ABSTRACT
The effects of light physical activity (LPA) interventions on adolescents’ learning were reviewed. Learning was operationalized as academic performance and academic-performance-related cognitive functions. Using PubMed, Web of Science, ERIC, PsycINFO, PsycARTICLES, Psychology and Behavioral Sciences Collection, and Cochrane Library, only English-language studies published until 19-07-2021 were included. Of the resulting 49, 34 studies posed a low risk of bias, covering: executive functions (inhibition, updating, and shifting), memory, and academic performance. The quality of evidence of each outcome was very low, mainly due to inconsistencies in results between studies. Nonetheless, most studies found positive (n = 15) or null (n = 14) effects of LPA interventions on adolescents’ learning. Negative effects of LPA interventions were only found from studies with on-task procedures (n = 4). One study reported positive and negative effects. Only two studies utilized longitudinal LPA interventions, of which one reported positive effects, and the other reported null effects. Future studies should explore the role of potential moderators, e.g. timing of LPA interventions with regard to the learning and testing phases. Overall, while introducing LPA in the classroom may be effective at breaking up prolonged sedentary behavior, more high-quality research is needed to establish its positive effect on learning.
1. Introduction
Students tend to spend long durations being sedentary (Buckworth & Nigg, Citation2010; Guthold et al., Citation2010), which can be detrimental for their health and wellbeing (Rezende et al., Citation2014; Tremblay et al., Citation2011). Light physical activity (LPA) has been introduced into classrooms to interrupt sedentary behavior (SB), for example by using standing and cycling desks (Freeze, Citation2016; Wallace, Citation2015). Although favorable associations of LPA interventions have been reported for physical health (Butte et al., Citation2007; Carson et al., Citation2013), no consensus exists regarding whether LPA may affect learning, especially since the classroom’s function is to facilitate learning.
In this review, learning is operationalized to include academic performance and academic-performance-related cognitive functions. Executive functions (also known as cognitive control) are subdivided into three related, yet distinct categories: updating, the constant altering of working memory content as required by the task at hand; shifting, the ability to switch swiftly between mental sets or tasks; and inhibition, the ability to resist irrelevant stimuli, memory intrusions, and inappropriate prepotent responses (Friedman & Miyake, Citation2004; Miyake et al., Citation2000). Although executive functions and memory contribute to learning, learning is a more multifaceted and complex concept. In literature, learning is described as changes in an organism (including observable behavior, neural processes, and conscious thought) caused by regular or multiple environmental stimuli (de Houwer et al., Citation2013). In this review, we focus on learning in formal educational settings, operationalized to include academic performance and academic-performance-related cognitive functions, the latter including but not limited to executive functions (Best et al., Citation2011; Bull & Scerif, Citation2001; Diamond, Citation2013; St Clair-Thompson & Gathercole, Citation2006), memory (Hassevoort et al., Citation2016), processing speed (Rohde & Thompson, Citation2007), and attention (Stevens & Bavelier, Citation2012), all positively correlating to academic performance. Hereafter, academic performance and academic-performance-related cognitive functions are categorized under the broader category of learning.
This review focuses on adolescence (age 10–25) to understand the effects of introducing LPA interventions on learning in educational institutions. Adolescence is a distinct period of brain plasticity, where neurocognitive maturation of brain regions, such as the prefrontal cortex (Choudhury et al., Citation2006; Crone & Dahl, Citation2012), can be influenced by external stimuli (Crews et al., Citation2007). There has been growing recognition of the wide adolescence age range, with earlier puberty bringing the onset of adolescence to around the age of 10 in many populations, and an increased understanding that maturation of brain regions such as the prefrontal cortex, which is important for learning-related cognitive functions, completes around age 25 (Arain et al., Citation2013; Gogtay et al., Citation2004; Sawyer et al., Citation2018). Furthermore, brain maturation rates are susceptible to individual differences, with some reaching maturity around 18-19, while others reaching maturity in their 20’s (Foulkes & Blakemore, Citation2018), necessitating a study of the entire, wide adolescence age range.
Several explanatory mechanisms have been proposed for LPA’s potential effects on learning. For example, acute LPA (LPA that is performed in an immediate, one-off manner) is thought to improve cognitive performance due to its resulting changes in cerebral blood flow, enhancing oxygenation of brain regions, such as the prefrontal cortex (Giles et al., Citation2014; Holtzer et al., Citation2011; Timinkul et al., Citation2008), which are believed to be important for cognitive functions (Basso & Suzuki, Citation2017; Koechlin et al., Citation2003; Royall et al., Citation2002). Acute LPA has also been reported to increase arousal levels (Byun et al., Citation2014), affecting the storage and retrieval of information (Eysenck, Citation1976; Kaplan et al., Citation1968; Kleinsmith & Kaplan, Citation1963; Quas & Lench, Citation2007). Increased arousal has also been shown to lead to more elaborate information-sharing in groups (Knight & Baer, Citation2014), which may affect socially constructed learning (Vygotsky, Citation2012). The effects of LPA may be different for chronic LPA, which is performed repeatedly over a longer term, such as a few weeks, months, or even years. Chronic LPA has been suggested to affect learning by stimulating neuroplasticity, modulated by neurotransmitters (Barenberg et al., Citation2011).
Other than acute and chronic durations, the effects of LPA on learning may differ depending on whether LPA is performed concurrently with learning (i.e. on-task), or before learning (i.e. off-task). Several theories, such as the transient hypofrontality hypothesis (Dietrich, Citation2006), the capacity sharing model (Kahneman, Citation1973), and the central capacity sharing model (Tombu & Jolicoeur, Citation2003), argue that the brain has limited metabolic resources, thus when shared between LPA and learning (i.e. on-task), a deterioration in performance may be seen.
With LPA already being introduced into classrooms, this review studies whether LPA interventions, compared to SB, have different effects on learning in adolescents. Additionally, we make several distinctions regarding the LPA interventions: acute or chronic, and on-task or off-task.
2. Methods
2.1. Protocol
This study’s protocol was registered in PROSPERO (Chim et al., Citation2018).
2.2. Inclusion and exclusion criteria
Studies that reported the effects of LPA interventions on learning were included. LPA encompasses all activities between SB and moderate-to-vigorous physical activity (Ainsworth et al., Citation1993; Tremblay et al., Citation2017), defined as the range between 1.6 and 2.9 metabolic equivalents (METs). For this review, standing is considered LPA, as it does not fall within the definition of SB that includes only activities done while sitting, lying, or reclining (Tremblay et al., Citation2017). Other than standing, the Compendium of Physical Activities (Ainsworth et al., Citation1993, Citation2000, Citation2011) was used if activities were narratively described. When relative intensities were provided, we referred to .
Table 1. LPA classification.
LPA interventions that were contaminated with other intensity levels or entailed other intervention components (e.g. diet, social exclusion, emotional frustration) were excluded. Furthermore, LPA interventions that were cognitively engaging or demanding (e.g. embodied learning, detailed coordination, exergaming, explicit instructions to prioritize certain activities, and learning novel movements such as balancing) were excluded. Referring to Mavilidi et al.’s (Citation2018) matrix of (temporal) integration and task relevance, LPA interventions included in our review have low task relevance and include both low and high temporal integration. Hereafter, we refer to LPA interventions that are performed concurrently with learning (high temporal integration) as on-task, and LPA interventions that are performed separately from learning (low temporal integration) as off-task.
LPA interventions were compared with a rested, seated control condition. No distinction was made between active and passive control conditions, as a previous meta-analysis found no meaningful differences between them (Au et al., Citation2020).
Learning outcomes included academic performance and academic-performance-related cognitive functions (e.g. memory, processing speed, attention, and executive functions). Studies that recruited physically and mentally healthy adolescents (including athletes, but excluding individuals with a clinical diagnosis) were included in this review. We define the adolescence range to span from 10 to 25 years. As there are several studies that include samples with a mean age of around 10 or 25 years, we made the decision to include studies where at least 84% of their sample (mean age plus one standard deviation) was within the age range of 10–25 years.
2.3. Search strategy and study selection
A systematic literature search was carried out using the electronic databases PubMed, Web of Science, ERIC, PsycINFO, PsycARTICLES, Psychology and Behavioral Sciences Collection, and Cochrane Library. For time- and resource-saving purposes, only English language articles published until 19-07-2021 were included. Using the PubMed Medical Subject Headings database, the search string, reported in Supplementary Material A, was used to search articles by title. Additionally, references in relevant studies were manually screened. Initially, we placed no restrictions on the study design, as reported in our protocol. However, to provide a more focused review on the causal effects of LPA interventions, we decided to include only intervention studies.
2.4. Data extraction
In total, 8,121 non-duplicated records were found (see ). Two authors independently screened 500 studies – first screening the title and abstract, then evaluating the full paper – achieving more than 80% agreement on the included studies. The remaining studies were divided between the two authors. The type of data to be extracted (i.e. sample size, sex, age, type of LPA intervention, intensity classification, duration of LPA, study design, outcome measure, type of test, measurement timing, and result direction) from the included studies was determined a priori with consensus from all authors. Considering potential speed-accuracy trade-off that may result from prioritizing perceptual, cognitive, and motor processes (Rinkenauer et al., Citation2004), reaction time and accuracy were considered as separate outcomes where possible. Studies were also split up between those that assessed learning outcomes during the LPA intervention (on-task) and those that assessed learning outcomes after (off-task), because on-task studies may be affected by the sharing of metabolic resources between learning and LPA (Dietrich, Citation2006; Kahneman, Citation1973; Tombu & Jolicoeur, Citation2003). Finally, studies that included longer-term/chronic LPA interventions were annonated to differentiate them from acute effects of LPA interventions.
Figure 1. PRISMA 2009 flow diagram (Moher et al., Citation2009).
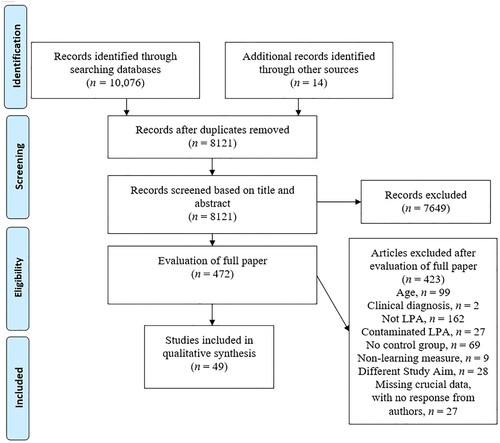
2.5. Quality assessment
Critical assessment of the included studies was done independently by two authors using the revised Cochrane risk of bias tool for randomized trials (RoB2) (Sterne et al., Citation2019). The RoB2 assesses biases that may affect the intervention outcomes: the randomization process, deviations from original protocol, missing data, measurement of outcome, and selective reporting. The overall judgement for the outcome can be rated as high risk of bias, some concerns, or low risk of bias, with the proposed ratings allowed to be overridden by the authors (Sterne et al., Citation2019). We overrode the RoB2 ratings for three situations. Firstly, for crossover studies that used counterbalancing, the randomization of allocation sequence would not influence the studies’ outcome, because we do not expect for participants or the experimenters to favor one condition over the other (i.e. the intervention or control). Secondly, concealment of allocation sequence is unnecessary because it is impossible to conceal the physical experience of LPA. Thirdly, although we recognize the importance of a published pre-specified plan, most papers in the current field have yet to adhere to this relatively new requirement. Therefore, we did not flag the studies as risky based solely on this criterion. Any discrepancies between the two authors were discussed among all authors and resolved through consensus. Only studies with a low risk of bias were extracted for further analysis to strengthen the argumentation of evidence.
The quality of evidence for each outcome, from only low risk of bias studies was assessed independently by two authors using the Grades of Recommendation, Assessment, Development, and Evaluation (GRADE; Guyatt et al., Citation2011). GRADE provides guidelines to rate the quality of evidence per outcome level, ranging from high to moderate, low, and very low quality. The assessment rating was based on five criteria: inconsistency, indirectness, imprecision, publication bias, and risk of bias. Inconsistency describes the heterogeneity of results. Indirectness indicates how well the evidence answers the research question. Imprecision informs on whether the 95% confidence interval around the absolute effect is sufficiently narrow to avoid random errors. Publication bias indicates whether selective publication has been detected.
3. Results
This review included 49 studies (see Supplementary Material B for study characteristics). The PRISMA flow diagram in provides more information on the number of records identified, screened, and full-text assessed.
3.1. Critical assessment of individual studies
Using the RoB2, several included studies posed risks to the current review’s aim of studying the effects of LPA interventions on learning, detailed in Supplementary Material C. Twelve studies were rated as high-risk (Akazawa et al., Citation2019; Brisswalter et al., Citation1997; Del Giorno et al., Citation2010; Frodsham et al., Citation2020; Haynes et al., Citation2019; Lindheimer et al., Citation2017; Lo Bue-Estes et al., Citation2008; Lowe et al., Citation2016; Martins et al., Citation2013; Tsukamoto et al., Citation2017; Williams et al., Citation1985; Yamazaki et al., Citation2017), due to deviations from the intended intervention or missing outcome data. Three studies were rated with some concerns (Hasegawa et al., Citation2001; Schramke & Bauer, Citation1997; Wick et al., Citation2018), because of potential bias from the randomization process or deviations from the intended intervention. Notably, although some studies were well-designed, their research aim was not congruent with the current review’s aim (e.g. the lack of counterbalancing may not be a risk within the individual studies, but poses a risk to the current review). The learning outcomes were analyzed solely from the 34 remaining studies with a low risk of bias.
3.2. Study characteristics
Of the 34 studies with low risk of bias, 19 focused purely on LPA interventions. Five studies aimed to measure moderate physical activity but their resulting intensities fall within the current review’s definition of LPA (Cuttler et al., Citation2018; Harveson et al., Citation2019; Joyce et al., Citation2009; Loprinzi, Koehler, et al., Citation2019; Soga et al., Citation2015). The remaining 10 studies studied the effects of various physical activity intensities, from which we only extracted the effects of LPA (Brush et al., Citation2016; Budde et al., Citation2010; Endo et al., Citation2013; Hötting et al., Citation2016; Kamijo et al., Citation2009; Loprinzi & Kane, Citation2015; Loprinzi, Day, et al., Citation2019; Olson et al., Citation2016; Wang et al., Citation2013; Zuniga et al., Citation2019).
Within-groups design was utilized in 19 studies, between-groups design in 10 studies, and mixed design in five studies. The included studies comprised of 1,643 participants, with sample sizes ranging between 10 and 117. The participants’ mean age per study ranged between 11 and 23 years. No individual study sampled the full age range of 10–25 years. Only one study explicitly focused on early-adolescence (mean age 11). All other studies focused on mid- to late–adolescence (mean age 14–23 years). All studies utilized acute interventions, except for two studies that used chronic interventions: using ergometers for approximately 11 min/week across eight weeks (Liu et al., Citation2017) and ergometers with desks for at least 120 min/week across 10 weeks (Pilcher et al., Citation2017).
3.3. Evidence synthesis
The learning outcomes were categorized into inhibition, updating, shifting, memory, and academic performance. Based on the GRADE assessment, the quality of evidence for each outcome was rated as ‘very low’, because of three main reasons. First, the effects found were inconsistent: with positive, null, and negative effects found for all outcomes (except for shifting, with positive and null effects only). Second, the aggregated sample size for updating, shifting, and memory was small, leading to issues of imprecision, according to GRADE. Third, the studies did not directly measure the full age range targeted in this review. The quality of evidence is summarized in , with detailed assessment for each outcome listed in . Of these studies, 15 studies reported positive effects, four studies reported negative effects, one study reported positive and negative effects, with the remaining 14 studies finding no significant effects. Nonetheless, the ‘very low’ quality of evidence indicates no clear effects of LPA interventions on learning among adolescents.
Table 2. Summary of quality of evidence according to GRADE.
Table 3. Effects of LPA interventions on inhibition.
Table 4. Effects of LPA interventions on updating.
Table 5. Effects of LPA interventions on shifting.
Table 6. Effects of LPA interventions on memory.
Table 7. Effects of LPA interventions on academic performance.
3.4. Timing of LPA intervention
A comparison was made for studies that used an on-task () or off-task protocol (). From separating the studies into on- or off-task, the only observable pattern was that negative effects from the LPA intervention were only present from on-task protocols (Abou Khalil et al., Citation2020; Larson, LeCheminant, Hill, et al., Citation2015; Olson et al., Citation2016; Pontifex & Hillman, Citation2007; Soga et al., Citation2015).
Table 8. Studies where the LPA intervention and the outcome measure are conducted concurrently (i.e. on-task).
Table 9. Studies where the LPA intervention are conducted before assessing the outcome measure (i.e. off-task).
A detailed look into the on-task studies reveals two further types of protocols, whether the LPA was administered before or during a learning phase, and whether the LPA was administered before or during the measurement of learning outcomes (see ). A learning phase was incorporated within seven studies, where the participants were given material to learn before a test. The timing of LPA differed between studies, with some administering LPA before or after the learning phase. Comparing the studies by Hötting et al. (Citation2016) and Schmidt-Kassow et al. (Citation2014), the participants had to translate newly learnt Polish vocabulary into the German language. Both groups of researchers administered LPA interventions that elicited similar heart rates (around 100 bpm) and measured similar outcomes 24 h later. Hötting and colleagues did not find a significant effect, whereas Schmidt-Kassow’s group reported that their LPA intervention boosted the learning of Polish-German words. The main difference is that, in Hötting et al.’s study, the participants learnt the Polish-German words while seated, then participated in the LPA intervention; whereas Schmidt-Kassow et al.’s participants learnt the Polish-German words during the LPA intervention. This finding that LPA during learning may lead to better performance is supported by Liu et al. (Citation2017), whose participants learnt a second-language while carrying out the LPA intervention, also finding improved performance.
Table 10. Timing of LPA intervention with respect to the learning phase.
Although there seems to be a pattern that LPA during learning improves language-learning performance, this finding was not replicated for an Introductory Psychology exam (Pilcher et al., Citation2017), where participants who cycled while studying did not do any better than their sedentary peers. This suggests that the effects of LPA interventions may be different depending on the context or subject. Furthermore, verbal memory appears to be affected differently, compared to language-learning. In a verbal memory study, participants who memorized lists of words in their native language while walking, hence having LPA during their learning, recalled less items than the group that was seated (Larson, LeCheminant, Hill, et al., Citation2015). Notably, Larson and colleagues’ intervention differed from the aforementioned studies (Schmidt-Kassow et al. (Citation2014) and Liu et al. (Citation2017) who found that LPA during learning enhanced performance) because both the learning and the testing phase were done while walking, suggesting that the timing of the LPA intervention relative to the learning measurement moderates its effects as well.
3.5. Timing of delayed testing
Related to the timing of the LPA intervention, nine studies measured learning after a delay. With 5-minute to 40-minute delays, positive effects were found for inhibition (Endo et al., Citation2013; Harveson et al., Citation2016; Joyce et al., Citation2009; O’Leary et al., Citation2011), shifting (Harveson et al., Citation2016; Jaffery et al., Citation2018), and mathematics (Harveson et al., Citation2019).
Although Endo et al. (Citation2013) found positive effects for inhibition 5 min after the LPA intervention, this effect was not replicated just 20 min later. Harveson et al. (Citation2016) reported positive effects for mathematics after a delay of 5-20 min, but no significant effect was found for inhibition. Likewise, no significant effects were found after a delay of 15-25 min for inhibition, updating, and shifting (Brush et al., Citation2016), Polish-German vocabulary (Hötting et al., Citation2016), and for memory (Loprinzi, Koehler, et al., Citation2019). Brush et al. (Citation2016) also measured inhibition, updating, and shifting after a 3-hour delay, and similarly did not find any significant effect from the LPA intervention.
Longer delays include a 24-hour and one-month temporal gap. After a 24-hour delay, Schmidt-Kassow et al. (Citation2014) found positive effects on learning Polish-German words, but Hötting et al. (Citation2016) did not find any significant effect. Only one study measured learning after a one-month delay (Liu et al., Citation2017), and found positive effects despite the long delay.
3.6. Duration, type, intensity, and frequency of LPA intervention
Across the included studies, LPA interventions lasted from 30 s to 240 min. Inconsistency remains a problem, as studies that administered the same duration of LPA find conflicting results (detailed in Supplementary Material B). No discernerable pattern was observed with regard to duration.
Reported effects were different depending on the type of LPA intervention. Alderman et al. (Citation2014) and Olson et al. (Citation2016) tested inhibition during LPA. Alderman et al. did not find that their LPA intervention had an effect, whereas Olson et al. found a deterioration in accuracy when performing the same inhibition test. The only difference is that participants walked in Alderman et al.’s study, while Olson et al.’s participants cycled on an ergometer. Therefore, the type of LPA appears to influence the effects found. Another example is the comparison of two studies by Harveson et al. (Citation2016, Citation2019). Shifting was found to improve from aerobic LPA (Harveson et al., Citation2016), whereas mathematics and inhibition improved only from resistance LPA (Harveson et al., Citation2019). One final example on how different types of LPA may lead to different results is provided by Abou Khalil et al. (Citation2020). In their study, immediate and delayed free recall of words were better during a bout of walking compared to sitting, but no significant differences were found between standing and sitting. Also, for mental arithmetic, walking (but not standing) led to a lower performance on the rate of correct answers, while standing (but not walking) led to a lower performance on the reaction time of correct answers. These patterns suggest that the different types of LPA may differentially affect learning.
Only one included study manipulated the intensity of LPA. Within the same group of participants, Endo et al. (Citation2013) found that light cycling at 40% maximal workload boosted performance on inhibition, whereas very light cycling at 20% maximal workload did not show any significant performance changes.
There were no studies that manipulated the frequency of LPA administration, for example, by changing how many times per week the participants were exposed to the LPA interventions. Of the two longitudinal studies that did administer frequent LPA, all three studies were very different in their outcome measure and overall design (detailed in Supplementary Material B). Once again, the LPA interventions were found to have inconsistent results, with no effects on a Psychology exam (Pilcher et al., Citation2017) and positive effects on language-learning (Liu et al., Citation2017).
3.7. Individual differences
Burgess and Hokanson (Citation1964) found that performance on an updating task only improved post-LPA within the fitter group with a lower resting heart rate, but not in the less fit group with a higher resting heart rates. Yet, Zuniga et al. (Citation2019) did not find differences between low-fit and high-fit participants on memory performance, with both groups benefiting from a bout of treadmill walking.
With regard to baseline performance, Budde et al. (Citation2010) compared between those who did well and those who did less well during their baseline updating performance. Only those who performed worse at baseline showed improvements in their updating performance after LPA.
3.8. Type of test used
The observable effects of LPA interventions differed when different types of tests were used, even if the different tests were supposed to measure the same outcome. For example, when testing inhibition, positive effects were found after acute LPA using the Flanker task (O’Leary et al., Citation2011), Stroop test (Byun et al., Citation2014; Endo et al., Citation2013; Harveson et al., Citation2016, Citation2019), Simon task (Ellemberg & St-Louis-Deschênes, Citation2010), and Stop-signal task (Joyce et al., Citation2009), but no effects were found for the Feature Match and Polygon tests (Loprinzi & Kane, Citation2015). Also regarding updating, positive effects were found after acute LPA using the n-back task (Delli Paoli et al., Citation2017) and Digit Span task (Budde et al., Citation2010; Burgess & Hokanson, Citation1964), but not using the Verbal Running Span task (Brush et al., Citation2016), Aospan task (Grubaugh & Rhea, Citation2014), Spatial Search task, or the Spatial Slider task (Loprinzi & Kane, Citation2015). For shifting, positive results after an acute bout of LPA were only found for the Trail Making test (Harveson et al., Citation2016; Jaffery et al., Citation2018). Even within the same study, Cuttler et al. (Citation2018) measured prospective memory after an acute bout of LPA and found that LPA interventions produced positive effects when tested with the Reminder Prospective Memory test, but null effects when tested with the Red Pen Prospective Memory test. Thus, it appears that different tests may differ in their sensitivity to the effects of LPA interventions.
3.9. Speed and accuracy
For the outcome of inhibition, positive effects of LPA were seen only in reaction time (Byun et al., Citation2014; Ellemberg & St-Louis-Deschênes, Citation2010; Endo et al., Citation2013; Harveson et al., Citation2016, Citation2019; Joyce et al., Citation2009; O’Leary et al., Citation2011), while negative effects were found only for accuracy (Olson et al., Citation2016; Pontifex & Hillman, Citation2007). As for the outcome of updating, negative effects were found for both accuracy (Larson, LeCheminant, Hill, et al., Citation2015) and reaction time (Soga et al., Citation2015), while positive effects were only found for accuracy (Delli Paoli et al., Citation2017). This contrasted the patterns found for inhibition, where positive effects were found for reaction time and negative effects for accuracy. Finally, for the outcomes of shifting and language-related academic performance, positive effects were found for both accuracy (Liu et al., Citation2017) and reaction time (Harveson et al., Citation2016; Jaffery et al., Citation2018; Liu et al., Citation2017). Overall, the effects of LPA interventions on speed and accuracy did not produce consistent, discernerable patterns.
4. Discussion
The aim of this paper was to systematically review the effects of LPA interventions on learning in healthy adolescents aged 10–25 years. A total of 49 studies was found, of which only 34 were judged to have low risk of bias. From these 34 studies, positive effects were found in 16 studies, negative effects in four studies, one study reported both positive and negative effects, and the remaining 14 studies reported null effect. The learning outcomes were grouped into five categories: (1) inhibition, (2) updating, (3) shifting, (4) memory, and (5) academic performance. The quality of evidence was very low, indicating no clear effects of LPA interventions on learning.
To improve future quality of evidence, the issues of inconsistency, imprecision, and indirectness need to be addressed. To reduce inconsistencies between future studies, one solution would be to account for potentially moderating variables, identified in the current review – a recommendation that has been strongly advocated before (Brisswalter et al., Citation2002; Tomporowski & Ellis, Citation1986). Considering the numerous potential moderators that we have identified, the inclusion of too many covariates may result in overfitting (Zhang, Citation2014). Therefore, we advise to recruit sufficiently large samples (other solutions to overfitting are discussed by Babyak (Citation2004) and Hawkins (Citation2004)), which would also narrow the margin of error, reducing imprecision over the aggregated included studies. To reduce indirectness, we implore for more representation of 10–25 age range, as early adolescence (10–13 years) is currently understudied in literature, and the overall age range is an important period for brain plasticity (Arain et al., Citation2013; Gogtay et al., Citation2004; Sawyer et al., Citation2018).
To better understand why inconsistencies exist in literature, variables that may have potential confounding effects have to be teased apart and studied. We recommend that researchers make careful choices with regard to: timing of LPA intervention, timing of delayed testing, duration and type of LPA, individual differences, and type of test used. With the various protocols currently utilized, it is difficult to make a direct comparison of the results. Furthermore, several factors remain understudied, such as the different LPA intensities and frequency of LPA interventions. Therefore, we encourage for more dose–response studies to explain the inconsistencies in results currently found.
With regard to timing of the LPA intervention, it appears that administering LPA during the learning phase fosters performance, especially in language-learning (Liu et al., Citation2017; Schmidt-Kassow et al., Citation2014). The positive effects could be ascribed to LPA increasing arousal level (Byun et al., Citation2014; Knight & Baer, Citation2014) or brain oxygenation (Giles et al., Citation2014; Holtzer et al., Citation2011; Timinkul et al., Citation2008). Administering LPA before the testing phase of learning may lead to boosted performance, while administering LPA during the testing phase may lead to a drop in performance. This finding aligns with the theories that suggest a sharing of metabolic resources between learning and maintaining concurrent LPA, leading to lower learning performance (Dietrich, Citation2006; Kahneman, Citation1973; Tombu & Jolicoeur, Citation2003). Therefore, the timing of LPA, in relation to the learning and testing phase, is highly likely a moderator.
When it comes to delayed testing, learning outcomes measured after a very short (5-minute) delay tend to show positive effects (Endo et al., Citation2013; Harveson et al., Citation2016, Citation2019; Jaffery et al., Citation2018). Longer delays (20 min to 3 h) produce inconsistent findings. A previous meta-analysis reported similar findings, with larger positive effects observed from shorter delays (Chang et al., Citation2012). For positive effects to persist after longer delays, the LPA intervention would need to last for longer durations or be of higher intensities (Chang et al., Citation2012). With regard to language-related outcomes, beneficial effects appear to withstand longer delays of 24 h (Schmidt-Kassow et al., Citation2014) and one month (Liu et al., Citation2017). Inconsistencies still exist, with Hötting et al. (Citation2016) not finding a significant effect on language-learning, but this could possibly be explained with the participants only carrying out LPA after learning the language (see and section 3.4). Overall, positive effects on learning can be found after a very short delay, while positive effects on language-related outcomes tend to withstand longer delays.
Inconsistency in results was high when scrutinizing on the duration and type of LPA. For example, with duration, when inhibition was measured after 30 min of LPA, positive effects (Ellemberg & St-Louis-Deschênes, Citation2010; Harveson et al., Citation2016; Joyce et al., Citation2009), negative effects (Olson et al., Citation2016), and null effects (Alderman et al., Citation2014; Loprinzi & Kane, Citation2015) were reported. As for the type of LPA, different types of LPA recruit different musculoskeletal functions and bodily systems (e.g. aerobic vs. resistance movements (Harveson et al., Citation2019)), possibly explaining the inconsistencies found. Further research into the potential moderators is required to understand the nuances that are present in extant literature.
When controlling for individual differences, two important factors were found from literature – fitness levels and baseline performance on the learning measure. The potential moderating effects of these two factors have been highlighted elsewhere (Pesce, Citation2009). Fitness levels were expected to only affect cognitive outcomes that were measured concurrently with physical activity (Pesce, Citation2009). In our review, Burgess and Hokanson (Citation1964) found that after an acute bout of LPA, physically fit individuals perform better in an updating task, compared to their less-fit peers. This hints at fitness levels affecting one’s capability to cope with the demands of a physical activity intervention even after the conclusion of the physical activity intervention. This result is supported by studies of higher intensity showing that fit individuals, compared to their unfit counterparts, show better cognitive performance during (Delignières et al., Citation1994) and after a bout of physical activity (Sjöberg, Citation1980). Nonetheless, one study from our review (Zuniga et al., Citation2019) did not find an effect of fitness levels, necessitating future studies to investigate the potential moderating effects of fitness on the relationship between LPA interventions and learning.
With regard to baseline performance of the learning measure, Budde et al. (Citation2010) found that those who performed worse from the onset experienced a larger improvement in their learning. Previous studies that used moderate-to-vigorous physical activity interventions align well with Budde et al.’s study, with those who performed less well at baseline benefiting more during measures of executive functions (Drollette et al., Citation2014; Sibley & Beilock, Citation2007) and classroom on-task behavior (Mahar et al., Citation2006). Budde and colleagues ruled out ceiling effects for the higher performing group, as no participant was able to complete the entire Letter Digit Span task of updating. Further research is needed to uncover the explanation for why physical activity interventions appear to be most beneficial for those with a larger learning margin. Overall, there are hints that individual differences may influence the relationship between LPA interventions and learning outcomes. However, due to the low number of studies that have accounted for individual differences, we cannot yet conclude which individual differences influence the effect of LPA interventions on learning and how these differences influence the effects.
In this review, different results were found for different tests, despite assessing the same outcome (see section 3.8 Type of test used). Assuming the tests used are valid and reliable, the effect of any intervention comes into question when different results are found with different types of tests assuming to measure the same construct. For future studies, we recommend researchers to select outcome measures based on clear theoretical rationales. For example, executive functions have over 128 different tests available (Etnier & Chang, Citation2009). Therefore, the type of test should be selected based on empirical evidence showing that the test is sensitive to the effects of LPA interventions on adolescents.
As for speed and accuracy, no consistent effect was found from the LPA interventions. Although previous studies reported a trade-off between speed and accuracy in cognitive tests (Rinkenauer et al., Citation2004), the studies included in our review did not find support for this trade-off. Initially, it appeared that LPA interventions may have a positive effect on the reaction time of inhibition and a negative effect on accuracy. However, these findings were not sufficient to indicate that the effects of LPA interventions were affected by a trade-off in speed-accuracy, because no one study reported both a positive effect on reaction time and a negative effect on accuracy. Furthermore, the pattern found for inhibition was contrasted by updating, shifting, and language-related academic performance (see section 3.9 Speed and Accuracy). Overall, the effects of LPA interventions on speed and accuracy remain inconsistent across studies.
Two factors remain understudied in extant literature – intensity and frequency of LPA interventions. Despite already focusing on the lighter end of the intensity spectrum, different intensities still appear to have a different effect on learning outcomes (e.g. 20% vs. 40% maximal workload in, Endo et al. (Citation2013)’s study). With only one study manipulating the intensity, more studies are needed to check whether intensity may moderate the effects of LPA interventions on learning. As for the frequency of LPA interventions, none of the included studies studied the frequency of LPA interventions (e.g. multiple LPA interventions in a week vs. once per week). Further research on manipulating the frequency of LPA interventions is needed because having more frequent LPA interventions may enlarge the effect size. Moreover, the LPA interventions introduced within classrooms, such as standing and cycling desks, are not meant for one-time use. These arguments can also be made for longitudinal designs. Yet, only two longitudinal studies were found in this review (Liu et al., Citation2017; Pilcher et al., Citation2017) with inconsistent results found. It is important to study the longitudinal effects of LPA interventions because the results may vary according to how experienced the participant is to the LPA intervention. In a qualitative study, participants explained that similar to starting a new exercise program, the novel experience of using standing desks is different from long-term use, requiring time needed for familiarization (Wilkerson et al., Citation2019). In sum, further research is needed on the frequency and long-term effects of LPA interventions, to explores its possible dose–response effect on learning.
Despite the inconsistencies between studies, we can still derive some practical implications from this review. Of the included studies, 15 studies reported a positive effect, while 14 studies reported null effects. Apart from the focus on learning, other researchers have found LPA to be beneficial for physical health (Chastin et al., Citation2019; Füzéki et al., Citation2017), counteracting the negative risks of prolonged SB (Rezende et al., Citation2014; Tremblay et al., Citation2011). Thus, adolescents should not be held back from the health benefits of LPA, considering the probable null or positive effects that LPA has on learning. However, we do not discount the negative effects found from four studies (Larson, LeCheminant, Hill, et al., Citation2015b; Olson et al., Citation2016; Pontifex & Hillman, Citation2007; Soga et al., Citation2015) and one study that reported both positive a and negative effects (Abou Khalil et al., Citation2020). Within these studies, learning outcomes were measured during the LPA intervention. Therefore, the practical recommendation would be to not administer LPA during exams (despite one study finding boosted performance on a mathematics exam when the students were standing (Isip, Citation2014)). Instead, we encourage education institutions to provide students with the option to perform LPA during their learning phase, especially during language-learning, or to provide LPA breaks in-between classes.
To our knowledge, this is the first systematic review to focus exclusively on LPA and its effects on learning in adolescents. Previous systematic reviews tend to focus on general physical activity, usually of moderate-to-vigorous intensities (Álvarez-Bueno et al., Citation2017; Biddle et al., Citation2019; Daly-Smith et al., Citation2018; Singh et al., Citation2018; Wassenaar et al., Citation2020). These moderate-to-vigorous physical activity reviews present similar findings as the current LPA review. Mainly, the majority of studies on physical activity interventions pointed towards either positive or null effects on cognitive and academic performance, yet the inconsistent effects lead to inconclusive evidence. Similarly, these reviews also highlight the high heterogeneity in the intervention design and measurement of outcomes, with a lack of studies attempting to clarify the dose–response effects of various potential moderators.
The limitation of this review is that during data extraction, only one author screened the majority of studies. For the first 500 studies that were screened by two authors, a high interrater agreement (> 80%) was achieved, therefore the remaining studies were screened by only one author for efficiency. Still, human error may have led to the overlooking of relevant articles that could have been included in this review. The strengths of this review include the thorough and independent assessment of each study by two authors. The RoB2 was used to assess the risk of bias from each study, then the quality of evidence at the outcome level was further evaluated using GRADE. Basing our analysis on only low-risk-of-bias studies also serves as a strength of this review, as the conclusions drawn would be less susceptible to methodological errors in trial design, conduct, or reporting.
Hitherto, there is a lack of high-quality evidence to substantiate the effects of LPA interventions on learning among adolescents. This review found 15 studies reporting positive effects, four studies reporting negative effects, one study reporting both positive and negative effects, while the remaining 14 studies reporting null effects. These studies were conducted in laboratory settings, with the exception of Pilcher et al.’s (Citation2017) library setting. Further studies are therefore needed for three reasons. First, to understand the effects of LPA interventions in field settings. Second, to elucidate potential moderators. And third, to replicate current studies to ascertain the reliability of results. Apart from learning, LPA has various benefits for physical health (Chastin et al., Citation2019; Füzéki et al., Citation2017), with a high feasibility and acceptance in classrooms (Benzo et al., Citation2016; Sherry et al., Citation2016). Thus, there is no reason to refrain from introducing LPA interventions among adolescents. The majority of included studies in this review find positive or null effects of LPA interventions on adolescents’ learning. This suggests that introducing more LPA in the classrooms to break up prolonged SB fosters learning, but further research is warranted.
Supplemental Material
Download MS Word (13.4 KB)Supplemental Material
Download MS Word (477 KB)Supplemental Material
Download MS Word (18 KB)Disclosure statement
No potential conflict of interest was reported by the author(s).
References
- (*denotes studies included in current review)
- *Abou Khalil, G., Dore-Mazars, K., Senot, P., Wang, D. P., & Legrand, A. (2020). Is it better to sit down, stand up or walk when performing memory and arithmetic activities? Experimental Brain Research, 238(11), 2487–2496. https://doi.org/10.1007/s00221-020-05858-z
- Ainsworth, B. E., Haskell, W. L., Herrmann, S. D., Meckes, N., Bassett, D. R., Tudor-Locke, C., Greer, J. L., Vezina, J., Whitt-Glover, M. C., & Leon, A. S. (2011). 2011 Compendium of physical activities: A second update of codes and MET values. Medicine & Science in Sports & Exercise, 43(8), 1575–1581. https://doi.org/10.1249/MSS.0b013e31821ece12
- Ainsworth, B. E., Haskell, W. L., Leon, A. S., Jacobs, J. D., Montoye, H. J., Sallis, J. F., & Paffenbarger, J. R. (1993). Compendium of physical activities: Classification of energy costs of human physical activities. Medicine & Science in Sports & Exercise, 25(1), 71–80. https://doi.org/10.1249/00005768-199301000-00011
- Ainsworth, B. E., Haskell, W. L., Whitt, M. C., Irwin, M. L., Swartz, A. M., Strath, S. J., O’Brien, W. L., Bassett Jr, D. R., Schmitz, K. H., & Emplaincourt, P. O. (2000). Compendium of physical activities: An update of activity codes and MET intensities. Medicine & Science in Sports & Exercise, 32(Supplement), S498–S516. https://doi.org/10.1097/00005768-200009001-00009
- *Akazawa, N., Kobayashi, N., Nakamura, Y., Kumagai, H., Choi, Y., & Maeda, S. (2019). Effect of sleep efficiency on salivary metabolite profile and cognitive function during exercise in volleyball athletes. European Journal of Applied Physiology, 119(10), 2215–2223. https://doi.org/10.1007/s00421-019-04205-7
- Alderman, B. L., Olson, R. L., & Mattina, D. M. (2014). Cognitive function during low-intensity walking: A test of the treadmill workstation. Journal of Physical Activity and Health, 11(4), 752–758. https://doi.org/10.1123/jpah.2012-0097
- Álvarez-Bueno, C., Pesce, C., Cavero-Redondo, I., Sánchez-López, M., Martínez-Hortelano, J. A., & Martínez-Vizcaíno, V. (2017). The effect of physical activity interventions on children’s cognition and metacognition: A systematic review and meta-analysis. Journal of the American Academy of Child and Adolescent Psychiatry, 56(9), 729–738. https://doi.org/10.1016/j.jaac.2017.06.012
- American College of Sports Medicine. (2018). General Principles of Exercise Prescription. In ACSM’s Guidelines For Exercise Testing and Prescription (10th ed., pp. 226–269). Lippincott Williams & Wilkins.
- Arain, M., Haque, M., Johal, L., Mathur, P., Nel, W., Rais, A., Sandhu, R., & Sharma, S. (2013). Maturation of the adolescent brain. Neuropsychiatric Disease and Treatment, 9, 449–461. https://doi.org/10.2147/NDT.S39776
- Arts, F., & Kuipers, H. (1994). The relation between power output, oxygen uptake and heart rate in male athletes. International Journal of Sports Medicine, 15(05), 228–231. https://doi.org/10.1055/s-2007-1021051
- Au, J., Gibson, B. C., Bunarjo, K., Buschkuehl, M., & Jaeggi, S. M. (2020). Quantifying the difference between active and passive control groups in cognitive interventions using two meta-analytical approaches. Journal of Cognitive Enhancement, 4(2), 192–210. https://doi.org/10.1007/s41465-020-00164-6
- Babyak, M. A. (2004). What you see may not be what you get: A brief, nontechnical introduction to overfitting in regression-type models. Psychosomatic Medicine, 66(3), 411–421. https://doi.org/10.1097/01.psy.0000127692.23278.a9
- Barenberg, J., Berse, T., & Dutke, S. (2011). Executive functions in learning processes: Do they benefit from physical activity? Educational Research Review, 6(3), 208–222. https://doi.org/10.1016/j.edurev.2011.04.002
- Basso, J. C., & Suzuki, W. A. (2017). The effects of acute exercise on mood, cognition, neurophysiology, and neurochemical pathways: A review. Brain Plasticity, 2(2), 127–152. https://doi.org/10.3233/BPL-160040
- Benzo, R. M., Gremaud, A. L., Jerome, M., & Carr, L. J. (2016). Learning to stand: The acceptability and feasibility of introducing standing desks into college classrooms. International Journal of Environmental Research and Public Health, 13(8), 823. https://doi.org/10.3390/ijerph13080823
- Best, J. R., Miller, P. H., & Naglieri, J. A. (2011). Relations between executive function and academic achievement from ages 5 to 17 in a large, representative national sample. Learning and Individual Differences, 21(4), 327–336. https://doi.org/10.1016/j.lindif.2011.01.007
- Biddle, S. J. H., Ciaccioni, S., Thomas, G., & Vergeer, I. (2019). Physical activity and mental health in children and adolescents: An updated review of reviews and an analysis of causality. Psychology of Sport and Exercise, 42, 146–155. https://doi.org/10.1016/j.psychsport.2018.08.011
- Borg, G. A. V. (1982). Psychophysical bases of perceived exertion. Medicine & Science in Sports & Exercise, 14(5), 337–381. https://doi.org/10.1249/00005768-198205000-00012
- *Brisswalter, J., Arcelin, R., Audiffren, M., & Delignieres, D. (1997). Influence of physical exercise on simple reaction time: Effect of physical fitness. Perceptual and Motor Skills, 85(3), 1019–1027. https://doi.org/10.2466/pms.1997.85.3.1019
- Brisswalter, J., Collardeau, M., & René, A. (2002). Effects of acute physical exercise characteristics on cognitive performance. Sports Medicine, 32(9), 555–566. https://doi.org/10.2165/00007256-200232090-00002
- *Brush, C. J., Olson, R. L., Ehmann, P. J., Osovsky, S., & Alderman, B. L. (2016). Dose–response and time course effects of acute resistance exercise on executive function. Journal of Sport & Exercise Psychology, 38(4), 396–408. https://doi.org/10.1123/jsep.2016-0027
- Buckworth, J., & Nigg, C. (2010). Physical activity, exercise, and sedentary behavior in college students. College Health, 53(1), 28–34. https://doi.org/10.3200/JACH.53.1.28-34
- *Budde, H., Voelcker-Rehage, C., Pietrassyk-Kendziorra, S., Machado, S., Ribeiro, P., & Arafat, A. M. (2010). Steroid hormones in the saliva of adolescents after different exercise intensities and their influence on working memory in a school setting. Psychoneuroendocrinology, 35(3), 382–391. https://doi.org/10.1016/j.psyneuen.2009.07.015
- Bull, R., & Scerif, G. (2001). Executive functioning as a predictor of children’s mathematics ability: Inhibition, switching, and working memory. Developmental Neuropsychology, 19(3), 273–293. https://doi.org/10.1207/S15326942DN1903_3
- *Burgess, M., & Hokanson, J. E. (1964). Effects of increased heart rate on intellectual performance. Journal of Abnormal Psychology, 68(1), 85–91. https://doi.org/10.1037/h0042849
- Butte, N. F., Puyau, M. R., Adolph, A. L., Vohra, F. A., & Zakeri, I. (2007). Physical activity in nonoverweight and overweight Hispanic children and adolescents. Medicine & Science in Sports & Exercise, 39(8), 1257–1266. https://doi.org/10.1249/mss.0b013e3180621fb6
- *Byun, K., Hyodo, K., Suwabe, K., Ochi, G., Sakairi, Y., Kato, M., Dan, I., & Soya, H. (2014). Positive effect of acute mild exercise on executive function via arousal-related prefrontal activations: An fNIRS study. NeuroImage, 98, 336–345. https://doi.org/10.1016/j.neuroimage.2014.04.067
- Carson, V., Ridgers, N. D., Howard, B. J., Winkler, E. A. H., Healy, G. N., Owen, N., Dunstan, D. W., & Salmon, J. (2013). Light-intensity physical activity and cardiometabolic biomarkers in US adolescents. PLoS ONE, 8(8), e71417. https://doi.org/10.1371/journal.pone.0071417
- Chang, Y. K., Labban, J. D., Gapin, J. I., & Etnier, J. L. (2012). The effects of acute exercise on cognitive performance: A meta-analysis. Brain Research, 1453, 87–101. https://doi.org/10.1016/j.brainres.2012.02.068
- Chastin, S. F. M., De Craemer, M., De Cocker, K., Powell, L., Van Cauwenberg, J., Dall, P., Hamer, M., Stamatakis, E., Sebastien, D., & Chastin, F. M. (2019). How does light-intensity physical activity associate with adult cardiometabolic health and mortality? Systematic review with meta-analysis of experimental and observational studies. British Journal of Sports Medicine, 53(6), 370–376. https://doi.org/10.1136/bjsports-2017-097563
- Chim, H. Q., Gijselaers, H., Savelberg, H., Gerven, P. v., OudeEgbrink, M., & Groot, R. d. (2018). Light intensity physical activity in relation to learning in adolescents: A systematic review. PROSPERO: International prospective register of systematic reviews, CRD4201809.
- Choudhury, S., Blakemore, S.-J., & Charman, T. (2006). Social cognitive development during adolescence. Social Cognitive and Affective Neuroscience, 1(3), 165–174. https://doi.org/10.1093/scan/nsl024
- Crews, F., He, J., & Hodge, C. (2007). Adolescent cortical development: A critical period of vulnerability for addiction. Pharmacology Biochemistry and Behavior, 86(2), 189–199. https://doi.org/10.1016/j.pbb.2006.12.001
- Crone, E. A., & Dahl, R. E. (2012). Understanding adolescence as a period of social–affective engagement and goal flexibility. Nature Reviews Neuroscience, 13(9), 636–650. https://doi.org/10.1038/nrn3313
- *Cuttler, C., Connolly, C. P., LaFrance, E. M., & Lowry, T. M. (2018). Resist forgetting: Effects of aerobic and resistance exercise on prospective and retrospective memory. Sport Exercise and Performance Psychology, 7(2), 205–217. https://doi.org/10.1037/spy0000112
- Daly-Smith, A. J., Zwolinsky, S., Mckenna, J., Tomporowski, P. D., Defeyter, M. A., & Manley, A. (2018). Systematic review of acute physically active learning and classroom movement breaks on children’s physical activity, cognition, academic performance and classroom behaviour: Understanding critical design features. BMJ Open Sport & Exercise Medicine, 4, 341. https://doi.org/10.1136/bmjsem-2018-000341
- Day, J. R., Rossiter, H. B., Coats, E. M., Skasick, A., & Whipp, B. J. (2003). The maximally attainable VO2 during exercise in humans: The peak vs. maximum issue. Journal of Applied Physiology, 95(5), 1901–1907. https://doi.org/10.1152/japplphysiol.00024.2003
- de Houwer, J., Barnes-Holmes, D., & Moors, A. (2013). What is learning? On the nature and merits of a functional definition of learning. Psychonomic Bulletin and Review, 20(4), 631–642. https://doi.org/10.3758/s13423-013-0386-3
- *Del Giorno, J. M., Hall, E. E., O’Leary, K. C., Bixby, W. R., & Miller, P. C. (2010). Cognitive function during acute exercise: A test of the transient hypofrontality theory. Journal of Sport & Exercise Psychology, 32(3), 312–323. https://doi.org/10.1123/jsep.32.3.312
- Delignières, D., Brisswalter, J., & Legros, P. (1994). Influence of physical exercise on choice reaction time in sports experts: The mediating role of resource allocation. Journal of Human Movement Studies, 27(4), 173–188.
- *Delli Paoli, A. G., Smith, A. L., & Pontifex, M. B. (2017). Does walking mitigate affective and cognitive responses to social exclusion? Journal of Sport & Exercise Psychology, 39(2), 97–108. https://doi.org/10.1123/jsep.2016-0202
- Diamond, A. (2013). Executive functions. The Annual Review of Psychology, 64(1), 135–168. https://doi.org/10.1146/annurev-psych-113011-143750
- Dietrich, A. (2006). Transient hypofrontality as a mechanism for the psychological effects of exercise. Psychiatry Research, 145(1), 79–83. https://doi.org/10.1016/j.psychres.2005.07.033
- Drollette, E. S., Scudder, M. R., Raine, L. B., Moore, R. D., Saliba, B. J., Pontifex, M. B., & Hillman, C. H. (2014). Acute exercise facilitates brain function and cognition in children who need it most: An ERP study of individual differences in inhibitory control capacity. Developmental Cognitive Neuroscience, 7, 53–64. https://doi.org/10.1016/j.dcn.2013.11.001
- *Ellemberg, D., & St-Louis-Deschênes, M. (2010). The effect of acute physical exercise on cognitive function during development. Psychology of Sport and Exercise, 11(2), 122–126. https://doi.org/10.1016/j.psychsport.2009.09.006
- *Endo, K., Matsukawa, K., Liang, N., Nakatsuka, C., Tsuchimochi, H., Okamura, H., & Hamaoka, T. (2013). Dynamic exercise improves cognitive function in association with increased prefrontal oxygenation. The Journal of Physiological Sciences, 63(4), 287–298. https://doi.org/10.1007/s12576-013-0267-6
- Etnier, J. L., & Chang, Y.-K. (2009). The effect of physical activity on executive function: A brief commentary on definitions, measurement issues, and the current state of the literature. Article in Journal of Sport & Exercise Psychology, 31, 469–483. https://doi.org/10.1123/jsep.31.4.469
- Eysenck, M. W. (1976). Arousal, learning, and memory. Psychological Bulletin, 83(3), 389–404. https://doi.org/10.1037/0033-2909.83.3.389
- *Finch, L., Tomiyama, A., & Ward, A. (2017). Taking a stand: The effects of standing desks on task performance and engagement. International Journal of Environmental Research and Public Health, 14(8), 939. https://doi.org/10.3390/ijerph14080939
- Foulkes, L., & Blakemore, S. J. (2018). Studying individual differences in human adolescent brain development. Nature Neuroscience, 21(3), 315–323. https://doi.org/10.1038/s41593-018-0078-4
- Freeze, A. (2016, January 15). First graders in Paramus, New Jersey are fidgeting less thanks to pedaling desk cycles. ABC7 New York. https://abc7ny.com/paramus-new-jersey-first-grade-pedaling-desk-cycles/1160727/#:~:text=PARAMUS%2C New Jersey (WABC),desk and pedal looks promising!
- Friedman, N. P., & Miyake, A. (2004). The relations among inhibition and interference control functions: A latent-variable analysis. Journal of Experimental Psychology: General, 133(1), 101–135. https://doi.org/10.1037/0096-3445.133.1.101
- *Frodsham, K. M., Randall, N. R., Carbine, K. A., Rodeback, R. E., LeCheminant, J. D., & Larson, M. J. (2020). Does type of active workstation matter? A randomized comparison of cognitive and typing performance between rest, cycling, and treadmill active workstations. PLoS ONE, 15(8 August), e0237348. https://doi.org/10.1371/journal.pone.0237348
- Füzéki, E., Engeroff, T., & Banzer, W. (2017). Health benefits of light-intensity physical activity: A systematic review of accelerometer data of the national health and Nutrition examination survey (NHANES). Sports Medicine, 47(9), 1769–1793. https://doi.org/10.1007/s40279-017-0724-0
- Giles, G. E., Brunyé, T. T., Eddy, M. D., Mahoney, C. R., Gagnon, S. A., Taylor, H. A., & Kanarek, R. B. (2014). Acute exercise increases oxygenated and deoxygenated hemoglobin in the prefrontal cortex. NeuroReport, 25(16), 1320–1325. https://doi.org/10.1097/WNR.0000000000000266
- Gogtay, N., Giedd, J. N., Lusk, L., Hayashi, K. M., Greenstein, D., Vaituzis, A. C., Nugent IIIT. F., Herman, D. V., Clasen, L. S., Toga, A. W., Rapoport, J. L., & Thompson, P. M. (2004). Dynamic mapping of human cortical development during childhood through early adulthood. Proceedings of the National Academy of Sciences of the United States of America, 101(21), 8174–8179. https://doi.org/10.1073/pnas.0402680101
- *Grubaugh, J., & Rhea, C. K. (2014). Gait performance is not influenced by working memory when walking at a self-selected pace. Experimental Brain Research, 232(2), 515–525. https://doi.org/10.1007/s00221-013-3759-y
- Guthold, R., Cowan, M. J., Autenrieth, C. S., Kann, L., & Riley, L. M. (2010). Physical activity and sedentary behavior Among schoolchildren: A 34-country comparison. Journal of Pediatrics, 157(1), 43–49. https://doi.org/10.1016/j.jpeds.2010.01.019
- Guyatt, G. H., Oxman, A. D., Schünemann, H. J., Tugwell, P., & Knottnerus, A. (2011). GRADE guidelines: A new series of articles in the Journal of Clinical epidemiology. Journal of Clinical Epidemiology, 64, 380–382. https://doi.org/10.1016/j.jclinepi.2010.09.011
- *Harveson, A. T., Hannon, J. C., Brusseau, T. A., Podlog, L., Papadopoulos, C., Durrant, L. H., Hall, M. S., & Kang, K. (2016). Acute effects of 30 min resistance and aerobic exercise on cognition in a high school sample. Research Quarterly for Exercise and Sport, 87(2), 214–220. https://doi.org/10.1080/02701367.2016.1146943
- *Harveson, A. T., Hannon, J. C., Brusseau, T. A., Podlog, L., Papadopoulos, C., Hall, M. S., & Celeste, E. (2019). Acute exercise and academic achievement in middle school students. International Journal of Environmental Research and Public Health, 16(19), 3527. https://doi.org/10.3390/ijerph16193527
- *Hasegawa, T., Inoue, K., Tsutsue, O., & Kumashiro, M. (2001). Effects of a sit–stand schedule on a light repetitive task. International Journal of Industrial Ergonomics, 28(3–4), 219–224. https://doi.org/10.1016/S0169-8141(01)00035-X
- Hassevoort, K. M., Khan, N. A., Hillman, C. H., & Cohen, N. J. (2016). Childhood markers of health behavior relate to hippocampal health, memory, and academic performance. Mind, Brain, and Education, 10(3), 162–170. https://doi.org/10.1111/mbe.12108
- Hawkins, D. M. (2004). The problem of overfitting. Journal of Chemical Information and Computer Sciences, 44(1), 1–12. https://doi.org/10.1021/ci0342472
- *Haynes, A. T., Frith, E., Sng, E., & Loprinzi, P. D. (2019). Experimental effects of acute exercise on episodic memory function: Considerations for the timing of exercise. Psychological Reports, 122(5), 1744–1754. https://doi.org/10.1177/0033294118786688
- Holtzer, R., Mahoney, J. R., Izzetoglu, M., Izzetoglu, K., Onaral, B., & Verghese, J. (2011). fNIRS study of walking and walking while talking in young and old individuals. The Journals of Gerontology Series A: Biological Sciences and Medical Sciences, 66A(8), 879–887. https://doi.org/10.1093/gerona/glr068
- *Hötting, K., Schickert, N., Kaiser, J., Röder, B., & Schmidt-Kassow, M. (2016). The effects of acute physical exercise on memory, peripheral BDNF, and cortisol in young adults. Neural Plasticity, 2016, 6860573. https://doi.org/10.1155/2016/6860573
- Howley, E. T. (2001). Type of activity: Resistance, aerobic and leisure versus occupational physical activity. Medicine & Science in Sports & Exercise, 33(6), S364–S369. https://doi.org/10.1097/00005768-200106001-00005
- *Isip, M. I. G. (2014). Effect of a standing body position during college students’ exam: Implications on cognitive test performance. Industrial Engineering and Management Systems, 13(2), 185–192. https://doi.org/10.7232/iems.2014.13.2.185
- *Jaffery, A., Edwards, M. K., & Loprinzi, P. D. (2018). The effects of acute exercise on cognitive function: Solomon experimental design. Journal of Primary Prevention, 39(1), 37–46. https://doi.org/10.1007/s10935-017-0498-z
- *Joyce, J., Graydon, J., McMorris, T., & Davranche, K. (2009). The time course effect of moderate intensity exercise on response execution and response inhibition. Brain and Cognition, 71(1), 14–19. https://doi.org/10.1016/J.BANDC.2009.03.004
- Kahneman, D. (1973). Attention and effort. Prentice-Hall Inc.
- *Kamijo, K., Hayashi, Y., Sakai, T., Yahiro, T., Tanaka, K., & Nishihira, Y. (2009). Acute effects of aerobic exercise on cognitive function in older adults. The Journals of Gerontology Series B: Psychological Sciences and Social Sciences, 64B(3), 356–363. https://doi.org/10.1093/geronb/gbp030
- Kaplan, S., Kaplan, R., & Sampson, J. R. (1968). Encoding and arousal factors in free recall of verbal and visual material. Psychonomic Science, 12(2), 73–74. https://doi.org/10.3758/BF03331199
- Kleinsmith, L. J., & Kaplan, S. (1963). Paired-associate learning as a function of arousal and interpolated interval. Journal of Experimental Psychology, 65(2), 190. https://doi.org/10.1037/h0040288
- Knight, A. P., & Baer, M. (2014). Get up, stand up: The effects of a non-sedentary workspace on information elaboration and group performance. Social Psychological and Personality Science, 5(8), 910–917. https://doi.org/10.1177/1948550614538463
- Koechlin, E., Ody, C., & Kouneiher, F. (2003). The architecture of cognitive control in the human prefrontal cortex. Science, 302(5648), 1181–1185. https://doi.org/10.1126/science.1088545
- *Larson, M. J., LeCheminant, J. D., Carbine, K., Hill, K. R., Christenson, E., Masterson, T., & LeCheminant, R. (2015a). Slow walking on a treadmill desk does not negatively affect executive abilities: An examination of cognitive control, conflict adaptation, response inhibition, and post-error slowing. Frontiers in Psychology, 6, 723. https://doi.org/10.3389/fpsyg.2015.00723
- *Larson, M. J., LeCheminant, J. D., Hill, K., Carbine, K., Masterson, T., & Christenson, E. (2015b). Cognitive and typing outcomes measured simultaneously with slow treadmill walking or sitting: Implications for treadmill desks. PLoS ONE, 10(4), e0121309. https://doi.org/10.1371/journal.pone.0121309
- *Lindheimer, J. B., O’Connor, P. J., McCully, K. K., & Dishman, R. K. (2017). The effect of light-intensity cycling on mood and working memory in response to a randomized, placebo-controlled design. Psychosomatic Medicine, 79(2), 243–253. https://doi.org/10.1097/PSY.0000000000000381
- *Liu, F., Sulpizio, S., Kornpetpanee, S., & Job, R. (2017). It takes biking to learn: Physical activity improves learning a second language. PloS One, 12(5), e0177624. https://doi.org/10.1371/journal.pone.0177624
- *Lo Bue-Estes, C., Willer, B., Burton, H., Leddy, J. J., Wilding, G. E., & Horvath, P. J. (2008). Short-term exercise to exhaustion and its effects on cognitive function in young women. Perceptual and Motor Skills, 107(3), 933–945. https://doi.org/10.2466/PMS.107.7.933-945
- *Loprinzi, P. D., Day, S., & Deming, R. (2019). Acute exercise intensity and memory function: Evaluation of the transient hypofrontality hypothesis. Medicina, 55(8), 445. https://doi.org/10.3390/medicina55080445
- *Loprinzi, P. D., & Kane, C. J. (2015). Exercise and cognitive function: A randomized controlled trial examining acute exercise and free-living physical activity and sedentary effects. Mayo Clinic Proceedings, 90(4), 450–460. https://doi.org/10.1016/j.mayocp.2014.12.023
- *Loprinzi, P. D., Koehler, L., Frith, E., Ponce, P., Delancey, D., Joyner, C., Ashpole, N. M., Zou, L., & Li, H. (2019b). Acute exercise, psychological stress induction, and episodic memory. American Journal of Health Behavior, 43(6), 1016–1029. https://doi.org/10.5993/AJHB.43.6.1
- *Lowe, C. J., Kolev, D., & Hall, P. A. (2016). An exploration of exercise-induced cognitive enhancement and transfer effects to dietary self-control. Brain and Cognition, 110, 102–111. https://doi.org/10.1016/j.bandc.2016.04.008
- Mahar, M. T., Murphy, S. K., Rowe’, D. A., Golden, J., Shields, A. T., & Raedeke’, T. D. (2006). Effects of a classroom-based program on physical activity and on-task behavior. Medicine & Science in Sports & Exercise, 38(12), 2086–2094. https://doi.org/10.1249/01.mss.0000235359.16685.a3
- *Martins, A. Q., Kavussanu, M., Willoughby, A., & Ring, C. (2013). Moderate intensity exercise facilitates working memory. Psychology of Sport and Exercise, 14(3), 323–328. https://doi.org/10.1016/j.psychsport.2012.11.010
- Mavilidi, M. F., Ruiter, M., Schmidt, M., Okely, A. D., Loyens, S., Chandler, P., & Paas, F. (2018). A narrative review of school-based physical activity for enhancing cognition and learning: The importance of relevancy and integration. Frontiers in Psychology, 9, 2079. https://doi.org/10.3389/fpsyg.2018.02079
- Miyake, A., Friedman, N. P., Emerson, M. J., Witzki, A. H., Howerter, A., & Wager, T. D. (2000). The unity and diversity of executive functions and their contributions to complex “frontal lobe” tasks: A latent variable analysis. Cognitive Psychology, 41(1), 49–100. https://doi.org/10.1006/cogp.1999.0734
- Moher, D., Liberati, A., Tetzlaff, J., & Altman, D. G., & PRISMA Group (2009). Preferred reporting items for systematic reviews and meta-analyses: The PRISMA statement. PLoS Medicine, 6(7), e1000097. https://doi.org/10.1371/journal.pmed.1000097
- *O’Leary, K. C., Pontifex, M. B., Scudder, M. R., Brown, M. L., & Hillman, C. H. (2011). The effects of single bouts of aerobic exercise, exergaming, and videogame play on cognitive control. Clinical Neurophysiology, 122(8), 1518–1525. https://doi.org/10.1016/j.clinph.2011.01.049
- *Olson, R. L., Chang, Y.-K., Brush, C. J., Kwok, A. N., Gordon, V. X., & Alderman, B. L. (2016). Neurophysiological and behavioral correlates of cognitive control during low and moderate intensity exercise. NeuroImage, 131, 171–180. https://doi.org/10.1016/j.neuroimage.2015.10.011
- Pesce, C. (2009). An integrated approach to the effect of acute and chronic exercise on cognition: The linked role of individual and task constraints. In T. McMorris, P. D. Tomporowski, & M. Audiffren (Eds.), Exercise and cognitive function (pp. 213–226). John Wiley and Sons. https://doi.org/10.1002/9780470740668.ch11
- *Pilcher, J. J., & Baker, V. C. (2016). Task performance and meta-cognitive outcomes when using activity workstations and traditional desks. Frontiers in Psychology, 7, 957. https://doi.org/10.3389/fpsyg.2016.00957
- *Pilcher, J. J., Morris, D. M., Bryant, S. A., Merritt, P. A., & Feigl, H. B. (2017). Decreasing sedentary behavior: Effects on academic performance, meta-cognition, and sleep. Frontiers in Neuroscience, 11, 219. https://doi.org/10.3389/fnins.2017.00219
- *Pontifex, M. B., & Hillman, C. H. (2007). Neuroelectric and behavioral indices of interference control during acute cycling. Clinical Neurophysiology, 118(3), 570–580. https://doi.org/10.1016/j.clinph.2006.09.029
- Quas, J. A., & Lench, H. C. (2007). Arousal at encoding, arousal at retrieval, interviewer support, and children’s memory for a mild stressor. Applied Cognitive Psychology, 21(3), 289–305. https://doi.org/10.1002/acp.1279
- Rezende, L. F. M. d., Rodrigues Lopes, M., Rey-López, J. P., Matsudo, V. K. R., & Luiz, O. d. C. (2014). Sedentary behavior and health outcomes: An overview of systematic reviews. PLoS ONE, 9(8), e105620. https://doi.org/10.1371/journal.pone.0105620
- Rinkenauer, G., Osman, A., Ulrich, R., Müler-Gethmann, H., & Mattes, S. (2004). On the locus of speed-accuracy trade-off in reaction time: Inferences from the lateralized readiness potential. Journal of Experimental Psychology: General, 133(2), 261–282. https://doi.org/10.1037/0096-3445.133.2.261
- Rohde, T. E., & Thompson, L. A. (2007). Predicting academic achievement with cognitive ability. Intelligence, 35(1), 83–92. https://doi.org/10.1016/j.intell.2006.05.004
- Royall, D. R., Lauterbach, E. C., Cummings, J. L., Reeve, A., Rummans, T. A., Kaufer, D. I., LaFrance, C., & Coffey, C. E. (2002). Executive control function: a review of its promise and challenges for clinical research. A report from the Committee on Research of the American Neuropsychiatric Association. The Journal of Neuropsychiatry and Clinical Neurosciences, 14(4), 377–405. https://doi.org/10.1176/jnp.14.4.377
- Sawyer, S. M., Azzopardi, P. S., Wickremarathne, D., & Patton, G. C. (2018). The age of adolescence. The Lancet Child & Adolescent Health, 2(3), 223–228. https://doi.org/10.1016/S2352-4642(18)30022-1
- *Schmidt-Kassow, M., Zink, N., Mock, J., Thiel, C., Vogt, L., Abel, C., & Kaiser, J. (2014). Treadmill walking during vocabulary encoding improves verbal long-term memory. Behavioral and Brain Functions, 10(24), 1–9. https://doi.org/10.1186/1744-9081-10-24
- *Schramke, C. J., & Bauer, R. M. (1997). State-dependent learning in older and younger adults. Psychology and Aging, 12(2), 255–262. https://doi.org/10.1037/0882-7974.12.2.255
- Sherry, A. P., Pearson, N., & Clemes, S. A. (2016). The effects of standing desks within the school classroom: A systematic review. Preventive Medicine Reports, 3, 338–347. https://doi.org/10.1016/j.pmedr.2016.03.016
- Sibley, B. A., & Beilock, S. L. (2007). Exercise and working memory: An individual differences investigation. Journal of Sport and Exercise Psychology, 29(6), 783–791. https://doi.org/10.1123/jsep.29.6.783
- Singh, A. S., Saliasi, E., van den Berg, V., Uijtdewilligen, L., de Groot, R. H. M., Jolles, J., Andersen, L. B., Bailey, R., Chang, Y.-K., Diamond, A., Ericsson, I., Etnier, J. L., Fedewa, A. L., Hillman, C. H., McMorris, T., Pesce, C., Pühse, U., Tomporowski, P. D., & Chinapaw, M. J. M. (2018). Effects of physical activity interventions on cognitive and academic performance in children and adolescents: A novel combination of a systematic review and recommendations from an expert panel. British Journal of Sports Medicine, 53(10), 640–647. https://doi.org/10.1136/bjsports-2017-098136
- Sjöberg, H. (1980). Physical fitness and mental performance during and after work. Ergonomics, 23(10), 977–985. https://doi.org/10.1080/00140138008924807
- *Soga, K., Shishido, T., & Nagatomi, R. (2015). Executive function during and after acute moderate aerobic exercise in adolescents. Psychology of Sport and Exercise, 16, 7–17. https://doi.org/10.1016/J.PSYCHSPORT.2014.08.010
- St Clair-Thompson, H. L., & Gathercole, S. E. (2006). Executive functions and achievements in school: Shifting, updating, inhibition, and working memory. Quarterly Journal of Experimental Psychology (2006), 59(4), 745–759. https://doi.org/10.1080/17470210500162854
- Sterne, J. A. C., Savović, J., Page, M. J., Elbers, R. G., Blencowe, N. S., Boutron, I., Cates, C. J., Cheng, H. Y., Corbett, M. S., Eldridge, S. M., Hernán, M. A., Hopewell, S., Hróbjartsson, A., Junqueira, D. R., Jüni, P., Kirkham, J. J., Lasserson, T., Li, T., McAleenan, A., … Higgins, J. P. T. (2019). Rob 2: A revised tool for assessing risk of bias in randomised trials. BMJ, 366, I4898. https://doi.org/10.1136/bmj.l4898
- Stevens, C., & Bavelier, D. (2012). The role of selective attention on academic foundations: A cognitive neuroscience perspective. Developmental Cognitive Neuroscience, 2, S30–S48. https://doi.org/10.1016/j.dcn.2011.11.001
- Timinkul, A., Kato, M., Omori, T., Deocaris, C. C., Ito, A., Kizuka, T., Sakairi, Y., Nishijima, T., Asada, T., & Soya, H. (2008). Enhancing effect of cerebral blood volume by mild exercise in healthy young men: A near-infrared spectroscopy study. Neuroscience Research, 61(3), 242–248. https://doi.org/10.1016/j.neures.2008.03.012
- Tombu, M., & Jolicoeur, P. (2003). A central capacity sharing model of dual-task performance. Journal of Experimental Psychology: Human Perception and Performance, 29(1), 3–18. https://doi.org/10.1037/0096-1523.29.1.3
- Tomporowski, P. D., & Ellis, N. R. (1986). Effects of exercise on cognitive processes. A review. Psychological Bulletin, 99(3), 338–346. https://doi.org/10.1037/0033-2909.99.3.338
- Tremblay, M. S., Aubert, S., Barnes, J. D., Saunders, T. J., Carson, V., Latimer-Cheung, A. E., Chastin, S. F. M., Altenburg, T. M., & Chinapaw, M. J. M. (2017). Sedentary behavior research network (SBRN)–terminology consensus project process and outcome. International Journal of Behavioral Nutrition and Physical Activity, 14(1), 1–17. https://doi.org/10.1186/s12966-017-0525-8
- Tremblay, M. S., LeBlanc, A. G., Kho, M. E., Saunders, T. J., Larouche, R., Colley, R. C., Goldfield, G., & Gorber, S. (2011). Systematic review of sedentary behaviour and health indicators in school-aged children and youth. International Journal of Behavioral Nutrition and Physical Activity, 8(1), 98. https://doi.org/10.1186/1479-5868-8-98
- *Tsukamoto, H., Takenaka, S., Suga, T., Tanaka, D., Takeuchi, T., Hamaoka, T., Isaka, T., & Hashimoto, T. (2017). Effect of exercise intensity and duration on postexercise executive function. Medicine & Science in Sports & Exercise, 49(4), 774–784. https://doi.org/10.1249/MSS.0000000000001155
- Vygotsky, L. S. (2012). Thought and language. MIT press.
- Wallace, K. (2015, December 10). Forget “sit down!” students now standing up to learn. CNN. https://edition.cnn.com/2015/12/10/health/standing-desks-impact-health-education/index.html
- *Wang, C. C., Chu, C. H., Chu, I. H., Chan, K. H., & Chang, Y. K. (2013). Executive function during acute exercise: The role of exercise intensity. Journal of Sport & Exercise Psychology, 35(4), 358–367. https://doi.org/10.1123/jsep.35.4.358
- Wassenaar, T. M., Williamson, W., Johansen-Berg, H., Dawes, H., Roberts, N., Foster, C., & Sexton, C. E. (2020). A critical evaluation of systematic reviews assessing the effect of chronic physical activity on academic achievement, cognition and the brain in children and adolescents: A systematic review. International Journal of Behavioral Nutrition and Physical Activity, 17(1), 1–18. https://doi.org/10.1186/s12966-020-00959-y
- *Wick, K., Faude, O., Manes, S., Zahner, L., & Donath, L. (2018). I can stand learning: A controlled pilot intervention study on the effects of increased standing time on cognitive function in primary school children. International Journal of Environmental Research and Public Health, 15(2), 356. https://doi.org/10.3390/ijerph15020356
- Wilkerson, A. H., Bhochhibhoya, S., Dragicevic, A., & Umstattd Meyer, M. R. (2019). An ecological investigation of barriers and facilitators impacting standing desk use in real working conditions: A qualitative study. American Journal of Health Education, 50(5), 308–317. https://doi.org/10.1080/19325037.2019.1642266
- *Williams, L. R. T., Pottinger, P. R., & Shapcott, D. G. (1985). Effects of exercise on choice reaction latency and movement speed. Perceptual and Motor Skills, 60(1), 67–71. https://doi.org/10.2466/pms.1985.60.1.67
- *Yamazaki, Y., Sato, D., Yamashiro, K., Tsubaki, A., Yamaguchi, Y., Takehara, N., & Maruyama, A. (2017). Inter-individual differences in exercise-induced spatial working memory improvement: A near-infrared spectroscopy study. Advances in Experimental Medicine and Biology, 977, 81–88. https://doi.org/10.1007/978-3-319-55231-6_12
- Zhang, Z. (2014). Too much covariates in a multivariable model may cause the problem of overfitting. Journal of Thoracic Disease, 6(9), E196–E197. https://doi.org/10.3978/j.issn.2072-1439.2014.08.33
- *Zuniga, K. E., Mueller, M., Santana, A. R., & Kelemen, W. L. (2019). Acute aerobic exercise improves memory across intensity and fitness levels. Memory, 27(5), 628–636. https://doi.org/10.1080/09658211.2018.1546875