ABSTRACT
The demand for wooden raw materials is constantly on the rise. Meeting this interest requires developing new timber supply chains, tackling illegal trade, using timber more efficiently, and enhancing the service life of wood-based products. From this point of view, sweet chestnut wood can be successfully used, alone or mixed with poplar, to manufacture Oriented Strand Board (OSB). This article assesses the fungal resistance of OSB/3 (load-bearing boards for use in humid conditions) made with different fractions of poplar (P) and sweet chestnut (SC) wood, in order to take advantage of the protective action of the tannins contained in the latter. Four types of OSB/3 were manufactured on an industrial scale: 100% P; 80% P and 20% SC; 50% P and 50% SC; 100% SC. The durability of the experimental boards was determined in accordance with the EN 113-3 standard (Citation2023). No significant differences in mass loss were found for OSB/3 with different sweet chestnut wood fractions. Instead, the mass loss of these panels was significantly lower (i.e. fungal resistance was higher) than that of the poplar OSB/3 and poplar plywood tested for comparison. Based on the results, the use of sweet chestnut wood has interesting potential for the manufacturing of OSB/3 made with adequate gluing quality, suitable for applications in environments where fungal decay may occur.
Introduction
The demand for wooden raw materials is constantly on the rise. This is largely due to the fact that wood is a renewable natural resource with a lower environmental impact than many alternative raw materials. Meeting the ever-growing interest in wood requires developing new timber supply chains, using legal timber more efficiently, and enhancing the service life of wood-based products. Clearly, these are not suitable for every application, so it is important to manufacture products that are fit for purpose and have an adequate service life in order to extend their associated benefits (Van Acker et al. Citation2023).
Wood-based panels are a broad category of products manufactured to overcome some of the disadvantages of solid wood: they offer larger dimensions and different shapes, have greater homogeneity, enable different woods to be mixed, can be made from ‘low quality’ raw material, etc. (Theomen et al. Citation2010). Among wood-based panels, Oriented Strand Board (OSB) is widely appreciated as a performing and cost-effective panel for the construction sector. OSB production in EU27/UK/EFTA amounted to around 6.4 million m3 in 2022, representing 11% of the wood-based panels produced in that year (EPF Citation2023). This is in line with the growing interest in wood from the construction sector, the main destination for OSB.
OSB is typically made of coniferous wood or by mixing it with a minor fraction of hardwood. However, the production of 100% poplar OSB started in Italy in 2012 by a manufacturer with a production capacity of 130.000 m3 per year. Over the years this panel has secured a stable market share (Cetera et al. Citation2018) and contributed to supporting the national supply chain of poplar wood. However, despite the growing interest in poplar cultivation in Italy (Zanuttini et al. Citation2020a, Zanuttini et al. Citation2021), some doubts have recently been raised about the consistency of poplar wood supply from national plantations.
Sweet chestnut is one of the most widespread species in Italy (about 800 000 ha, Tabacchi et al. Citation2007) and in Europe (2.5 million ha, Conedera et al. Citation2016). Sweet chestnut could ensure a consistent supply of raw material, capable of providing the large volumes of wood required at the industrial level for the manufacturing of OSB. In this respect, Zanuttini et al. (Citation2020b) assessed the physico-mechanical properties of sweet chestnut and mixed poplar-sweet chestnut OSB, with promising results in terms of feasibility. The quality of Italian sweet chestnut timber, mostly coming from coppices, is actually quite limited (Militz et al. Citation2003, Zanuttini et al. Citation2020b) in terms of the size of the assortments and the frequent occurrence of ring shake. Nevertheless, sweet chestnut wood is suitable for the production of OSB, as this panel does not require particularly demanding raw material features. Overall, the use of sweet chestnut for the manufacturing of OSB can reduce the pressure on national poplar plantations while exploiting the wide potential of sweet chestnut wood, and improving the overall performance of such panels.
Sweet chestnut (Castanea sativa Mill.) wood is known for its high durability and favorable anisotropy ratio. For centuries, it has been widely used for heavy-duty outdoor equipment such as poles, pile dwellings, and construction in general (Adua Citation2000, Gérard et al. Citation2011, Chavenetidou et al. Citation2020).
Sweet chestnut wood contains a high proportion of phenolic extractives, mainly ellagitannins, that are responsible for both its fungal durability and dimensional behavior (Eichhorn et al. Citation2017, Chavenetidou et al. Citation2020). This timber is generally classified as durable against fungi (Durability Class, DC 2) (Gérard Citation2011; EN 350, Citation2016) to very durable when its evaluation includes only laboratory tests (EN 350, Citation2016). Militz et al. (Citation2003) evaluated the durability of sweet chestnut wood from Italian coppice stands through laboratory and field tests. Laboratory evaluation against Basidiomycete fungi allowed this timber to be classified as durable (DC 2), although a wide variability (from very durable “DC 1” to non-durable “DC 5”) has been observed in a few test samples. However, no significant difference has been observed between mature and juvenile wood.
The average good durability of sweet chestnut wood can be an added value for the manufacturing of OSB/3, defined by EN 300 (Citation2006) as load-bearing boards for use in humid conditions bonded with suitable adhesive systems. To date, in fact, the use of OSB in exterior environment is not envisaged by EN 300 (Citation2006). This standard prescribes the use of OSB only in dry or humid conditions, which refer to Service Classes 1 and 2 as described in EN 1995-1-1 (Citation2005), and reflect indicatively the biological risks described in Use Classes (UC) 1 and 2 according to EN 335 (Citation2013). Nonetheless, OSB is used in some non-structural, exterior applications such as hoarding () where long service life is not required (BM TRADA Citation2023).
Over the past years, various studies investigated the improvement of the durability of OSB through the use of chemicals or different modifications. For instance, Cai et al. (Citation2020) studied the effect of β-cyclodextrin-allyl isothiocyanate complex as a natural preservative for OSB; Papadopoulos (Citation2021) investigated the decay resistance of acetylated OSB in the ground; Okino et al. (Citation2007) assessed the durability of thermally-treated cypress OSB. Another way to improve the service life of OSB in hazardous environment is to use durable wood species, either alone or in optimal combinations with non-durable species (Amusant et al. Citation2009).
The present study analyzes the fungal resistance against Basidiomycetes of sweet chestnut and mixed chestnut-poplar OSB/3 manufactured on an industrial scale with a gluing quality suitable for use in humid conditions. The objective was to assess to what extent the use of sweet chestnut wood, alone or mixed with non-durable poplar wood (DC 5 EN 350, Citation2016, Spavento et al. Citation2019), improves the fungal resistance of such boards. In fact, the use of any wood-based panel in humid or exterior conditions requires both moisture-resistant gluing and adequate fungal resistance and depends on the expected service life.
Materials and methods
The raw material consisted of logs of poplar I-214 clone (P), from traditionally managed 10–12 years old plantations in Northwestern Italy, and logs of sweet chestnut (SC), of 10–35 cm in diameter and 150–200 cm in length from 40-50-years old coppice stands in Northwestern Italy. Sweet chestnut sapwood is non-durable against fungi (Yurkewich et al. Citation2017). However, the presence of sapwood was considered negligible because once the chestnut logs were debarked, very little or no sapwood remained.
OSB/3 was produced through the process described in detail in Zanuttini et al. (Citation2020b). Briefly, OSB/3 18 mm thick with dimensions of 1250 × 2500 mm was manufactured on an industrial scale (I-PAN S.p.a., Casale Monferrato, Italy). Continuous pressing was at 248 °C at a pressure of 4 MPa, with a line speed of 95 mm/s. A commercial polymeric diphenylmethane diisocyanate (PMDI) resin (5 kg of resin per 100 kg of wood), suited for the manufacturing of OSB/3 according to the EN 300 standard (Citation2006), was used for gluing.
OSB/3 was manufactured by mixing poplar (P) and sweet chestnut (SC) wood. Four OSB/3 typologies were obtained, characterized by different fractions of P and SC, by weight of strands made from freshly cut timber: 100% P; 80% P-20% SC; 50% P-50% SC; 100% SC ( left).
Figure 2. Left: mattress made of poplar and sweet chestnut strands entering the continuous pressing phase. Right: specimens subjected to a durability test with Coniophora puteana according to the EN113-3 standard.
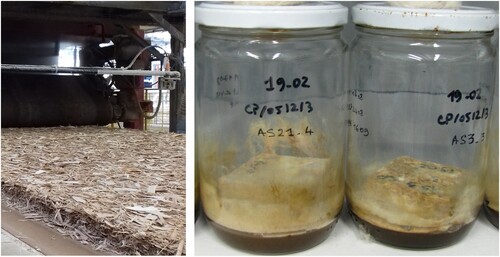
All OSB/3 specimens were cut from ten boards per type that were randomly sampled from the continuous production process. Specimens were randomly cut from boards coming from the same production batch, using EN 326-1 (Citation1994) standard as a reference and the same sampling method for density.
The durability of the above boards was rigorously assessed in accordance with the EN 113-3 standard(Citation2023) ( right), which recently superseded ENV 12038 (2002) from which it was derived. The ENV 12038 (2002) applied to wood-based panels in general terms but was mainly developed with a specific reference to plywood (Van Acker et al. Citation2001). Overall, there has been little research into the use of ENV 12038 (2002) or EN 113-3 (Citation2023) to assess the fungal resistance of OSB (Amusant et al. Citation2009).
In accordance with the EN 113-3 standard (Citation2023), the fungi used were Coniophora puteana (Schumacher ex Fries) Karsten (brown rot); Trametes versicolor (Linnaeus) Quélet and Pleurotus ostreatus (Jacquin ex Fries) Quélet (both white-rots) from the CIRAD mycobank. The same testing was also performed on 18 mm thick poplar (I-214 clone) plywood bonded with urea-melamine-formaldehyde resin (100% PPly), commonly available on the national market. Solid beech wood (B) and Scotch pine sapwood (PiS) were used as controls to check the virulence of each fungal strain. The nominal dimensions of each OSB/3 and poplar plywood specimen were 50 × 50 mm (L × W) × board thickness. The dimensions of the solid wood specimens (B and PiS) were 50 × 25 × 15 mm (L,R,T). Replicates per panel type and per fungus were: 32 for 80% P-20% SC, 50% P-50% SC, 100% SC; 12 for 100% P; 12 for 100% PPly. 10 replicates of B and PiS were used for virulence control.
Prior to the biological testing, all panel samples were preconditioned at 20°C, 65% Relative Humidity (RH) for 12 weeks pre-conditioning (rotating weekly) and maintained under the same conditions for an additional 4 weeks. The density of all specimens was measured at 20°C and 65% RH. The panels and solid wood samples were gamma ray sterilized before the 16-week fungal exposure.
Statistical analysis was performed using IBM SPSS 27.0 software (IBM Corp., Armonk, NY, USA). Following the same approach as in Zanuttini et al. (Citation2020a), differences in durability were investigated using the Kruskal–Wallis non-parametric test, with a Campbell-Skillings stepwise stepdown comparison as a post hoc. Significance was always set at the 0.05 level.
Results and discussion
The results of the fungal decay tests are shown in and . At the end of testing, all specimens had a moisture content >25%, as required by the EN 113-3 standard (Citation2023) to ensure that fungi have adequate growth conditions. For each test modality (material type and fungal strain), indicates the density, durability class based on median mass loss (according to the EN 350, Citation2016) as well as the average mass loss, and the results of the statistical analysis. shows the durability class based on the median mass loss, as well as the durability distribution, and then assesses the variability of resistance against fungi. The number of specimens tested is quite large, so these datasets are relevant to evaluate the decay susceptibility (as well as the associated variability) of the different materials, in order to make a reliable prediction of the service life of these wood-based products (De Windt et al. Citation2013).
Table 1. Density, average mass loss (AML), standard deviation, statistical grouping (a, b, c, d) and durability Class (based on median mass loss values according to the EN 350 standard) for the different fungi used and for solid wood used for virulence control.
Table 2. Median mass loss (MML), durability Class and durability class distribution (in accordance with the EN 350 standadr, Citation2016) for the different fungi used and for the different materials tested.
display the results of the durability test. Significant differences in mass loss were found for C. puteana (P < 0.001), P. ostreatus (P < 0.001), and T. versicolor (P < 0.001).
Figure 3. Mass loss of OSB/3, plywood and solid wood exposed to C. puteana, grouped (a, b, c) based on statistical analysis.
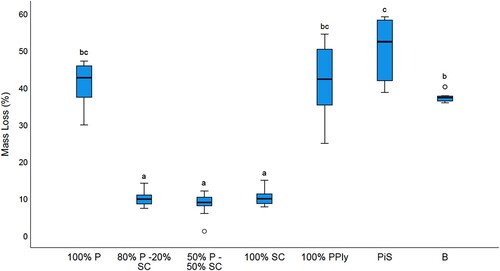
Figure 4. Mass Loss of OSB/3, plywood and solid wood exposed to P. ostreatus, grouped (a, b, c, d) based on statistical analysis.
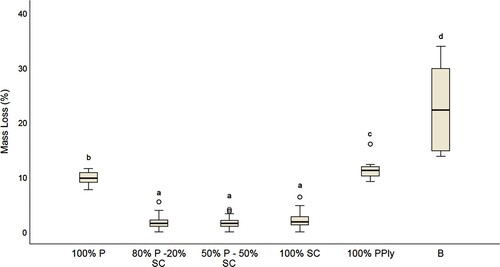
Figure 5. Mass Loss of OSB/3, plywood and solid wood exposed to T. versicolor, grouped (a, b, c) based on statistical analysis.
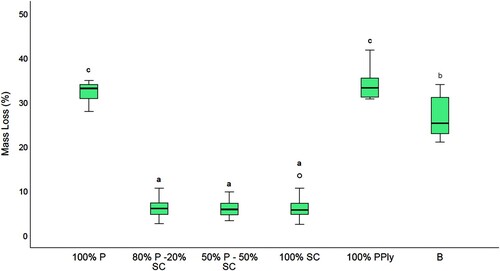
The results of the virulence controls allowed validation of the test: TV and PO showed mean and median mass loss above 20% on beech wood samples; CP showed mean and median mass loss above 30% on both beech (36.2% and 36.9%, respectively) and pine sapwood samples (49.9% and 52.3%, respectively). Overall, CP remains the most discriminating fungal strain. CP has already been reported as the most critical fungal strain for both softwood and hardwood and wood-based materials (Van Acker et al. Citation2003, Faraji Citation2005).
PO is a mandatory fungal strain when testing wood-based panels made of hardwood species for their decay susceptibility (EN 113-3 Citation2023). PO has been reported to be more virulent than CP, while also being rather indifferent to the glue type (Van den Bulcke et al. Citation2011). However, despite the use of vermiculite, and its performance on beech control samples in accordance with the requirements of EN 113-3 (Citation2023), PO was found to be the less active fungal strain. It is therefore questionable in terms of reliability to draw conclusions from the PO degradation results in this work. However, for the boards containing sweet chestnut strands, it can be noted as an indication that the results of the durability test against PO were similar to the results against CP and TV.
Poplar plywood and OSB/3 – 100% P obtained the same durability classification (DC 5 against both CP and TV). This suggests that the change in wood shape and size (veneers/strands) and the glue type (UMF or PMDI), quantity and distribution (in glue lines or mixed with strands) were not relevant.
No statistical difference in mass loss was found among OSB/3 boards with different sweet chestnut wood fractions. After exposure to all the fungi tested, all the panels containing sweet chestnut wood [80%P-20%SC, 50%P-50%SC and 100%SC (group a)] showed lower mass loss than poplar OSB/3 (100% P, groups b and c).
This can be attributed to the fact that sweet chestnut extractives, which typically contain hydrolysable tannins of mixed and complex composition, can be partially solubilized during the production process, due to heat and moisture release, even with a limited process duration (Aires et al. Citation2016, Campo et al. Citation2016, Gagić et al. Citation2019). Such solubilized chestnut tannins can spread through the boards and impregnate the thin poplar strands (Sen et al. Citation2017). The mixed OSB types, containing (1) residual tannins in the chestnut strands and (2) tannin-impregnated poplar strands, benefit from the improved durability against a range of three wood degrading fungi, as a result of the biological and antioxidant effect of tannins (Schultz and Nicholas Citation2002, Tomak and Gonultas Citation2018, Das et al. Citation2020). Based on the results, it seems that 20% of sweet chestnut wood already allows reaching a fungitoxic threshold (Broda Citation2020) and is sufficient to significantly increase the durability of the OSB boards. This can be explained by the high tannin content of sweet chestnut wood, around 8–10% in oven dry weight (Eichhorn et al. Citation2017).
The fact that only 20% (instead of 50% or 100%) of sweet chestnut wood is sufficient to activate the above improvements can be an advantage in terms of industrial feasibility. In fact, given the continuous production process, switching from 100% poplar wood to 100% sweet chestnut wood would result in a relevant fraction of non-marketable boards with mixed, undefined composition and characteristics. The addition of only 20% sweet chestnut timber, instead, can easily be done at the beginning of the production line, thus enabling a constant composition of the boards.
Of note, the new version of the standard EN 460 (2023) “Durability of wood and wood-based products – Guidance on performance” addresses the relationship between biological durability class, Use Class and expected service life. In this context, the better fungal resistance of OSB/3 made of sweet chestnut wood, compared to the same panel made of 100% poplar, allows to hypothesize its future use in a covered exterior (or even exterior) environment. This is on condition that the other technical properties, in particular swelling in thickness, are maintained for the specific use.
Conclusions
OSB/3 made entirely or partially from sweet chestnut wood was found to have a higher natural durability than standard poplar OSB/3. A fraction of 20% of sweet chestnut wood was already sufficient to determine a significant improvement in terms of natural durability, which may represent a relevant added value for potential applications. In addition to the increased durability, the potential use of sweet chestnut wood to manufacture OSB/3 offers other advantages: reduced environmental impact compared to the use of preservatives or treatments; large availability at local level, with limited emissions associated with the transport phase; beneficial socio-economic impact on the territory; securing the supply of raw material at reasonable costs. Also, based on this work and as a future perspective, sweet chestnut tannin extractives could be effectively added as a natural preservative in the glue mix during the manufacturing of poplar OSB/3.
The outcome of this work can be useful to optimize the industrial manufacturing process of OSB/3 made of sweet chestnut wood, alone or mixed with poplar wood. Notably, the normative framework should be adapted by including an OSB/3 type suitable for non-structural use in covered exterior conditions or even in exterior exposure, where swelling of the panel thickness might be admitted.
Disclosure statement
No potential conflict of interest was reported by the authors.
Data availability statement
Data can be required to [email protected].
Additional information
Funding
References
- Adua, M., 2000. The chestnut culture in Italy towards 2000. Ecologia Mediterranea, 26, 15–31. doi:10.3406/ecmed.2000.1888.
- Aires, A., Carvalho, R., and Saavedra, M.J., 2016. Valorization of solid wastes from chestnut industry processing: Extraction and optimization of polyphenols, tannins and ellagitannins and its potential for adhesives, cosmetic and pharmaceutical industry. Waste Management, 48, 457–464. doi:10.1016/j.wasman.2015.11.019.
- Amusant, N., et al., 2009. Biological properties of an OSB eco-product manufactured from a mixture of durable and non-durable species and natural resins. European Journal of Wood and Wood Products, 67, 439–447. doi:10.1007/s00107-009-0335-z.
- BM TRADA, 2023. TimberWISE 4.1 (2023/24), pp. 700. TRADA Bookshop (UK). https://bookshop.trada.co.uk/bookshop/view/fccd3211-37b8-4ec9-b9c7-7fdb2f13b473.
- Broda, M., 2020. Natural compounds for wood protection against fungi – A review. Molecules, 25 (15), 3538. doi:10.3390/molecules25153538.
- Cai, L., et al., 2020. β-Cyclodextrin-allyl isothiocyanate complex as a natural preservative for strand-based wood composites. Composites Part B: Engineering, 196, 108037. doi:10.1016/j.compositesb.2020.108037.
- Campo, M., Pinelli, P., and Romani, A., 2016. Hydrolyzable tannins from sweet chestnut fractions obtained by a sustainable and eco-friendly industrial process. Natural Product Communications, 11 (3), 409–415.
- Cetera, P., et al., 2018. Physico-mechanical properties of thermo-treated poplar OSB. Forests, 9 (6), 345. doi:10.3390/f9060345.
- Chavenetidou, M., Kakavas, K.V., and Birbilis, D., 2020. Shrinkage and swelling of Greek chestnut wood (Castanea sativa Mill.) in relation to extractive presence. Iop Conference Series: Materials Science and Engineering, Volume 908, 5th international Conference on Energy Materials and Applications. 6-9 May 2020, Paris (France). doi:10.1088/1757-899X/908/1/012004.
- Conedera, M., Tinner, W., Krebs, P., de Rigo, D., and Caudullo, G., 2016. Castanea sativa in Europe: distribution, habitat, usage and threats. In J. San-Miguel-Ayanz, D. de Rigo, G. Caudullo, T. Houston Durrant and A. Mauri, eds., European Atlas of Forest Tree Species. Luxembourg: Publ. Off. EU, 78–79.
- Das, A.K., et al., 2020. Review on tannins: Extraction processes, applications and possibilities. South African Journal of Botany, 135, 58–70. doi:10.1016/j.sajb.2020.08.008.
- De Windt, I., et al., 2013. Statistical analysis of durability tests - Part 1: Principles of distribution fitting and application on laboratory tests. The International Research Group on Wood Protection, 44th annual meeting, 16-20/06/2013, Stockholm, Sweden, Doc IRG/WP 13-20504, p. 12.
- Eichhorn, S., et al., 2017. Determination of the phenolic extractive content in sweet chestnut (Castanea sativa Mill.) Wood. Wood Research, 62, 181–196. http://www.woodresearch.sk/cms/determination-of-the-phenolic-extractive-content-in-sweet-chestnut-castanea-sativa-mill-wood/.
- EN113-3:2023, Durability of Wood and Wood-Based Products. Test Method Against Wood Destroying Basidiomycetes. Part 3: Assessment of Durability of Wood-Based Panels. Brussels, Belgium: European Committee for Standardization.
- EN 1995-1-1:2005, Eurocode 5: Design of Timber Structures - Part 1-1: General - Common Rules and Rules for Buildings. Brussels, Belgium: European Committee for Standardization.
- En 300:2006, Oriented Strand Boards (OSB) - Definitions, Classification and Specifications. Brussels, Belgium: European Committee for Standardization.
- EN 326-1:1994, Wood-based Panels - Sampling, Cutting and Inspection - Part 1: Sampling and Cutting of Test Pieces and Expression of Test Results. Brussels, Belgium: European Committee for Standardization.
- EN 335:2013, Durability of Wood and Wood-Based Products – Use Classes: Definitions, Application to Solid Wood and Wood-Based Products. Brussels, Belgium: European Committee for Standardization.
- EN 350:2016, Durability of Wood and Wood-Based Products – Testing and Classification of the Durability to Biological Agents of Wood and Wood-Based Materials. Brussels, Belgium: European Committee for Standardization.
- EPF, 2023. Annual report 2020–2021 oriented strand board. European Panel Federation, Brussels. Available online: https://europanels.org/ [accessed on 02 Oct 2023].
- Faraji, F., 2005. Study on the natural durability of mixed plywood panels composed the durable and non-durable veneers. Life Sciences [q-bio]. ENGREF (AgroParisTech), 2005. English. ffNNT: ff. ffpastel-00001664, pp. 247, https://pastel.archives-ouvertes.fr/pastel-00001664.
- Gagić, T., Knez, Ž,, and Škerget, M., 2019. Hydrothermal hydrolysis of sweet chestnut (Castanea sativa) tannins. Journal of the Serbian Chemical Society, 84, 1–14. doi:10.2298/JSC190711108G.
- Gérard, J., et al., 2011. Tropix 7 (Version 7.5.1) [Computer software]. CIRAD. doi:10.18167/74726F706978.
- Militz, H., Busetto, D., and Hapla, F., 2003. Investigation on fungal durability and sorption properties of Italian Chestnut (Castanea sativa Mill.) from coppice stands. Holz als Roh- und Werkstoff, 61, 133–141. doi:10.1007/s00107-002-0357-2.
- Okino, E.Y.A., Teixeira, D.E., and Del Menezzi, C.H.S., 2007. Post-thermal treatment of oriented strandboard (OSB) made from cypress (Cupressus glauca Lam.). Maderas. Ciencia y Tecnología, 9 (3), 199–210. doi:10.4067/S0718-221X2007000300001.
- Papadopoulos, A., 2021. Fungal durability of acetylated OSB in ground stake test: total decay after 102 months of testing. January 2011. European Journal of Wood and Wood Products, 70, 1–3. doi:10.1007/s00107-011-0547-x.
- Schultz, T.P., and Nicholas, D.D., 2002. Development of environmentally-benign wood preservatives based on the combination of organic biocides with antioxidants and metal chelators. Phytochemistry, 61 (5), 555–560. doi:10.1016/S0031-9422(02)00267-4.
- Sen, S., et al., 2017. Larvicidal activities of some bark and wood extracts against wood-damaging insects. Maderas. Ciencia y Tecnología, 19 (3), 273–284. doi:10.4067/S0718-221X2017005000023.
- Spavento, E., et al., 2019. Susceptibility of Populus x euramericana ‘I-214’ of Spanish origin to xylophagous attacks: durability tests for its possible inclusion in European standard. Forest Systems, 28 (2), e008. doi:10.5424/fs/2019282-14660.
- Tabacchi, G., et al., 2007. INFC – Le Stime Di Superficie 2005–Prima Parte. Inventario Nazionale Delle Foreste E Dei Serbatoi Di Carbonio (in Italian). MiPAF–Corpo Forestale dello Stato-Ispettorato Generale-CRA–ISAFA: Trento, Italy, 2007; p. 409.
- Theomen, H., Irle, M., and Sernek, M., 2010. Wood Based Panels. An Introduction for Specialists. London, UK: Brunel University Press, 283.
- Tomak, E.D., and Gonultas, O., 2018. The wood preservative potentials of Valonia, Chestnut, Tara and Sulphited oak tannins. Journal of Wood Chemistry and Technology, 38 (3), 183–197. doi:10.1080/02773813.2017.1418379.
- Van Acker, J., et al., 2003. Biological durability of wood in relation to end-use. Holz als Roh- und Werkstoff, 61, 35–45. doi:10.1007/s00107-002-0351-8.
- Van Acker, J., Stevens, M., and De Clercq, E., 2001. Evaluation of the European standard ENV 12038 for durability testing of plywood. The International Research Group on Wood Protection, 32nd annual meeting, 20-25/05/2001, Nara, Japan, Doc IRG/WP 01-20237, p. 13.
- Van Acker, J., Van den Bulcke, J., Forsthuber, B., and Grüll, G. 2023. Wood preservation and wood finishing. In P. Niemz, A. Teischinger, and D. Sandberg, eds. Springer Handbook of Wood Science and Technology. Cham: Springer Handbooks. Springer.793–872.
- Van den Bulcke, J., et al., 2011. Moisture dynamics and fungal susceptibility of plywood. International Biodeterioration and Biodegradation, 65 (5), 708–716. doi:10.1016/j.ibiod.2010.12.015.
- Yurkewich, J.I., Castaño, C., and Colinas, C., 2017. Chestnut red stain: Identification of the fungi associated with the costly discolouration of Castanea sativa. Forest Pathology, 47, e12335. doi:10.1111/efp.12335.
- Zanuttini, R., et al., 2020a. Thermo-vacuum treatment of Poplar (Populus spp.) plywood. Holzforschung, 74 (1), 60–67. doi:10.1515/hf-2019-0049.
- Zanuttini, R., et al., 2020b. Preliminary assessment of sweet chestnut and mixed sweet chestnut-poplar OSB. Forests, 11, 496. doi:10.3390/f11050496.
- Zanuttini, R., Negro, F., and Cremonini, C., 2021. Hardness and contact angle of thermo-treated poplar plywood for biobuilding. iForest - Biogeosciences and Forestry, 14, 274–277. doi:10.3832/ifor3662-014.