ABSTRACT
This study is concerned with establishing a uniform experimental protocol for the determination of phenolic extractives, which play an essential role in the durability of the wood of selected species included in the field tests. European larch (Larix decidua), Norway spruce (Picea abies), Scots pine (Pinus sylvestris), European oak (Quercus sp.), sweet chestnut (Castanea sativa), and black locust (Robinia pseudoacacia) were included in the study. Extraction yield data were collected for extraction in a Soxhlet apparatus using common polar extraction solvents (ethanol, acetone, methanol, water) and for single static extraction cycles in accelerated solvent extraction (ASE). The suitability of the solvents and the appropriate extraction conditions were evaluated based on the results of gravimetric analysis of hydrophilic extractives, spectrophotometric analysis of total phenols, and chromatographic analysis using high-performance liquid chromatography. Extraction in the ASE system with methanol proved to be a quick and accurate way to prepare samples for chemical analysis of extractives of selected wood species. With the chemical monitoring of targeted phenolic extractives in wood subjected to leaching, we propose a novel protocol suitable also for for measuring the effects of weathering on the natural durability of wood in future studies.
1. Introduction
Wood, as a natural building material, is susceptible to weathering, significantly affecting its performance in outdoor conditions (Brischke et al. Citation2006). Standard EN 335 (CEN Citation2013) defines five possible use conditions of the wood. The use class 3 (above ground, uncovered, frequent wetting) is one of the most commercially important in terms of outdoor use application of wood. Typical applications in use-class three are cladding, decking and garden furniture. The critical degradation factors in respective use-class are mainly fluctuating moisture that results in cracking, temperature differences and biological factors such as moulds, blue stains, and wood-degrading fungi. How these factors alter the properties of wood depends not only on environmental factors and construction but also on its inherent durability (natural or improved) and water exclusion efficacy (Brischke et al. Citation2006, Meyer-Veltrup et al. Citation2017). An important factor affecting the natural durability of wood species is the presence or absence of biologically active substances, i.e. extractives (secondary metabolites) (Kirker et al. Citation2013, Brischke and Alfredsen Citation2022). These non-structural chemical constituents are mainly formed in heartwood as a result of tissue ageing or occur in response to environmental stress and are species-specific. The concentration of biologically active extractives is on average, much higher in heartwood than in sapwood (Taylor et al. Citation2002, Vek et al. Citation2014). Absence of biologically active extractives in sapwood results in high susceptibility of the sapwood of all wood species to degradation (CEN Citation2016). Extractives can affect the performance of wood-based materials by influencing decay, hydrophobicity, colour, and odour (Kai Citation1991, Oven et al. Citation2015, Vek et al. Citation2019). One group of compounds, polyphenols (e.g. stilbenes, lignans, flavonoids, and tannins), significantly influence durability (Eichhorn et al. Citation2017). The high variation of the extractives is one of the reasons for the high variability of the wood durability within the species (Oven et al. Citation2015). Some of these compounds have fungicidal activity, while others have good antioxidant properties (Scalbert Citation1991, Eichhorn et al. Citation2017, Vek et al. Citation2021). For example, Vek et al. (Citation2020) have shown that stilbene-containing Scots pine (Pinus sylvestris) extracts effectively inhibit fungal growth, while flavonoid-rich black locust (Robinia pseudoacacia) extracts are a potent free radical scavenger. In addition it was reported that extracts derived from black locust heartwood can increase the natural durability of European beech (Fagus sylvatica) from non-durable to moderately durable (Singh and Singh Citation2012, Sabik et al. Citation2016, Broda Citation2020).
In addition, solvent type influences the extraction yield. With water, we can extract most of the biologicaly active extractives, with the exception of resins at conifers (Oven et al. Citation2015). Unfortunately, most polyphenols are water-soluble and, therefore, leach from wood when exposed to rainfall (Baar et al. Citation2019, Keržič and Humar Citation2021). The leaching dynamics are significantly influenced by their microdistribution and the anatomical structure of the wood (Taylor et al. Citation2002, Aloui et al. Citation2004). Decomposition is also caused by UV spectra of light and a variety of biological factors (Williams Citation2005). Respective factors cause hydrolysis and other reactions that lead to changes in the composition of the extracts (Vek and Oven Citation2011). As a result, the durability decreases (Baar et al. Citation2019). In order to more accurately predict the service life of wood, it is of great interest to investigate the presence of extractives in wood exposed to weathering in above-ground applications. In addition, knowledge of the leaching of biologically active substances from wood is also critical for developing novel wood preservatives. Therefore, a comprehensive assessment of the extractives content in wood is of great scientific interest. Namely, sampling and the right choice of extraction technique, extraction conditions and further analyses are required.
The extraction yield depends on the extraction method, solvent polarity, extraction temperature and time (Fang et al. Citation2013). The most commonly used extraction methods are conventional Soxhlet extraction, sonication, and maceration (Vek et al. Citation2019). A suitable alternative to Soxhlet extraction is accelerated solvent extraction (ASE), which offers comparable or even better extraction yields, higher purity of extracted products, and greater sustainability (Pietarinen et al. Citation2006, Vek et al. Citation2014). ASE consists of several static extraction cycles in which the extraction cell is brought to the selected temperature and then filled with the solvent. This increases the pressure in the extraction cell. When the temperature and pressure are in equilibrium, a static extraction cycle occurs and lasts for a preset time. When the static extraction is complete, the valve opens, and the extract is poured into a collection bottle. The extraction cell is leached with solvent or blown out with inert gas (e.g. nitrogen) before and after extraction (Priego-Capote and Delgado de la Torre Citation2013). Since the liquid phase is used at high pressure and temperature but below a critical point, the processing time is shortened, and the amount of solvent required for extraction decreases (Priego-Capote and Delgado de la Torre Citation2013, Zule et al. Citation2016). Therefore, this method is suitable for the sequential analysis of many samples, e.g. for analysing extractives in wood exposed to weathering.
Extractives can be classified according to the polarity of the extracting solvent. Generally, they are divided into hydrophilic extractives, soluble in polar solvents and water, and lipophilic extractives, soluble in nonpolar solvents. The amount and composition of the extract obtained with each solvent vary (Vek and Oven Citation2011, Vek et al. Citation2019). Thus, the choice of the appropriate solvent depends on the research objectives, especially the target compounds or class of compounds (e.g. polyphenols), and on the further analytical procedures in the research (Vek and Oven Citation2011). The content of polyphenols in black locust heartwood obtained by different solvents and extraction methods has been previously studied (Vek et al. Citation2019). and show the concentrations of extractives and phenols in wood of certain species obtained by different extraction methods and solvents found in the existing literature. However, the extraction parameters for the isolation of polyphenols from the respective wood species have not been systematically studied. Therefore, this research aims to optimise the polyphenol extraction process to accurately investigate the relationship between the leaching of extractives and the natural durability of wood. Although extraction using ASE is not very time-consuming, it is still an important goal to optimise the duration of each extraction for a large number of samples while ensuring sufficient extraction yields.
Table 1. Total extractives content in the heartwood of certain species obtained by different extraction methods and solvents found in the existing literature.
Table 2. Total phenols content in the heartwood of certain species obtained by different extraction methods and solvents found in the existing literature.
The development of a universal extraction method that would allow quick, safe and efficient preparation of wood extracts from different tree species for chemical monitoring of extractives in wood represents an important step for our future research activities. The current study was conducted on non-weathered samples taken from sawn timber. Under the selected extraction conditions established in this research, samples exposed to natural weathering for different periods are extracted (). Then, the total extractives, total phenols and index extractives, determined in this research are analyzed. In addition, fungal and water performance tests will be conducted to provide a more comprehensive understanding of the effects of extractives on the resistance of wood to wood-degrading fungi.
2. Materials and methods
2.1. Chemicals
All solvents and reagents were of analytical grade. Extraction solvents (water, acetone, ethanol, methanol and hexane) were purchased from Merck (Sigma-Aldrich Chemie, Taufkirchen, Germany), Carlo Erba Reagents (Chaussée du Vexin, France), J.T. Baker (Phil-lipsburg, NJ, USA) and Fisher Scientific (Franklin Lakes, NJ, USA). Folin–Ciocalteu phenol reagent (2 N), sodium carbonate (anhydrous), gallic acid monohydrate (HPLC assay, 99%), castalagin (HPLC assay, ≥ 95.0%), castalin (HPLC assay, ≥ 98.0%), vescalagin (HPLC assay, ≥ 95.0%), (±)-dihydrokaempferol (HPLC assay, ≥ 95.0%), kaempferol (HPLC assay, ≥ 99.0%), matairesinol (HPLC assay, ≥ 95.0%), trans-piceatannol (HPLC assay, ≥ 95.0%), secoisolariciresinol (HPLC assay, ≥ 95.0%), pinosylvin (HPLC assay, ≥ 97.0%), pinosylvin monomethyl ether (HPLC assay, ≥ 95.0%), catechin (HPLC assay, ≥ 99.0%), gallic acid (HPLC assay, ≥ 98.0%), 4-hydroxybenzoic acid (HPLC assay, ≥ 99.0%) and resveratrol (HPLC assay, ≥ 99.0%) were also provided by Merck (Sigma Aldrich Chemie, Taufkirchen, Germany). Epicatechin (HPLC assay, ≥ 99.0%), coumaric acid (HPLC assay, ≥ 90.0%), taxifolin (HPLC assay, ≥ 99.0%), dihydrorobinetin (HPLC assay, ≥ 99.0%) and robinetin (HPLC assay, ≥ 95.0%) were from Extrasynthese (Genay, France). Lariciresinol (purity ≥ 95%) and isolariciresinol (purity ≥ 95%) were kindly provided by our colleagues from Åbo Akademi University, Laboratory of Organic Chemistry (prof. dr. Stefan Willför, dr. Patrik Eklund).
2.2. Wood material
The study included heartwood samples from three naturally durable hardwood species, European oak (Quercus sp.), sweet chestnut (Castanea sativa Mill.) and black locust (Robinia pseudoacacia L.), and heartwood from two moderately durable conifers, Scots pine (Pinus sylvestris L.) and European larch (Larix decidua Mill.). All of these species are considered relatively rich in polyphenols. Spruce (Picea abies Karst.) was used as a reference species. Wood blocks (1.5 cm × 2.5 cm × 5.0 cm) used for the field tests were defect-free, with no visible fungal growth or discolouration, as recommended in the standard EN 113 (CEN Citation2020). In the present study, only non-exposed samples that had not been previously exposed to weathering were used. For each material, five dried samples were ground on a Retsch SM 2000 cutting mill with a 1 mm screen. The ground samples were stored in a dark and cool place until the start of the chemical analyses. Before extraction, all samples were lyophilised in a Telstar LyoQuest CC1930 freeze dryer at –85 °C and 0.045 mbar for 24 h.
2.3. Extraction
2.3.1. Soxhlet extraction
Different polar solvents were used in Soxhlet extraction, because in accelerated solvent extraction (ASE) the differences between more polar solvents are not so apparent due to the effect of physical factors (temperature and pressure) (Priego-Capote and Delgado de la Torre Citation2013). According to the adapted TAPPI test method T 264 cm-97 (Wood preparation for chemical analysis, Citation1997), a standard Soxhlet extraction was performed using 2.5 g of a freeze-dried wood sample and 250 mL of solvent (Vek et al. Citation2019). The process took 6 h at a temperature of 110 °C and above (160 °C for water), so keeping the liquid boiling briskly and the siphoning from the extractor is no less than 4 times per hour. After extraction with hexane, the excess solvent was removed by evaporation in a vacuum chamber at room temperature and a vacuum of 0.01 MPa. This was followed by extraction with polar solvents. To determine the optimum polar solvent for extraction and further analysis, extractions were carried out with ethanol, methanol, acetone and water.
2.3.2. Accelerated solvent extraction
The cycle analysis was performed with the selected solvent on wood species that had not been addressed in the literature and were expected to contain more significant amounts of extractives and therefore require more extraction cycles for complete extraction. To determine the optimal number of static extraction cycles, sequential extraction was performed in an accelerated solvent extraction system ASE 350 (Thermo Scientific Dionex). First, lipophilic extractives were extracted in two 5-min static cycles with hexane at a solvent temperature of 90 °C and a pressure of 13.8 MPa (Willför et al. Citation2003a). This was followed by methanol extraction at a solvent temperature of 110 °C and a pressure of 13.8 MPa. The temperature was set above 100 °C as 10% water (v/v) was added to the methanol. The extracts were collected separately after the 1st, 2nd, 3rd, 4th and 5th cycle of extraction.
2.4. Analysis of phenolic compounds
2.4.1. Gravimetric analysis
Total lipophilic and hydrophilic extractives were determined gravimetrically. Aliquots of the extracts were dried to constant weight. Results were expressed in milligrams of extractives per gram of dry wood (mg/g dw) (Vek et al. Citation2019).
2.4.2. Spectrophotometric analysis
The content of total phenols in the extracts was measured by means of ultraviolet, and visible spectrophotometry according to the Folin–Ciocalteu method (Singleton and Rossi Citation1965, Scalbert et al. Citation1989, Vek et al. Citation2019). The wood extracts were filtered through a 0.45-μm polyamide syringe filter. To each wood extract, an aqueous solution of Folin–Ciocalteu phenol reagent, and an aqueous solution of sodium carbonate were added. After incubating the reaction mixtures, the absorbance was measured at 765 nm using a Perkin-Elmer’s Lambda UV-Vis spectrophotometer. The standard curve of gallic acid was used for the semi-quantitative evaluation of total phenols in wood. The results were expressed in milligrams of gallic acid equivalents per gram of dried wood sample (mg GAE/g dw).
2.4.3. Chromatographic analysis
The wood extracts were analyzed chromatographically with a Thermo Accela system for high-performance liquid chromatography, as already described (Vek et al. Citation2021). The extracts were filtered through a PA syringe filter (d = 25 mm) into the amber glass vials. 3 µL of the sample was introduced on ODS column (Thermo, particle size of 2.6 µm, dimensions of 4.6 mm ID × 150 mm). Water as A (+ 0.1% of formic acid, v/v) and methanol as B (+ 0.1% of formic acid, v/v) were used as the mobile phase. The column was thermostated at 30 °C, the flow rate was 1 mL min−1. Separation of wood extractives was achieved by applying a gradient from 5 to 95 of solvent B in 20 min. The separated extractives were detected with a PDA detector at 280 nm. For the separated compounds, spectra were measured at 200 - 400 nm. The compounds were identified by comparing the retention times and UV spectra with the retention times and UV spectra of reference compounds used. The quantitative analysis was performed utilising integration and the comparison of peak areas. The HPLC method was linear (R2 > 0,99).
2.5. Statistical analysis
All results (for each wood species separately) were statistically analysed using the R-Commander programme (Rcommander.com, v.2.6-x, Hamilton, Canada). First, the total variance was analysed using a one-way analysis of variance (ANOVA) (p = 0.95). Then the Tukey multiple comparisons test was performed. The test determined the significance of differences between the mean values at a 5% significance level and divided the materials by letters from a to d. There were no statistically significant differences between values labelled with the same letter.
3. Results and discussion
3.1. Solvent selection
The amounts of total extractives and phenols obtained with the four extraction solvents differed among all wood species (ANOVA, p < 0.001) (). The highest amounts of total extractives were obtained with water and methanol, averaging 84.28 and 68.95 mg/g, respectively. Lower concentrations of wood extractives were obtained with ethanol (average 50,73 mg/g) and acetone (average 32.23 mg/g).
Table 3. Total extractives content per gram of absolutely dry wood (mg/g dw) in the heartwood of European larch “Ld” (Larix decidua), Norway spruce “Pa” (Picea abies), Scots pine “Ps” (Pinus sylvestris), European oak “Q” (Quercus sp.), Sweet chestnut “Cs” (Castanea sativa) and black locust “Rp” (Robinia pseudoacacia) obtained with extraction using hexane, acetone, ethanol, methanol and water
shows the amount of total extractives in investigated wood species. For most wood species, the higher yield of phenolic compounds was obtained with methanol (average 36.78 mg/g). For spruce and oak, most phenolics were extracted with water. For pine, ethanol was the most effective solvent. The lowest amounts of total phenols were obtained with acetone (average 16.86 mg/g). However, acetone is generally known to be a very strong and selective solvent for the extraction of phenolic extractives from woody tissues (Willför et al. Citation2006, Dünisch et al. Citation2010).
Figure 2. Content of total phenols per gram of absolutely dry wood (mg GAE/g dw) in the heartwood of European larch (Larix decidua), Norway spruce (Picea abies), Scots pine (Pinus sylvestris), European oak (Quercus sp.), Sweet chestnut (Castanea sativa) and black locust (Robinia pseudoacacia) obtained with extraction using acetone, ethanol, methanol and water. Significance letters above box plots from a to d represent the significance of differences between the mean values at a 5% significance level due to Tukey multiple comparisons test. There were no statistically significant differences between materials with the same label.
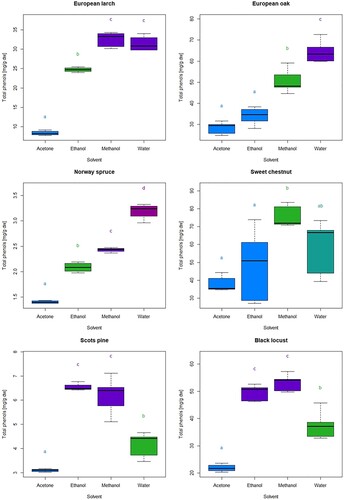
The most marked differences in the content of total extractives obtained with different solvents were found in larch (). The aqueous extracts contained by far the most extractives, 161.20 mg extractives per gram of absolutely dry wood. Gierlinger and Wimmer (Citation2004) obtained 14.4% water-soluble extractives from the heartwood of a 146-year-old larch from Langau (Austria) and 19.4% from a 250-year-old larch from Montgenévre (France). In the same study, slightly less than 3.5% acetone-soluble extractives were measured in larches from both sites, while in our study, we extracted 15.12 mg/g, only. Extractions with methanol and ethanol yielded 46.82 and 35.44 mg/g extractives, respectively. These results agree with those of Zule et al. (Citation2016), who extracted larch heartwood first with hexane and then with 95% ethanol, obtaining about 30 mg/g of extractives. In contrast to total extractives, the content of total phenols in larch does not differ drastically between the different solvents (). It is noticeable that the least phenols were contained in acetone extract (8.34 mg/g dw). The extractive contents at ethanol, and methanol were mainly attributed to phenols. On average, the extracts obtained with these solvents contained 24.74 mg GAE/g dw and 32.49 mg GAE/g dw of total phenols, corresponding to an average of 70% of the total extractives content. The aqueous extract contained a comparable phenolic content to the methanolic extracts (31.39 mg GAE/g dw). However, phenols accounted for only 20% of the total extracts, which Gerlinger et al. (Citation2004) and Gierlinger and Wimmer (Citation2004) attributed to the extraction of the non-structural, hemicellulose-type water-soluble polysaccharide arabinogalactan, which can represent up to 30% w/w of the larch wood (Füchtner et al. Citation2020). The results concerning the quantities of phenols in the wood of European larch are in agreement with the reports of other research groups (Gerlinger et al. Citation2004, Gierlinger and Wimmer Citation2004). Furthermore, very similar quantities of total phenols obtained from European larch heartwood with acetone and hot water were reported by Gerlinger et al. (Citation2004), i.e. 27 mg/g; meanwhile, the reports by Gerlinger and Wimmer (Citation2004) mention slightly higher values, namely 37 mg/g.
Spruce wood contained considerably lower amounts of extractives compared to other wood species. Our sample contained 7.30 mg/g acetone extract, 10.52 mg/g ethanol extract, and 14.09 mg/g methanol extract (). Most of the extractable substances were extracted with water, i.e. 20.50 mg/g. The results compare well with the existing literature. Willför et al. (Citation2004) extracted 2.5 mg to 5.2 and 4 mg to 26 mg extractives with 95% acetone from one gram of wood from sapwood and heartwood, respectively. Similar results were reported by Füchtner et al. (Citation2020), who extracted about 10 mg/g of extractives with ethanol and water after extraction with heptane and dichloromethane. They also reported that extraction yield was about the same when extracted with water only and below 20 mg/g when extracted with 96% ethanol. The spruce extracts contained between 15% and 20% phenols, depending on the amount of total extractives (). On average, they contained 2.28 mg/g of phenols. Most of the phenols were extracted with water (3.19 mg/g) and the fewest with acetone (1.40 mg/g) (). However, Bartnik et al. (Citation2020) obtained comparable concentrations of phenols with acetone but used ferulic acid as a standard. They reported that the mean concentration of phenolic compounds in spruce sawdust was 2.38 mg/g and varied from 0.25–9.67 mg/g.
Extraction with polar solvents from Scots pine wood gave an average content of hydrophilic extractives of 24.97 mg/g. The content of hydrophilic extractives was highest in methanol extracts (30.82 mg/g) and lowest in acetone extracts (19.95 mg/g) (). The sample also contained a noticeable amount of hexane soluble extractives (16.07 mg/g), which contained a small proportion of phenolic compounds (0.53 mg/g or 3% of the total extract). Oven et al. (Citation2015) reported similar hydrophilic extractives in Scots pine heartwood but mentioned much higher amounts of lipophilic extractives, about 85 mg/g. Fang et al. (Citation2013) also obtained higher extraction yields with hexane (97 mg/g) and similar amounts of extractives obtained with 95% acetone (24 mg/g) and hot water. Extraction yields for non-sequential extraction with water are temperature dependent. Water at 100 °C gave 20 mg/g extractives, while 43 mg/g was obtained at 140 °C (Fang et al. Citation2013). The polar solvent extracts contained 20% phenols. Ethanol extracts (6.55 mg GAE/g dw) contained the most phenols, while acetone extracts (3.09 mg/g) contained the least. The values were generally similar or somewhat lower than the average phenolic content reported by Merela et al. (Citation2019) (ca. 6 mg GAE/g dw) and Karppanen et al. (Citation2007) (ca. 10 mg TAE/g dw) for extracts obtained with a mixture of acetone and water.
Oak, widely known for its high content of extractives, especially tannins (Peng et al. Citation1991), contained between 54.32 mg/g (acetone) and 115.78 mg/g of extractives (water) in our study (). The applied extraction method with ethanol gave an average content of 68.21 mg/g and with methanol 98.39 mg/g of total extractives (). Wagenführ (Citation2000) determined the extractive content of Q. robur and Q. petraea to be between 7% and 19%, depending on the solvent. Baar et al. (Citation2017, Citation2019) noted a similarly wide range of total extractives, who reported much lower amounts of extractives obtained with 50% (v/v) aqueous methanol, ranging from 3.52% to 12.99%. Similar amounts of extractives after extraction with acetone/water (7:3, v/v) from the heartwood of a 95-year-old European oak (Q. robur or Q. petraea) were reported by Klumpers et al. (Citation1994), who extracted 94 mg/g extractives from the outer heartwood and 69 mg/g from the inner heartwood. The extracts contained about 50% phenolic compounds. Their concentration in the wood varied from 28.35 mg GAE/g dw (acetone) to 64.50 mg GAE/g dw (water) (), which is in the same range as the values reported by other authors (Scalbert et al. Citation1989, Klumpers et al. Citation1994, Aloui et al. Citation2004, Baar et al. Citation2017). While Scalbert et al. (Citation1989) concluded that extraction of Quercus robur heartwood with cold 80% aqueous methanol at room temperature or with hot 89% aqueous acetone under reflux yielded similar amounts of polyphenols, our results showed that there were differences between solvents when the same extraction parameters were used. In our case, extraction with methanol (50.64 mg/g) gave a higher yield of polyphenols than extraction with acetone or ethanol (33.99 mg/g) ().
The heartwood of Sweet chestnut generally contains slightly more extractives and polyphenols than oak, almost regardless of the solvent (, ). Only extraction with water resulted in fewer extracted compounds. The results are in good agreement with reports from other research groups (Scalbert et al. Citation1989, Sanz et al. Citation2010, Thaler et al. Citation2014, Faraone et al. Citation2021). Thaler et al. (Citation2014) reported slightly higher extraction yields (11.0% for 70% methanol and 14.2% for water). Vázquez et al. (Citation2008) indicated that extraction yields were significantly higher when aqueous solvents were used compared to extraction with organic solvents alone. Faraone et al. (Citation2021) obtained between 11% (maceration extraction) and 23% (ASE extraction) of extractives when extracting Sweet chestnut with ethanol: water (70/30, v/v). Indeed, the yield of extractable compounds increased with solvent polarity, and extraction techniques influenced the yield. The largest contribution of polyphenols was provided by methanol, which was used to extract 75.79 mg of polyphenols from one gram of absolutely dry wood (). Sanz et al. (Citation2010) obtained a much lower polyphenol content (40.8 mg/g) with 50% methanol, but the extraction was performed at room temperature. Aqueous extracts also contained comparable amounts of polyphenols (58.30 mg/g) () to those reported in the literature (53.4 mg/g) (Scalbert et al. Citation1989). On the other hand, Faraone et al. (Citation2021) extracted more phenols with 70% aqueous ethanol, ranging from 85 to 150 mg/g.
For black locust, acetone (33.22 mg/g) was the most different from the other solvents in terms of extraction yield, while the other solvents had quite similar extraction yields, ranging from 81.70 mg/g (ethanol) to 98.40 mg/g (methanol) (). Similar amounts of extractives after hot water extraction of black locust heartwood were reported by Hart (Citation1968), So et al. (Citation1980) and Adamopoulos et al. (Citation2005). Similarly, methanol and ethanol extracts contained higher amounts of polyphenols (53.13 and 49.52 mg/g) (). In comparison, acetone and water extracts contained slightly lower amounts, namely 21.86 mg GAE/g dw and 37.59 mg GAE/g dw (). Vek et al. (Citation2019), who performed extractions with aqueous solutions of acetone, ethanol and methanol, gained on average 93.01 mg/g of extractives and 38.7 mg/g of polyphenols with Soxhlet extraction, irrespective of the solvents used. They also performed extractions by maceration with stirring and sonication extraction, resulting in significantly lower extractive yields, namely 68.41 and 51.92 mg/g, respectively.
3.2. Cycle analysis
As can be seen from of the first through fifth extraction cycles, the concentrations of phenols in the 90% (v/v) aqueous methanol extracts are significantly reduced after the first cycle. Over 80% of all extractives or phenols were extracted in the first extraction cycle, and over 90% of the compounds were extracted after the second cycle in all cases. These findings somehow correlate with the results of the investigation on heartwood extractives of black locust, indicating that the first part of a Soxhlet extraction is the most productive regarding the extraction yield (Vek et al. Citation2019). However, extraction in ASE system was confirmed again as a quick and reliable technique for efficient extraction of wood material.
Table 4. Amount of total extractives and total phenols in wood extracts of European larch “Ld” (Larix decidua), European oak “Q” (Quercus sp.) and sweet chestnut “Cs” (Castanea sativa). Amount of extractives gained regarding the number of static cycles (ASE extraction).
For larch, 49.89 mg/g extractives and 31.04 mg/g phenols were extracted with the first cycle, and 5.99 mg/g extractives and 2.82 mg/g phenols were extracted with the second cycle (). All subsequent cycles contained less than 5 mg/g extractives or less than 1 mg/g phenols, indicating that using at least two extraction cycles is reasonable. Zule et al. (Citation2016) also used two extraction cycles with 95% ethanol for larch extraction. Oak and chestnut also gave significant amounts of extracts in the first two cycles; 92.82 mg/g in the first and 5.99 mg/g in the second cycle in the case of European oak, and 120.92 and 10.99 mg/g in the two consecutive extractions of sweet chestnut. However, these two wood species contained detectable amounts of phenols (above 1 mg/g) in the fourth extraction cycle (). Faraone et al. (Citation2021) used three extraction cycles for chestnut extraction, and according to our results, three or four extraction cycles would also be appropriate.
3.3. Chromatographic analysis
shows the chemical composition of the phenolic extractives in the wood extracts of the selected wood species. Using this method, the characteristics and the most abundant compounds described in the literature were detected for all wood species studied except spruce. Willför et al. (Citation2004) described 7-hydroxymatairesinol as the most abundant lignan in the heartwood of Picea abies, but it is present only in very small amounts. Therefore, it is possible that it could not be detected in our studies. In larch, taxifolin (Tax, tr = 9.73 min) eluted first, followed by dihydrokaempferol (DHKae, tr = 11.09 min). Zule et al. (Citation2016) determined nine different phenolic compounds in ethanol extracts from the heartwood of European larches using different chromatographic techniques. Among them, the flavonoids axifolin and dihydrokaempferol dominated, while the lignan secoisolariciresinol was characteristic of the higher parts of the trees. Pinosylvin (PS, tr = 15.35 min) and pinosylvin monomethyl ether (PSMME, tr = 17.89 min) were successfully identified in Scots pine heartwood. These two stilbenes, which constitute about 1% (w w–1) of the heartwood, are also cited by Willför et al. (Citation2003b) as characteristic of Pinus sylvestris. Three peaks occurred in chestnut, and the compounds eluted in the following order: castalin (CST, tr = 1.55 min), vescalagin (VCLG, tr = 3.02 min), and castalagin (CTLG, tr = 3.65 min). Mayer et al. (Citation1967) reported that the most important compounds in sweet chestnut heartwood are the isomers castalagin and vescalagin, which account for 80% of the hydrolysable tannin content. In addition, they isolated castalin and vescalin as tannin hydrolysis products of the two prominent ellagitannins, which are also considered important tannin constituents of heartwood (Mayer et al. Citation1967). The chromatogram of oak also contained the peaks of castalin, vescalagin, and castalagin (Maemmelae et al. Citation2000). Baar et al. (Citation2019) also considered these ellagitannins to be characteristic of oaks. Masson et al. (Citation1995) estimated that castalagin and vescalagin together accounted for 40% to 70% of the total ellagitannin content in oaks. In black locust heartwood, dihydrorobinetin (DHR) was detected as a characteristic non-structural phenolic compound (DHR, tr = 6.90 min). Dihydrorobinetin was previously described by many authors (Destandau et al. Citation2016, Vek et al. Citation2019) as the most abundant compound in black locust heartwood. These compounds will be referred to as the index extractives of the studied wood species. As characteristic and most abundant compounds among phenols, they have a crucial influence on wood properties, which has already been described for some wood species. For example, Venäläinen et al. (Citation2004) reported a significant difference in the average concentration of pinosylvines between the decay-resistant and susceptible Scots pine heartwood. Therefore, monitoring the content of these index extractives in wood exposed to leaching during weathering is of great interest for further research on factors affecting natural durability.
Figure 3. HPLC-PDA chromatograms of methanol extracts monitored at 280 nm, in the heartwood samples of sweet chestnut (Castanea sativa), European oak (Quercus sp.), European larch (Laricx decidua), black locust (Robinia pseudoacacia) and Scots pine (Pinus sylvestris). Index phenolic extractives are highlighted with yellow: castalin (CTL, tr = 1.55 min), vescalagin (VCLG, tr = 3.02 min), castalagin (CTLG, tr = 3.65 min), taxifolin (Tax, tr = 9.73 min), dihydrokaempferol (DHKae, tr = 11.09 min), dihydrorobinetin (DHR, tr = 6.90 min), pinosylvin (PS, tr = 15.35 min) and pinosylvin monomethyl ether (PSMME, tr = 17.89 min).
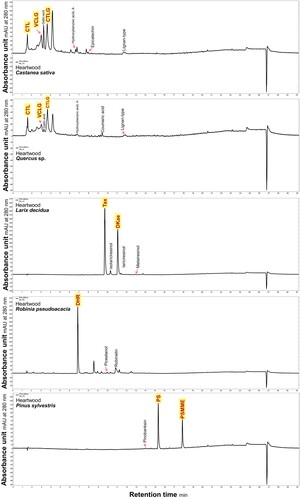
4. Conclusions
In regard to the results of our investigation, it can be concluded that aqueous methanol is a suitable solvent for the extraction of phenolic compounds from native Central European wood species. It was also demonstrated that high extraction yields could also be achieved by using water as a solvent. Extraction of the wood samples with aqueous methanol in a system for accelerated solvent extraction by applying four static cycles was demonstrated to be efficient for extracting selected wood species. The obtained phenolic extractives were qualitatively and quantitatively checked with a reliable and rapid HPLC method that is based on using an octadecyl-silica (ODS) column packed with solid core particles and methanol as eluent. With this study, we propose a way how analyse the influence of leaching of extractives on the natural durability of wood, with chemical monitoring of selected phenolic extractives.
Acknowledgements
This work was financially supported by the Slovenian Research Agency (ARRS) within the program young researcher, research program P4-0015 (Wood and lignocellulosic composites), applicative project L4-2623 (ArsAlbi) and the infrastructural centre (IC LES PST 0481-09). Part of the published research was also supported by the Ministry of Agriculture, Forestry and Food in the frame of project V4-2017.
Conceptualisation V.V. and E.K.; methodology V.V. and E.K.; validation E.K. and V.V., formal analysis E.K. and V.V.; investigation E.K. and V.V.; resources M.H., P.O., and V.V.; data curation E.K. and V.V.; writing – original draft preparation E.K. and V.V.; writing – review & editing V.V., M.H. E.K. and P.O.; visualisation E.K. and V.V.; supervision V.V. and M.H.; project administration M.H.; funding acquisition M.H. All authors have read and agreed to the published version of the manuscript.
Disclosure statement
No potential conflict of interest was reported by the author(s).
Data availability
The experimental data used in this study are available on request from the corresponding author.
Additional information
Funding
References
- Adamopoulos, S., Voulgaridis, E. and Passialis, C. (2005) Variation of certain chemical properties within the stemwood of black locust (Robinia pseudoacacia L.). Holz Als Roh - Und Werkstoff, 63(5), 327–333. doi:10.1007/s00107-005-0018-3
- Aloui, F., Nizar, A., Charrier, F. and Charrier, B. (2004) Durability of European oak (Quercus petraea and Quercus robur) against white rot fungi (Coriolus versicolor): relations with phenol extractives. European Journal of Wood and Wood Products, 62(4), 286–290. doi:10.1007/s00107-004-0489-7
- Baar, J., Paschová, Z., Hofmann, T. and Hapla, F. (2017) Effect of site conditions on extractives content in Sessile oak. IUFRO Division 5 Conference 2017 and 60th SWST International Convention – Forest Sector Innovations for a Greener Future: Final Program, Proceedings and Abstracts, Unpaged. Madison: Society of Wood Science and Technology.
- Baar, J., Paschová, Z., Hofmann, T., Kolář, T., Koch, G., Saake, B. and Rademacher, P. (2019) Natural durability of subfossil oak: Wood chemical composition changes through the ages. Holzforschung, 74(1), 47–59. doi:10.1515/hf-2018-0309
- Bartnik, C., Nawrot-Chorabik, K. and Woodward, S. (2020) Phenolic compound concentrations in Picea abies wood as an indicator of susceptibility towards root pathogens. Forest Pathology, 50(6), 1–9. doi:10.1111/efp.12652
- Brischke, C. and Alfredsen, G. (2022) Biological durability of pine wood. Wood Material Science and Engineering, 1–15. doi:10.1080/17480272.2022.2104134
- Brischke, C., Bayerbach, R. and Otto Rapp, A. (2006) Decay-influencing factors: A basis for service life prediction of wood and wood-based products. Wood Material Science and Engineering, 1(3–4), 91–107. doi:10.1080/17480270601019658
- Broda, M. (2020) Natural compounds for wood protection against fungi—a review. Molecules, 25(15), 1–24. doi:10.3390/molecules25153538
- CEN (2013) European standard EN 335: Durability of wood and wood-based products. Use classes: definitions, application to solid wood and wood-based products (Brussels, Belgium: CEN-European Committee for Standardization).
- CEN (2016) European standard EN 350: 2016 durability of wood and wood-based products. Testing and classification of the durability tobiological agents of wood and wood-based materials (Brussels, Belgium: CEN-European Committee for Standardization).
- CEN (2020) European standard EN 113-2: Durability of wood and wood-based products - test method against wood destroying basidiomycetes - part 2: Assessment of inherent or enhanced durability (Brussels, Belgium: CEN-European Committee for Standardization).
- Destandau, E., Charpentier, J. P., Bostyn, S., Zubrzycki, S., Serrano, V., Seigneuret, J. M. and Breton, C. (2016) Gram-scale purification of dihydrorobinetin from Robinia pseudoacacia L. Wood by centrifugal partition chromatography. Separations, 3(3), 1–12. doi:10.3390/separations3030023
- Dünisch, O., Richter, H. G. and Koch, G. (2010) Wood properties of juvenile and mature heartwood in Robinia pseudoacacia L. Wood Science and Technology, 44, 301–313. doi:10.1007/s00226-009-0275-0
- Eichhorn, S., Erfurt, S., Hofmann, T., Seegmüller, S., Németh, R. and Hapla, F. (2017) Determination of the phenolic extractive content in sweet chestnut (Castanea Sativa Mill.) Wood. Wood Research, 62(2), 181–196.
- Fang, W., Hemming, J., Reunanen, M., Eklund, P., Pineiro, E. C., Poljansek, I., Oven, P. and Willför, S. (2013) Evaluation of selective extraction methods for recovery of polyphenols from pine. Holzforschung, 67(8), 843–851. doi:10.1515/hf-2013-0002
- Faraone, I., Russo, D., D’Auria, M., Bruno, M. R., Cetera, P., Todaro, L. and Milella, L. (2021) Influence of thermal modification and extraction techniques on yield, antioxidant capacity and phytochemical profile of chestnut (Castanea sativa Mill.) wood. Holzforschun, 75(3), 260–268. doi:10.1515/hf-2020-0037
- Füchtner, S., Brock-Nannestad, T., Smeds, A., Fredriksson, M., Pilgård, A. and Thygesen, L. G. (2020) Hydrophobic and hydrophilic extractives in Norway spruce and kurile larch and their role in Brown-Rot degradation. Frontiers in Plant Science, 11(June), doi:10.3389/fpls.2020.00855
- Gierlinger, N., Jacques, D., Schwanninger, M., Wimmer, R. and Pâques, L. E. (2004) Heartwood extractives and lignin content of different larch species (Larix sp.) and relationships to brown-rot decay-resistance. Trees, 18(March), 230–236. doi:10.1007/s00468-003-0300-0
- Gierlinger, N. and Wimmer, R. (2004) Radial distribution of heartwood extractives and lignin in mature European larch. Wood and Fiber Science, 36(3), 387–339.
- Hart, J. H. (1968) Morphological and chemical differences between sapwood, discolored sapwood, and heartwood in Black Locust and Osage Orange. Forest Science, 14(3), 334–338. doi:10.1093/forestscience/14.3.334
- Kai, Y. (1991) Chemistry of extractives. In D. N. S. Hon, and N. Shiraishi (eds.), Wood and Cellulosic Chemistry (Nex York: Marcel Dekker), pp. 215–255.
- Karppanen, O., Venäläinen, M., Harju, A. M., Willför, S., Pietarinen, S., Laakso, T. and Kainulainen, P. (2007) Knotwood as a window to the indirect measurement of the decay resistance of Scots pine heartwood. Holzforschung, 61(5), 600–604. doi:10.1515/HF.2007.091
- Keržič, E. and Humar, M. (2021) Studies on the material resistance and moisture dynamics of wood after artificial and natural weathering. Wood Material Science and Engineering, 17(6), 551–557. doi:10.1080/17480272.2021.1902388
- Kirker, G. T., Blodgett, A. B., Arango, R. A., Lebow, P. K. and Clausen, C. A. (2013) The role of extractives in naturally durable wood species. International Biodeterioration and Biodegradation, 82, 53–58. doi:10.1016/j.ibiod.2013.03.007
- Klumpers, J., Scalbert, A. and Janin, G. (1994) Ellagitannins in European oak wood: Polymerisation during wood ageing. Phytochemistry, 36(5), 1249–1252. doi:10.1016/S0031-9422(00)89646-6
- Mämmelä, P., Savolainen, H., Lindroos, L., Kangas, J. and Vartiainen, T. (2000) Analysis of oak tannins by liquid chromatography-electrospray ionisation mass spectrometry. Journal of Chromatography A, 891(1), 75–83. doi:10.1016/S0021-9673(00)00624-5
- Masson, G., Moutounet, M. and Puech, J. L. (1995) Ellagitannin content of oak wood as a function of species and of sampling position in the tree. American Journal of Enology and Viticulture, 46(2), 262–268.
- Mayer, W., Gabler, W., Riester, A. and Korger, H. (1967) Die Isolierung von Castalagin, Vescalagin, Castalin und Vescalin. Liebigs Annalen der Chemie, 707, 177–181.
- Merela, M., Turičnik, V., Vek, V. and Oven, P. (2019) Anatomske, kemijske in sorpcijske lastnosti beljave in jedrovine rdečega bora. Acta Silvae et Ligni, 119, 43–54. doi:10.20315/asetl.119.4
- Meyer-Veltrup, L., Brischke, C., Alfredsen, G., Humar, M., Flæte, P. O., Isaksson, T., Larsson Brelid, P., Westin, M. and Jermer, J. (2017) The combined effect of wetting ability and durability on outdoor performance of wood: development and verification of a new prediction approach. Wood Science and Technology, 51(3), 615–637. doi:10.1007/s00226-017-0893-x
- Oven, P., Poljanšek, I. and Vek, V. (2015) Bioaktivne spojine v lesu borov / Pinosylvins as valuable bioactive compounds in the wood of pines. In H. Kraigher and M. Humar (Eds.), Monitoring v gozdarstvu, lesarstvu in papirništvu: proceedings of the scientific meeting Gozd in les, (Vol. May 2015) (Ljubljana, Slovenia: Slovenian Forestry Institute, The Silva Slovenica Publishing Centre), pp. 14–17.
- Peng, S., Scalbert, A. and Monties, B. (1991) Insoluble ellagitannins in Castanea sativa and Quercus petraea woods. Phytochemistry, 30(3), 775–778. http://dx.doi.org/10.1016/0031-9422(91)85250-4
- Pietarinen, S. P., Willför, S. M., Ahotupa, M. O., Hemming, J. E. and Holmbom, B. R. (2006) Knotwood and bark extracts: Strong antioxidants from waste materials. Journal of Wood Science, 52(5), 436–444. doi:10.1007/s10086-005-0780-1
- Preparation of wood for chemical analysis TAPPI T264 cm-97 (standard) (1997), available at: https://books.google.si/books?id = XnsYNAAACAAJ.
- Priego-Capote, F. and Delgado de la Torre, M. del P. (2013) Accelerated liquid extraction. In Rostagno M. A., and Prado J. M (eds.), Natural Product Extraction: Principles and Applications ( Cambridge, United Kingdom, Royal Society of Chemistry), pp. 157–195.
- Sablík, P., Giagli, K., Pařil, P., Baar, J. and Rademacher, P. (2016) Impact of extractive chemical compounds from durable wood species on fungal decay after impregnation of non-durable wood species. European Journal of Wood and Wood Products, 74(2), 231–236. doi:10.1007/s00107-015-0984-z
- Sanz, M., Cadahía, E., Esteruelas, E., Muñoz, ÁM, Fernández De Simón, B., Hernández, T. and Estrella, I. (2010) Phenolic compounds in chestnut (Castanea sativa Mill.) heartwood. Effect of Toasting at Cooperage. Journal of Agricultural and Food Chemistry, 58(17), 9631–9640. doi:10.1021/jf102718t
- Scalbert, A. (1991) Antimicrobial properties of tannins. Phytochemistry, 30(12), 3875–3883. doi:10.1016/0031-9422(91)83426-L
- Scalbert, A., Monties, B. and Janin, G. (1989) Tannins in wood: Comparison of different estimation methods. Journal of Agricultural and Food Chemistry, 37(5), 1324–1329. doi:10.1021/jf00089a026
- Singh, T. and Singh, A. P. (2012) A review on natural products as wood protectant. Wood Science and Technology, 46(5), 851–870. doi:10.1007/s00226-011-0448-5
- Singleton, V. L. and Rossi, J. A. (1965) Colorimetry of total phenolics with phosphomolybdic-phosphotungstic acid reagents. American Journal of Enology and Viticulture, 16(3), 144–158. available at: http://www.ajevonline.org/cgi/content/abstract/16/3/144.
- So, W. T., Park, S. J., Kim, J. K., Shim, K., Lee, K. Y., Hyun, J. W., Lee, H. S., Kang, S. K. and Jo, J. M. (1980) On wood properties of imported (introduced) species grown in Korea. Wood properties of Pinus stobus, Pinus silvestris, Pinus banksiana, Picea abies and Robinia pseudoacacia. Research Reports of the Forest Research Institute, Korea, 27, 7–31.
- Taylor, A. M., Gartner, B. L. and Morrell, J. J. (2002) Heartwood formation and natural durability - a review. Wood and Fiber Science, 34, 587–611. available at: https://ir.library.oregonstate.edu/concern/articles/rj430494s?locale = en%0Apapers3://publication/uuid/AA7D8AD3-4D4F-4B28-87AA-D1E224F47D36.
- Thaler, N., Žlahtič, M. and Humar, M. (2014) Performance of recent and old sweet chestnut (Castanea sativa) wood. International Biodeterioration and Biodegradation, 94, 141–145. doi:10.1016/j.ibiod.2014.06.016
- Vázquez, G., Fontenla, E., Santos, J., Freire, M., González-Álvarez, J. and Antorrena, G. (2008) Antioxidant activity and phenolic content of chestnut (Castanea sativa) shell and eucalyptus (Eucalyptus globulus) bark extracts. Industrial Crops and Products, 28(3), 279–285. doi:10.1016/j.indcrop.2008.03.003
- Vek, V., Balzano, A., Poljanšek, I., Humar, M. and Oven, P. (2020) Improving fungal decay resistance of less durable sapwood by impregnation with scots pine knotwood and black locust heartwood hydrophilic extractives with antifungal or antioxidant properties. Forests, 11(9), 1–23. doi:10.3390/F11091024
- Vek, V., Keržič, E., Poljanšek, I., Eklund, P., Humar, M. and Oven, P. (2021) Wood extractives of silver fir and their antioxidant and antifungal properties. Molecules, 26(21), doi:10.3390/molecules26216412
- Vek, V. and Oven, P. (2011) Influence of different polar solvents on contents of total phenols in wood extracts. Les, 63(3), 45–48.
- Vek, V., Oven, P., Ters, T., Poljanšek, I. and Hinterstoisser, B. (2014) Extractives of mechanically wounded wood and knots in beech. Holzforschung, 68(5), 529–539. doi:10.1515/hf-2013-0003
- Vek, V., Poljanšek, I. and Oven, P. (2019) Efficiency of three conventional methods for extraction of dihydrorobinetin and robinetin from wood of black locust. European Journal of Wood and Wood Products, 77(5), 891–901. doi:10.1007/s00107-019-01430-x
- Venäläinen, M., Harju, A. M., Saranpää, P., Kainulainen, P., Tiitta, M. and Velling, P. (2004) The concentration of phenolics in brown-rot decay resistant and susceptible Scots pine heartwood. Wood Science and Technology, 38(2), 109–118. doi:10.1007/s00226-004-0226-8
- Wagenführ, R. (2000) Holzatlas (München: Fachbuchverlag Leipzig im Carl Hanser Verlag).
- Willför, S., Hemming, J., Reunanen, M., Eckerman, C. and Holmbom, B. (2003a) Lignans and lipophilic extractives in Norway spruce knots and stemwood. Holzforschung, 57(1), 27–36. doi:10.1515/HF.2003.005
- Willför, S., Hemming, J., Reunanen, M. and Holmbom, B. (2003b) Phenolic and lipophilic extractives in Scots pine knots and stemwood. Holzforschung, 57(4), 359–372. doi:10.1515/HF.2003.054
- Willför, S., Nisula, L., Hemming, J., Reunanen, M. and Holmbom, B. (2004) Bioactive phenolic substances in industrially important tree species. part 1: Knots and stemwood of different spruce species. Holzforschung, 58, 335–344.
- Willför, S. M., Smeds, A. I. and Holmbom, B. R. (2006) Chromatographic analysis of lignans. Journal of Chromatography A, 1112(1–2), 64–77. doi:10.1016/j.chroma.2005.11.054
- Williams, R. S (2005) Weathering of wood. In Rowell, R.M. (ed.), Handbook of Wood Chemistry and Wood Composites (Boca Raton, Florida, Taylor and Francis, CRC Press), pp. 139–185.
- Zule, J., Čufar, K. and Tišler, V. (2016) Hidrofilni ekstraktivi u srži europskog ariša (Larix decidua Mill.) /Hydrophilic extractives in heartwood of European larch (Larix decidua Mill.). Drvna Industrija, 67(4), 363–370. doi:10.5552/drind.2016.1618