promising candidate for the delivery of anticancer medicines [Citation17–19]. Many GNPs complexes have also been developed for a novel class of anticancer medicines for cancer treatment. GNPs complex is a potential antineoplastic drug carrier because it influences gene expression, detects analytes in cells, and governs cell function. As a result, research into better cancer treatments is seeing an increase in the importance of functionalized GNPs [Citation20–22].
Regorafenib, an oral multikinase inhibitor, has been sanctioned by the United States Food and Drug Administration (USFDA) as third- or later-line treatments for patients who respond poorly to first- and second-line treatments. Its authorization was based on the clinical trial [Citation23]. It is also accepted by the European Medicines Agency and the China Food and Drug Administration. Recently, another oral VEGFR inhibitor, fruquintinib, was also approved by the USFDA for third-line treatment of mCRC [Citation24]. The quality of patient’s lives can be enhanced by oral chemotherapy [Citation23, Citation24]. Nearly seventy oral small-molecule protein kinase inhibitors have been utilized in chemotherapy administered by mouth in the decades since [Citation25]. Yet, the chemotherapeutic impact of these drugs remains restricted by poor bioavailability due to their poor water solubility, poor epithelial permeability, pre-systemic metabolism, and undesirable biodistribution. Better therapeutic effectiveness from chemotherapeutic medicines requires improved formulations that better control their pharmacokinetic characteristics [Citation26–28]. Regorafenib (Reg) is an oral multikinase inhibitor that inhibits vascular endothelial growth factor receptors (VEGFR), tyrosine kinase with immunoglobulin and epidermal growth factor homology domain 2, platelet-derived growth factor receptor alpha, and fibroblast growth factor receptor [Citation27]. Activity inhibits oncogenic kinases (KIT, RET, RAF-1, BRAF, and mutant BRAF), suppressing cell growth and inducing apoptosis. The FDA has given its blessing for treating mCRC and gastrointestinal stromal cancer. As of 2017, REGO can be used as a second-line treatment for those with HCC who have already been treated with sorafenib [Citation29]. Non-biodegradable microspheres are already available as commercial goods like DC Beads and HepasphereTM for TACE. Positively charged drugs were often protected by an ion exchange method, and the ionic environment of physiological fluids influenced the drug elution kinetics [Citation30].
The advantages of previous research on the fabrication of gold NPs are taken together to develop the Reg@GNPs delivery methods with various diameters of GNPs. We hypothesized that a straightforward, cost-effective, environmentally friendly approach to Reg@GNPs manufacturing would result in stabilized, biocompatible GNPs with a high loading of Reg. Maximum Reg loading onto the GNPs is desired. Based on our findings, our secondary objective was to investigate how much of an impact Reg@GNPs size had on their interaction with liver cancer cells. A panel of human liver cell lines ranging in malignant potential and androgen-responsivity (Hep3B, BEL7402, and HepG2) and non-cancerous L929 cells were used to evaluate the anticancer potential of freshly synthesized Reg@GNPs.
2. Methods and materials
2.1. Materials and cell line maintenance
Regorafenib was purchased from Sigma-Aldrich, Germany. Tetrachloroaurate trihydrate (HAuCl4·3H2O, and Ethanol were purchased from Xi’an Sanpu Chemical Reagent Co., Ltd. (Xi’an, China). Cell culture medium DMEM, 3-(4,5-dimethylthiazol-2-yl)-2,5-diphenyltetrazolium bromide (MTT), trypsin, fetal bovine (FBS), Dimethyl sulfoxide (DMSO) were purchased from TransGen Biotech, Beijing China. Acridine orange (AO), propidium iodide (PI), and DAPI were purchased from Nanjing Jiancheng, China. Phosphate-buffered saline (PBS) and trypsin solution were purchased from Gibco, CA, USA. Annexin V-FITC/PI apoptosis kit was purchased from Beyotime Biotechnology, Shanghai, China. MilliQ water was prepared using a MilliQ system (Bedford, MA, America). All chemicals and solvents were of analytical grade. The magnet was purchased from Niroku Seisakusho Co. Ltd., Japan (strength 240 mT; diameter 7 mm and thickness 1.5 mm).
Human liver cancer cell lines (Hep3B, BEL7402, and HepG2) and non-cancerous cell line (L929) cells were purchased from the Shanghai Institute of Cell Biology in the Chinese Academy of Sciences. The cells were cultured in DMEM medium, respectively, supplemented with 10% fetal bovine serum (FBS) and antibiotics. The cells were cultured under recommended conditions. Cell lines were incubated in a humidified atmosphere of 5% CO2 at 37 °C.
2.2. Formation of Reg@GNPs conjugates
To develop Regorafenib-stabilized GNPs, we used a simple chemical method [Citation31–33], adjusting the starting concentrations of Reg and Au3+. The described synthesis protocol was modified to synthesize GNPs of varying sizes and load as much Reg as possible onto each particle. Conjugates designated Reg@GNPs1 and Reg@GNPs2 were produced by adding 20/25 mg Reg dissolved in 10 mL DMSO to 90 mL of 0.2/0.4 mM HAuCl4·3H2O. After 5 min of boiling and stirring, the reactions in Reg@GNPs1 and Reg@GNPs2 produced their respective violet and brownish-green colours. At room temperature, the prepared conjugates were collected through centrifugation. Residues were re-suspended in methanol or dried to acquire the blackish solid powder, and then the DMSO and unbound Reg were removed during the subsequent centrifugation cycle. The inductively coupled plasma atomic emission spectrometer (ICP-AES) measured 0.03 mM for the Au content of Reg@GNPs1 and 0.04 mM for Reg@GNPs2.
2.3. Characterization of Reg@GNPs conjugates
UV-visible spectroscopy investigations were assessed in a HELIOS-ZETA spectrophotometer. Fourier-transformed infrared (FTIR) spectra were collected in a Thermo Nicolet Nexus spectrometer equipped with a Goldengate attenuated total reflectance (ATR) device. Dynamic Light Scattering (DLS) with the same Malvern instrument measured the hydrodynamic parameter of the nanoparticles. Transmission Electron Microscopy (TEM) was performed with a JEOL JEM 1400 or 2100 instruments (JEOL Ltd., Tokyo, Japan) operated at 120 and 200 kV, respectively, and equipped with a CDD camera (KeenView Camera). Sample preparation was accomplished by separating ca. 1 mg of sample in 1 mL of Ethanol, followed by sonication in a low-power sonicator bath (Selecta, Spain) for 5 min, and succeeding deposition of one drop of the suspension onto carbon-coated copper grids. The amount of cation released from GNPs nanosystems in aqueous media was assayed by Inductively Coupled Plasma Atomic Emission Spectroscopy (ICP-AES) through the Au emission lines at 328.068 and 202.548 nm, respectively, in a Varian Vista AX Pro spectrometer.
2.4. Cell viability assessment by MTT assay
To determine the cytotoxicity, HepG2 (1 × 104 cells), BEL7402 (1 × 104 cells) and Hep3B cells (1 × 104 cells) were seeded into 96-well plates and incubated for 24 h at 37 °C. After being rinsed with PBS, the cells were incubated with Reg, Reg@GNPs1 and Reg@GNPs2 at various concentrations for 24 h at 37 °C. The medium was removed and washed twice with PBS, and the cell viability was determined using a standard MTT. After 4h, the formazan formation examined cell viability as previously reported [Citation34–38].
2.5. Acridine orange and propidium iodide staining
HepG2 (1 × 103 cells), BEL7402 (1 × 103 cells) and Hep3B cells (1 × 103 cells) were cultured in 6-well plates for 24 h. The cells were then exposed to Reg, Reg@GNPs1 and Reg@GNPs2 (IC50 concentration) for 24 h. Then, the cells were stained with acridine orange and propidium iodide (AO-PI) staining according to the manufacturer’s instructions [Citation39–42]. The cells were imaged using an Olympus CKX53 microscope.
2.6. DAPI staining
HepG2 (1 × 103 cells), BEL7402 (1 × 103 cells) and Hep3B cells (1 × 103 cells) were cultured in 6-well plates for 24 h. The cells were then exposed to Reg, Reg@GNPs1 and Reg@GNPs2 (IC50 concentration) for 24 h. Then, the cells were stained with DAPI staining according to the manufacturer’s instructions [Citation43–46]. The cells were imaged using an Olympus CKX53 microscope.
2.7. Apoptosis analysis
HepG2 (1 × 103 cells), BEL7402 (1 × 103 cells) and Hep3B cells (1 × 103 cells) were cultured in 6-well plates for 24 h. The cells were then exposed to Reg, Reg@GNPs1 and Reg@GNPs2 (IC50 concentration) for 24 h. Next, the cells were harvested and stained with Annexin V-FITC and PI (KeyGEN BioTECH). Flow cytometry was performed to analyze these cells [Citation47–49].
2.8. Caspase activity assay
A chromogenic assay using caspase-3, caspase-8 and 9 colorimeter activity assay kits determined the caspase activity assay. HepG2 (1 × 107 cells), BEL7402 (1 × 107 cells) and Hep3B cells (1 × 107 cells) were seeded in a 6-well plate. After incubation for 24-h, the medium was removed, and 2 ml of fresh medium containing Reg, Reg@GNPs1 and Reg@GNPs2 (IC50 concentration), the cells lysed with cell lysis buffer (50 mM HEPES, 100 mM NaCl, 0.1% CHAPS, 1 mM DTT, 100 mM EDTA) followed by centrifugation at 10,000 rpm for 1 min. About 50 ml of supernatant was incubated with the specific substrate (at 37 °C) for 2 h in a water bath [Citation50–52]. The absorbance of the cleaved substrate was measured at 405 nm using a microtiter plate reader.
3. Results and discussions
3.1. Characterization of Reg@GNPs
Cancer research involves many gold nanoparticles (GNPs) because of their amazing biocompatibility, distinctive optical features, surface plasmon resonance (SPR), and simplicity of production [Citation53–55]. Various malignancies have been targeted with specialized GNPs. Photothermal therapy, immunotherapy, photodynamic therapy, gene therapy, targeted therapy, and combinations of these and other therapies are used to diagnose and treat cancer by considering the tumour’s microenvironment and the receptors expressed on its surface.
The coherent electron motion in the GNPs develops two seemingly comparable colloidal dispersion forms of Reg@GNPs with varying size and content of Au and Reg, with Reg@GNPs1 having a violet colour and Reg@GNPs2 a brownish-green colour. UV-Vis absorption spectra investigation showed that produced GNPs exhibit hallmark bands at 537 and 587 nm, indicating surface plasmon resonance (SPR). Their environment’s size, shape, and dielectric characteristics substantially affect their SPR tunability (). Therefore, the two different-sized GNPs with Reg adsorbed on the surfaces of gold NPs, as seen by the red-shifted SPR bands compared to GNPs with or without absorbed molecules.
Figure 1. Characterization of GNPs. (A) UV–Vis spectral analysis of Reg@GNPs nanoconjugates dispersions in methanol.
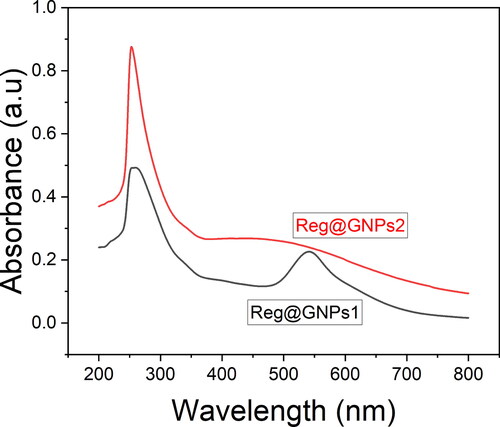
The development of uniformly sized and distributed GNPs, whose shape, size, and dispersion were studied by TEM. As shown in , the size range of capped GNPs is relatively narrow, and the particles have a spherical form. The average particle diameter (dav) of Reg@GNPs1 was 10 ± 2 nm, and that of Reg@GNPs2 was 23 ± 3 nm. The increased second conjugate diameter can be attributed to the influence of more excellent Reg and Au3+ concentrations. In addition, aggregation of the GNPs is unaffected by the adsorption of Reg (), suggesting that the particles have been effectively stabilized. In addition, the SAED diffraction profile of the polycrystalline structure of GNPs assemblies shows the apparent crystallographic planes presented in , indexed as (111), (200), (220), (222), and (311). Inter-planar distances of 2.05, 1.25, and 1.19 were in the HR-TEM images (), and these values correspond to the (002), (113), and (113). DLS technology measures the hydrodynamic size of NPs, which is much greater than TEM’s particle diameter measurement. Reg@GNPs1 was around 65.0 ± 1.7 nm, whereas Reg@GNPs2 was 153.0 ± 2.2 nm. Thus, the latter approach accounts for the particle’s solvent shell (Ethanol) and stabilizer (Reg) in its final particle size measurement. The polydisperse index (PDI) of the NPs are 0.25 and 0.30 for Reg@GNPs1, and Reg@GNPs2, respectively. GNPs treated with Reg in Ethanol had their charge and stability measured similarly. DLS measures the zeta potential of NPs are −35.0 ± 2.5 and −37.0 ± 1.6 for Reg@GNPs1, and Reg@GNPs2, respectively. Reg@GNPs conjugates have been demonstrated to have a distinct set of characteristics compared to the GNPs-GE system, as previously published (dav = 22 nm, hydrodynamic size 65 nm, ZP = 19.34 mV, Reg loading 5%). Our conjugates contain nearly ten times as much Reg as the previously described system, increasing Reg concentrations in the organs of interest. Our conjugates also showed increases in hydrodynamic diameter and ZP (charge).
Figure 2. Morphological characterization of GNPs. A and C) TEM images Reg@GNPs1 and Reg@GNPs2, and subsequent SAED patterns. Reg@GNPs1 (upper row) and Reg@GNPs2 (bottom row).
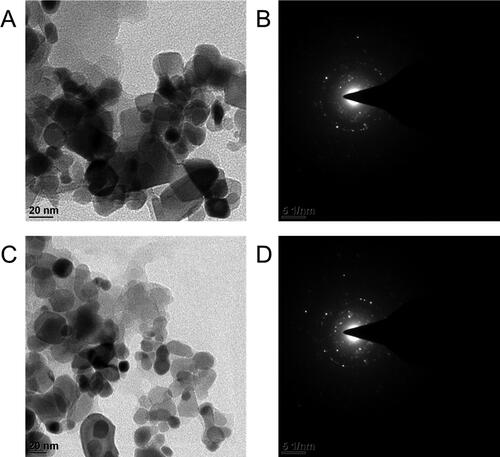
FTIR and PXRD spectroscopy were used to examine the molecular structure of Reg and the nature of its interaction with GNPs. Several distinct peaks may be found in the FTIR spectra of Reg (). Though the distinctive Reg peaks can be seen in the spectra recorded from Reg@GNPs assemblies, both spectra also display considerable variation. These modifications are most pronounced in the spectral shift of the Reg band at 1633 cm−1 v(C = C)A, to higher wavenumbers (1654 cm−1), two weak bands centred at 845 cm−1 (C-H) and 1191 cm−1 (in-plane bend). Broadband at 639 cm−1 (Reg) spectral shifts towards lower frequency (at 630 cm−1 in Reg@GNPs2 and 620 cm−1 in the Reg@GNPs1). Both spectra effects will likely increase in oscillator intensity as Reg accumulates on the GNPs surface. The hydroxyl groups of Reg at the ring presumably interacted with the metal surface, causing the direction of Reg on the GNPs surface, as evidenced by the alterations in the spectra of Reg@GNPs assemblies. Furthermore, the observed modifications suggest that this interaction may modify the spatial organization of Reg close to the metal surface.
Figure 3. (A) FTIR spectral analysis of Reg and Reg@GNPs nanoconjugates. (B) Power X-ray diffraction spectral analysis of Reg and Reg@GNPs nanoconjugates.
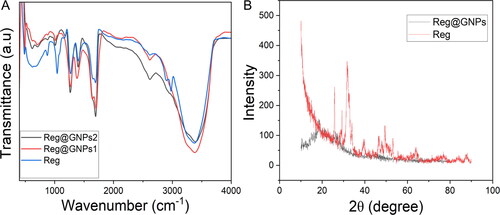
Powder X-Ray diffraction (PXRD) examination was used to verify the crystalline phases of the Reg and Reg@GNPs. The PXRD profile of the Reg@GNPs are shown in . The distinct peaks were reflected at 2θ values of 37.1°, 40.1°, 63.5°, and 78.5° for the Bragg reflection peaks of Reg and Reg@GNPs, which were indexed to the (1 1 1), (2 0 0), (2 2 0), and (3 1 1) planes of the FCC structures, respectively. Likewise, the peaks of Reg@GNPs at 38.5°, 44.7°, 64.8°, and 77.9° correspond to the same planes as that of Reg@GNPs. We compared these diffraction peaks to the standards (JCPDS card no. 04-0783 and JCPDS card no. 04-0784).
3.2. In vitro proliferation study
Three different liver cancer cell lines (HepG2, BEL7402, and Hep3B) were utilized to test the efficacy of Reg and its GNPs conjugates. Although they all originate from metastases, the stages of development and reliance on hormones they display vary widely. HepG2 cells are a model of hormone-dependent cancer because they are differentiated and androgen-dependent. The MTT assay measured cell viability 24 h after exposure to a dosage range of 1 to 50 μg/mL of Reg and Reg@GNPs vitro. shows the outcomes. Most physicochemical characteristics, such as the quantity of bounded Reg, were quite comparable in the Reg@GNPs1 and Reg@GNPs2 systems. The significant distinction between the two was size; Reg@GNPs1 was more negligible in diameter and hydrodynamic diameter than Reg@GNPs2. Reg therapy resulted in a dose-dependent reduction in cell number, as measured by mitochondrial respiration in cell culture. HepG2 had IC50 values of 13.9 ± 0.8, 19.6 ± 0.1, and 29.3 ± 5.1, respectively, for Reg, Reg@GNPs1 and Reg@GNPs2. BEL7402 had IC50 values of 21.0 ± 0.6, 39.6 ± 0.6, and >50 for Reg, Reg@GNPs1 and Reg@GNPs2. Hep3B had IC50 values of 22.3 ± 1.3, 22.6 ± 1.6, and 46.3 ± 1.1 for Reg, Reg@GNPs1 and Reg@GNPs2, respectively. Reg concentrations observed are consistent with those reported in the literature. show that BEL7402 and Hep3B respond less to treatment than HepG2, which is consistent with the determined nature of these two cells. Given that the loading of Reg onto both GNPs was just below 50%, binding to GNPs increased Reg’s cytotoxicity. For HepG2 cells, the activity concentrations of Reg for Reg@GNPs1 (46% of loaded Reg) and Reg@GNPs2 (48% of loaded Reg) were 10 μg/mL and 15 μg/mL, respectively. While this formulation does not significantly boost Reg’s anticancer potential, the improved stability and distribution may be helpful in future in vivo applications.
Figure 4. The effect of Reg and Reg@GNPs nanoconjugates on the cell viability of liver cancer cells (Hep3B, BEL7402, and HepG2). cell viability was verified after 24 h of treatment by MTT test. The data are presented as mean ± SD from the representative of three independent experiments.
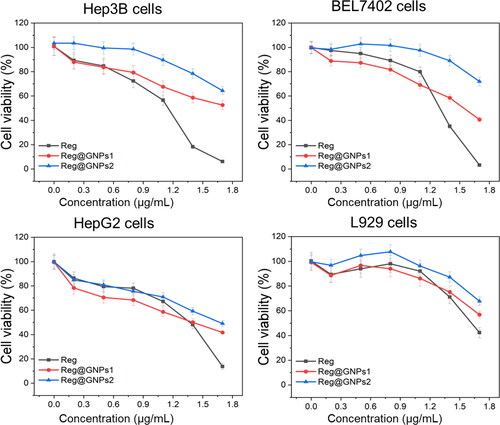
Additionally, our GNPs, like those coated with the flavanone glycoside hesperidin, may stimulate an immunological response in vivo, hence adding to the anti-cancer benefits of Reg. Reg@GNPs’ potential immunomodulatory effects are hinted at by their physicochemical properties, such as a diameter of less than 20 nm. L929 had IC50 values of 40.4 ± 3.2, >50, and >50, respectively, for Reg, Reg@GNPs1 and Reg@GNPs2. Reg@GNPs were selective for the malignant (). In contrast, non-cancerous L929 cells were less susceptible to the treatment with Reg@GNPs compared with free Reg. Consistent with previous studies, we found that Reg@GNPs1 significantly reduced tumour cell viability compared to Reg@GNPs2, suggesting that the larger size of Reg@GNPs1 facilitated more efficient cellular absorption. The enhanced cytotoxicity which may be due to the particle size of the nanoparticles. Therefore, this method of administration was chosen for further mechanistic studies.
3.3. Acridine orange and propidium iodide staining
Differences in cell shape or morphology in monolayer culture are the first noticeable effect of nanoparticles on cell morphology after cells have been exposed to toxic substances. Therefore, we used fluorescence labelling to analyze cellular morphology [Citation56]. The nuclei of the live cells were coloured a vivid green due to the infiltration of AO. Dead cells had PI within, and the nuclei were dyed a brilliant red colour, but PI could not enter the cells when they were alive or still in the early stages of apoptosis. As a result, a healthy cell had a uniformly bright green nucleus and cytoplasm; an early apoptotic cell had intact membranes and a green nucleus; a late apoptotic cell had bright orange areas of condensed chromatin in the nucleus; and a necrotic cell had a uniformly bright orange nucleus (). As a result, AO-PI staining helped distinguish between the various cell types of present.
Figure 5. Examination of cell death by acridine orange and ethidium bromide (AO-EB) staining in Hep3B, BEL7402, and HepG2 cells treated with Reg, Reg@GNPs1 and Reg@GNPs2 with their IC50 concentration for 24-h. Scale bar 50 µm.
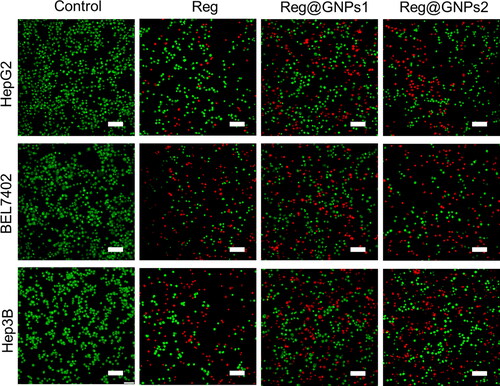
Figure 6. Assessment of nuclear damages by hoechst 33342 staining in Hep3B, BEL7402, and HepG2 cells treated with Reg, Reg@GNPs1 and Reg@GNPs2 with their IC50 concentration for 24-h. Scale bar 50 µm.
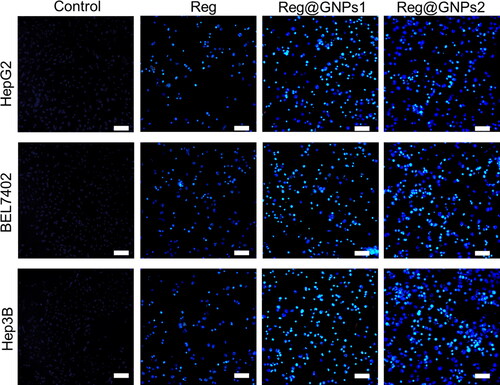
Figure 7. Apoptosis finding by Annexin V-FITC and PI staining in Hep3B, BEL7402, and HepG2 cells treated with Reg, Reg@GNPs1 and Reg@GNPs2 with their IC50 concentration for 24-h by flow cytometry.
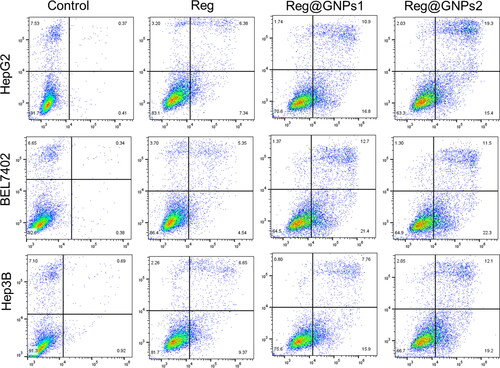
After being stained with AO-PI, HepG2, BEL7402, and Hep3B, liver cancer cells with various morphologies are shown in figure. Many more non-viable cells after Reg, Reg@GNPs1, and Reg@GNPs2-treated cells reached their IC50 concentrations than there were viable cells (), and all the cells shrunk and acquired an amorphous form. The findings showed that the IC50 concentrations of Reg, Reg@GNPs1, and Reg@GNPs2 significantly suppressed cell growth. In other words, cells treated with Reg contained no detectable dead cells. It showed that as compared to Reg@GNPs2, Reg exhibited significantly less cell cytotoxicity. The microscopic examinations showed that cells at IC50 concentrations of Reg@GNPs1 underwent cellular shrinkage and acquired an atypical shape, resulting in aberrant cell size. When the number of viable cells in the Reg@GNPs1 significantly decreased while the number of early-stage, late-stage, and necrotic, apoptotic cells significantly increased.
3.4. DAPI staining
It is crucial to evaluate it to tell apoptosis from necrosis [Citation57]. Nuclear fragmentation is a prominent feature of the apoptotic process that causes cell death. Therefore, apoptosis-related cell death and morphological changes in cells were investigated using the fluorescent DNA-binding agent DAPI. The treated cells shattered apoptotic bodies, and smaller nuclei, as opposed to the healthy and expanded nucleus in the control cells, were evidence of the NPs’ capacity to induce apoptosis (). As shown in DAPI-stained images (), like the untreated cells, the HepG2, BEL7402, and Hep3B liver cancer cells treated with IC50 concentration of Reg, Reg@GNPs1, and Reg@GNPs2 displayed homogeneous fluorescence without visible nuclear-fragmented bodies and segmented nuclei. In contrast, revealing the liver cancer cells to the Reg@GNPs1 and Reg@GNPs2 resulted in nuclear fragmentation in their chromatin. Compared to Reg@GNPs1, the cells planted with Reg@GNPs2 exhibited a more reduced DAPI dye intensity, a higher degree of reduced cell number and nuclear fragmentation, representative of the greater efficacy of Reg@GNPs1 for apoptosis induction in HepG2, BEL7402, and Hep3B liver cancer cells. These outcomes were in various cells with the MTT assay.
3.5. Apoptosis investigation by flow cytometry
Annexin V-FITC-PI, a widely used apoptosis detection assay, was utilized to validate apoptosis induction, and distinguish between viable cells and those in various stages of apoptosis [Citation58]. Reg, Reg@GNPs1, and Reg@GNPs2 were shown to impose the apoptosis cell death manner, as evidenced by the marked shift to early and late apoptotic cell populations. Most anticancer drugs work by promoting cancer cell death via the triggering of apoptotic signalling pathways. Cancer cells were stained with FITC Annexin V and PI, and the statistical evaluation of the early and late apoptosis of cells was verified by fluorescence-activated cell sorting (FACS) via flow cytometry (). This apoptosis and necrosis were performed on HepG2, EL7402, and Hep3B liver cancer cells treated with IC50 concentrations of Reg, Reg@GNPs1, and Reg@GNPs2. After 24 h, the apoptosis assay showed that cancer cells treated with Reg, Reg@GNPs1, and Reg@GNPs2 had a substantial early apoptosis cell population of 12.91% and a late apoptosis cell population of 45.03%, but untreated cells exhibited no significant apoptosis.
3.6. Evaluation of caspase-3/8/9 activation
The apoptosis mode of cell death was investigated by caspase activation by ELISA investigation. In eukaryotes, caspases (protease enzymes) play a crucial role in amplification, transduction, and induction of intracellular apoptosis signals. The amino acid configuration, caspases are split into three sub-families: mediator, executioner, and activator. Suitably, the executioner and initiator caspases perceive within the apoptotic signalling cascade. Caspase-8 and -9 are the initiators of the extrinsic and intrinsic cell death pathways, respectively. Both mechanisms converge in the activation of the executioner caspase-3. Hence, the IC50 concentration of Reg, Reg@GNPs1 and Reg@GNPs2 (IC50 concentration) at 24-h were treated on HepG2, EL7402, and Hep3B cells with caspases-3, -8 and -9. The outcomes confirmed that Reg@GNPs1 and Reg@GNPs2 efficiently upregulated the expression caspase-8 among other caspases-3 and -9 for triggering cancer cell apoptosis. The results also showed a Reg@GNPs1 and Reg@GNPs2 increase in caspase activity than control cells ().
4. Conclusion
We designed a new gold nanoparticle conjugate, Reg@GNPs1 and Reg@GNPs2, but with somewhat different sizes. Biocompatible conjugates were prepared by the facile chemistry method, with Reg serving a dual role to decrease Au3+ and stabilize the resulting GNPs. Reg@GNPs increased the therapeutic efficacy of Reg and indicated nanoparticle safety in perspective biological applications by demonstrating cytotoxicity in liver cancer cells while retaining minimal cytotoxicity. The cytotoxic potential of nanoparticles was affected by their size, with the smaller Reg@GNPs1 conjugation displaying anti-liver tumour effects exhibited by antioxidative and antiproliferative activity. This study’s findings provide promising evidence that engineered nanoparticles can be used to treat liver cancer. This novel potential drug delivery method will be shown to be effective and safe for multifunctional chemotherapeutic use, including various cancers, in future in vivo research.
Consent for publication
Not applicable.
Ethics approval and consent to participate
Not applicable.
Availability of data and materials
The datasets used and analyzed during the current study are available from the corresponding author upon request.
Disclosure statement
No potential conflict of interest was reported by the authors.
Additional information
Funding
Notes on contributors
Meng Yue
Meng Yue is from Jinan city, Shandong, China. She graduated from Shandong University School of Medicine with a master’s degree and now engaged in gastroenterology.
Rui Yang
Rui Yang, from Wudi city, Shandong, China. Graduated from Shandong University with a master’s degree, majoring in stomatology.
Yakun Jiang
Yakun Jiang, from Jinan city, Shandong, China.graduated from Shandong University School of Medicine with a master’s degree and now engaged in gastroenterology.
Xiuhua Yang
Xiuhua Yang, from Jinan city, Shandong, China. Graduated from the School of Nursing of Shandong University.
References
- Balogh J, Victor D, III, Asham EH, et al. Hepatocellular carcinoma: a review. J Hepatocell Carcinoma. 2016;3:1–16. doi: 10.2147/JHC.S61146.
- Befeler AS, Di Bisceglie AM. Hepatocellular carcinoma: diagnosis and treatment. Gastroenterology. 2002;122(6):1609–1619. doi: 10.1053/gast.2002.33411.
- Bruix J, Gores GJ, Mazzaferro V. Hepatocellular carcinoma: clinical frontiers and perspectives. Gut. 2014;63(5):844–855. doi: 10.1136/gutjnl-2013-306627.
- Yang JD, Roberts LR. Hepatocellular carcinoma: a global view. Nat Rev Gastroenterol Hepatol. 2010;7(8):448–458. doi: 10.1038/nrgastro.2010.100.
- Motola-Kuba D, Zamora-Valdés D, Uribe M, et al. Hepatocellular carcinoma. An overview. Ann Hepatol. 2006;5(1):16–24. doi: 10.1016/S1665-2681(19)32034-4.
- Llovet JM, Bruix J. Novel advancements in the management of hepatocellular carcinoma in 2008. J Hepatol. 2008;48 Suppl 1: s20–S37. doi: 10.1016/j.jhep.2008.01.022.
- Thorgeirsson SS, Grisham JW. Molecular pathogenesis of human hepatocellular carcinoma. Nat Genet. 2002;31(4):339–346. doi: 10.1038/ng0802-339.
- Pandey V, Haider T, Chandak AR, et al. Surface modified silk fibroin nanoparticles for improved delivery of doxorubicin: development, characterization, in-vitro studies. Int J Biol Macromol. 2020;164:2018–2027. doi: 10.1016/j.ijbiomac.2020.07.326.
- Wang Q, Li WL, Zou HY, et al. Nonstoichiometric Cu2 − xSe nanocrystals in situ produced on the surface of carbon nanotubes for ablation of tumor cells. New J. Chem. 2016;40(7):6315–6324. doi: 10.1039/C5NJ03379A.
- Liu Y, Chen Z, Liu C, et al. Gadolinium-loaded polymeric nanoparticles modified with anti-VEGF as multifunctional MRI contrast agents for the diagnosis of liver cancer. Biomaterials. 2011;32(22):5167–5176. doi: 10.1016/j.biomaterials.2011.03.077.
- Shi S, Chen X, Wei J, et al. Platinum(IV) prodrug conjugated Pd@Au nanoplates for chemotherapy and photothermal therapy. Nanoscale. 2016;8(10):5706–5713. doi: 10.1039/c5nr09120a.
- Tambe P, Kumar P, Paknikar KM, et al. Decapeptide functionalized targeted mesoporous silica nanoparticles with doxorubicin exhibit enhanced apoptotic effect in breast and prostate cancer cells. Int J Nanomedicine. 2018;13:7669–7680. doi: 10.2147/IJN.S184634.
- Paroha S, Verma J, Dubey RD, et al. Recent advances and prospects in gemcitabine drug delivery systems. Int J Pharm. 2021;592:120043. doi: 10.1016/j.ijpharm.2020.120043.
- Rana A, Yadav K, Jagadevan S. A comprehensive review on green synthesis of nature-inspired metal nanoparticles: mechanism, application and toxicity. J Cleaner Prod. 2020;272:122880. doi: 10.1016/j.jclepro.2020.122880.
- Balachandran S. Magnetic nanoparticles for cancer treatment. In: Magnetic nanoparticles. Singapore: Springer, 2021: pp. 133–147.
- Caizer IS, Caizer C. Superparamagnetic hyperthermia study with cobalt ferrite nanoparticles covered with γ-Cyclodextrins by computer simulation for application in alternative cancer therapy. Int J Mol Sci. 2022;23(8):4350. doi: 10.3390/ijms23084350.
- Chung CYS, Fung SK, Tong KC, et al. A multi-functional PEGylated gold(iii) compound: potent anti-cancer properties and self-assembly into nanostructures for drug co-delivery. Chem Sci. 2017;8(3):1942–1953. doi: 10.1039/c6sc03210a.
- Kim J, Pramanick S, Lee D, et al. Polymeric biomaterials for the delivery of platinum-based anticancer drugs. Biomater Sci. 2015;3(7):1002–1017. doi: 10.1039/c5bm00039d.
- Han W, Shi L, Ren L, et al. A nanomedicine approach enables co-delivery of cyclosporin a and gefitinib to potentiate the therapeutic efficacy in drug-resistant lung cancer. Signal Transduct Target Ther. 2018;3(1):16. doi: 10.1038/s41392-018-0019-4.
- Parker JP, Ude Z, Marmion CJ. Exploiting developments in nanotechnology for the preferential delivery of platinum-based anti-cancer agents to tumours: targeting some of the hallmarks of cancer. Metallomics. 2016;8(1):43–60. doi: 10.1039/c5mt00181a.
- Shen J, He Q, Gao Y, et al. Mesoporous silica nanoparticles loading doxorubicin reverse multidrug resistance: performance and mechanism. Nanoscale. 2011;3(10):4314–4322. doi: 10.1039/c1nr10580a.
- Delplace V, Couvreur P, Nicolas J. Recent trends in the design of anticancer polymer prodrug nanocarriers. Polym Chem. 2014;5(5):1529–1544. doi: 10.1039/C3PY01384G.
- Xu X, Yu Y, Liu M, et al. Efficacy and safety of regorafenib and fruquintinib as third-line treatment for colorectal cancer: a narrative review. Transl Cancer Res. 2022;11(1):276–287. doi: 10.21037/tcr-20-3539.
- Pazoki-Toroudi HR, Hesami A, Vahidi S, et al. The preventive effect of captopril or enalapril on reperfusion injury of the kidney of rats is independent of angiotensin II AT1 receptors. Fundam Clin Pharmacol. 2003;17(5):595–598. doi: 10.1046/j.1472-8206.2003.00188.x.
- Zhao P, Wang Y, Kang X, et al. Dual-targeting biomimetic delivery for anti-glioma activity via remodeling the tumor microenvironment and directing macrophage-mediated immunotherapy. Chem Sci. 2018;9(10):2674–2689. doi: 10.1039/C7SC04853J.
- Sravani B, Kiranmai S, Rajasekhara Reddy G, et al. Highly sensitive detection of anti-cancer drug based on bimetallic reduced graphene oxide nanocomposite. Chemosphere. 2022;287(Pt 3):132281. doi: 10.1016/j.chemosphere.2021.132281.
- Wang R, Liu Y, Mi X, et al. Sirt3 promotes hepatocellular carcinoma cells sensitivity to regorafenib through the acceleration of mitochondrial dysfunction. Arch Biochem Biophys. 2020;689:108415. doi: 10.1016/j.abb.2020.108415.
- Ervin SM, Hanley RP, Lim L, et al. Targeting Regorafenib-Induced toxicity through inhibition of gut microbial β-Glucuronidases. ACS Chem Biol. 2019;14(12):2737–2744. doi: 10.1021/acschembio.9b00663.
- Zhou Z. Co-drug delivery of regorafenib and cisplatin with amphiphilic copolymer nanoparticles: enhanced in vivo antitumor cancer therapy in nursing care. Drug Deliv. 2020;27(1):1319–1328. doi: 10.1080/10717544.2020.1815897.
- Khan K, Cascinu S, Cunningham D, et al. Imaging and clinical correlates with regorafenib in metastatic colorectal cancer. Cancer Treat Rev. 2020;86:102020. doi: 10.1016/j.ctrv.2020.102020.
- Vinita NR, Nirala RP. One step synthesis of AuNPs@MoS2-QDs composite as a robust peroxidase-mimetic for instant unaided eye detection of glucose in serum, saliva and tear. Sensor Actuat B: Chem. 2018;263:109–119. doi: 10.1016/j.snb.2018.02.085.
- Zhu X, Han W, Liu Y, et al. Rational design of a prodrug to inhibit self-inflammation for cancer treatment. Nanoscale. 2021;13(11):5817–5825. doi: 10.1039/D1NR00132A.
- Maqsood H, Uroos M, Muazzam R, et al. Extraction of basil seed mucilage using ionic liquid and preparation of AuNps/mucilage nanocomposite for catalytic degradation of dye. Int J Biol Macromol. 2020;164:1847–1857. doi: 10.1016/j.ijbiomac.2020.08.073.
- Mohan N, Mohamed Subarkhan MK, Ramesh R. Synthesis, antiproliferative activity and apoptosis-promoting effects of arene ruthenium(II) complexes with N, O chelating ligands. J Organomet Chem. 2018;859:124–131. doi: 10.1016/j.jorganchem.2018.01.022.
- Mohamed Kasim MS, Sundar S, Rengan R. Synthesis and structure of new binuclear ruthenium(II) arene benzil bis(benzoylhydrazone) complexes: investigation on antiproliferative activity and apoptosis induction. Inorg. Chem. Front. 2018;5(3):585–596. doi: 10.1039/C7QI00761B.
- Subarkhan MKM, Ramesh R. Ruthenium(II) arene complexes containing benzhydrazone ligands: synthesis, structure and antiproliferative activity. Inorg. Chem. Front. 2016;3(10):1245–1255. doi: 10.1039/C6QI00197A.
- Raj Kumar R, Mohamed Subarkhan MK, Ramesh R. Synthesis and structure of nickel(ii) thiocarboxamide complexes: effect of ligand substitutions on DNA/protein binding, antioxidant and cytotoxicity. RSC Adv. 2015;5(58):46760–46773. doi: 10.1039/C5RA06112A.
- Giriraj K, Mohamed Kasim MS, Balasubramaniam K, et al. Various coordination modes of new coumarin schiff bases toward cobalt(III) ion: synthesis, spectral characterization, in vitro cytotoxic activity, and investigation of apoptosis. Appl Organom Chemis. 2022;36(3):e6536. doi: 10.1002/aoc.6536.
- Sathiya Kamatchi T, Mohamed Subarkhan MK, Ramesh R, et al. Investigation into antiproliferative activity and apoptosis mechanism of new arene Ru(ii) carbazole-based hydrazone complexes. Dalton Trans. 2020;49(32):11385–11395. doi: 10.1039/D0DT01476A.
- Mohamed Subarkhan MK, Ren L, Xie B, et al. Novel tetranuclear ruthenium(II) arene complexes showing potent cytotoxic and antimetastatic activity as well as low toxicity in vivo. Eur J Med Chem. 2019;179:246–256. doi: 10.1016/j.ejmech.2019.06.061.
- Swaminathan S, Haribabu J, Mohamed Subarkhan MK, et al. Impact of aliphatic acyl and aromatic thioamide substituents on the anticancer activity of Ru(ii)-p-cymene complexes with acylthiourea ligands—in vitro and in vivo studies. Dalton Trans. 2021;50(44):16311–16325. doi: 10.1039/D1DT02611A.
- Swaminathan S, Haribabu J, Mohamed Subarkhan MK, et al. Coordination behavior of acylthiourea ligands in their Ru(II)–benzene complexes—structures and anticancer activity. Organometallics. 2022;41(13):1621–1630. doi: 10.1021/acs.organomet.2c00127.
- Pilliadugula R, Haribabu J, Mohamed Subarkhan MK, et al. Effect of morphology and (Sn, Cr) doping on in vitro antiproliferation properties of hydrothermally synthesized 1D GaOOH nanostructures. J Sci: Adv Mater Devices. 2021;6(3):351–363. doi: 10.1016/j.jsamd.2021.03.003.
- Kalaiarasi G, Mohamed Subarkhan M, Fathima Safwana CK, et al. New organoruthenium(II) complexes containing N, X-donor (X = O, S) heterocyclic chelators: synthesis, spectral characterization, in vitro cytotoxicity and apoptosis investigation. Inorg Chim Acta. 2022;535:120863. doi: 10.1016/j.ica.2022.120863.
- Mohamed Subarkhan MK, Ramesh R, Liu Y. Synthesis and molecular structure of arene ruthenium(II) benzhydrazone complexes: impact of substitution at the chelating ligand and arene moiety on antiproliferative activity. New J. Chem. 2016;40(11):9813–9823. doi: 10.1039/C6NJ01936F.
- Balaji S, Mohamed Subarkhan MK, Ramesh R, et al. Synthesis and structure of arene Ru(II) N∧O-Chelating complexes: in vitro cytotoxicity and cancer cell death mechanism. Organometallics. 2020;39(8):1366–1375. doi: 10.1021/acs.organomet.0c00092.
- Gao X, Zheng Y, Ruan X, et al. Salinomycin induces primary chicken cardiomyocytes death via mitochondria mediated apoptosis. Chem Biol Interact. 2018;282:45–54. doi: 10.1016/j.cbi.2018.01.009.
- Edlich A, Volz P, Brodwolf R, et al. Crosstalk between core-multishell nanocarriers for cutaneous drug delivery and antigen-presenting cells of the skin. Biomaterials. 2018;162:60–70. doi: 10.1016/j.biomaterials.2018.01.058.
- Wang X-P, Wang Q-X, Lin H-P, et al. Anti-tumor bioactivities of curcumin on mice loaded with gastric carcinoma. Food Funct. 2017;8(9):3319–3326. doi: 10.1039/C7FO00555E.
- Ke Y, Al Aboody MS, Alturaiki W, et al. Photosynthesized gold nanoparticles from catharanthus roseus induces caspase-mediated apoptosis in cervical cancer cells (HeLa. Artif Cells Nanomed Biotechnol. 2019;47(1):1938–1946.) doi: 10.1080/21691401.2019.1614017.
- Li Y, Yu H, Zhao L, et al. Effects of carbon nanotube-mediated Caspase3 gene silencing on cardiomyocyte apoptosis and cardiac function during early acute myocardial infarction. Nanoscale. 2020;12(42):21599–21604. doi: 10.1039/D0NR05032F.
- Zeng C, Guo B, Chen J, et al. Antitumor effects of chrysophanol in malignant optic nerve meningioma cell lines are mediated via caspase activation, induction of mitochondrial mediated apoptosis, mitochondrial membrane depolarization and targeting the mitogen-activated protein kinase S. Pharmacology. 2019;104(1–2):28–35. doi: 10.1159/000499336.
- Dheyab MA, Aziz AA, Khaniabadi PM, et al. Gold nanoparticles-based photothermal therapy for breast cancer. Photodiagnosis Photodyn Ther. 2023;42:103312. doi: 10.1016/j.pdpdt.2023.103312.
- Hu X, Li X, Zhang Y, et al. Multi-wavelength output based on gold nanoparticles in erbium-doped fiber lasers. Appl. Opt. 2020;59(10):3196–3202. doi: 10.1364/AO.388605.
- Cho J-H, Kim A-R, Kim S-H, et al. Development of a novel imaging agent using peptide-coated gold nanoparticles toward brain glioma stem cell marker CD133. Acta Biomater. 2017;47:182–192. doi: 10.1016/j.actbio.2016.10.009.
- Pang X-L, He G, Liu Y-B, et al. Endoplasmic reticulum stress sensitizes human esophageal cancer cell to radiation. World J Gastroenterol. 2013;19(11):1736–1748. doi: 10.3748/wjg.v19.i11.1736.
- Amini N, Abdul Majid FA, Marvibaigi M, et al. CervicareTM induces apoptosis in HeLa and CaSki cells through ROS production and loss of mitochondrial membrane potential. RSC Adv. 2016;6(29):24391–24417. doi: 10.1039/C5RA25654B.
- Li X, Gao Y. Synergistically fabricated polymeric nanoparticles featuring dual drug delivery system to enhance the nursing care of cervical cancer. Process Biochem. 2020;98:254–261. doi: 10.1016/j.procbio.2020.09.010.