1. Introduction
Radiation exposures from accidents or other sources of radiation can result in different types of radiation injuries, dictating the diagnostic and therapeutic measures. The clinical progression of acute radiation syndrome (ARS) depends on the tissue-absorbed radiation dose. Multi-organ involvement and organ system failure need to be taken into consideration [Citation1,Citation2]. The extent of radiation-induced injury to lymphohematopoietic tissues, gastrointestinal (GI) and neurovascular systems, lung, and skin plays a vital role in the diagnosis, treatment and the prognosis of victims exposed to radiation. Surviving acute high dose ionizing radiation exposure of the whole-body carries significant health risks that often have disastrous outcomes. These pathological syndromes are delayed in expression and associated with pathologies within multiple organ systems of the body. When fully expressed, these delayed effects’ of acute radiation exposure, often known with the acronym as ‘DEARE’ and ultimately results in multiple organ system failure and death.
Medical countermeasures (MCMs) for radiation are divided into three distinct classes. Radioprotectors are prophylactic agents that are administered prior to radiation exposure to prevent radiation-induced cellular and molecular injury [Citation3]. Radiomitigators are MCMs administered soon after exposure that accelerate recovery from radiation injury. Radiation therapeutics or treatments are MCMs given after symptoms manifest to promote repair or regeneration. All three Food and Drug Administration (FDA)-approved MCMs for hematopoietic ARS (H-ARS) are radiomitigators [Citation4]. Several candidate MCMs have been identified and are currently being developed for FDA approval. There are several animal models to study the ARS and DEARE and the development of MCMs for these sub-syndromes [Citation5,Citation6].
The Strategic National Stockpile (SNS)/Vendor-Managed Inventory (VMI) is a federally owned and managed national repository of antibiotics, antivirals, life support pharmaceuticals, chemical antidotes, antitoxins, vaccines, intravenous (iv) administration supplies, airway maintenance supplies, masks, pandemic countermeasures, and medical/surgical items to supplement and re-supply state and local agencies in the event of an incident following either the use of weapons of mass destruction or a major natural or technological disaster [Citation7]. The mission of the SNS is to provide above items in a rapid and safe manner [Citation8]. The SNS program has 12-Hour Push Packs delivered anywhere in the United States or its territories within 12 h of a federal decision to deploy.
2. Animal models for ARS
No single animal model can appropriately portray human conditions for all parameters. Therefore, it is important to be familiar with the various animal models available to select the most appropriate model for the individual study’s objectives. As stated above, nonhuman primates (NHPs) are the species closest to humans in respect of genetic homology and pathophysiology. The lack of suitable substitutes for the NHP model(s) for predicting the response in humans serves as a bottleneck for the development of radiation MCM and understanding the mechanism of radiation injury. Canines are easy to handle and have a comprehensive background database for acute radiation effects, toxicology, and safety evaluation. However, canines have considerable ethical issues. Canines lack the organic anion transporter, OAT3, and requires a higher dose of some agents. Even though minipigs have been used considerably less frequently than either the canine or the NHP models in pharmaceutical research, the Göttingen minipig has been proposed as a model for the H- and gastrointestinal ARS (GI-ARS) [Citation9]. It has a well-characterized genotype and gene sequence homology with humans [Citation10]. The body thickness of a minipig is comparable to a young human, resulting in radiation absorption patterns that are quite similar. In studies of cutaneous component, the minipig may mimic the human response to irradiation more closely than any other animal. However, the larger size and body weight relative to the NHP, need for vascular access port (VAP), and lack of an integrated dose- and time-dependent response relationship for the ARS have marginalized the minipig relative to MCM efficacy testing. It has a relatively steep dose response relationship and markedly reduced LD50/60 value for the H-ARS relative to the canine, NHP, and human [Citation9]. Additionally, the acute GI-ARS is not well-studied relative to its dose response and time course post-exposure. In studies involving a cutaneous component, the minipig may represent the human response more closely than any other animal model [Citation11]. If an agent is metabolized by aldehyde oxidase, N-acetyltransferase, or certain cytochrome P450 enzymes which are not expressed in canine, the minipig may be the appropriate model. On the other hand, minipigs may not be the optimal choice, if sulphation is an important pathway in the human metabolism of the candidate drug. Some groups are developing the rabbit as another animal model of whole-body irradiation [Citation12]. Though, adult animals of various species have been used for the study of radiation injury, as well as for testing efficacy of MCMs against radiation-induced injuries, recently, Göttingen minipig pediatric model has been developed to investigate radiation injury and evaluation of MCMs for ARS [Citation13].
3. Development of radiation countermeasures for ARS following FDA animal rule
In 2002, the U.S. FDA issued the ‘Animal Rule’ intended to expedite the development of new drugs and biologics that can act as MCM against chemical, biological, radiological, and nuclear (CBRN) threats. The rule applies exclusively to new agents for which definitive human efficacy studies cannot be conducted [Citation14]. The U.S. FDA can grant marketing approval to new products that have been demonstrated in well-controlled animal studies to be safe and capable of producing clinical benefit in humans. The criteria of the FDA’s Animal Rule relevant to development using animal models are as follows: (a) There should be a reasonably well-understood pathophysiological mechanism for toxicity of the agent (radiation), and its prevention, or substantial reduction by the drug; (b) The effect of the drug or biologic is demonstrated in more than one animal species, for which the expected effect is predictive for humans. The multiple animal requirement can be abrogated if the effect can be demonstrated in a single well-characterized animal model which sufficiently predicts the response in humans; (c) The animal study’s end point is related to a desired benefit in humans, generally involving the enhancement of survival or prevention of major morbidity; (d) Pharmacokinetics and pharmacodynamics, or other relevant data or information of the product, in animals and humans, allows selection of an effective human dose.
The Animal Rule does not entirely eliminate the testing of the drug in human clinical trials (). A clinical phase I study is still required to evaluate the safety of the countermeasure and to help determine the optimal dose of the drug for efficacy in humans. Substituting animals for humans in efficacy tests was not intended to make it easier to obtain FDA approval for novel MCM. In fact, more information is required about the animal model itself, the mechanism and course of disease, and the mechanism of action of the MCM than when efficacy studies can be performed in humans. Without convincing human efficacy data, it is more difficult to understand how an MCM works, why it works, and to generate data providing confidence that the MCM will be effective in humans. Biomarkers for radiation injury and MCM efficacy are particularly important [Citation15,Citation16]. Such biomarkers may serve as a predictive indicator for the MCM efficacy in humans and also for MCM dose conversion from animals to human, beyond allometric conversion.
Figure 1. Steps involved in countermeasure development following FDA animal rule. Such countermeasures under development may receive pre-emergency use authorization (pre-EUA) prior to full approval for human use by FDA. BLA, biologics license applications; IND, investigational new drug; NDA, new drug application.
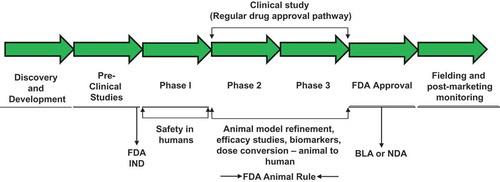
Animal-efficacy data may not be as convincing as the human data used for other drugs. Thus, a different mind-set and strategy are required for the approval of MCM that will be needed during a CBRN defense emergency. The FDA also requires post-marketing human-efficacy studies if the MCM is used in humans, as a response to a radiation event, a process that would be extremely difficult while in the midst of a national public health crisis.
4. Radiation countermeasures approved by the FDA for ARS following the animal rule
To date, only three radiation countermeasures for ARS, Neupogen, Neulasta, and Leukine, have received U.S. FDA approval for human use and are stocked in the SNS () [Citation17–Citation22]. Thus far, no radioprotector has received FDA approval for ARS, H-ARS, or GI-ARS. Further, all three FDA-approved radiomitigators for H-ARS were in clinical use for decades before they were repurposed for an additional indication. The FDA dual-use approval for such MCM was comparatively easy since these agents were already in clinical use for other indications of chemotherapy-induced myelosuppression. For such agents, one needed to demonstrate efficacy against additional indications since their safety and toxicity pose no threat. There are several reasons for failure for radioprotector development. It is important to note that radioprotectors have more risk and require additional scrutiny since these agents will be administered to healthy individuals in anticipation of radiation exposure, unlike radiomitigators, which are administered to the victims post-exposure to radiation.
Table 1. U.S. FDA-approved biologics for H-ARS following animal rule and available in SNS/VMI.
In addition to the abovementioned FDA-approved agents for H-ARS, there are several potential MCM listed in which have received FDA investigational new drug (IND) status and the vast majority of them are being investigated in healthy volunteers for safety and in large animal models for efficacy [Citation4,Citation23].
Table 2. Radiation countermeasures under development following the FDA animal rule criteria for ARS with U.S. FDA IND status.
5. Conclusion
MCMs for ARS are being developed following the U.S. FDA Animal Rule since efficacy evaluation for CBRN MCMs is not possible in human volunteers since it would be unethical to knowingly expose humans to lethal doses of radiation for which field trials have not been feasible. For studying injuries caused by acute lethal doses of radiation, only two large animal models, NHP and canine, have been well characterized. For the last several years, investigators at various institutions are working to develop and characterize the minipig model, particularly Göttingen and Sinclaira, for radiation injury and MCM development. The availability of suitable experimental animal models is one of the limiting factors for developing radiation MCMs following the FDA Animal Rule. MCMs are usually tested in rodents using 30-d survival as the primary end point and in a 60-d survival end point in NHP or canine. These time intervals have been selected to reflect the ability of the agents to provide protection against or mitigate ARS following total-body or partial-body irradiation. Delayed or late effects of whole-body or partial-body exposures are gaining traction in the field of radiation MCM investigation.
As stated above, only three radiation MCMs (Neupogen®, Neulasta®, Leukine®) have received U.S. FDA approval following the Animal Rule for H-ARS and all three are radiomitigators that have been extensively studied for efficacy and safety using several small and large animal models. To date, no MCM has been approved by the FDA which can be used as a radioprotector. Further, no MCM has been approved for GI-ARS, either protector or mitigator. In brief, there are significant opportunities to develop radiation MCMs using various animal models under development but there are also challenges due to lack of multiple animal models for each sub-syndromes of ARS or DEARE to satisfy FDA for the approval of MCMs under Animal Rule.
6. Expert opinion
There are several radiosensitive organ systems of the body in addition to the hematopoietic and GI systems, including the vascular, the pulmonary, kidney, and cutaneous systems. All these organ systems are in need of thorough investigation in terms of developing safe and effective radiation MCMs. In addition, the efficacy of MCMs in the setting of exposure to particular type of radiation (specifically high LET (linear energy transfer) irradiation), such as protons, neutrons, and heavier ions, is another interesting and unexplored area relative to space exploration. The majority of studies in the past utilized low LET radiation such as γ-radiation source or X-rays.
There are several reasons for the lack of FDA approval of MCM for various sub-syndromes of ARS, and DEARE (lung fibrosis, cardiovascular disease and multiple organ injury). One important challenge is the lack of additional, reliable, well-characterized, and validated large animal models suitable to represent the pathophysiology of humans for studying radiation injury. The advanced development and FDA approval of radiation MCM for ARS requires demonstration that the mechanism of action of such MCM in animal models is predictive of the response in humans. The FDA Animal Rule emphasizes the need for a great understanding of pathophysiology of radiation injury and mechanism of action of radiation MCM under development. For many types of radiation injury (specifically not for H-ARS), the mechanism of the radiation injury itself is subject to considerable debate and this makes the mechanism of action requirement by FDA quite difficult to meet. Though the Animal Rule permits MCM approval based on a single well-characterized animal model, the usual expectation is that efficacy will be demonstrated in studies in more than one species. Specific nonclinical laboratory studies projected to support approval under the Animal Rule must be carried out under the good laboratory practice (GLP) setting. In addition, it also requires the identification and validation of biomarkers to serve as a predictive marker for the efficacy in humans and for drug dose conversion from animal to human [Citation24]. The challenges of fulfilling the Animal Rule requirements, compared with the traditional drug licensure, may be one reason that it has been used to the limited extent to approve new MCMs.
Overcoming these hurdles to develop countermeasures for ARS for FDA approval following the Animal Rule is difficult. Due to these reasons, some MCM become stagnant during their development (e.g. 5-androstenediol). Early discussions with the FDA are of paramount importance to identify the appropriate and validated animal models, and study endpoints that would yield acceptable and convincing data to the FDA. Prior to conducting Phase I clinical trials of new therapeutic agents for safety and toxicity, an application for an Investigational New Drug (IND) must be submitted to FDA.
For drug repurposing under the FDA Animal Rule, there is a critical need for intellectual property rights protection. There are several off-patent FDA-approved pharmaceuticals for other indications that look promising as MCMs (specifically DEARE), but the lack of intellectual property rights protection means that companies have no financial incentive to seek a label for ARS indication. Another impediment to ARS MCM development is that the FDA Animal Rule requires enhancement of survival in irradiated animals or prevention of morbidity which often conflicts with institutional animal care and use committee (IACUC) requirements to euthanize the animals before such endpoints are attained. In a clash between FDA Animal Rule requirements and IACUC rules, the IACUC cannot be overruled. As a result of such impediments, corporate partners sometimes lose interest to develop such MCMs. The critical path to MCM development requires early interaction with the FDA and clear dual paths for development of the proposed MCM for efficacy against clinical disease and radiological/nuclear threats. Well-planned experimental design and use of valid animal models in concert with FDA consideration is the most appropriate course to assess efficacy under the Animal Rule. In brief, additional research effort is needed to further develop large animal models for improving the prediction of clinical safety and effectiveness of radiation countermeasures for ARS and DEARE in humans.
Declaration of interest
The authors have no relevant affiliations or financial involvement with any organization or entity with a financial interests or conflicts with the subject matter or materials discussed in the manuscript. This includes employment, consultancies, honoraria, stock ownership or options, expert testimony, grants or patents received or pending, or royalties.
Reviewer disclosures
Peer reviewers on this manuscript have no relevant financial or other relationships to disclose
Acknowledgments
The opinions or assertions contained herein are the professional views of the authors and do not necessarily represent the Armed Forces Radiobiology Research Institute, the Uniformed Services University of the Health Sciences, or the Department of Defense, U.S.A. Mention of specific therapeutic agents does not constitute endorsement by the U.S. Department of Defense, and trade names are used only for the purpose of clarification.
Additional information
Funding
References
- Dorr H, Meineke V. Acute radiation syndrome caused by accidental radiation exposure – therapeutic principles. BMC Med. 2011;9:126.
- Fliedner TM, Dorr DH, Meineke V. Multi-organ involvement as a pathogenetic principle of the radiation syndromes: a study involving 110 case histories documented in SEARCH and classified as the bases of haematopoietic indicators of effect. Br J Radiol Suppl. 2005;27:1–8.
- Stone HB, Moulder JE, Coleman CN, et al. Models for evaluating agents intended for the prophylaxis, mitigation and treatment of radiation injuries. Report of an NCI Workshop, December 3–4, 2003. Radiat Res. 2004;162:711–728.
- Singh VK, Seed TM. A review of radiation countermeasures focusing on injury-specific medicinals and regulatory approval status: part I. Radiation sub-syndromes, animal models and FDA-approved countermeasures. Int J Radiat Biol. 2017;93:851–869.
- Williams JP, Brown SL, Georges GE, et al. Animal models for medical countermeasures to radiation exposure. Radiat Res. 2010;173:557–578.
- Williams JP, Jackson IL, Shah JR, et al. Animal models and medical countermeasures development for radiation-induced lung damage: report from an NIAID Workshop. Radiat Res 2012;177:e0025–39
- Centers for Disease Control and Prevention. Strategic National Stockpile (SNS). 2014. Available at: http://www.cdc.gov/phpr/stockpile/stockpile.htm [Last accessed Feb 15, 2014]
- Esbitt D. The Strategic National Stockpile: roles and responsibilities of health care professionals for receiving the stockpile assets. Disaster Manag Response. 2003;1:68–70.
- Moroni M, Coolbaugh TV, Lombardini E, et al. Hematopoietic radiation syndrome in the Gottingen minipig. Radiat Res. 2011;176:89–101.
- Dalgaard L. Comparison of minipig, dog, monkey and human drug metabolism and disposition. J Pharmacol Toxicol Methods. 2015;74:80–92.
- Riccobono D, Agay D, Scherthan H, et al. Application of adipocyte-derived stem cells in treatment of cutaneous radiation syndrome. Health Phys. 2012;103:120–126.
- Gratwohl A, John L, Baldomero H, et al. FLT-3 ligand provides hematopoietic protection from total body irradiation in rabbits. Blood. 1998;92:765–769.
- Kaur A, Vemalapally N, Severson G, et al. Development of pediatric model of hemtopoietic acute radiation syndrome (H-ARS) and countermeasure testing using the G0ttingen minipig. Rad J. 2017;2:75–81.
- U.S. Food and Drug Administration. Guidance for industry: product developoment under the Animal Rule. 2015. Available at: http://www.fda.gov/downloads/Drugs/GuidanceComplianceRegulatoryInformation/Guidances/UCM399217.pdf [Last accessed 2016 Jul 5]
- Valente M, Denis J, Grenier N, et al. Revisiting biomarkers of total-body and partial-body exposure in a baboon model of irradiation. PLoS One. 2015;10:e0132194.
- Kraus VB. Biomarkers as drug development tools: discovery, validation, qualification and use. Nat Rev Rheumatol. 2018. in press. DOI:10.1038/s41584-018-0005-9
- U.S. Food and drug Administration. FDA approves Leukine for acute radiation syndrome. 2018. Available at: https://www.fda.gov/downloads/EmergencyPreparedness/Counterterrorism/MedicalCountermeasures/AboutMCMi/UCM603226.pdf [Last accessed 2018 Apr 01]
- Farese AM, Cohen MV, Katz BP, et al. Filgrastim improves survival in lethally irradiated nonhuman primates. Radiat Res. 2013;179:89–100.
- U.S. Food and Drug Administration. FDA approves Neupogen for treatment of patients with radiation-induced myelosuppression following a radiological/nuclear incident. 2015. Available at: http://www.fda.gov/EmergencyPreparedness/Counterterrorism/MedicalCountermeasures/AboutMCMi/ucm443245.htm [Last accessed 2016 Jul 6]
- Clayton NP, Charpentier EJJ, LaCasse ER, et al. Sargramostim significantly improved the mortality rate at Day 60 in a non-human primate model of hematopoietic acute radiation syndrome with minimal supportive care when administered 48 h after total body irradiation. 42nd Conference of the European Radiation Reasearch Society. Amsterdam, Netherlands 2016:190
- Hankey KG, Farese AM, Blaauw EC, et al. Pegfilgrastim improves survival of lethally irradiated nonhuman primates. Radiat Res. 2015;183:643–655.
- National Institute of Allergic and Infectious Diseases. Pegfilgrastim approved for treating acute radiation syndrome. 2015. Available at: https://www.niaid.nih.gov/topics/radnuc/Pages/pegfilgrastim.aspx [Last accessed Aug 18, 2016]
- U.S. Food and Drug Administration. Investigational New Drug (IND) Application. 2017. Available at: https://www.fda.gov/drugs/developmentapprovalprocess/howdrugsaredevelopedandapproved/approvalapplications/investigationalnewdrugindapplication/default.htm [Last accessed 2017 Dec 23]
- Singh VK, Newman VL, Romaine PL, et al. Use of biomarkers for assessing radiation injury and efficacy of countermeasures. Expert Rev Mol Diagn. 2016;16:65–81.