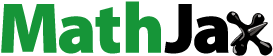
ABSTRACT
The demand for construction timber is continuously increasing, due to its favourable characteristics. However, the adequate protection of wood is key to its successful use, as it is flammable and susceptible to biodegradation. Given that thermal modification enhances the durability of wood, and mineralisation with CaCO3 considerably improves its fire properties, it is worth considering the combined effects of the two methods.
European beech (Fagus sylvatica) was selected to determine the effects of a) thermal modification, b) mineralisation through the in-situ formation of CaCO3, and c) a combination of the two procedures, on resistance to decay fungi, reaction to fire and the mechanical properties of the wood. Microscopic analysis and comparisons of the samples before and after exposure to fungi were also conducted.
Mineralised wood generally had a slightly alkaline pH value and higher equilibrium moisture content, while thermal modification lowered the equilibrium moisture content. The present study demonstrated the combined effect of thermal modification and mineralisation: the best response to fire as well as resistance to fungi was achieved when the two treatments were combined. Results from the Brinell hardness and three-point bending tests indicate that both modification procedures can slightly impair the mechanical properties of the wood.
Introduction
Throughout history, wood has been considered to be one of the most important materials, and has been used for a wide variety of applications (Dunningham and Sargent Citation2015, Eder Citation2021). The main disadvantages of wood are its poor dimensional stability, flammability and susceptibility to biodegradation (Sandberg et al. Citation2017, Humar et al. Citation2020). Durability is especially important for outdoor applications. When the inherent natural durability of wood is insufficient, it needs to be protected to prolong its service life. The more intensive use of wood in recent decades, primarily for construction purposes, has increased the need for its protection (Dunningham and Sargent Citation2015). It is predicted that there will be an even greater shortage of wood over the coming decades (Odppes et al. Citation2021). Due to environmental awareness, in most countries a ban has been imposed on most conventional active ingredients used in the production of wood preservatives, and this, combined with a lack of supply of naturally durable wood species, has shifted development towards so-called green methods of wood protection (Singh and Singh Citation2012, Dunningham and Sargent Citation2015). One of the most important and environmentally acceptable methods is thermal modification (TM), which involves heating wood in conditions of reduced oxygen concentration to temperatures between 160°C and 240°C (Hill Citation2006, Zelinka et al. Citation2022). This method omits the need for potentially hazardous chemicals (Poncsak et al. Citation2009). An improvement in the inherent durability to fungal decay is only one of several positive changes achieved by thermal modification (Humar et al. Citation2020), but unfortunately the fire performance of wood is also compromised (Čekovska et al. Citation2017). Wood-based materials are known to have poor fire resistance, making them a fire safety hazard and presenting a limiting factor for the broader utilisation of wood in construction (Huang et al. Citation2018, Ali et al. Citation2019). Conventionally, fire-retardants have been used to address this problem. They are beneficial in the early stages of fire development and are crucial for the safe evacuation of a burning building (Yona et al. Citation2021). On the other hand, there are many drawbacks of fire-retardant treatments, which can cause environmental and health issues (Merk et al. Citation2015, Guo et al. Citation2019) as well as increase the hygroscopicity, impair the dimensional stability, impair adhesion, increase the roughness, and reduce the mechanical properties of wood, amongst other things, as well as cause corrosion of fasteners (Taghiyari Citation2012). Many effective commercial fire-retardants are based on phosphate or borate salts to reduce flame spread. The mechanism of acid dehydration, however, which effectively reduces flame spread, can also lead to a loss of strength in treated wood (Lebow and Winandy Citation1999, Ozyhar et al. Citation2021). Improving the fire properties of wood is challenging, because it is difficult to meet the ever-evolving fire safety standards while minimising the impact on the environment and human health (Ozyhar et al. Citation2021). Over time, several methods have been developed to improve the reaction of wood to fire. One such method is mineralisation with inorganic materials such as carbonates, which can be considered an environmentally acceptable alternative to conventional fire-retardant treatments (Merk et al. Citation2016). Various carbonate mineralisation processes have been proposed, primarily differing in the way in which minerals are introduced into the structure of the wood (Pondelak et al. Citation2021b). Many authors have demonstrated an improved reaction to fire in wood mineralised with CaCO3 (Merk et al. Citation2015, Merk et al. Citation2016, Huang et al. Citation2018, Moja et al. Citation2020 and Pondelak et al. Citation2021b). A similar improvement has also been observed in composites made from pine flakes mineralised with CaCO3 (Tao et al. Citation2019). Due to this positive effect on the fire properties, the mineralisation of wood with carbonates improves its reliability for use in buildings without compromising its intrinsic properties, meaning that wood modified in this way has excellent potential for use in buildings (Merk et al. Citation2015) and even outdoor applications (Pondelak et al. Citation2021b). But on the other hand, the influence of mineralisation on mechanical properties of wood has not yet been fully investigated, therefore its use in construction purposes might be hindered. Additionally, CaCO3 acts as an adsorbent and can neutralise hazardous acidic combustion emissions (i.e. hydrochloric acid) during burning (Ozyhar et al. Citation2021).
The synergistic effect of thermal modification and mineralisation on the resistance of wood to fungal decay was confirmed in a study by Repič et al. (Citation2022), but the effects on its fire properties were not determined. The aim of the present study was to comprehensively investigate the combined or even synergistic effects of these two environmentally acceptable methods (mineralisation and thermal modification) on the relevant properties of wood i.e. flammability, susceptibility to biodegradation and mechanical properties. The possibility of improving the durability and fire properties of wood by combining these two methods was investigated, furthermore mechanical properties and visual appearance of the wood following each modification were considered as well.
Materials
European beech wood (Fagus sylvatica) was selected as the model wood species because it is the most widespread wood species in Slovenia (Brus Citation2012) and the most common hardwood in Europe (Gryc et al. Citation2008, Beqo et al. Citation2015). We prepared various samples (described in detail for each method) of thermally modified (BT) and mineralised (B Ca) beech wood, as well as samples treated using both procedures (BT Ca). The samples treated with both protection methods were first thermally modified and then mineralised. In parallel, we also tested untreated beech wood (B) as a reference. All samples were made from wood of the same origin, such that variability was kept to a minimum.
The wood was thermally modified at 220°C, according to the commercial Silvapro® process (Rep and Pohleven Citation2002, Rep et al. Citation2012). The entire modification process, including cooling, lasted approximately 24 h. The mass loss after modification was between 11% and 15%, indicating the severity of the modification. Following modification, the wood was conditioned for three weeks under standard laboratory conditions (T = 20°C; RH = 65%) before further processing.
Mineralisation was carried out according to the procedure proposed by Pondelak et al. (Citation2021b), where the wood is impregnated with a solution of calcium acetoacetate, which is then converted to calcium carbonate during post-treatment (Pondelak et al. Citation2021a, Citation2021b). During post-treatment, wood was exposed to high relative humidity (RH ˃ 80%) at a temperature of 80°C. The synthesis of the calcium acetoacetate solution is described in detail by Škrlep et al. (Citation2014, Example 2). Following mineralisation, the wood was conditioned under standard laboratory conditions (T = 20°C; RH = 65%) for three weeks. The retention of carbonates was 12 wt. % ± 4 wt. %.
Methods
From the data obtained regarding mass (m) and volume (V), the density of the oven dry samples (ρ0) according to the method by Kollman (Citation1951) was calculated. Next, the water performance of the wood was investigated using various methods. In addition to the moisture content of the samples, the data for which was taken from Repič et al. (Citation2022), the fibre saturation (FS) was determined by calculating the ratio of volume swelling (αv) to dry density (ρ0), according to EquationEq. (1(1)
(1) ), and correcting for the density of adsorbed water (Gorišek, Citation2009):
(1)
(1) Where the density of adsorbed water (ρvv) is higher than the density of free water and is 1130 kg/m3 as stated by Gorišek (Citation2009).
Five samples of each material, 50 mm × 25 mm × 15 mm in size, were placed upright in a rig such that only the cross-sectional surfaces of the samples were submerged, to a depth of approximately 0.5 mm below the water surface. Capillary uptake of liquid water by the cross-sectional surface was measured after 200 s of exposure. The samples were weighed and the amount of water absorbed per unit area was determined. The average and standard deviation of the 5 measurements were then calculated. Similarly, five samples of each material, 50 mm × 25 mm × 15 mm in size, were then immersed in water and the water uptake was determined after 1 and 24 h. The liquid water uptake results are presented as the average and standard deviation of 5 parallels given in grams, assuming that the area of all the samples was approximately equal. Volumetric shrinkage (βv) was calculated as the percentage change in volume from the fresh (Vvl) to the oven dry state (V0). To determine the dimensions of the samples in the completely wet state, the samples were pressure impregnated with water at the end of the water uptake experiments. The volumetric shrinkage of each sample was determined according to EquationEq. (2(2)
(2) ) by Gorišek (Citation2009) and presented as the average and standard deviation of the 5 measurements:
(2)
(2) The anti-shrinking efficiency (ASE) was also determined based on the volumetric shrinkages (βv) calculated. ASE was determined for all the treated samples with respect to the untreated reference sample, according to EquationEq. (3
(3)
(3) ) by Gennari et al. (Citation2021):
(3)
(3) where βref is the volumetric shrinkage of the untreated reference sample, and βtre is the volumetric shrinkage of the treated sample.
In addition to the hot water extract pH data taken from Repič et al. (Citation2022), surface pH value was determined using a Titrino titrator (Metrohm, Switzerland), as described by Ammann et al. (Citation2016). Five samples, 100 mm × 100 mm × 10 mm in size, were conditioned for three weeks under laboratory conditions (T = 20°C, RH = 65%), sanded, wiped with a damp cloth and dried before measurement. First, 1 mL of deionised water was applied to the surface, then the probe was brought into contact with the surface. The measurement ended when the pH value remained constant for 30 s. The pH value was measured to an accuracy of two decimal places. The total duration of the measurement was approximately 3 min. No change in the amount of water applied to the surface of the sample was observed during this time. The results given are the average of a total of 10 measurements taken on 5 different samples of the same material. Measurements were performed in a room subject to laboratory conditions (T = 20°C, RH = 65%). Both the pH value of the hot water extract and the pH value of the surface are reported in this work.
Resistance to wood decay fungi was determined according to the standard (CEN Citation2020a). The procedure is described in detail by Repič et al. (Citation2022). Briefly, two different wood decay fungi were used: white rot, represented by Trametes versicolour (TV) (ZIM L057), and brown rot, represented by Gloeophyllum trabeum (GT) (ZIM L017). The fungi were obtained from the fungal collection of the Biotechnical Faculty, University of Ljubljana, which is available to research institutions on demand. Detailed information regarding the origin and identification of the fungal isolates are available in the respective catalogue (Raspor et al. Citation1995). Potato dextrose agar (DIFCO, USA) was used as the culture medium, which was poured into glass jars. The sterile samples were then placed in the jars and subjected to fungal decomposition for 16 weeks. After 16 weeks the samples were cleaned, dried to a dehydrated state, and weighed, then the amount of weight loss was determined. 5 samples of each material were exposed to each of the fungi.
Microscopic analysis was conducted with a scanning electron microscope (Joel IT 500 LV, Japan). The working distance and accelerating voltage were 10 mm and 10 kV, respectively. Micrographs were captured at 200× magnification. The samples for SEM analysis originated from the resistance to wood decay fungi experiment. Exposed and unexposed samples were cut to a length of 10 mm, vacuum impregnated with a commercial epoxy resin, Crystalres (Samson Kamnik, Slovenija), and polished with a diamond suspension, MicroPolish (Buehler, USA) (0.3 μm), using a Beta vector grinder-polisher (Buehler, USA). The samples were placed on a carbon tape and observed in low vacuum mode.
The fire properties of the samples were determined using a cone calorimeter (FTT, UK) according to the standard (ISO Citation2018). Samples with dimensions of 100 mm × 100 mm × 10 mm were conditioned under laboratory conditions prior to the test, wrapped in aluminium foil and exposed to uniform radiation of 50 kW/m2. The fire properties were determined in five samples of each material. Each measurement lasted 600 s. Using ConeTools software (RISE, Sweden), the data measured were used to simulate measurements according to the standard (CEN Citation2020b) (single burning item - SBI) to determine values for two key criteria in the classification of reaction to fire according to the standard (CEN Citation2019); namely, the total heat release in the first 600 s of the test (THR600s) and the fire growth rate index (FIGRA). The time of ignition for the samples was also measured.
Two different experiments were performed to evaluate the mechanical properties of the samples. The hardness and bending properties of all samples were determined according to the standards (CEN Citation2020c and ISO Citation2014). The samples were conditioned for three weeks under laboratory conditions (T = 20°C, RH = 65%) before conducting the mechanical tests.
Brinell hardness was measured using a Zwick Z100 testing machine (Zwick Roell AG, Germany). A polished, hardened steel ball with a diameter of 10 mm was used as the indenter. A force of 1000 N was reached after approximately 15 s. This 1000 N force was maintained for another 25 s, then the indenter was removed. Three minutes after the indenter was withdrawn, residual indentation measurements were made, such that two diameters were obtained for each indentation (across the grain (d1) and along the grain (d2)). The average indentation diameter (d) was then calculated from d1 and d2. Brinell hardness was calculated according to EquationEq. (4(4)
(4) ) as prescribed by the standard (CEN Citation2020c):
(4)
(4) Where F is the applied force (1000 N), D is the diameter of the indenter (10 mm) and d is the average indentation diameter.
The hardness was measured on samples of 100 mm × 100 mm × 10 mm in size. Ten measurements were made for each material tested, and the average results are reported along with standard deviations.
Bending strength was determined using a Zwick Z100 testing machine (Zwick Roell AG, Germany), with 10 replicates tested for each material. The dimensions of the samples were 360 mm × 20 mm × 20 mm, and the distance between supports was 280 mm. The samples were oriented half-radially (the inclination of the growth rings on the cross-section was approximately 45°). The radius of the loading head was 40 mm. The test was conducted at a speed of 10 mm/min, with test duration of approximately 3 min for all samples. The modulus of elasticity, modulus of rupture and strain at break were reported as an average of 10 measurements per material and calculated using the software testXpert III (Zwick Roell AG, Germany).
Results and discussion
Water performance of wood
The moisture content (MC) of wood plays an important role on its service life, as it is one of the main factors influencing fungal decay (Thybring et al. Citation2018). The moisture performance of wood cannot be assessed by a single test, therefore several tests were performed (Meyer-Veltrup et al. Citation2017). In addition to the moisture content of the wood exposed to laboratory conditions (T = 20°C; RH = 65%), as obtained in a study by Repič et al. (Citation2022), the capillary water uptake over 200 s and the water uptake over 24 h (in the submersion test) were also determined, and the fibre saturation, volumetric shrinkage and anti-shrinking efficiency were calculated from these measurements ().
Table 1. Physical properties of samples. Data on moisture content and pH value of hot water extract were taken from Repič et al. (Citation2022).
The moisture content of wood was determined after conditioning at RH = 65%. The lowest MC was observed in thermally modified beech (BT), while the reference beech (B) had the highest equilibrium moisture content (). Under the conditions given, the mineralised beech (B Ca) and beech with the combined treatment (thermally modified and mineralised) (BT Ca) had a moisture content lower than the reference beech, but higher than that of the TM beech. This is in line with data from the literature, confirming that thermal modification improves the sorption properties of wood (Esteves and Pereira Citation2009).
The effect of thermal modification on fibre saturation (FS) is evident; BT reaches FS at approx. 19% MC, compared to at approx. 33% MC in the reference sample. The effect of mineralisation on FS is not as pronounced. B Ca and BT Ca reached FS at approx. 30% MC, which is comparable to in the reference sample. The FS in BT Ca sample was about 10% higher than in the BT sample (approx. 19%). This is very positive, as the introduction of crystals did not affect the sorption properties of the wood in this regard.
shows that liquid water capillary uptake in the axial direction was most notable in BT Ca, followed by B and B Ca. The lowest capillary uptake was observed in the BT sample and was about one third of that of the BT Ca sample. A similar result was obtained for water uptake, which was determined by the 1 and 24 h submersion tests. In general, mineralisation increased water uptake in the submersion test, while thermal modification had the opposite effect (). An increased water uptake in submersion tests following mineralisation with CaCO3 was also reported by Moya et al. (Citation2020), while crystals in the wood were previously seen to have a similar effect on sorption properties in wood treated with NaCl and H3BO3 (Lesar et al. Citation2011). In mineralised thermally modified wood, the thermal modification somehow neutralises the effect of mineralisation. This is one of the benefits of combining mineralisation with thermal modification.
It is known that European beech wood is subject to considerable dimensional changes (Grych et al. Citation2008). Volumetric shrinkage from a water-saturated state to the oven dry state was found to be highest in B Ca (approx. 17%), followed by B (). The values of volumetric shrinkage are comparable to those for European beech wood published by Skaravelis and Mantanis (Citation2013) (15.2–15.6%). BT samples show the lowest shrinkage of approx. 9%, while BT Ca samples show a shrinkage the B and BT sample. The shrinking of wood is related to its MC, and the good performance of the BT sample can be attributed to its favourable sorption properties (Sandberg et al. Citation2017). On the other hand, the increased shrinkage of the mineralised sample (B Ca) can be attributed to its higher moisture content, relatively high density, and low porosity. The anti-shrinkage efficiency (ASE) was calculated for all treated samples in relation to the reference (). Mineralisation has a negative effect on ASE, as the shrinkage of the B Ca sample was 13% higher than that of the reference. The highest ASE is achieved through thermal modification (38%), while a combination of the two methods led to an ASE of 9%. A similar (40–60%) reduction in volumetric swelling in different wood species as a result of thermal modification was also reported by Militz and Altgen (Citation2014) and Gennari et al. (Citation2021).
pH value
In general, wood is slightly acidic, and in the case of thermal modification, the pH may decrease slightly, which may also affect fungal degradation (Boonstra et al. Citation2007). With the process of mineralisation, the pH value of the wood increases significantly. This information is important when considering the protection of wood against fungal decay, as fungi require a slightly acidic environment for decay to occur (Maurice et al. Citation2011). The results of the pH value measurements on the surface of the samples are presented in . Mineralised samples have higher pH values (approx. 8) than the reference beech and TM beech (approx. 5). The increase in the pH value of mineralised wood is attributed to the incorporation of CaCO3, which is basic (pH 9) (Martín-Martínez Citation2002).
Table 2. Mechanical properties of samples.
The results obtained in this study (by measuring the pH value on the surface of the samples) show the same trend as presented in our previous study, where the pH values of the hot water extract were measured (Repič et al. Citation2022). In general, it can be seen that the standard deviation is much lower when the pH value of the hot water extract is measured. With both methods, the pH values determined for both the reference and the TM sample are also within the standard deviation of the mean. A slightly more significant difference can be seen in the mineralised samples, as the pH value of the surface is higher than that of the hot water extract. We believe this is due to the higher concentration of alkaline CaCO3 at the surface of the sample. Using both methods, we have shown that the pH value at the surface can differ from the average pH value of the whole sample. We therefore believe that both measurements are important, especially when the material is subjected to surface treatment or adhesive bonding.
Resistance to wood decay fungi
A well-established method for assessing the resistance of wood to decay fungi is to determine the weight loss after 16 weeks of exposure to the specified fungi (CEN Citation2020a). We selected two fungi, Gloeophyllum trabeum, representing brown rot, and Trametes versicolour, representing white rot, to investigate the durability of wood samples against fungi. The percentage of mass loss of the samples is shown in , and the samples after exposure to fungi are shown and compared with the non-degraded samples (on top) in and . It should be pointed out that the appearance of samples exposed to fungi can be misleading, as deposits of calcium carbonate and some mycelium are visible on the surface of mineralised samples, but do not represent decay.
Figure 1. Samples following exposure to Gloeophyllum trabeum in a) the reference beech wood, b) the mineralised wood, c) the thermally modified wood, and d) the wood treated using both procedures. The unexposed sample is placed on the top of the degraded samples for comparison.
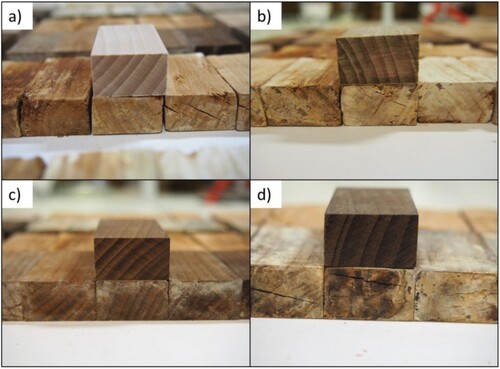
Figure 2. Samples following exposure to Trametes versicolour in a) the reference beech wood, b) the mineralised wood, c) the thermally modified wood, and d) the wood treated using both procedures. The unexposed sample is placed on the top of degraded samples for comparison.
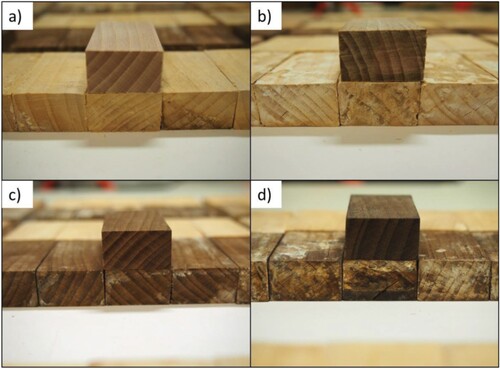
In their work Repič et al. (Citation2022) established that the maximum mass loss occurred in the reference beech (B), and that the two treatments together provided a synergistic effect against fungal decay, which was particularly pronounced in the case of Gloeophyllum trabeum – the mass loss for B was 41.5 ± 2.5%, while after both treatments (BT Ca) it was only 5.7 ± 1.0%.
A similar trend can be observed between the moisture content of the wood and its mass loss. The improved durability of the mineralised samples could also be attributed to the increased pH values (on average the pH of mineralised beech was 2.5 higher than that of non-mineralised beech). The results of fungal durability were also confirmed by microscopic analysis, described in following chapter.
Microscopic analysis
SEM images of the samples before and after exposure to GT fungi are shown in and , respectively. As can be seen in all the images in , some cracks occurred, mainly in the cell walls of the fibres and between the ray cells and the axial elements. These cracks are partly attributed to the way in which the samples were prepared, the harsh drying before SEM observation and the vacuum drying of the samples during SEM observation. The main cause of this structural damage is likely to be the surface tension forces of the water during sample drying, although the use of low vacuum settings reduces the likelihood of cracking (Turkulin et al. Citation2005, Das Murtey and Ramasamy Citation2016). It can be seen by comparing and that the samples have more cracks and distortions after exposure to fungi (), which is to be expected, as the samples are degraded. Brown rot fungi caused a thinning of the cell wall and degradation of cellulose (Schmidt Citation2006). Degraded wood is therefore very brittle and can easily be destroyed during sample preparation.
Figure 3. SEM images of the (a) reference beech, (b) mineralised beech, (c) thermally modified beech, and (d) thermally modified and mineralised beech.
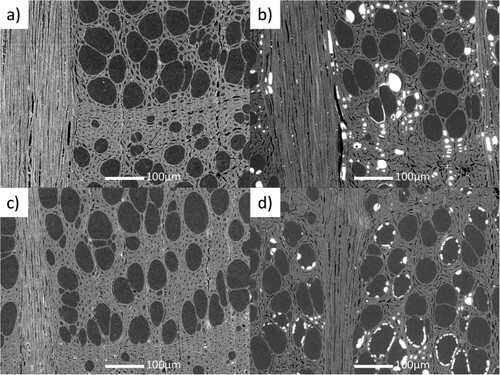
Figure 4. SEM images of samples after exposure to fungi in: (a) the reference beech, (b) the mineralised beech, (c) the thermally modified beech, and (d) the thermally modified and mineralised beech.
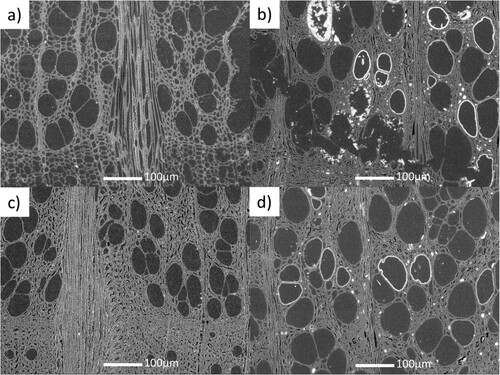
The epoxy resin used to prepare the samples for SEM analysis filled most of the empty vessels. It did not, however, manage to penetrate all the fibres of the reference beech wood (a), which is not surprising given that Zelaya-Lainez et al. (Citation2019) found that embedding wood with resin does not necessarily lead to the pores being completely filled. It should be remembered that beech wood belongs to the group of easily treatable wood species; impregnation of wood species such as Norway spruce would, therefore, be even more challenging. Better penetration of the resin was observed in the TM beech, where it penetrated even into the fibres (c), which could be ascribed to the degradation of tyloses and new micro cracks in the cell wall resulting from the TM process, as reported by Awoyemi and Jones (Citation2011). It can be concluded that TM has a positive effect on impregnability, presumably due to increased permeability (Zelinka et al. Citation2022). TM was therefore used in the study in question to increase the uptake of mineralising chemicals. This is in line with the results from Bender et al. (Citation2022), who found that thermal modification can also have the side effect of increasing impregnability, due to the reduced density of TM wood. The increase in permeability resulting from TM, however, strongly depends on the type of wood species used and the process of thermal modification, predominantly with respect to the modification temperature (Bender et al. Citation2022). It can be seen in b and d that the epoxy resin does not fill all the voids in the mineralised sample. The mineralisation could, to some extent, cause a bulking effect, hindering impregnability as the CaCO3 particles become deposited around or distributed within the vessels (b and d) (Pondelak et al. Citation2021b). By comparing and , it can also be concluded that exposure to fungi generally improved the epoxy impregnability of the samples in this study. This is consistent with the reference data, as fungi can be used to incise the wood prior to impregnation (Thaler et al. Citation2012).
Before fungal exposure, the anatomical characteristics of the reference beech are easily distinguished (vessels, fibres, and parenchyma rays) (a). It is known that TM at high temperatures can lead to collapse, longitudinal fractures in the vessels, deformation of the fibres, radial cracks and merging of the earlywood vessels (Welzbacher et al. Citation2011). The afore-mentioned degradation processes were not very pronounced in the present study. TM resulted in a thinning of the cell walls (c) and opening of the pits, which allows for better impregnability (Awoyemi and Jones Citation2011).
After mineralisation carbonate crystals are found throughout the sample cross-sections, exactly in vessels, parenchyma rays, and also fibres (b and d; b and d). The calcium carbonate forms spherical vaterite crystals of varying sizes (Pondelak et al. Citation2021b), which are attached to the cell wall around the perimeter of the vessels or bulk the cross-section of the cell (b and d). It appears that thermal modification has a negligible effect on mineralisation, as the total amount of carbonates incorporated into the wood structure is roughly the same for both the B Ca and BT Ca samples.
Severe degradation of the reference beech sample can be observed following exposure to fungi (a). The degradation and thinning of the cell walls can be seen in the axial elements as well as in the parenchyma rays. The most significant reduction is seen in the thickness of the fibre cell wall (a). A similar but less pronounced degradation is observed in the TM beech samples, where a stronger deformation of the axial elements is visible (c).
The degradation was less pronounced in the mineralised samples. The damage (crack) seen in b is not exclusively due to fungal degradation, but was caused during sample preparation. It is clearly visible in the fibre cells that the cell wall of the mineralised sample is thicker than the reference sample, which indicates slower degradation by the fungi. It can be observed (b) that the thin cell walls between the vessels are degraded more than the thick cell walls of the fibres. Similar degradation of the thin cell walls can also be observed in the TM sample (c). The samples treated with both procedures resulted in the best fungal resistance of all the samples tested (d). In these samples, no degradation can be seen in the fibres and minimal degradation can be observed in the thin cell walls of the vessels. It can be concluded that the reference sample was most degraded by fungi (a), followed by the TM sample (c), while the sample treated with both processes (d) performed best, followed by the mineralised sample (b).
The crystal structure of the calcium carbonate in the mineralised samples changed from vaterite (b and d) to calcium oxalate hydrate (b and d) during exposure to fungi and was also translocated. This phenomenon has been described previously by Repič et al. (Citation2022) and is attributed to the fungi secreting oxalic acid and precipitating calcium oxalate (Connolly et al. Citation1999, Guggiari et al. Citation2011). This conversion of the crystal structure was not the only difference observed. b and d shows that the cell walls of the vessels look as if they are internally lined with carbonates, although initially only spherical particles were found. After 16 weeks of exposure to fungi the characteristic vaterite crystals were nowhere to be found.
Fire properties
shows the heat release profiles during burning from cone calorimeter experiments on all the samples investigated. The ignition times measured and simulation results, THR600s and FIGRA values obtained from the profiles in are further listed in . The ignition time was evaluated as the time between the beginning of an exposure to radiation and the appearance of a flame. The simulated parameter, THR600s, represents the total heat released in the first 600 s of the test, while the parameter FIGRA is the fire growth rate. Both parameters are crucial for classifying a material's reaction to fire. Materials with lower THR600s and FIGRA values have better fire properties and are subsequently categorised into a better reaction to fire class. A longer ignition time can also be considered as an indicator of improved fire properties.
Figure 5. Heat release profiles of the reference beech (B), thermally modified beech (BT), mineralised beech (B Ca) and beech modified by both methods (BT Ca), as determined by cone calorimetry; the respective curves represent the average of five samples.
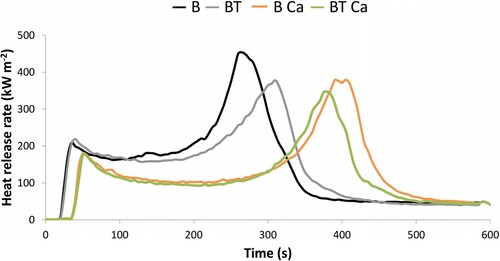
It can be seen that B and BT burn the fastest and that BT releases more heat during burning, while mineralisation shifts the ignition time and reduces the overall amount of heat released (, ). The longest ignition times were measured in samples B Ca and BT Ca (approx. 46 s), which were almost twice as long as those for B and BT samples. Looking at the THR600s values of the samples, it can also be seen that significantly less heat was released when burning the mineralised beech wood compared to the reference or thermally modified beech wood. The lowest heat release occurred in the beech wood treated with both procedures (BT Ca). The same trend can be observed for the fire growth index (FIGRA). Due to the inhibitory effect of moisture on wood burning, the poorer fire properties (shorter ignition time, higher values of THR600s and FIGRA) of BT are attributed to its low equilibrium moisture content ().
Although only mineralisation was previously shown to significantly improve the fire properties of beech wood (Pondelak et al. Citation2021a, Citation2021b), the beech wood subjected to the combined processes of thermal modification and mineralisation (BT Ca) showed the best reaction to fire, as reflected in all three parameters studied.
The longest ignition time, the lowest heat released, and the lowest FIGRA were all found in the BT Ca wood. All parameters investigated were significantly improved compared to the reference wood; ignition time was prolonged by approximately 17 s, while THR600s was reduced by approximately 18 MJ and FIGRA by approximately 331 Ws−1. Mineralisation and thermal modification therefore have a combined or even synergistic effect on improving the fire properties of beech wood. It can be concluded that, with respect to the fire properties of the wood, mineralisation is more efficient on thermally modified wood than it is on unmodified wood.
Mechanical properties
shows the density and porosity measurements of the oven dry samples and the mechanical properties of the materials tested. As can immediately be seen from , the density of the mineralised wood is higher than that of the reference or TM wood. The densities of B and BT were approx. 595 kg/m3, while B Ca and BT Ca have higher density. The higher or comparable density of B and BT is in part due to the variability of the wood. In addition, after TM, there is often more shrinkage as the OH groups are more tightly connected. As the dimensions decrease, the wood density often increases in beech wood (Humar et al. Citation2020). It can be concluded that the porosity of the wood is reduced by mineralisation (). The density measured in the reference samples is in accordance with data for beech wood previously published by Fengel and Wegener (Citation1984) (490–880 kg/m3).
One might expect that mineralisation would increase the hardness of the wood, but this effect was not particularly significant (). The highest hardness was found in the reference sample (approx. 38.5 MPa), while mineralisation and thermal modification slightly decreased the hardness of the wood to approx. 35.5 MPa, and a very similar hardness was measured in the samples treated by both processes. Lower hardness values after thermal modification compared to the reference were also reported by Sedlar et al. (Citation2019). It should be noted that the difference in hardness values are all within the standard deviation of the mean. The hardness of the reference sample measured in this study is slightly higher than that measured by Beqo et al. (Citation2015) (27.7–33.4 MPa) and Wagenführ (Citation2007) (34 MPa), but is within the range specified in the standard (28–41 MPa) (CEN Citation2020c) for reference beech wood.
The modulus of elasticity (MOE), the modulus of rupture (MOR) and the strain at break for each of the samples are also listed in . The modulus of elasticity is comparable in samples B and BT (approx. 10 000 MPa), while the mineralised samples have a slightly lower modulus of elasticity (B Ca = 7450 ± 2920 MPa and BT Ca = 9470 ± 512 MPa), but the results are all within standard deviations of the mean. The MOE values for the reference beech wood are in line with the results published by Badescu (Citation2013). Similarly, the modulus of rupture also decreased following all types of modification. The MOR was highest in the reference (B) sample, but decreased slightly following mineralisation (B Ca). MOR also varies greatly according to the sample itself. Gašparik and Gaff (Citation2015) reported that the MOR ranges from about 140 MPa to 175 MPa in reference beech, while Badescu (Citation2013) found values between 77 and 115 MPa. It is evident that the thermal modification also significantly lowers the modulus of rupture, with similar results having been reported previously by Arnold (Citation2010). We can conclude that both methods of modification influence the mechanical properties of the wood.
Conclusion
This study has demonstrated the combined effect of two discrete modification methods, thermal modification and mineralisation with soluble calcium compounds, which were combined to improve the reaction to fire and biological durability of European beech wood (Fagus sylvatica). Although only mineralisation significantly improves beech wood’s reaction to fire, the best response to fire was achieved by combining the two processes. The best results regarding fire properties were found in wood treated by both processes. All parameters investigated were significantly improved compared to the reference wood; ignition time was considerably prolonged while total heat released and fire growth rate index were considerably reduced. A similar combined effect of the two processes was also observed with respect to the wood’s fungal durability. Improving fire performance helps to increase the use of wood in buildings. Regarding findings in this study the potential use of modified wood has been suggested: as an internal floor or wall panelling or as an external building envelope or cladding, since its durability is improved as well. However, large-scale tests are definitely needed in this area to determine the behaviour of this material in a real-life application.
Furthermore, the effect of the combination of the two modification processes on the mechanical properties was also considered, which is particularly important when modified wood would be used for structural purposes. Results from the Brinell hardness and 3-point bending tests showed that both modification processes can impair the mechanical properties of the wood. There was little difference in the hardness and modulus of elasticity between the reference beech wood and that modified by both processes, while the modulus of rupture appears to be significantly reduced after all types of modification. More research is needed in the area of mechanical properties before suggested modified wood can be used for structural purposes. In particular, it would be useful to identify the reasons for the deterioration in mechanical properties of mineralised wood and their impact at a broader scale rather than just at laboratory level.
Based on the favourable fire properties, the improved resistance to decay fungi and the ecological acceptability of the two modification methods applied (mineralisation and thermal modification), it can be concluded that such wood-inorganic composites have great potential for use in a variety of non-structural applications.
Author contributions
Conceptualisation: RR, AP, ASŠ; Data curation: RR, MH. NK, AP; Formal analysis: RR, MH, NK, DK; Funding acquisition: MH, ASŠ; Investigation: RR, AP, DK, NK; Methodology: MH, FK, DK; Project administration: ASŠ; Resources: ASŠ, MH; Supervision: ASŠ, MH; Validation: AP, DK, MH, ASŠ, FK, NK; Visualisation: RR, AP; Writing – original draft: RR; Writing – review & editing: RR, AP, DK, MH, ASŠ, FK, NK.
Preprint/AOM citation
This manuscript has been posted on Research Square as a preprint (AOM).
Rožle REPIČ, Andreja PONDELAK, Davor KRŽIŠNIK et al. Environmentally friendly protection of European beech wood (Fagus sylvatica) against fire and fungal decay using a combination of thermal modification and mineralisation. 23 February 2023, PREPRINT (Version 1) available at Research Square [https://doi.org/10.21203/rs.3.rs-2612478/v1]
Acknowledgements
We are very much obliged to Catherine Earles for proof-reading the English manuscript.
Disclosure statement
No potential conflict of interest was reported by the author(s).
Additional information
Funding
References
- Ali, S., Hussain, D. S. A. and Tohir, M. Z. M. (2019) Fire test and effects of fire retardant on the natural ability of timber: a review. Pertanika Journal of Science and Technology, 27(2), 867–895.
- Ammann, S., Schlegel, S., Beyer, M., Aehlig, K., Lehmann, M., Jung, H. and Niemz, P. (2016) Quality assessment of glued ash wood for construction engineering. European Journal of Wood and Wood Products, 74(1), 67–74. doi:10.1007/s00107-015-0981-2
- Arnold, M. (2010) Effect of moisture on the bending properties of thermally modified beech and spruce. Journal of Materials Science, 45, 669–680. doi:10.1007/s10853-009-3984-8
- Awoyemi, L. and Jones, I. P. (2011) Anatomical explanations for the changes in properties of western red cedar (Thuja plicata) wood during heat treatment. Wood Science and Technology, 45, 261–267. doi:10.1007/s00226-010-0315-9
- Badescu, L. A. M. (2013) Static bending strength and modulus of elasticity in static bending along the height of beech wood (Fagus sylvatica L.) obtained from forest thinning. Recent Advances in Engineering Mechanics, Structures and Urban Planning (Proceedings of the 6th International Conference on Engineering Mechanics, Structures, Engineering Geology (EMESEG ‘13) 20-22 February, Cambridge, UK). Published in Mathematics and Computers in Science and Engineering, series 8:21-26 ISSN:2227-4588 ISBN:978-1-61804-165-4.
- Bender, T., Munk, C., Pfreim, A., Damay, J., Fredon, E., Rémond, R., Méausoone, P. J., Akong, F. O., Gérardin, P. and Jousserand, M. (2022) Influence of Thermal Modification on the impregnability of Beech (Fagus sylvatica [L.]) and European Maple (Acer pseudoplatanus [L.]). Proceedings of the 10th European Conference on Wood Modification (ECWM10) 2022, Nancy, France.
- Beqo, S., Lato, E., Thoma, H. and Quku, D. (2015) The determination of brinnell hardness for beech wood (Fagus sylvatica L) in Albania. International Journal of Current Engineering and Technology, 5(6), 3593–3595.
- Boonstra, M. J., van Acker, J., Kegel, E. and Stevens, M. (2007) Optimisation of a two-stage heat treatment process: durability aspects. Wood Science and Technology, 41, 31–57. doi:10.1007/s00226-006-0087-4
- Brus, R. (2012) Drevesne vrste na slovenskem (ENG: Tree species in Slovenia) (2nd edition). Ljubljana: Self-published).
- Čekovska, H., Gaff, M., Osvald, A., Kačik, F., Kubš, J. and Kaplan, L. (2017) Fire resistance of thermally modified spruce wood. BioResources, 12(1), 947–959.
- CEN (2019) EN 13501-1 Fire Classification of Construction Products and Building Elements - Part 1: Classification Using Data from Reaction to Fire Tests (Brussels: European committee for standardisation).
- CEN (2020a) EN 113-1 Wood Preservatives - Test Method for Determining the Protective Effectiveness Against Wood Destroying Basidiomycetes - Determination of the Toxic Values (Brussels: European committee for standardisation).
- CEN (2020b) EN 13823 Reaction to Fire Tests for Building Products - Building Products Excluding Floorings Exposed to the Thermal Attack by a Single Burning Item (Brussels: European committee for standardisation).
- CEN (2020c) EN 1534 Wood Flooring and Parquet - Determination of Resistance to Indentation – Test Method (Brussels: European committee for standardisation).
- Connolly, J. H., Shortle, W. C. and Jellison, J. (1999) Translocation and incorporation of strontium carbonate derived strontium into calcium oxalate crystals by the wood decay fungus Resinicium bicolor. Canadian Journal of Botany, 77, 179–187. doi:10.1139/b99-018
- Das Murtey, M. and Ramasamy, P. (2016) Sample preparations for scanning electron microscopy – life sciences. In M. Janecek, and R. Kral (eds.), Modern Electron Microscopy in Physical and Life Sciences (Rijeka: IntechOpen). 161–185. doi:10.5772/61720
- Dunningham, E. and Sargent, R. (2015) Review of New and Emerging International Wood Modification Technologies (Melbourne: Forest & Wood Products of Australia).
- Eder, M., Schäffner, W., Burgert, I. and Fratzl, P. (2021) Wood and the activity of dead tissue. Advanced Materials, 33, doi:10.1002/adma.202001412
- Esteves, B. M. and Pereira, H. M. (2009) Wood modification by heat treatment: A review. BioResources, 4(1), 370–404. doi:10.15376/biores.4.1.Esteves
- Fengel, D. and Wegener, G. (1984) Wood: Chemistry, Ultrastructure, Reactions (Berlin, Germany: Walter de Gruyter).
- Gašparik, M. and Gaff, M. (2015) Influence of densification on bending strength of beech wood. Wood research, 60(2), 211–218.
- Gennari, E., Picchio, R. and Lo Monaco, A. (2021) Industrial heat treatment of wood: study of induced effects on ayous wood (Triplochiton scleroxylon K. Schum). Forests, 12(6), 730. doi:10.3390/f12060730
- Gorišek, Ž (2009) Les - Zgradba in lastnosti. Njegova variabilnost in heterogenost. (ENG: Wood - Structure and properties. Its variability and heterogeneity.). (Ljubljana: Biotehniška fakulteta, Oddelek za lesarstvo).
- Gryc, V., Vavrčík, H. and Gomola, Š (2008) Selected properties of European beech (Fagus sylvatica L.). Journal of Forest Science, 54, 418–425. doi:10.17221/59/2008-JFS
- Guggiari, M., Bloque, R., Aragno, M., Verrecchia, E., Job, D. and Junier, P. (2011) Experimental calcium-oxalate crystal production and dissolution by selected wood-rot fungi. International Biodeterioration & Biodegradation, 65(6), 803–809. doi:10.1016/j.ibiod.2011.02.012
- Guo, H., Luković, M., Mendoza, M., Schlepütz, C. M., Griffa, M., Xu, B., Gaan, S., Herrmann, H. and Burgert, I. (2019) Bioinspired struvite mineralization for fire-resistant wood. ACS Applied Materials & Interfaces, 11(5), 5427–5434. doi:10.1021/acsami.8b19967
- Hill, C. A. S. (2006) Wood Modification: Chemical, Thermal and Other Processes (NJ, USA: John Wiley and Sons).
- Huang, L., Yao, X., Huang, Y. and Wang, Q. (2018) The preparation of CaCO3/wood composites using a chemical precipitation method and its flame-retardant and mechanically beneficial properties. Bioresources, 13(3), 6694–6706.
- Humar, M., Repič, R., Kržišnik, D., Lesar, B., Cerc Korošec, R., Brischke, C., Emmerich, L. and Rep, G. (2020) Quality control of thermally modified timber using dynamic vapor sorption (DVS) analysis. Forests, 11, 666. doi:10.3390/f11060666
- ISO (2014) ISO 13061-3 Physical and Mechanical Properties of Wood — Test Methods for Small Clear Wood Specimens - Part 3: Determination of Ultimate Strength in Static Bending (Geneva, Switzerland: International Organization for Standardization).
- ISO (2018) ISO 5660-1 Reaction-to-Fire Tests - Heat Release, Smoke Production and Mass Loss Rate - Part 1: Heat Release Rate (Cone Calorimeter Method) and Smoke Production Rate (Dynamic Measurement) (Geneva, Switzerland: International Organization for Standardization).
- Kollman, F. (1951) Technologie des Holzes und der Holzwerkstoffe (2nd Ed). Berlin., Germany: SpringerVerlag).
- Lebow, S. T. and Winandy, J. E. (1999) Effect of fire-retardant treatment on plywood pH and the relationship of pH to strength properties. Wood Science and Technology, 33, 285–298. doi:10.1007/s002260050116
- Lesar, B., Straže, A. and Humar, M. (2011) Sorption properties of wood impregnated with aqueous solution of boric acid and montan wax emulsion. Journal of Applied Polymer Science, 120(3), 1337–1345. doi:10.1002/app.33196
- Martín-Martínez, M. J. (2002) Adhesion science and engineering. Adhesion Science and Engineering, 2, 573–675. doi:10.1016/b978-044451140-9/50013-5
- Maurice, S., Coroller, L., Debaets, S., Vasseur, V., Le Floch, G. and Barbier, G. (2011) Modelling the effect of temperature, water activity and pH on the growth of Serpula lacrymans. Journal of Applied Microbiology, 111(6), 1436–1446. doi:10.1111/j.1365-2672.2011.05161.x
- Merk, V., Chanana, M., Gaan, S. and Burgert, I. (2016) Mineralization of wood by calcium carbonate insertion for improved flame retardancy. Holzforschung, 70(9), 867–876. doi:10.1515/hf-2015-0228
- Merk, V., Chanana, M., Keplinger, T., Gaand, S. and Burgert, I. (2015) Hybrid wood materials with improved fire retardance by bio-inspired mineralisation on the nano- and submicron level. Green Chemistry, 17, 1423–1428. doi:10.1039/C4GC01862A
- Meyer-Veltrup, L., Brischke, C., Alfredsen, G., Humar, M., Flæte, P. O., Isaksson, T., Brelid, P. L., Westin, M. and Jermer, J. (2017) The combined effect of wetting ability and durability on outdoor performance of wood: development and verification of a new prediction approach. Wood Science and Technology, 51(3), 615–637. doi:10.1007/s00226-017-0893-x
- Militz, H. and Altgen, M. (2014) Acs symposium series. Deterioration and Protection of Sustainable Biomaterials, 1158, 269–285. doi:10.1021/bk-2014-1158.ch016
- Moya, R., Gaitan-Alvarez, J., Barrocal, A. and Araya, F. (2020) Effect of CaCO3 on the wood properties of tropical Hardwood species from fast-growth plantation in Costa Rica. Bioresources, 15(3), 4802–4822.
- Odppes, G. F., Bulle, C. and Ugaya, C. M. L. (2021) Wood forest resource consumption impact assessment based on a scarcity index accounting for wood functionality and substitutability (WoodSI). The International Journal of Life Cycle Assessment, 26, 1045–1061. doi:10.1007/s11367-021-01880-7
- Ozyhar, T., Tschannen, C., Hilty, F., Thoemen, H., Schoelkopf, J. and Zoppe, J. O. (2021) Mineral-based composition with deliquescent salt as flame retardant for melamine–urea–formaldehyde (MUF)-bonded wood composites. Wood Science and Technology, 55, 5–32. doi:10.1007/s00226-020-01230-0
- Poncsak, S., Kocaefe, D., Younsi, R., Kocaefe, Y. and Gastonguay, L. (2009) Thermal treatment of electrical Poles. Wood Science and Technology, 43, 471–486. doi:10.1007/s00226-009-0243-8
- Pondelak, A., Sever Škapin, A., Knez, N., Knez, F. and Pazlar, T. (2021b) Improving the flame retardancy of wood using an eco-friendly mineralisation process. Green Chemistry, 23, 1130. doi:10.1039/D0GC03852K
- Pondelak, A., Sever Škapin, A., Knez, N., Repič, R., Škrlep, L., Pazlar, T., Knez, F. and Legat, A. (2021a) A Process of Wood Mineralisation Using Acetoacetate Solutions to Improve the Essential Properties of Wood. Patent Number: SI25944A 2021-06-30 (Ljubljana: The Slovenian Intellectual Property Office).
- Raspor, P., Smole-Možina, S., Podjavoršek, J., Pohleven, F., Gogala, N., Nekrep, V. F. and Hacin, J. (1995) ZIM: Collection of industrial microorganisms. Catalogue of Cultures (Ljubljana: University of Ljubljana, Biotechnical Faculty).
- Rep, G. and Pohleven, F. (2002) Modifikacija lesa - obećavajuća metoda za zaštitu drva (ENG: Wood modification - a promising method for wood preservation). Drvna industrija, 52(2), 71–76.
- Rep, G., Pohleven, F. and Košmerl, S. (2012) Development of the industrial kiln for thermal wood modification by a procedure with an initial vacuum and commercialisation of modified Silvapro wood. in: Proceedings of the 6th European Conference on Wood Modification, 11–17.
- Repič, R., Pondelak, A., Kržišnik, D., Humar, M. and Sever Škapin, A. (2022) Combining mineralisation and thermal modification to improve the fungal durability of selected wood species. Journal of Cleaner Production, 351, 131530. doi:10.1016/j.jclepro.2022.131530
- Sandberg, D., Kutnar, A. and Mantanis, G. (2017) Wood modification technologies - a review. Iforest - Biogeosciences and Forestry, 10, 895–908. doi:10.3832/ifor2380-010
- Schmidt, O. (2006) Wood and tree fungi: Biology, damage, protection, and use. In D. Czeschlik (ed.), Wood and TreeFungi: Biology, Damage, Protection, and Use. (Heidelberg, Germany: Springer Berlin). doi:10.1007/3-540-32139-X
- Sedlar, T., Sinković, T., Perić, I., Jarc, A., Stojnić, S. and Šefc, B. (2019) Tvrdoća toplinski modificirane bukovine i grabovine. Šumarski List, 143(9-10), 433–433. doi:10.31298/sl.143.9-10.4
- Singh, T. and Singh, A. P. (2012) A review on natural products as wood protectant. Wood Science and Technology, 46, 851–870. doi:10.1007/s00226-011-0448-5
- Skaravelis, M. and Mantanis, G. I. (2013) Physical and mechanical properties of beech wood harvested in the Greek public forests. Wood Research, 58(1), 123–130.
- Škrlep, L., Pondelak, A. and Sever Škapin, A. (2014) Postopek utrjevanja poroznih mineralnih gradbenih materialov in uporaba raztopin kalcijevega acetoacetata v ta namen (ENG: The Consolidation of Porous Mineral Building Materials and the use of Calcium Acetoacetate Solutions for This Purpose). Patent Number: Si 24392 (A) (Ljubljana: The Slovenian Intellectual Property Office).
- Taghiyari, H. R. (2012) Fire-retarding properties of nano-silver in solid woods. Wood Science and Technology, 46, 939–952. doi:10.1007/s00226-011-0455-6
- Tao, Y., Li, P., Cai, L. and Shi, S. Q. (2019) Flammability and mechanical properties of composites fabricated with CaCO3-filled pine flakes and Phenol Formaldehyde resin. Composites Part B: Engineering, 167, 1–6. doi:10.1016/j.compositesb.2018.12.005
- Thaler, N., Lesar, B., Kariž, M. and Humar, M. (2012) Bioincising of Norway spruce wood using wood inhabiting fungi. International Biodeterioration & Biodegradation, 68, 51–55. doi:10.1016/j.ibiod.2011.11.014
- Thybring, E. E., Kymäläinen, M. and Rautkari, L. (2018) Moisture in modified wood and its relevance for fungal decay. iForest - Biogeosciences and Forestry, 11, 418–422. doi:10.3832/ifor2406-011
- Turkulin, H., Holzer, L., Richter, K. and Sell, J. (2005) Application of the ESEM technique in wood research: part I. optimization of imaging parameters and working conditions. Wood and Fiber Science, 37(4), 552–564.
- Wagenführ, R. (2007) Holzatlas: mit zahlreichen Abbildungen (ENG = Atlas of Wood: with Numerous Illustrations) (Leipzig: Fachbuchverlag).
- Welzbacher, C. R., Rassam, G., Talaei, A. and Brischke, C. (2011) Microstructure, strength and structural integrity of heat-treated beech and spruce wood. Wood Material Science and Engineering, 6(4), 219–227. doi:10.1080/17480272.2011.622411
- Yona, A. M. C., Žigon, J., Pavlič, M. and Petrič, M. (2021) Potentials of silicate-based formulations for wood protection and improvement of mechanical properties: A review. Wood Science and Technology, 55, 887–918. doi:10.1007/s00226-021-01290-w
- Zelaya-Lainez, L., Lahayne, O., Balduzzi, G. and Hellmich, C. (2019) Micromechanics of non-embedded spruce wood: Novel polishing and indentation protocol. In R. Zemick, and J. Krystek (eds.), Conference Proceedings 157-158. 36th Danubia Adria Symposium on Advances in Experimental Mechanics (Pilsen, Czech Republic: University of West Bohemia). 157–158.
- Zelinka, S. L., Altgen, M., Emmerich, L., Guigo, N., Keplinger, T., Kymäläinen, M. and Thybring, E. E. (2022) Review of wood modification and wood functionalization technologies. Forests, 13(7), 1004. doi:10.3390/f13071004