ABSTRACT
Introduction: Protein kinases are a major target for small-molecule drug development. However, relatively few compounds are free of off-target toxicity and reach the clinic. Because the 500-plus kinases share conserved ATP-binding clefts, the site targeted by competitive inhibitors, generation of specific therapeutics remains a nearly intractable challenge.
Areas covered: Inducing degradation, instead of inhibition by occupancy-driven drugs, is an emerging strategy that offers the long-sought specificity, as well as mechanistic benefits. Currently approved inhibitors require steady-state binding and leave proteins intact for interactions in multi-protein complexes. After a general background about induced protein degradation, perspectives on protein kinases are provided.
Expert opinion: Induced degradation by state-of-the-art compounds (proteolysis-targeting chimeras, PROTACs) has been shown for protein kinases, albeit in early pre-clinical stages. Further work is required to expand the number of enzymes that could be exploited to direct proteins for degradation by ubiquitylation. In addition, despite the simple modularity of the chimeras, generation of hits will require empirical approaches due to the role of protein–protein interactions and distribution of tagging sites. However, given the advantages of degradation, drug discovery efforts targeting protein kinases should increasingly shift toward generation and screening of inducers of degradation and away from occupancy-based inhibitors of old.
1. Introduction
Induced protein degradation by small molecules, an alternative to occupancy-driven inhibition, has steadily grown as an attractive, promising drug discovery avenue over the last two decades. Perturbation of homeostasis and turnover of specific proteins by means of induced degradation is an event-driven strategy (), thus comparable to other down-regulation methods such as gene silencing and editing [Citation1,Citation2]. Rather than simply inhibiting phosphotransferase activity through steady-state occupancy of binding sites (active or allosteric), the small molecules serve as non-stoichiometric cofactors in cycles of catalytic degradation of the targeted proteins, an irreversible process. Thus, small-molecule inducers of protein degradation would be anticipated to show dramatically different activities relative to their traditional counterparts on both the molecular and cellular levels.
Table 1. Mechanisms of action underlying inhibition of cellular processes in drug discovery, comparing occupancy-driven with event-driven methods, proteinbinding inhibitors with nucleic acid targeting techniques, covalent with non-covalent inhibitors of enzyme activity or protein-protein interactions and two disparatetypes of inducers of protein degradation. See Expert Opinion section for further comparison and discussion
Though not a new concept (cf. 2.1–2.3), during just the last several years, the field has undergone a renaissance, due in large part to the innovation of hetero-bifunctional inducers of protein degradation, particularly proteolysis-targeting chimeras (PROTACs). Therapeutics that inhibit through degradation of proteins implicated in the pathology of diseases and disorders are already FDA-approved, including lenalidomide, which serendipitously targets a protein kinase (casein kinase 1α) along with two lymphoid transcription factors (see below) [Citation3]. Many others are in drug development pipelines as representatives of a cutting-edge approach in the pharmaceutical industry [Citation4]. To date, most inducers of protein degradation are arising from small start-up companies that are invested in or acquired by the larger pharmaceutical concerns. For example, in July 2014 in an agreement worth up to $1.725 billion, Genentech acquired Seragon Pharmaceuticals, a developer of selective estrogen receptor degraders (SERDs, cf. 2.2), to diversify the portfolio of the parent company Roche of hormone-receptor positive breast cancer drugs. In announcements, Genentech has metaphorically described the mechanism of action of the degraders as ‘designed to work by throwing the key driver of disease into what is essentially a cellular garbage can’ (the proteasome complex).
The more recently developed and far more widely applicable inducers of degradation, the above-mentioned PROTACs, originated in academic laboratories (Craig Crews, Yale; Raymond Deshaies, Cal Tech; Jay Bradner, Dana-Farber; Alessio Ciulli, Dundee; Nathanael Gray, Harvard; and others) and now are undergoing preclinical advancement at recently launched spinout companies such as Arvinas (New Haven, CT in 2013), C4 Therapeutics (Cambridge, MA in 2016), Kymera Therapeutics, (Cambridge, MA 2017); and Captor Therapeutics (Wroclaw, Poland and Allschwil, Switzerland in 2017) [Citation5]. As a reflection of burgeoning interest in the promising therapeutic avenue by industry, in just the last 3 years, three major pharmaceutical companies have poured large capital into Arvinas alone [Citation1]: Merck, up to $434 million [2015, Citation2] Genentech/Roche, more than $300 million (2015) and [Citation3] Pfizer, up to more than $830 million (2018) [Citation6]. Both C4 and Kymera Therapeutics have also announced similar partnerships in the short time since the inception of these academic spinoffs.
Big pharma, which could usher inducers of protein degradation through clinical trials, has good reason to be optimistic, as evidenced by the success of lenalidomide. Marketed as an IMiD or immunomodulatory drug by Celgene, the drug is a blockbuster that was not found through design but by chance. The IMiD lenalidomide, an analog of the banned drug thalidomide, binds the specificity-imparting recognition interface of an E3 ubiquitin ligase complex (RING-type, EC 2.3.2.27, as opposed to HECT-type, EC 2.3.2.26) [Citation7], antagonizing the ubiquitylation of endogenous substrates. In addition, however, the IMiD acts as a ‘molecular glue’ that unexpectedly redirects the E3 ligase by forming cryptic interfaces which promote recruitment of clinically important targets [two lymphoid transcription factors, Ikaros (IKZF1) and Aiolos (IKZF3), as well as the protein kinase (casein kinase 1α)]. Thus, due to this serendipitous neofunction, lenalidomide can act as a cofactor, rather than an inhibitor, in ubiquitylation and targeted degradation of the proteins [Citation8,Citation9]. With indications for multiple hematological disorders (myelodysplastic syndromes MDS), the lenalidomide topped the list of 10 best-selling cancer drugs of Q1 – Q3 of 2017 [Citation10]. Note that only two of the top 10 were conventional small-molecule kinase inhibitors. Monoclonal antibodies (biologics) made up half the list. Another was also a biologic, a growth factor for a blood cell disorder, neutropenia. The tenth was an inhibitor of protein degradation (proteasomal), meaning that of the top-ten cancer drugs, two were regulators of protein turnover (one positive, one negative).
Because Crews et al. and a host of others worldwide have authored numerous in-depth reviews focused on the chimeric degraders specifically and on induced protein degradation in general [Citation2,Citation9,Citation11–Citation16], in addition to seminal publications marking milestones in the development of PROTACs, this expert opinion will aim to draw attention to a specific promising application. Hence, following the presentation of a concise history of the topic to provide background and context, the review will focus on a subset of pharmacological targets, the protein kinase family, with perspectives on early efforts, current advances and the challenges and opportunities going forward with the development of efficacious inducers of kinase degradation.
2. Induced protein degradation by small molecules mediated by chaperones
2.1. Human epidermal growth factor receptor 2 (HER2) and EGFR: HSP90 chaperone-based mechanisms
Twenty years ago, a new class of inhibitor targeting tyrosine kinase receptors (EC 2.7.10.1) for growth factors was identified. Some irreversible inhibitors of HER2/Erb-2 [Citation17] were found to increase both ubiquitylation and endocytosis of the receptor kinase, leading to intracellular destruction of the proteins. Increased antitumor effects were observed in animals that appeared to stem from degradation rather than simple inactivation [Citation18–Citation20]. Though the degradative pathway was mediated by chaperones such as heat shock protein 90 (HSP90; EC 3.6.4.10), inhibition by small molecules (17AAG, related to geldanamycin, and PU24FC1, a purine-scaffold synthetic derivative) [Citation21,Citation22] at two sites within the chaperone was shown to also block the activity of HER2 [Citation23,Citation24] and another transmembrane kinase, EGFR (epidermal growth factor receptor; Erb-1), as well as other proteins involved in signal transduction such as steroid receptors and the RAF1 kinase (EC 2.7.11.1) [Citation25–Citation27]. HER2 degradation was also achieved with a depsipeptide which acetylated HSP90, along with downregulation of ERB1, RAF1and p53 [Citation28].
All of the above-mentioned mechanisms of degradation are based on the indispensable role of the molecular chaperone HSP90 in folding, stability, and function of ‘client’ proteins or substrates. Many client proteins sensitive to the activity of HSP90 are implicated in the pathogenesis of breast cancers (steroid hormone receptors ER and PR, receptor tyrosine kinases HER2 and EGFR, and components of oncogenic signal transduction cascades, AKT and RAF1). Inhibition of HSP90 activity causes the sensitive client proteins to misfold or destabilize, adopting aberrant conformations that induce polyubiquitylation and subsequent proteasomal degradation [Citation29–Citation31].In summary, small molecules have long been identified that regulated protein activity through degradation involving the chaperone HSP90; however, none were apparently sufficiently specific or efficacious to enter or emerge from the clinic [Citation32,Citation33].
2.2. Selective estrogen receptor modulators and degraders: ligand-binding alters conformation
Selective estrogen receptor modulators (SERMs) are small molecules with a history of acting as agonists or antagonists, at times differentially depending on tissue type. Selective estrogen receptor degraders (SERDs) are also targeted at the hormone-binding site of the nuclear receptors; however, they induce destruction of the protein [Citation34,Citation35]. Fulvestrant, a first-in-class SERD breast cancer therapeutic developed by AstraZeneca and in use since 2002, induces degradation by one of two ways [Citation1]: a ubiquitin-mediated mechanism due to recruitment of a specific E3 ligase by the SERD-estrogen receptor complex, or [Citation2] through exposure of hydrophobic residues due to destabilization by the SERD at the hormone-binding site, and recruitment of chaperones, which escort the partially unfolded receptor to the proteasome [Citation9]. The latter mechanism is derived from a co-crystal structure of estrogen receptor bound by a SERM, which interacts with the main chain through a carboxylate warhead, inducing a hydrophobic bulge, which mimics endogenous misfolding that recruits chaperones [Citation36]. Interestingly, though the results of recent extensive, sophisticated analyses by a large team predominantly from Genentech did not provide further insight into the underlying structural-functional mechanism of action of fulvestrant, an unanticipated cellular basis for induced degradation by the therapeutic antagonist was revealed: increased ER turnover is a consequence of intranuclear ER immobilization in the nuclear matrix, with low chromatin accessibility [Citation37].
2.3. HyT: hydrophobic tags, mimicking misfolded protein, acting as bait for chaperones
Based on earlier observations that the recruitment of chaperones, which typically recognize and assist in the refolding of misfolded proteins, could also lead to intracellular degradation, a method was developed that is referred to as hydrophobic tagging, or HyT [Citation38–Citation40]. A bifunctional or chimeric molecule, with a site-specific ligand on one end and a bulky hydrophobic moiety on the other recruits chaperones that fail to refold the baited protein, which is either escorted directly to the proteasome, or indirectly as a result of forming a ternary complex with an E3 ubiquitin ligase and undergoing covalent modification for targeting to the proteasome [Citation9]. As an example of the latter case, a HyT hydrophobic-tag (TX1-85–1) developed for the HER3 (human epidermal growth factor receptor 3, also known as ERB3) pseudokinase is a covalent bifunctional ligand that targets a cysteine residue (Cys781 in the ATP-binding site of HER3), resulting in the direct binding of chaperones followed by indirect recruitment of E3 ligases [Citation41]. The TX1-85–1 hydrophobic tag represented the first selective HER ligand. In the case of HER3 HyTs and for SARDs (selective androgen receptor degraders) [Citation39], inhibition of HSP90 accentuates targeted degradation, just as for HER2 irreversible inhibitors and SERDs.
2.4. H-SAAD/Ds: allosteric destabilizers of native state targeting the key hub of protein kinases
Recently, an in silico screen aiming to identify small-molecule stabilizers, or pharmacological chaperones, of a dysregulated bone morphogenetic protein receptor kinase (cf. 5.1, below) was performed by targeting a shallow surface site formed in part by the αC-β4 loop, the key hub responsible for allosteric regulation of activity of protein kinases. No stabilizing compounds were found, however, destabilizing hits were identified that shared warhead groups with sp2 nitrogen atoms in heterocyclic rings. They were hypothesized to serve as hydrogen-bond acceptors, triggering instability of the loop through interaction with the multi-valent guanidino group of an arginine residue (Arg 258) within the segment (). Deemed hypoxia-selective (H-S) Activin receptor-like kinase Allosteric Destabilizers and Degraders due to the requisite participation of an ionizable histidine (His 318) within the hub, the set of commercially available compounds have thus far been studied exclusively in vitro [Citation42].
Figure 1. A hypoxia-selective allosteric destabilizer and degrader (C28, green) with sp2 nitrogen hydrogen-bond acceptor warhead, docked on a ring-like, surface pocket of a bone morphogenetic protein (BMP) receptor kinase that is comprised in part by the αC-β4 loop (red), the key allosteric hub responsible for conformational plasticity and instability of protein kinases
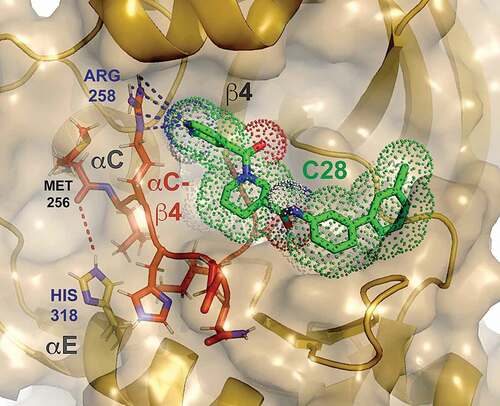
Thus, cell-based studies will be required to establish whether the destabilizers also act as inducers of degradation. Nonetheless, the chaperone-mediated turnover of other conformationally perturbed or HyT-tagged proteins provides solid precedent for the likelihood of inducing degradation in the cell. Moreover, given that the destabilizing activities of H-SAAD/Ds were not only markedly sensitive to changes in pH within the physiological range (pH 6.5 > 7.25), but also to changes in temperature (37°C > 30°C), the efficacies of the allosterically acting compounds and thus the potential for degradation of the target activin receptor-like kinase would be enhanced under hypoxic conditions in mammalian cell-based assays, in mouse models and in patients (cf. 5.1).
3. Induced protein degradation by small molecules mediated by polyubiquitin-tagging
3.1. Bifunctional target-specific, E3 ubiquitin ligase-baiting inducers of degradation
In addition to HyT-tagging, a second method which arose earlier has been continuously developed in parallel that is based on tagging with ubiquitin chains, which mark the targets for direct degradation by the proteasome instead of through an indirect chaperone-mediated process. Proteolysis-targeting chimeras (PROTACs), like the hydrophobic tags, are hetero-bifunctional molecules, yet functionally more refined. Rather than chaperone-baiting hydrophobic moieties, E3 ubiquitin ligase-baiting ligands are employed, again fused to a target-specific ligand, such as a competitive inhibitor [Citation2,Citation11–Citation16,Citation43–Citation48]. A pair of related examples are depicted in [Citation49]. PROTAC 3 and PROTAC 7 share von Hippel-Lindau E3 ligase-recruiting moieties to direct polyubiquitylation of the target proteins, yet differ with respect to the target-specific ligands. The former, with a gefitinib-based warhead, binds specifically to the EGFR kinase, whereas the latter, with a foretinib counterpart, binds c-Met.
Figure 2. Modular composition of proteolysis-targeting chimeras for protein kinase degradation [Citation45]. 2D structures: PROTAC 3 – Pubchem CID 135156947, PROTAC 7 – Pubchem CID 13810841
![Figure 2. Modular composition of proteolysis-targeting chimeras for protein kinase degradation [Citation45]. 2D structures: PROTAC 3 – Pubchem CID 135156947, PROTAC 7 – Pubchem CID 13810841](/cms/asset/5a7ac2b4-0029-414c-9afd-2ca1445ebcde/iedc_a_1660641_f0002_oc.jpg)
An additional difference, under-appreciated in two-dimensions, is the length and composition of the polyethylene glycol linkers connecting the E3 ligase- and kinase-binding ligands. As shown below in a model of a crystal structure of a PROTAC-mediated complex [Citation50], the proper parameters could prove crucial for providing for an extensive and stable protein–protein interface (). Specifically, Cuilli and coworkers deduced that rational design of a bifunctional molecule that ‘folds on itself’ is of key importance, resulting in recruitment of E3 ligase and target protein into close proximity and formation of a stable ternary complex. Though burial of extensive surface area, formation of specific protein–protein interactions and reduction in entropic cost were all cited as potential beneficial effects of optimal linker length, composition and attachment positions and vectors, the degree of cooperative complexation was found to be dictated by the extent of surface complementarity between E3 ligase and target protein, that in turn was determined by the relative orientation of the two macromolecules directed by a modular hetero-bifunctional ligand.
3.2. PROTACs provide major advantages over HyT-tagging and occupancy-based inhibitors
As anticipated, a chief advantage of the PROTACS method has been experimentally confirmed: the extent and duration of signal inhibition resulting from degradation is significantly greater than for steady-state competition by a simple active site, occupancy-based inhibitor [Citation49]. Less anticipated was the finding that the relative orientations or positions of the components of the ternary complex of target protein, PROTAC, and specific E3 ligase could dramatically alter degradative activity. A broad-spectrum inhibitor conjugate was found to result in the degradation of a limited subset of protein kinases that bound the inhibitor alone (cf. 4.11) [Citation1,Citation9,Citation47,Citation49]. On the one hand, the substantial additional level of specificity that could be achieved is a major plus, however, on the other, despite the simplistic modularity of the approach in principle, in practice, empirical testing and optimization would likely be necessary for each specific protein targeted.
In addition to the aspect of enhanced selectivity, another significant benefit of PROTACs methodology has been brought to the fore by two independent groups within the last year: potential for poly-pharmacological mechanisms to accentuate the therapeutic effect of targeted degradation. Mikihiko Naito and coworkers at the National Institute of Health Sciences (Japan) have successfully combined target protein knockdown with cytocidal activities by also degrading cellular inhibitor of apoptosis protein 1 (cIAP1) or X-linked inhibitor of apoptosis protein (XIAP) [Citation51–Citation53]. The chimeric dual-degraders are referred to by the acronym, SNIPERs: Specific and Nongenetic IAP-dependent Protein ERasers. Craig Crews and coworkers have succeeded in constructing a chimera that degrades a target protein (BRD4, bromodomain-containing protein 4, an epigenetic regulator that recognizes acetylated lysine residues) while also stabilizing p53, producing a synergistic antiproliferative effect of multiple cancer cell lines. The nutlin-based PROTAC, one of few, hijacks the E3 ligase MDM2, knocking down BRD4 and upregulating the tumor-suppressor protein. As a result of the poly-pharmacological mechanism, proliferation is inhibited more effectively than a corresponding VHL-utilizing PROTAC with similar BRD4-degrading activity [Citation54].
3.3. Future applications of PROTACs for a broad spectrum of protein targets in drug discovery
Arvinas, in agreements with Pfizer, has developed PROTACs for estrogen (ARV-471) and androgen receptors (ARV-110) for oral delivery with improved pharmacodynamic and pharmacokinetic properties [Citation55]. The AR-degrading chimera is the first PROTAC drug to enter the clinic, currently in Phase 1 to evaluate safety and tolerability in patients with metastatic castration-resistant prostate cancer who have progressed on standard care therapies. According to Arvinas, in contrast to traditional antagonism through a competitive- and occupancy-driven process, degradation is iterative and hence less susceptible to increases in expression and mutations in the hormone receptors, enabling PROTACs to overcome known mechanisms of resistance to current standards of care.
Other targets of interest include the so-called ‘undruggable’ proteins that lack biologically relevant binding sites (substrate or ligand), but might possess clefts or pockets that could afford modest affinity for specific or selective small molecules identified in screens of compound libraries (chemical or in silico) or by rational design. As will be advocated below, protein kinase targets should be revisited or explored with the cutting-edge method. First though, for proof-of-concept, published preclinical successes will be briefly summarized.
4. Protein kinases targeted for proteolysis by PROTACS: preclinical proof-of-concept
For historical perspective and to provide supporting experimental evidence for the applicability of PROTAC-induced degradation to protein kinases, a roster of examples is presented below. For more in-depth background, see two recent excellent reviews by Tan and Gray [Citation56] and by Ferguson and Gray [Citation57]. In addition to a typical modular pair depicted above (, PROTAC 3 and PROTAC 7), the chemical structures of hetero-bifunctional molecules targeting protein kinases are available in the primary references cited in each subsection, or can be viewed and compared in the representative collection compiled by Tan and Gray [Citation56].
4.1. Receptor-interacting serine-threonine kinase 2 (RIPK2): first application of VHL ligands
In 2015, the first applications of a VHL E3 ligase ligand as a component of modular PROTAC designs were reported, including one for degradation of a protein kinase, RIPK2 (EC 2.7.10.2) [Citation58]. As a central mediator of the innate immune response triggering cytokine release, aberrant RIPK2 signaling is implicated in inflammatory bowel disease and sarcoidosis, which could thus be potentially treated by RIPK2-selective PROTACs [Citation45]. Previous constructs suffered from multiple undesirable properties due to their reliance on an inferior HIF1α peptide fragment as bait for E3 ligase. The synthetic VHL ligand was actually a tert-butyl-hydroxyproline derivative (ligand 7; N-acetyl-3-methyl-L-valyl-(4R)-4-hydroxy-N-[4-(4-methyl-1,3-thiazol-5-yl)benzyl]-L-prolinamide) of the first compound (ligand 1; methyl 4-(((2S,4R)-4-hydroxy-1-(2-(3-methylisoxazol-5-yl)acetyl)pyrrolidine-2-carboxamido)methyl)benzoate) that had been identified through in silico and fragment-based screens announced 3 years earlier [Citation59,Citation60]. Through structure–activity relationship studies conducted by Ciulli and coworkers in Dundee, ligand 7 was imparted with a significantly lower KD (185 nM) than the parent molecule [Citation61].
Consistent with the high affinity for of the synthetic derivative for the VHL E3 ligase, RIPK2_PROTAC was shown to act catalytically and reduce endogenous protein levels by 90% at nanomolar concentrations. Furthermore, of the approximately 7,000 proteins quantitatively analyzed, only one other, MAPKAPK3 (MAP kinase-activated protein kinase 3), was significantly degraded, hence RIPK2_PROTAC proved to be highly selective as well as active. Interestingly, in keeping with the high selectivity afforded by the hetero-bifunctional compound, other kinases in the screen were bound by RIPK2_PROTAC but not degraded (RIPK3, ABL, and TESK), at least under the conditions of the assay [Citation58].
4.2. TANK-binding kinase 1 (TBK1): specifically degraded despite non-specifically inhibited
TANK-Binding Kinase 1 [TBK1; tumor necrosis factor receptor-associated factor or TRAF-family member-associated NF-κB activator (encoded by the TANK gene) kinase 1; EC 2.7.11.10] is an IκB (IKK)-related kinase that can activate NF-κB in a kinase-dependent manner [Citation62,Citation63]. PROTACs targeting TBK1 were synthesized with VHL-binding ligand to recruit the E3 ligase. Intriguingly, despite the kinase-binding moiety inhibiting two related kinases (TBK1/IKKε), only one was degraded (TBK1) and not the other (IKKε).
4.3. Human epidermal growth factor receptor 2 (Her2) and EGFR: first PROTACs for RTKs
Her2 and EGFR are validated targets for a subtype of non-small cell lung cancers (NSCLCs) and other tumors as well as one type of breast cancer, with FDA-approved inhibitors in the clinic. In the first demonstration of the applicability of the strategy to the receptor tyrosine kinase (RTK) family, PROTACs for the two have been produced with the specific inhibitors linked to a VHL-binding ligand [Citation49].
4.4. Mesenchymal-to-epithelial transition RTK (c-MET): degradation versus simple inhibition
In a seminal paper from Crews and coworkers, the c-MET proto-oncogene, a receptor tyrosine kinase is targeted by PROTACs, along with two others, the EGFR and HER2 as well as multiple mutants of EGFR and c-MET (mesenchymal–epithelial transition factor, the receptor for hepatocyte growth factor/scatter factor or HGF/SF ligand) [Citation49]. Fully functional, target-degrading PROTACs were compared with target-inhibiting variants that contain an inactivated E3 ligase-recruiting ligand (rather than the parent tyrosine kinase inhibitor warheads alone). The results of the studies show significant advantages of degradation versus simple inhibition [Citation1]: degradation provides a more sustained down-regulation of signaling even after washout [Citation2], activity-independent, protein–protein interaction scaffolding functions are eliminated and [Citation3] mutated kinases that might otherwise switch off as a result of treatments are disposed of. The findings provide a major proof-of-concept for the broader application of PROTACs to target transmembrane receptor kinases and the efficacy of recruitment of the VHL E3 ligases for down-regulation of the signaling proteins from the plasma membrane.
4.5. ABL: first clinical small-molecule kinase inhibitors employed as warheads in PROTACs
Derivatives of imatinib, the first small-molecule kinase inhibitor (SMKI) to reach the clinic, were synthesized by Takeda (Japan) to induce to degradation of BCR-ABL, a fusion of the breakpoint cluster region protein (BCR) and the Abelson murine leukemia viral oncogene homolog 1 (ABL1) tyrosine kinase (EC 2.7.10.2) often found in chronic myelogenous leukemia (CML) and acute lymphocytic leukemia [Citation64,Citation65]. Hetero-bifunctional compounds of imatinib, dasatinib, and two other ABL inhibitors were conjugated to three different E3 ligase baits via PEG linkers of varying length. Similarly, Crews and coworkers synthesized dasatinib-based PROTACs targeting the ABL kinase [Citation66]. Inhibitor specificity, linker lengths and the specific type of E3-interacting bait were all found to be important, empirically determined parameters. Recently, Takeda and academic partners dissected the degrader and inhibitor functions of dasatinib-based PROTACs, demonstrating that a BCR-ABL degrader provides more sustained inhibition of CML cell growth than the ABL kinase inhibitor counterpart [Citation67].
4.6. Cyclin-dependent kinase 9 (CDK9): unanticipated gain or loss of selectivity of PROTACs
Cyclin-dependent kinases (EC 2.7.11.22) are perhaps best known for their role in cell cycle control; however, a second major functional group is involved in the control of transcription. For example, CDK9 is the catalytic subunit of transcription elongation factor b (P-TEFb), which phosphorylates the C-terminal domain of RNA polymerase II (EC 2.7.7.6), stimulating transcription [Citation68,Citation69]. Because P-TEFb phosphorylates other components (transcription factors) that regulate elongation of transcription in an array of cellular and physiological processes, CDK9 has been deemed an appealing candidate for therapeutic intervention [Citation70].
In order to specifically target the multifunctional cyclin-dependent kinase, Gray and coworkers recently reported the development of a PROTAC for CDK9 (Thal-SNS-032) based on a selective CDK2/7/9 inhibitor (SNS-032) [Citation71]. In eleven leukemia cell lines, the PROTAC-induced significant degradation of CDK9, and importantly, enhanced anti-proliferative effects compared to the inhibitor alone. Because the PROTAC only degraded CDK9, rather than other targets of the inhibitor as well, and because chimeras based on a more selective and potent CDK9 inhibitor (NVP-2) proved less efficient, two key aspects of the approach were brought to light [Citation1]: PROTACs can exhibit more selectivity than the parental inhibitors, which however [Citation2] do not necessarily translate their efficacies as modular components of hetero-bifunctional degraders.
4.7. Bruton’s tyrosine kinase (BTK): cooperative protein–protein interactions not required
BTK is a non-specific, non-receptor tyrosine kinase (EC 2.7.10.2) required for B cell maturation and a therapeutic target for cancers of the blood. Pfizer has investigated the applicability of the PROTACs methodology for BTK with an eleven-compound library with ligand to recruit the E3 ligase cereblon (CRBN) and linkers of different lengths. Beyond simply determining whether ternary complexes of BTK, CRBN, and PROTAC formed, cooperativity of binding was also assessed. In contrast to a key role for cooperativity proposed for other protein complexes assembled by the hetero-bifunctional ligands, none was observed nor was found necessary for efficient degradation of the POI. However, consistent with most if not all other studies with small libraries of PROTACs, linker length was crucial for alleviating steric clashes between the two protein components brought together by the chimeric small molecule [Citation72].
4.8. Focal-adhesion kinase (FAK): elimination of protein scaffold function, not simply activity
FAK is another non-specific, non-receptor tyrosine kinase (EC 2.7.10.2), which is required in tumor invasion and metastasis, functions as more than a phosphotransferase enzyme, but also as a scaffold for protein complex formation. Perhaps not surprisingly then, in preclinical studies, FAK inhibitors combined with other therapeutics are significantly more efficacious than either unaided agent [Citation73]. To determine whether degradation of the FAK scaffold and the concerted abolishment of kinase activity would improve the performance of FAK inhibitors alone, a selective and potent FAK degrader (PROTAC-3; ) was compared with the parent compound, defactinib [Citation74]. Indeed, the inducer of degradation outperformed the inhibitor as judged by measurement of activation of the FAK enzyme and cellular processes mediated by FAK (cell migration and tissue invasion), providing evidence of the potential for PROTACs to control dysregulation stemming from protein kinases with enzymatic and scaffolding functions.
4.9. Extracellular signal-related kinases 1 and 2 (ERK1/2): a permeable pro-drug PROTAC
ERK1 and ERK2 (mitogen-activated protein kinases; EC 2.7.11.24) regulate a broad array of cytosolic and nuclear proteins as substrates of phosphorylation and are part of an activation cascade (Ras-Raf-MEK-ERK) responsible for approximately one-third of all cancers [Citation75]. The highly mutated RAS small monomeric GTPase (EC 3.6.5.2) has been recalcitrant to efficacious inhibitor development to date, thus attention has been focused on other components of the signal transduction cascade.
Toward that end, ERK1 and ERK2 have been targeted by two small prodrug precursors with good permeability that can combine intracellularly via bio-orthogonal click chemistry (inverse electron demand Diels-Alder or IEDDA cycloaddition) to form an active, wholePROTAC or CLIPTAC (Astex Pharmaceuticals, Cambridge, UK) [Citation76]. Performing the click chemistry prior to addition to cultured cells (human melanoma cell line) resulted in undetectable levels of targeted degradation in western blot assays. In marked contrast, step-wise addition of the two prodrugs at 1 μM each produced complete degradation of ERK2 and near-so for ERK1 after 16 h co-incubation. Given the relatively high molecular weights inherent to linked, bifunctional compounds, synthesis of PROTACs as self-assembling prodrug pairs (CLIPTACs) is an attractive option for future design strategies for drugs entering the clinic.
4.10. Promiscuous, multi-kinase inhibitors as modules of PROTACs: additional selectivity
Two seminal papers with respect to development of PROTACs for degradation of proteins-of-interest (POIs) in general, but focusing on protein kinases specifically were published as a suite along with that of the RTK-targeting studies of Crews and coworkers (cf. 4.4) in Cell Chemical Biology in January of 2018 and highlighted with a preview article [Citation1]. In one of the two, Crews and coworkers also synthesized PROTACs composed of the promiscuous protein kinase inhibitor foretinib (cf. 3.1: for structure, cf. , warhead component of PROTAC 7), polyethylene glycol linkers and VHL or cereblon ligands (VHL PROTAC 1 and CRBN PROTAC 2) [Citation47]. Similarly, in the other seminal paper, Nathanael Gray and coworkers surveyed the degradable kinome with a promiscuous kinase-directed hetero-bifunctional degrader synthesized from a versatile diaminopyrimidine scaffold directed at the ATP-binding site (TL12-186), polyethylene glycol linkers and the cereblon-recruiting IMiD analog, pomalidomide [Citation43].
Both studies demonstrated that additional selectivity arises such that only small subsets of the kinases tested were effectively or even detectably degraded [Citation43,Citation47]. The structural and functional origins of the additional layers of selectivity were hypothesized to include complementary protein–protein interactions between the POIs and E3 ligase recruited by the bait moiety of the PROTACs, as well as possible low affinity but cooperative interactions in the ternary complex composed of the hetero-bifunctional ligand, POI and E3 ligase. Orientation of the E3 complex with respect to target lysine residues (cf. 6.5, below) might also be one of the multiple factors responsible for high specificity and low off-target toxicity that has been long-sought after for small-molecule protein kinase therapeutics.
Indeed, while this article was under review, Crews and coworkers had further reported that one isoform of a protein family could be selectively targeted over others simply by varying the linker length and orientation of a single E3 ligase. Moreover, stable ternary complex formation was found to be necessary but not sufficient for robust degradation, consistent with the hypothesis above [Citation77].
5. Protein kinases in multi-protein complexes offer the enhanced potential for quenching dysregulated signaling: mutant ALK2 BMP receptor kinase as a putative case
5.1. Targeting dysregulated BMP signaling that leads to severely debilitating bone deposition
Though rare, the heterozygous mutation (R206H) in a type I (ligand-activated) bone morphogenetic protein (BMP) receptor kinase (ACVR1/ALK2; activin receptor-like kinase 2; receptor protein serine/threonine kinase, EC 2.7.11.30) is linked to a severe musculoskeletal disorder (FOP; Fibrodysplasia Ossificans Progressiva; OMIM 135100) causes metamorphosis of soft tissues into extra-skeletal or heterotopic bone, restricting mobility throughout the body in a stepwise, cumulative manner. At present, at least six pharmaceutical companies (BioCryst, Blueprint, Clementia/Ipsen, Keros, La Jolla, Regeneron) have made efforts to develop a therapeutic for FOP, which cannot currently be effectively managed much less prevented or even diminished in frequency or extent. All but two are developing ATP-competitive, selective inhibitors of ALK2 receptor kinase. Regeneron has entered phase 1 clinical trials with a monoclonal antibody that neutralizes the non-canonical ligand (activin) with a specific neofunction of activating the R206H mutant but not wildtype ALK2 receptor kinase in the heterozygous FOP patients. Clementia/Ipsen has ushered a retinoic acid receptor-γ agonist (orphan drug designation, initially developed by Roche), palovarotene, through ongoing phase 2 and phase 3 trials, that broadly dampens BMP signaling by reducing SMAD1/5/8 phosphorylation [Citation78]. Unfortunately, palovarotene showed severely adverse effects on skeletal maturation in a mouse model, perhaps due to the dampening of all BMP signaling and/or the chronic administration required to retard or restrain HO [Citation79]. Interestingly, proteasome-mediated degradation of the phosphorylated downstream effectors is the proposed mechanism of action, based on the promotion of degradation of phosphorylated SMAD1 by all-trans-retinoic acid [Citation80].
Because heterotopic bone formation also arises without mutation yet due solely to soft-tissue trauma from high-velocity gunshot wounds and explosive injuries (IEDs), extensive burns, brain, and spinal cord injuries and from complications of elective arthroplasties (hip and knee replacements), inhibition of aberrant BMP signaling have applications in additional larger patient populations, both military and civilian [Citation81,Citation82]. In mouse models without the gain-of-function mutation in ALK2, trauma triggers upregulation of transcription of genes encoding BMP ligands, which in turn then are hypothesized to increase signaling aberrantly through any or all of the three activin receptor-like kinases (ALK2, ALK3, ALK6) that transduce BMP signals.
Of note, Levi and coworkers showed that knockdown of any one of the three ALK-encoding genes did not inhibit trauma-induced HO (tHO), however, combined ablation of ALK2 and ALK3 produced near-total elimination [Citation81]. Thus, for treatment of non-genetic tHO patients, broadly selective therapeutics targeting two or all three of the redundantly acting type I BMP receptors, or an ALK2-selective in combination with an ALK3- and/or ALK6-selective compound, would be necessary. Such a therapeutic might be tolerated for the treatment of acute cases, whereas for FOP, which is a life-long, chronic affliction, a highly selective ALK2-targeting drug would almost certainly be required. Ideally, the drug could be administered as a prophylactic against the stepwise accumulation of mobility-arresting heterotopic bone.
5.2. Promiscuous PROTAC does not induce degradation of ALK2
Intriguingly, although in non-pathological contexts, ligand-activated ALK2 receptor kinase is degraded by an endogenous negative feedback mechanism involving a specific E3 ubiquitin ligase in a ternary complex with a negative regulatory protein [Citation83–Citation86] (cf. 5.3), induced degradation of the protein was not observed with the putatively promiscuous PROTACs (VHL PROTAC 1, CRBN PROTAC 2) described above (4.10) [Citation47]. Though the promiscuous parent inhibitor (foretinib, 10 μM) was detectably diminished phage-displayed ALK2 receptor kinase activity in a KinomeScan assay (70%), inhibition by VHL PROTAC 1 was negligible (6%) and inhibition by CRBN PROTAC 2 abolished (both at 10 μM). Hence, ALK2 receptor kinase apparently must be targeted by a modified PROTAC with different linker length, composition or site of attachment, or with a ligand bait for a different E3 ligase.
In addition to a systematic, modular screening approach, consideration of the structure and mechanism of assembly of the multi-protein signaling complex containing ALK2 could enhance and streamline the development of an efficacious drug for the diverse, debilitating bone-forming musculoskeletal pathologies (cf. 5.4, below). In support of the proposed drug discovery approach, two proteins interacting with the cytoplasmic kinase of ALKs, a downstream-effector substrate (R-Smad3) [Citation87] and a dampening protein (FK-506 binding protein 12 kDa, FKBP12; peptidylprolyl isomerase, EC 5.2.1.8) [Citation88], can be degraded with hetero-bifunctional small molecules. In the case of the signaling-dampening binding protein, induced degradation should be avoided, since competitive inhibition of type I BMP receptor binding of FKBP12 by the FK506 ligand leads to receptor activation and promotes osteogenic differentiation [Citation89].
In the case of the downstream-effector SMADs, the therapeutic effect of targeted degradation would be anticipated to be commensurate with that of the ALK2 receptor kinase itself [Citation90–Citation93], however, since the effect would be broad, quenching all BMP signaling, the result for chronically dosed FOP patients would be highly undesirable. That said, for acute treatment of non-genetic tHO, non-selective quenching of BMP signaling from the pool of type I receptor kinases could prove adventitious, offering an alternative avenue from ATP-competitive inhibitors that have undesirable off-target effects [Citation81].
Since type II receptor kinases (activin type II receptors ActRIIA, IIB and the bone morphogenetic protein type II receptor, BMPRII, EC 2.7.11.30) serve dual roles as upstream phosphorylating enzymes and as scaffolds that form heterodimers with ALK2 [Citation94], targeting this component of the signaling complex for destruction might also prove efficacious. Unlike directed degradation of BMP-specific SMADs (1/5/8) recruited to the signaling complex, directed degradation of type II receptors including ActRIIA and IIB, which are required for signaling by activin ligands through SMADs 2/3, the effects would therefore be overly broad. Hence just as for PROTACs composed of ALK2-selective warheads that might induce the degradation of signaling-dampening FKBP12, those that lead to or allow for degradation of type II receptors would be undesirable.
Note that an overarching conclusion from consideration of the effects of degradation of the proximal components in the signaling complex directed by a PROTAC composed of an ALK2-selective warhead is the importance and necessity for determining the effects on the stability of not just the type I receptor kinase but all of the components in the ligand-induced signaling complex.
5.3. Activated ALKs are degraded by an endogenous negative feedback mechanism in complex
In the canonical pathway, signals are transduced by BMP/TGF-β superfamily ligands solely by downstream-effector substrates (receptor-regulated or R-SMADs) of activated endocytic receptor complexes that, upon phosphorylation and hetero-trimerization, translocate into nuclei of the receiving cells to turn on- and off-target genes [Citation95]. To perhaps compensate for the simplicity of the transduction mechanism, two negative feedback loops down-regulate signaling through proteasomal degradation of pathway components. In one case that is independent of signaling, pools of R-SMADs are maintained at basal levels to set the sensitivity to signal input, while in a distinct, signaling-dependent process the activated receptor kinases in complex with inhibitory I-SMA6 or 7 are targeted for destruction [Citation83–Citation86].
In the latter case, binding of inhibitory I-Smad to activated type I receptors (ALKs), which form stable complexes, leads to association with and modification by specific E3 ubiquitin ligases (SMURFs1/2; SMAD ubiquitin regulatory factors). Thus, I-SMADs act as adaptors for composite interactions with an E3 ligase and activated ALK receptor kinase. In a pathological context, after pharmacological (AMPK activators metformin or aspirin) upregulation of inhibitory SMAD6 and SMURF1 E3 ligase in fibroblasts and induced pluripotent stem (iPS) cells from a FOP patient, activated ALK2 mutant receptor was shown to be degraded by the ubiquitin-proteasome system, presumably as a result of enhanced formation of a receptor kinase:I-SMAD:E3 ligase complex [Citation96].
In summary, activated wildtype and mutant ALK2 receptor kinases are downregulated by endogenous or pharmacologically induced degradation by the ubiquitin-proteasome pathway, respectively, yet PROTACs derived from a promiscuous protein kinase inhibitor (cf. 5.2), fail to bind much less induce the degradation of the susceptible protein. Therefore, toward design and synthesis of ALK2-targeted PROTACs, rather than by trial-and-error, a more rational approach is called for.
5.4. Model for ALK2-containing heterotetramer with R-Smad effector substrate recruited
The serine-threonine receptor kinase (Activin receptor-like kinase 2 or ALK2) is a component of a ligand-assembled, heterotetrameric-receptor complex, which due to multiple binding sites and interacting partners, affords manifold opportunities for quenching the dysregulated signaling of a pathology-triggering, gain-of-function mutant (R206H). To date, only the crystal structures of the extracellular assemblages of BMP/TGF-β signaling complexes have been determined [Citation97–Citation100]. Interactions between the cytoplasmic kinases of the receptors and the substrate R-SMADs have been thoroughly characterized [Citation101,Citation102]; however, no binary or ternary complex structures crystallized, save for FKBP12-bound ALKs.
In order to guide the rational, modular design of PROTACs as therapeutics for dysregulated ALK2 signaling, a stepwise in silico-docked model of the cytoplasmic components of the BMP signaling complex was generated that serves as a paradigm for targeting other protein kinase complexes (). Since the two types of receptor kinases (EC 2.7.11.30) associate as heteromeric dimers in the absence of ligand [Citation94,Citation103], in the first step crystal structures of cytoplasmic constructs of ALK2 (PDB ID 3H9R) and BMPRII (PDB ID 3G2F) kinases were docked in silico with state-of-the-art routines via the ClusPro web server [Citation104]. In the next step, after partial truncation (autoinhibitory α-helix 1) of ALK2 kinase in the docked heterodimer, the crystal structure (1KHUA) of the protein-interacting (MH2), phosphopeptide-recognition or forkhead-associated (FHA) domain of a BMP R-Smad was then docked to form a ternary complex of the preformed kinase heterodimer and effector substrate.
Figure 4. Stepwise in silico-docked model of homodimeric bone morphogenetic protein (BMP) ligand-induced, heterotetrameric signaling complex that would provide expansive surface area, including six unique composite protein:protein interfaces, for recruitment of substrate-recognition E3 ligases, which, in addition to those of the PROTAC warhead-binding protein kinase itself (type I), could potentially modify an even broader assortment of lysine residues
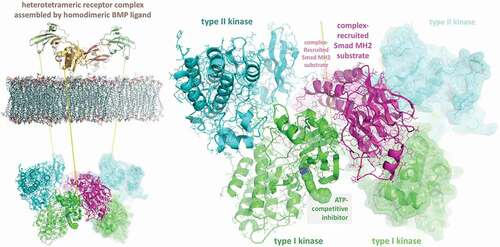
In the final step, two ternary complexes were in turn docked to generate the ligand-induced, cooperatively assembled cytoplasmic signal transduction complex of two kinase heterodimers with two R-Smad substrates recruited for phosphorylation. In addition to the requisite and pronounced complementarity of the manifold surfaces within the six-chain complex, several structural elements are juxtaposed to provide for the specificity of the interactions and site-specific phosphorylations, hence the in silico model generated by the ClusPro routines appears reliable, suggesting that other signaling complexes might be investigated via a similar step-wise process.
Consideration of the structure of a multi-protein signaling complex in the modular design of PROTACs provides several potential benefits. First, the position of the linker extending from the kinase inhibitor might require relocation, in order to prevent steric clashes or to render to the other moiety, the E3 ligase ligand, greater potential for protein–protein interactions within the complex. Second, determination of the length and composition of the linker itself might be facilitated by rational design, rather than blind, systematic syntheses and functional tests, or at minimum by iterations of tests of small sets followed by rational design. Third, as demonstrated in the BMP heterotetrameric-receptor assembly, a second kinase active site (type II receptor) could be targeted by a competitive inhibitor linked to an E3 ligand. Fourth, visualization of a model of a multi-component complex reveals the necessity of analyzing all of the proteins with respect to degradation, rather than simply that bound by the inhibitor module of the PROTAC.
Finally, in silico screening of virtual libraries of small molecules that target pockets or clefts created at protein–protein interfaces within the complex could provide not only new leads, but ones which would limit degradation to components actively involved in signaling, rather than the total cellular pool in the absence of activation. Along those lines, the PROTAC for the downstream-effector substrate (R-Smad3) [Citation87] referred to above (cf. 5.2) was developed through in silico docking and rational design. However, since the R-SMAD was targeted as a free effector, rather than as a component of an activated signaling complex, the low efficacy and toxicity of the designed compound might be overcome by investigating second-generation derivatives based on an in silico-docked model for the related TGF-β receptor assemblage.
5.5. Degradation of BMP signaling complexes in other contexts underscores feasibility for therapeutic intervention with rationally designed PROTACs
Note that, in addition to the SMURF1/2 and SMAD6/7 negative feedback loops discussed above (cf. 5.3), BMP signaling complexes have been shown to be subject to degradation by the ubiquitin-proteasome system in other cellular contexts. For example, the Drosophila BMP receptor kinase ortholog, TKV, is degraded by the proteasome downstream of an S6 serine-threonine kinase family member (S6KL), inhibiting the growth of neuromuscular junctions and stimulating endocytosis at the synapse [Citation105]. In another case, activated BMP receptor-regulated SMAD was shown to be degraded following interaction with and polyubiquitylation by an E3 ligase, NEDD4 (neural precursor cell expressed, developmentally down-regulated 4) [Citation106]. Such examples of the degradation of receptors and effectors underscore the feasibility for therapeutic intervention by application – ostensibly through rational design – of PROTAC methodology in human diseases and disorders caused by dysregulated BMP/TGF-β signaling.
5.6. Another potential application of rational design of PROTACs for protein kinase complexes
Of course, the model of a complex of signaling proteins focused on above is only one example. If available, crystal structures of complexes of protein isoforms to be selectively targeted would be preferable and provide three-dimensional templates for the rational design of PROTACs that stabilize ternary complexes in a productive fashion (cf. 4.10) [Citation77]. One such candidate would be complexes of CDKs and cyclins, which are well represented in the Protein Data Bank (e.g. 1FIN, CDK2-Cyclin A; 3G33, CDK4-Cyclin D3; 1G3N, CDK6-Cyclin D; 5HQ0, CDK1-Cyclin B; 5L2W, CDK2-Cyclin E; 3TN8, CDK9-Cyclin T; 3RGF, CDK8-Cyclin C; 4YC3, CDK1-Cyclin B1). Superposition of the complexes (PyMOL) reveals a deep cleft between the chains proximal to the ATP/competitive inhibitor-binding site that is structurally highly variable, primarily due to dramatic differences in the conformation of the activation segment or A-loop. Hence, structure-based design might impart specificity to otherwise only marginally selective or even promiscuous inhibitors of CDKs if rationally coupled to E3 ligases.
5.7. Considerations and concerns inherent to protein kinases composed of catalytic and regulatory subunits or proteins
Similar to the concerns raised about the misdirected degradation of the type I BMP receptor-dampening protein FKBP12 by a PROTAC composed of an ATP-competitive inhibitor as warhead targeting the cytoplasmic kinase (cf. 5.2), rational design and subsequent analyses of the activities of PROTACs targeting CDK-Cyclin complexes should bear in mind the potential for misdirected degradation of the cyclin regulatory proteins rather than specific CDKs. Such concerns should actually be extended to any protein kinase composed of catalytic and regulatory subunits or proteins, and would be expected to vary in effect depending on the nature of the protein pair, e.g. whether or not the regulatory subunits interact with more than one kinase. If so, off-target effects might arise indirectly through degradation of the regulatory subunit pool, altering stoichiometries and concomitantly the activities of other kinases or binding partners.
6. Non-degradative effects: further considerations and caveats of protein ubiquitylation
As will be reiterated amongst the conclusions, because the focus of this review has thus far been on the degradative effects of polyubiquitylation, the caveat should be voiced that modification with ubiquitin-protein moieties can also have a multitude of non-degradative effects that newcomers to the field should become mindful of and keep under consideration, such as inhibition of degradation and activation of protein kinases. In addition, enzyme-catalyzed deubiquitination is also a burgeoning field of study that is currently commonly included in conferences focusing on PROTAC-mediated degradation. The specific examples given below of non-degradative effects by different linkages and E3 ligase, as well as removal of ubiquitin modifications, are meant to draw attention to potential obstacles and opportunities in continued development of PROTACs as therapeutics targeting protein kinases.
6.1. E3 ligase binding can inhibit, rather than promote, polyubiquitylation and degradation
The E3 ligase SMURF1, a component of a negative feedback loop that leads to degradation of ALK receptor kinases (cf. 5.3), conversely facilitates estrogen receptor alpha signaling by increasing the stability of ER-alpha, hypothetically due to inhibition of K48-specific poly-ubiquitylation [Citation107]. Conceivably, therefore, endogenous downregulation of kinases could be blocked by exogenous recruitment of E3 ligases into unproductive complexes by candidate PROTAC compounds. For a promiscuously binding inhibitor moiety, a linked E3 ligase could simultaneously degrade a targeted protein while blocking the endogenous negative regulation of another, representing a new category of off-target toxicity unique to PROTAC inhibitors.
6.2. Non-degradative regulation of the TGF-β signaling pathway: positive and negative
The transforming growth factor-β (TGF-β) signaling pathway, which utilizes a separate subset of downstream effectors (SMADs 2 and 3) than the BMP counterpart (SMADs 1, 5 and 8), is both positively and negatively regulated by non-degradative mechanisms through modulation of the regulated (TβRI/ALK5, TGF-β type I/activin receptor-like kinase 5) receptor (EC 2.7.11.30), the regulated effectors (R-SMADs) and the inhibitory effectors (I-SMADs) [Citation108–Citation110]. Itch (or Itchy), an E3 ubiquitin ligase involved in the modulation of immune responses, catalyzes non-degradative, TGF-β-induced ubiquitylation of SMAD2, which in turn enhances protein–protein interactions between TβRI receptor kinase and the SMAD substrate, and concomitantly, TGF-β-induced transcription. Conversely, in keeping with the caveat posed above about non-productive binding of E3 ligases and differential effects, the Itch protein inhibits TGF-β signaling by binding SMAD7 and enhancing the association of the I-SMAD with activated TβRI receptor kinase [Citation111]. Though similar with respect to down-regulation of the signaling pathway as for the Smurf1/2 E3 ligases discussed above (cf. 5.3), inhibition by Itch was not dependent on any form of ubiquitylation, simply binding, whereas the SMURF1/2 E3 ligase induced the degradation of activated ALK receptor kinase by polyubiquitylation.
6.3. Ubiquitylation appears to allosterically alter structure and dynamics of protein kinases
Because covalently linking proteins with ubiquitin was observed to be non-degradative in different contexts, the post-translational modifications were hypothesized to perhaps alter the structure and function of proteins much like phosphorylation or glycosylation [Citation112]. With proteomic methods, Jacobsen and coworkers identified ubiquitin-tagged protein kinases in a human cell down to the sites of ubiquitylation, which were commonly in structured regions involved in regulation and activity. Subsequent molecular dynamics simulations focused on one enzyme, ZAP-70 (70 kDa zeta-chain associated protein; a non-specific protein-tyrosine kinase, EC 2.7.10.2), indicated that ubiquitin modifications affect the structure of the protein and have site-specific effects. For example, at K377, modification induced structural changes that resembled the inactive state, whereas at K476, a site on the opposite side of the protein had the inverse effect. Thus, non-degradative ubiquitylation of protein kinases, similar to site-specific phosphorylation, might allosterically alter structure and dynamics and therefore activity and function of the conformationally regulated family of enzymes.
6.4. Polyubiquitylation through linkages on Ub K63 can induce activation of protein kinases
The polymeric structure of the polyubiquitin chain dictates whether or not the tagged protein is targeted for proteasomal degradation. Linkages from the sidechain ε-amino of ubiquitin K48 typically promote proteasomal degradation. In contrast, K63-linked polyubiquitylation does not trigger proteasomal inhibition but in fact, like mono-ubiquitylation, has been found to contribute to protein–protein interfaces to enhance associations and formations of complexes. Activation of protein kinases and trafficking of proteins is two cellular processes regulated by K63-linked non-degradative polyubiquitylation, which can influence cell survival and cancer development [Citation113].
TANK-binding kinase (TBK1), successfully targeted by a PROTAC for proteasomal degradation (cf. 4.2), becomes modified by non-degradative K63-linkages at two sites, one in the kinase domain (KD, Lys30) and the other in the scaffold/dimerization domain (SDD, Lys401). These post-translational modifications are required for the formation of dimers, which are necessary for activation of TBK1; hence, serve crucial roles [Citation114].
A specific E3 ubiquitin ligase, TRAF6 (tumor necrosis factor-associated protein 6), has been shown to regulate several protein kinases via polyubiquitylation with K63 linkages. TβRI, the type I TGF-β receptor kinase interacts with TRAF6, which polyubiquitylates TAK1 (transforming growth factor-β kinase 1 or mitogen-activated protein kinases kinase kinase, MAP3K7) leading to activation, thus without the receptor kinase acting directly on TAK1 [Citation115]. The structural basis for the activating effects of the K63-polyubiquitin chain could be due to alteration of protein–protein interactions with components of the pathway, and/or as a result of changes in structure and dynamics.
In addition to TAK1, the E3 ligase TRAF6 also has been shown to promote the activation of AKT/PKB (protein kinase B) through polyubiquitylation with K63 linkages [Citation116]. In this case, the mechanism is clearer, though indirect. Following growth factor stimulation, AKT/PKB, primarily localized in the cytosol, is actively recruited to the plasma membrane as a result of K63-chain polyubiquitylation by TRAF6. Interestingly, a mutation of AKT that leads to an increase in polyubiquitylation, membrane localization, and phosphorylation was found to be associated with human cancers (breast, ovarian, colon and bladder carcinomas, malignant melanoma), demonstrating an important role for the K63-linked polymer in oncogenic AKT activation [Citation113,Citation117].
Recently, adding to the complexity of the so-called ubiquitin code of multiple types, sites, and mixtures of polymers, proteomic studies revealed that branched forms are common in mammalian cells. The E3 ligases TRAF6 and HUWE1 (HECT-type ubiquitin ligase HECT, UBA and WWE Domain Containing 1) work in concert to create branches at K48 and K63, and importantly, though K48 linkages are sufficient for proteasomal degradation, K48 branching in K63 chains serves to enhance NF-kB signaling by stabilizing the downstream cascade of the pathway [Citation118,Citation119].
6.5. Different ubiquitylation modes and protein fates dictated by specific E2:E3 ligase pairs
Although this review has necessarily focused on the E3 ubiquitin ligases, which are recruited to targets of degradation by PROTACs, the role and influence of E2 ligases (EC 2.3.2.23) should not be ignored. Tethering of an ubiquitin-conjugating E2 enzyme to a substrate in the absence of an E3 is sufficient for transfer of the activated ubiquitin protein [Citation120]. However, specific sites of ubiquitylation are determined by an E3 ligase in complex with the E2 and substrate protein by positioning the E2 active site near the long flexible sidechain of a lysine residue. From a catalytic standpoint, ubiquitylation results from the nucleophilic attack of the terminal ε-amino group of the lysine on the thioester bond linking the activated ubiquitin group to the E2 catalytic cysteine. Consequently, a particular E3 ligase will dictate which lysine is covalently tagged and the type (mono- or polyubiquitinylated, branched or unbranched) but must act in complex with a specific E2 for catalytic transfer. Thus, E3 ligases recruited by PROTACs for degradation of target proteins should be considered more as specific E2:E3 ligase pairs, rather than autonomous actors in the ubiquitin-proteasomal system [Citation121].
6.6. Ubiquitylation of proteins is reversible in vivo: de-ubiquitinating enzymes (DUBs)
As a final consideration or caveat, along with the focus on PROTAC-induced degradation through polyubiquitylation of target proteins, a counter-acting cellular process should be kept in mind, i.e. de-ubiquitination of proteins by a family of de-ubiquitinating enzymes or DUBs (ubiquitinyl hydrolases or thiolesterases, EC 3.4.19.12). Much like the albeit relatively smaller family of phosphatases employed by the cell to reverse effects of protein kinase phosphorylation of regulated proteins, the DUBs are a smaller family relative to the E3 ligases and serve a similar function, i.e. reverse the effects of covalent modification with a small protein or polymers thereof. In fact, TGF-β family signaling pathways have been shown to be controlled by de-ubiquitination by DUBs [Citation109,Citation122].
7. Conclusion
Induced protein degradation is an event-driven means of elimination of targets, in contrast to simple and conventional inhibition through occupancy-driven mechanisms. The clinical potential of induced protein degradation mediated by small molecules has been clearly established by two drugs (fulvestrant, a SERD and lenalidomide, an IMiD) that were not designed as such but subsequently found to act through degradative mechanisms (cf. Introduction). Two inductive avenues have been observed to lead to proteasomal degradation of target proteins: chaperone-mediated (cf. 2.1–2.4) and by enzyme-catalyzed polyubiquitylation. The latter has been the focus of drug discovery in recent years, in particular, the modular design of PROTACs, which hijack E3 ubiquitin ligases to modify specific targets and induce proteasomal degradation (cf. 3.1–3.3). A roster of examples presented within (cf. 4.1–4.10) demonstrate the applicability of the chimeric inducers for targeting the large and clinically important family of protein kinases. Beyond these paradigms, a case was made for seeing protein kinases not as isolated entities, but as components of multi-protein complexes which offer additional sites for E3 ligase binding and modification of lysine residues (cf. 5.1–5.4). Finally, because this review has focused on the degradation of proteins mediated by polyubiquitylation, a caveat was made that modification with ubiquitin-protein moieties can have a multitude of non-degradative effects which should be kept in mind, such as inhibition of degradation and activation of protein kinases (cf. 6.1–6.6).
8. Expert opinion
In marked contrast to conventional occupancy-based inhibitors, therapeutics that act through an event-driven mechanism offer significant advantages, primarily with respect to dynamics, since steady-state levels of inhibitors need not be maintained. Of the avenues identified to date (cf. ), inducers of protein degradation appear to be the most desirable and have already been proven in the clinic, albeit in a limited number of cases reflecting the relative infancy of the field. Molecular-genetic modification of nucleic acids, though powerful, is only recently showing true promise and might reach the clinic well into the future, if at all. That is to say, gene-silencing of RNAs has only recently emerged as efficacious due to barriers to the introduction, despite the initial promise and excitement following the award of a Nobel prize for the ground-breaking studies. In a similar vein, gene-editing methods such as CRISPR-Cas9 (Cas9: CRISPR-associated nuclease 9, a translocase of a new class of EC 7 hydrolases) remain plagued by off-target effects, which though likely to be resolved, might be much less a concern than the ethical barriers which ultimately could keep the method out of clinics and restricted to a laboratory research tool. With respect to protein targets, covalent inhibitors (active and allosteric site) offer the benefits of event-driven therapeutics, are surprisingly non-toxic and commercially successful (aspirin, penicillin). The major barrier to the widespread application of this class is the requirement for a specific nucleophilic group at the binding site to react with an electrophilic warhead on the drug. Thus, small-molecule inducers of protein degradation, in particular, PROTACs, have emerged as the leading candidates from the wide repertoire of event-driven therapeutics currently on hand.
In the case of protein kinases, targeting with PROTACs offers even more than the pharmacodynamic benefits, in that an additional, often times much-needed layer of specificity can be imparted by the E3 ligase interactions with the substrate protein or complex. Moreover, substantially less effort might be needed to generate preclinical leads for further development. That is, even target-selective inhibitors with modest affinity and specificity would suffice when paired with E3 ligase interactions and limited lysine substrate accessibility. As a result, drug discovery might prove dramatically less computationally intensive for rational design avenues, or require less extensive screening of compound libraries. Since both require a great deal of expertise and resources, typically limited to well-staffed and well-funded groups in industry or academia, with the growing implementation of PROTAC design and synthesis, the entry level into drug development would likely be lowered, allowing more and more laboratories to productively engage in translational projects that can contribute to the volume of the flow of candidates in or into commercial pipelines and/or clinical trials. In keeping with the expected growth in adoption of the methodology by laboratories with modest resources, modular components for the synthesis of PROTACs as well as promiscuous test compounds have recently become commercially available (Tocris, R&D Systems; Life Sensors).
Though much remains to be explored and many hurdles need to be overcome such as enhanced permeability of the relatively large chimeras, characterization of other E3 ligases and screening for corresponding bait ligands [Citation1], development of occupancy-based protein kinase inhibitors is anticipated to continue to shift toward protein degraders over the next 5 years, as evidenced by the last. With the substantial investments by major pharmaceutical companies in inducers of protein degradation in general (cf. Introduction), due to the long-standing interest in protein kinases throughout the industry and the encouraging proofs-of-concept presented above (cf. 4.1–4.10), a dramatic increase in safe and efficacious therapeutics targeting this large and clinically important family of enzymes is expected to reach the clinic to treat a wide range of pathologies, not the least of which are the many protein kinase-linked cancers.
Article highlights
Induced degradation of proteins by novel small molecules is an unconventional approach for down-regulation of targets offering significant advantages over occupancy-driven inhibition.
Arising from initial unanticipated effects two decades ago, the field has evolved to current structure-based, rational designs of modular small-molecule degraders of specific targets.
Proteolysis-targeting chimeras, or PROTACs, are optimized hetero-bifunctional inducers of protein degradation that are rapidly emerging as a next-generation strategy or drug modality.
Examples are given of PROTACs that have been produced to target protein kinases; the first bona fide composition was developed by the Crews laboratory at Yale and published in 2015.
Because protein kinases, in particular, those involved in signaling, form complexes, a case is made for PROTACs directed to a Ser-Thr receptor kinase (Activin receptor-like kinase 2) that forms a hetero-tetrameric complex, which affords manifold opportunities for quenching dysregulated signaling by mutant ALK2, an aim of at least six pharmaceutical companies.
Though proof-of-concept has been established for the application of the PROTACs methodology to protein kinase targets, technological hurdles remain to be surmounted, and each case appears to require a systematic, empirical design approach, hence possibly labor-intensive.
Nevertheless, given that the field has only recently come of age and shown such significant promise, and that the large and important family of protein kinases has remained relatively untapped with respect to drugs reaching the clinic, induced degradation by novel chimeras is expected to become a next-generation approach to be vigorously pursued in drug discovery.
This box summarizes key points contained in the article.
Declaration of interest
J Groppe has no other relevant affiliations or financial involvement with any organization or entity with a financial interest in or financial conflict with the subject matter or materials discussed in the manuscript apart from those disclosed.
Reviewer disclosures
Peer reviewers on this manuscript have no relevant financial or other relationships to disclose.
Additional information
Funding
References
- Müller MP, Rauh D. Try me: promiscuous inhibitors still allow for selective targeted protein degradation. Cell Chem Biol. 2018;25(1):4–6.
- Cromm PM, Crews CM. Targeted protein degradation: from chemical biology to drug discovery. Cell Chem Biol. 2017 Sep 21;24(9):1181–1190.
- Kronke J, Fink EC, Hollenbach PW, et al. Lenalidomide induces ubiquitination and degradation of CK1alpha in del(5q) MDS. Nature. 2015 Jul 9;523(7559):183–188.
- Lebraud H, Heightman TD. Protein degradation: a validated therapeutic strategy with exciting prospects. Essays Biochem. 2017 Nov 8;61(5):517–527.
- An S, Fu L. Small-molecule PROTACs: an emerging and promising approach for the development of targeted therapy drugs. EBioMedicine. 2018 Oct;36:553–562.
- Phillippidis A. Pfizer joins yale spinout in up-to-$830 protein degradation drug collaboration. Genet Eng Biotechnol News. 2018. Available from: https://www.genengnews.com/news/pfizer-joins-yale-spinout-in-up-to-830m-protein-degradation-drug-collaboration/
- Mori T, Ito T, Liu S, et al. Structural basis of thalidomide enantiomer binding to cereblon. Sci Rep. 2018 Jan 22;8(1):1294.
- Petzold G, Fischer ES, Thoma NH. Structural basis of lenalidomide-induced CK1alpha degradation by the CRL4(CRBN) ubiquitin ligase. Nature. 2016 Apr 7;532(7597):127–130.
- Huang X, Dixit VM. Drugging the undruggables: exploring the ubiquitin system for drug development. Cell Res. 2016 Apr;26(4):484–498.
- Phillippidis A. Top 10 best-selling cancer drugs, Q1–Q3 2017. Genet Eng Biotechnol News. 2018. Available from: https://www.genengnews.com/a-lists/top-10-best-selling-cancer-drugs-q1-q3-2017/
- Bondeson DP, Crews CM. Targeted protein degradation by small molecules. Annu Rev Pharmacol Toxicol. 2017 Jan;6(57):107–123.
- Churcher I. Protac-induced protein degradation in drug discovery: breaking the rules or just making new ones? J Med Chem. 2018 Jan 25;61(2):444–452. doi:https://doi.org/10.1021/acs.jmedchem.7b01272.
- Neklesa TK, Winkler JD, Crews CM. Targeted protein degradation by PROTACs. Pharmacol Ther. 2017 Jun;174:138–144.
- Ottis P, Crews CM. Proteolysis-targeting chimeras: induced protein degradation as a therapeutic strategy. ACS Chem Biol. 2017 Apr 21;12(4):892–898.
- Raina K, Crews CM. Targeted protein knockdown using small molecule degraders. Curr Opin Chem Biol. 2017 Aug;39:46–53.
- Salami J, Crews CM. Waste disposal-an attractive strategy for cancer therapy. Science. 2017 Mar 17;355(6330):1163–1167.
- Fry DW, Bridges AJ, Denny WA, et al. Specific, irreversible inactivation of the epidermal growth factor receptor and erbB2, by a new class of tyrosine kinase inhibitor. Proc Natl Acad Sci U S A. 1998 Sep 29;95(20):12022–12027.
- Neckers L, Schulte TW, Mimnaugh E. Geldanamycin as a potential anti-cancer agent: its molecular target and biochemical activity. Invest New Drugs. 1999;17(4):361–373.
- Huezo H, Vilenchik M, Rosen N, et al. Microtiter cell-based assay for detection of agents that alter cellular levels of Her2 and EGFR. Chem Biol. 2003 Jul;10(7):629–634.
- Citri A, Alroy I, Lavi S, et al. Drug-induced ubiquitylation and degradation of ErbB receptor tyrosine kinases: implications for cancer therapy. Embo J. 2002 May 15;21(10):2407–2417.
- Chiosis G, Lucas B, Huezo H, et al. Development of purine-scaffold small molecule inhibitors of Hsp90. Curr Cancer Drug Targets. 2003 Oct;3(5):371–376.
- Vilenchik M, Solit D, Basso A, et al. Targeting wide-range oncogenic transformation via PU24FCl, a specific inhibitor of tumor Hsp90. Chem Biol. 2004 Jun;11(6):787–797.
- Chiosis G, Lucas B, Shtil A, et al. Development of a purine-scaffold novel class of Hsp90 binders that inhibit the proliferation of cancer cells and induce the degradation of Her2 tyrosine kinase. Bioorg Med Chem. 2002 Nov;10(11):3555–3564.
- Chiosis G, Timaul MN, Lucas B, et al. A small molecule designed to bind to the adenine nucleotide pocket of Hsp90 causes Her2 degradation and the growth arrest and differentiation of breast cancer cells. Chem Biol. 2001 Mar;8(3):289–299.
- Marcu MG, Schulte TW, Neckers L. Novobiocin and related coumarins and depletion of heat shock protein 90-dependent signaling proteins. J Natl Cancer Inst. 2000 Feb 2;92(3):242–248.
- Schulte TW, Akinaga S, Soga S, et al. Antibiotic radicicol binds to the N-terminal domain of Hsp90 and shares important biologic activities with geldanamycin. Cell Stress Chaperones. 1998 Jun;3(2):100–108.
- Schulte TW, Neckers LM. The benzoquinone ansamycin 17-allylamino-17-demethoxygeldanamycin binds to HSP90 and shares important biologic activities with geldanamycin. Cancer Chemother Pharmacol. 1998;42(4):273–279.
- Yu X, Guo ZS, Marcu MG, et al. Modulation of p53, ErbB1, ErbB2, and Raf-1 expression in lung cancer cells by depsipeptide FR901228. J Natl Cancer Inst. 2002 Apr 3;94(7):504–513.
- Taipale M, Jarosz DF, Lindquist S. HSP90 at the hub of protein homeostasis: emerging mechanistic insights. Nat Rev Mol Cell Biol. 2010 Jul;11(7):515–528.
- Whitesell L, Lindquist SL. HSP90 and the chaperoning of cancer. Nat Rev Cancer. 2005 Oct;5(10):761–772.
- Trepel J, Mollapour M, Giaccone G, et al. Targeting the dynamic HSP90 complex in cancer. Nat Rev Cancer. 2010 Aug;10(8):537–549.
- Radli M, Rudiger SGD. Dancing with the diva: Hsp90-client interactions. J Mol Biol. 2018 Sep 14;430(18 Pt B):3029–3040.
- Wang L, Li L, Gu K, et al. Targeting Hsp90-Cdc37: a promising therapeutic strategy by inhibiting Hsp90 chaperone function. Curr Drug Targets. 2017;18(13):1572–1585.
- McDonnell DP, Wardell SE. The molecular mechanisms underlying the pharmacological actions of ER modulators: implications for new drug discovery in breast cancer. Curr Opin Pharmacol. 2010 Dec;10(6):620–628.
- McDonnell DP, Wardell SE, Norris JD. Oral selective estrogen receptor downregulators (serds), a breakthrough endocrine therapy for breast cancer. J Med Chem. 2015 Jun 25;58(12):4883–4887.
- Wu YL, Yang X, Ren Z, et al. Structural basis for an unexpected mode of SERM-mediated ER antagonism. Mol Cell. 2005 May 13;18(4):413–424.
- Guan J, Zhou W, Hafner M, et al. Therapeutic ligands antagonize estrogen receptor function by impairing its mobility. Cell. 2019 Aug 8;178(4):949–63 e18.
- Neklesa TK, Tae HS, Schneekloth AR, et al. Small-molecule hydrophobic tagging-induced degradation of HaloTag fusion proteins. Nat Chem Biol. 2011 Jul 3;7(8):538–543.
- Gustafson JL, Neklesa TK, Cox CS, et al. Small-molecule-mediated degradation of the androgen receptor through hydrophobic tagging. Angew Chem Int Ed Engl. 2015 Aug 10;54(33):9659–9662.
- Tae HS, Sundberg TB, Neklesa TK, et al. Identification of hydrophobic tags for the degradation of stabilized proteins. Chembiochem. 2012 Mar 5;13(4):538–541.
- Xie T, Lim SM, Westover KD, et al. Pharmacological targeting of the pseudokinase Her3. Nat Chem Biol. 2014 Dec;10(12):1006–1012.
- Lu G, Tandang-Silvas MR, Dawson AC, et al. Hypoxia-selective allosteric destabilization of activin receptor-like kinases: A potential therapeutic avenue for prophylaxis of heterotopic ossification. Bone. 2018 Jul;112:71–89.
- Huang H-T, Dobrovolsky D, Paulk J, et al. A chemoproteomic approach to query the degradable kinome using a multi-kinase degrader. Cell Chem Biol. 2018 Jan 18;25(1):88–99.e6.
- Tomoshige S, Nomura S, Ohgane K, et al. Discovery of small molecules that induce the degradation of huntingtin. Angew Chem Int Ed Engl. 2017 Sep 11;56(38):11530–11533.
- Jones LH. Small-molecule kinase downregulators. Cell Chem Biol. 2018 Jan 18;25(1):30–35. doi:https://doi.org/10.1016/j.chembiol.2017.10.011. Epub 2017 Nov 22.
- Lai AC, Crews CM. Induced protein degradation: an emerging drug discovery paradigm. Nat Rev Drug Discov. 2017 Feb;16(2):101–114.
- Bondeson DP, Smith BE, Burslem GM, et al. Lessons in PROTAC design from selective degradation with a promiscuous warhead. Cell Chem Biol. 2018 Jan 18;25(1):78–87.e5.
- Hughes SJ, Ciulli A. Molecular recognition of ternary complexes: a new dimension in the structure-guided design of chemical degraders. Essays Biochem. 2017 Nov 8;61(5):505–516.
- Burslem GM, Smith BE, Lai AC, et al. The advantages of targeted protein degradation over inhibition: an RTK case study. Cell Chem Biol. 2018 Jan 18;25(1):67–77.e3.
- Gadd MS, Testa A, Lucas X, et al. Structural basis of PROTAC cooperative recognition for selective protein degradation. Nat Chem Biol. 2017 May;13(5):514–521.
- Shibata N, Nagai K, Morita Y, et al. Development of protein degradation inducers of androgen receptor by conjugation of androgen receptor ligands and inhibitor of apoptosis protein ligands. J Med Chem. 2018 Jan 25;61(2):543–575.
- Ohoka N, Morita Y, Nagai K, et al. Derivatization of inhibitor of apoptosis protein (IAP) ligands yields improved inducers of estrogen receptor alpha degradation. J Biol Chem. 2018 May 4;293(18):6776–6790.
- Ohoka N, Ujikawa O, Shimokawa K, et al. Different degradation mechanisms of Inhibitor of Apoptosis Proteins (IAPs) by the Specific and Nongenetic IAP-Dependent Protein Eraser (SNIPER). Chem Pharm Bull (Tokyo). 2019 Mar 1;67(3):203–209.
- Hines J, Lartigue S, Dong H, et al. MDM2-recruiting PROTAC offers superior, synergistic antiproliferative activity via simultaneous degradation of BRD4 and stabilization of p53. Cancer Res. 2019 Jan 1;79(1):251–262.
- Salami J, Alabi S, Willard RR, et al. Androgen receptor degradation by the proteolysis-targeting chimera ARCC-4 outperforms enzalutamide in cellular models of prostate cancer drug resistance. Commun Biol. 2018;1:100.
- Tan L, Gray NS. When kinases meet PROTACs. Chin J Chem. 2018;36(10): 971–977.
- Ferguson FM, Gray NS. Kinase inhibitors: the road ahead. Nat Rev Drug Discov. 2018 May;17(5):353–377.
- Bondeson DP, Mares A, Smith IE, et al. Catalytic in vivo protein knockdown by small-molecule PROTACs. Nat Chem Biol. 2015 Aug;11(8):611–617.
- Buckley DL, Van Molle I, Gareiss PC, et al. Targeting the von hippel-lindau E3 ubiquitin ligase using small molecules to disrupt the VHL/HIF-1alpha interaction. J Am Chem Soc. 2012 Mar 14;134(10):4465–4468.
- Van Molle I, Thomann A, Buckley DL, et al. Dissecting fragment-based lead discovery at the von hippel-lindau protein: hypoxiainducible factor 1alpha protein-protein interface. Chem Biol. 2012 Oct 26;19(10):1300–1312.
- Galdeano C, Gadd MS, Soares P, et al. Structure-guided design and optimization of small molecules targeting the protein-protein interaction between the von hippel-lindau (VHL) E3 ubiquitin ligase and the hypoxia inducible factor (HIF) alpha subunit with in vitro nanomolar affinities. J Med Chem. 2014 Oct 23;57(20):8657–8663.
- Chariot A, Leonardi A, Muller J, et al. Association of the adaptor TANK with the I kappa B kinase (IKK) regulator NEMO connects IKK complexes with IKK epsilon and TBK1 kinases. J Biol Chem. 2002 Oct 4;277(40):37029–37036.
- Pomerantz JL, Baltimore D. NF-kappaB activation by a signaling complex containing TRAF2, TANK and TBK1, a novel IKK-related kinase. Embo J. 1999 Dec 1;18(23):6694–6704.
- Demizu Y, Shibata N, Hattori T, et al. Development of BCR-ABL degradation inducers via the conjugation of an imatinib derivative and a cIAP1 ligand. Bioorg Med Chem Lett. 2016 Oct 15;26(20):4865–4869.
- Shibata N, Miyamoto N, Nagai K, et al. Development of protein degradation inducers of oncogenic BCR-ABL protein by conjugation of ABL kinase inhibitors and IAP ligands. Cancer Sci. 2017 Aug;108(8):1657–1666.
- Lai AC, Toure M, Hellerschmied D, et al. Modular PROTAC design for the degradation of oncogenic BCR-ABL. Angew Chem Int Ed Engl. 2016 Jan 11;55(2):807–810.
- Shibata N, Shimokawa K, Nagai K, et al. Pharmacological difference between degrader and inhibitor against oncogenic BCR-ABL kinase. Sci Rep. 2018 Sep 10;8(1):13549.
- de Azevedo WF Jr., Canduri F, Da Silveira NJ. Structural basis for inhibition of cyclin-dependent kinase 9 by flavopiridol. Biochem Biophys Res Commun. 2002 Apr 26;293(1):566–571.
- Canduri F, Perez PC, Caceres RA, et al. CDK9 a potential target for drug development. Med Chem. 2008 May;4(3):210–218.
- Eyvazi S, Hejazi MS, Kahroba H, et al. CDK9 as an appealing target for therapeutic interventions. Curr Drug Targets. 2019;20(4):453–464.
- Olson CM, Jiang B, Erb MA, et al. Pharmacological perturbation of CDK9 using selective CDK9 inhibition or degradation. Nat Chem Biol. 2018 Feb;14(2):163–170.
- Zorba A, Nguyen C, Xu Y, et al. Delineating the role of cooperativity in the design of potent PROTACs for BTK. Proc Natl Acad Sci U S A. 2018 Jul 31;115(31):E7285–E92.
- Golubovskaya VM. Targeting FAK in human cancer: from finding to first clinical trials. Front Biosci (Landmark Ed). 2014 Jan 1;19:687–706.
- Cromm PM, Samarasinghe K, Hines J, et al. Addressing kinase-independent functions of Fak via PROTAC-mediated Degradation. J Am Chem Soc. 2018 Nov 16.
- Roskoski R Jr. Targeting oncogenic Raf protein-serine/threonine kinases in human cancers. Pharmacol Res. 2018 Sep;135:239–258.
- Lebraud H, Wright DJ, Johnson CN, et al. Protein degradation by in-cell self-assembly of proteolysis targeting chimeras. ACS Cent Sci. 2016 Dec 28;2(12):927–934.
- Smith BE, Wang SL, Jaime-Figueroa S, et al. Differential PROTAC substrate specificity dictated by orientation of recruited E3 ligase. Nat Commun. 2019 Jan 10;10(1):131.
- Shimono K, Tung WE, Macolino C, et al. Potent inhibition of heterotopic ossification by nuclear retinoic acid receptor-gamma agonists. Nat Med. 2011 Apr;17(4):454–460.
- Lees-Shepard JB, Nicholas SE, Stoessel SJ, et al. Palovarotene reduces heterotopic ossification in juvenile FOP mice but exhibits pronounced skeletal toxicity. Elife. 2018;18:7.
- Sheng N, Xie Z, Wang C, et al. Retinoic acid regulates bone morphogenic protein signal duration by promoting the degradation of phosphorylated Smad1. Proc Natl Acad Sci U S A. 2010 Nov 2;107(44):18886–18891.
- Agarwal S, Loder SJ, Breuler C, et al. Strategic targeting of multiple BMP receptors prevents trauma-induced heterotopic ossification. Mol Ther. 2017 Aug 2;25(8):1974–1987.
- Dey D, Wheatley BM, Cholok D, et al. The traumatic bone: trauma-induced heterotopic ossification. Transl Res. 2017;186:95–111.
- Zhu H, Kavsak P, Abdollah S, et al. A SMAD ubiquitin ligase targets the BMP pathway and affects embryonic pattern formation. Nature. 1999 Aug 12;400(6745):687–693.
- Yan X, Liu Z, Chen Y. Regulation of TGF-β signaling by Smad7. Acta Biochim Biophys Sin (Shanghai). 2009 Apr;41(4):263–272.
- Kavsak P, Rasmussen RK, Causing CG, et al. Smad7 binds to Smurf2 to form an E3 ubiquitin ligase that targets the TGF beta receptor for degradation. Mol Cell. 2000 Dec;6(6):1365–1375.
- Ebisawa T, Fukuchi M, Murakami G, et al. Smurf1 interacts with transforming growth factor-beta type I receptor through Smad7 and induces receptor degradation. J Biol Chem. 2001 Apr 20;276(16):12477–12480.
- Wang X, Feng S, Fan J, et al. New strategy for renal fibrosis: targeting Smad3 proteins for ubiquitination and degradation. Biochem Pharmacol. 2016 Sep 15;116:200–209.
- Winter GE, Buckley DL, Paulk J, et al. Phthalimide conjugation as a strategy for in vivo target protein degradation. Science. 2015;348(6241):1376–1381.
- Kugimiya F, Yano F, Ohba S, et al. Mechanism of osteogenic induction by FK506 via BMP/Smad pathways. Biochem Biophys Res Commun. 2005 Dec 16;338(2):872–879.
- Cao Y, Wang C, Zhang X, et al. Selective small molecule compounds increase BMP-2 responsiveness by inhibiting Smurf1-mediated Smad1/5 degradation. Sci Rep. 2014 May 14;4:4965.
- Zhang Y, Wang C, Cao Y, et al. Selective compounds enhance osteoblastic activity by targeting HECT domain of ubiquitin ligase Smurf1. Oncotarget. 2017 Aug 1;8(31):50521–50533.
- Piacentino ML, Bronner ME. Intracellular attenuation of BMP signaling via CKIP-1/Smurf1 is essential during neural crest induction. PLoS Biol. 2018 Jun;16(6):e2004425.
- Li H, Cui Y, Wei J, et al. VCP/p97 increases BMP signaling by accelerating ubiquitin ligase Smurf1 degradation. Faseb J. 2019 Feb;33(2):2928–2943.
- Bagarova J, Vonner AJ, Armstrong KA, et al. Constitutively active ALK2 receptor mutants require type II receptor cooperation. Mol Cell Biol. 2013 Jun;33(12):2413–2424.
- Arora K, Warrior R. A new Smurf in the village. Dev Cell. 2001 Oct;1(4):441–442.
- Lin H, Ying Y, Wang YY, et al. AMPK downregulates ALK2 via increasing the interaction between Smurf1 and Smad6, leading to inhibition of osteogenic differentiation. Biochim Biophys Acta. 2017 Dec;1864(12):2369–2377.
- Groppe J, Hinck CS, Samavarchi-Tehrani P, et al. Cooperative assembly of TGF-β superfamily signaling complexes is mediated by two disparate mechanisms and distinct modes of receptor binding. Mol Cell. 2008 Feb 01;29(2):157–168.
- Greenwald J, Groppe J, Gray P, et al. The BMP7/ActRII extracellular domain complex provides new insights into the cooperative nature of receptor assembly. Mol Cell. 2003 Mar;11(3):605–617.
- Townson SA, Martinez-Hackert E, Greppi C, et al. Specificity and structure of a high affinity activin receptor-like kinase 1 (ALK1) signaling complex. J Biol Chem. 2012 Aug 10;287(33):27313–27325.
- Hart PJ, Deep S, Taylor AB, et al. Crystal structure of the human TβR2 ectodomain–TGF-β3 complex. Nat Struct Biol. 2002 Mar;9(3):203–208.
- Macias MJ, Martin-Malpartida P, Massague J. Structural determinants of smad function in TGF-β signaling. Trends Biochem Sci. 2015 Jun;40(6):296–308.
- Shi Y, Massague J. Mechanisms of TGF-β signaling from cell membrane to the nucleus. Cell. 2003 Jun 13;113(6):685–700.
- Nohe A, Hassel S, Ehrlich M, et al. The mode of bone morphogenetic protein (BMP) receptor oligomerization determines different BMP-2 signaling pathways. J Biol Chem. 2002 Feb 15;277(7):5330–5338.
- Kozakov D, Hall DR, Xia B, et al. The cluspro web server for protein-protein docking. Nat Protoc. 2017 Feb;12(2):255–278.
- Zhao G, Wu Y, Du L, et al. Drosophila S6 kinase like inhibits neuromuscular junction growth by downregulating the BMP receptor thickveins. PLoS Genet. 2015 Mar;11(3):e1004984.
- Kim BG, Lee JH, Yasuda J, et al. Phospho-Smad1 modulation by nedd4 E3 ligase in BMP/TGF-β signaling. J Bone Miner Res. 2011 Jul;26(7):1411–1424.
- Yang H, Yu N, Xu J, et al. SMURF1 facilitates estrogen receptor a signaling in breast cancer cells. J Exp Clin Cancer Res. 2018 Feb 12;37(1):24.
- Tang LY, Zhang YE. Non-degradative ubiquitination in smad-dependent TGF-β signaling. Cell Biosci. 2011 Dec 28;1(1):43.
- Imamura T, Oshima Y, Hikita A. Regulation of TGF-β family signalling by ubiquitination and deubiquitination. J Biochem. 2013 Dec;154(6):481–489.
- Inoue Y, Imamura T. Regulation of TGF-β family signaling by E3 ubiquitin ligases. Cancer Sci. 2008 Nov;99(11):2107–2112.
- Lallemand F, Seo SR, Ferrand N, et al. AIP4 restricts transforming growth factor-beta signaling through a ubiquitination-independent mechanism. J Biol Chem. 2005 Jul 29;280(30):27645–27653.
- Ball KA, Johnson JR, Lewinski MK, et al. Non-degradative ubiquitination of protein kinases. PLoS Comput Biol. 2016 Jun;12(6):e1004898.
- Wang G, Gao Y, Li L, et al. K63-linked ubiquitination in kinase activation and cancer. Front Oncol. 2012;2:5.
- Tu D, Zhu Z, Zhou AY, et al. Structure and ubiquitination-dependent activation of TANK-binding kinase 1. Cell Rep. 2013 Mar 28;3(3):747–758.
- Sorrentino A, Thakur N, Grimsby S, et al. The type I TGF-β receptor engages TRAF6 to activate TAK1 in a receptor kinase-independent manner. Nat Cell Biol. 2008 Oct;10(10):1199–1207.
- Yang WL, Wang J, Chan CH, et al. The E3 ligase TRAF6 regulates Akt ubiquitination and activation. Science. 2009 Aug 28;325(5944):1134–1138.
- Yang WL, Wu CY, Wu J, et al. Regulation of Akt signaling activation by ubiquitination. Cell Cycle. 2010 Feb 1;9(3):487–497.
- Ohtake F, Saeki Y, Ishido S, et al. The K48-K63 branched ubiquitin chain regulates NF-kappaB signaling. Mol Cell. 2016 Oct 20;64(2):251–266.
- Ohtake F, Tsuchiya H, Saeki Y, et al. K63 ubiquitylation triggers proteasomal degradation by seeding branched ubiquitin chains. Proc Natl Acad Sci U S A. 2018 Feb 13;115(7):E1401–E08.
- David Y, Ternette N, Edelmann MJ, et al. E3 ligases determine ubiquitination site and conjugate type by enforcing specificity on E2 enzymes. J Biol Chem. 2011 Dec 23;286(51):44104–44115.
- Sadowski M, Sarcevic B. Mechanisms of mono- and poly-ubiquitination: ubiquitination specificity depends on compatibility between the E2 catalytic core and amino acid residues proximal to the lysine. Cell Div. 2010 Aug 13;5:19.
- Guo YC, Zhang SW, Yuan Q. Deubiquitinating enzymes and bone remodeling. Stem Cells Int. 2018;2018:3712083.