ABSTRACT
Green nanotechnology is an eco-friendly method for the biosynthesis of metallic nanoparticles, which are being used due to their unique features and bio-applications in various fields. World Health Organization (WHO) stated that the use of medicinal plants to treat a variety of disorders, such as diabetes mellitus (DM), cancer, HIV infection, hepatitis, tuberculosis (TB), and malaria is very effective. Green synthesis is a cost-effective, environment- friendly, and safe method. There are a wide variety of native medicinal plants around the world, owing to their unique physical, chemical, and biological characteristics, used for gold and silver nanoparticle (NP) synthesis, and are potent among all metal nanoparticles. However, as an alternative to several traditional techniques to synthesize nanoparticles, green chemistry has arisen. This review covers the green synthesis of AuNPs/AgNPs and its significance as a potent anticancer and antidiabetic agent. All literature surveys will be helpful in terms of further progress as to go from summarizing or concluding data along challenges and future perceptions.
GRAPHICAL ABSTRACT
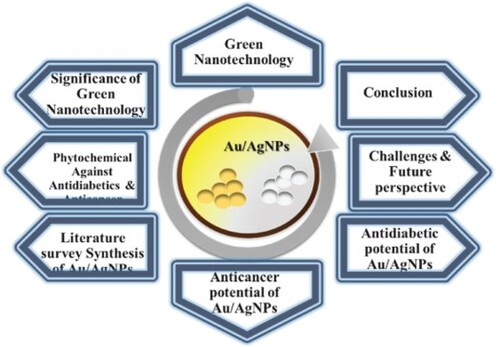
1. Introduction
The largest interdisciplinary fields (Citation1) that work at the nanoscale (1 to 100 nm) are known as nanoscience (Citation2) and nanotechnology (Citation3). The capacity of living cells, and how to alter materials at the nanoscale scale was first reported by Norio Taniguchi of Tokyo University in 1974 (Citation4). Nanoparticles have a large surface area (Citation5–7), which makes it easier for them to interact with various functional ligands (Citation8). Despite their tiny size (Citation9) they may be employed in a variety of fields (Citation10), such as biomedicals (Citation11), pharmaceutics (Citation12), and for the treatment of environmental hazards. A novel alternative to the traditional procedures (Citation13) for the production of nanoparticles, green nanotechnology is a clean, non-toxic, and environment-friendly method (Citation14). This also employs microorganisms (Citation15,Citation16), plant biomass, or extracts (Citation17). Metallic nanoparticles, such as silver (Ag) (Citation18), gold (Au) (Citation19), platinum (Pt) (Citation20), and palladium (Pd) (Citation21), have been thoroughly researched in recent years compared to bulk metals or metal ions (Citation22). Gold and silver metal nanoparticles (Citation23) have drawn a lot of interest in the fields of catalysis (Citation24), optics (Citation25), sensing (Citation26), imaging (Citation27), and biomedical devices (Citation28).
The synergistic capabilities of the plant and metal NPs (Citation29) are important in phyto-nanotherapy (Citation30) because they provide therapeutic benefits that may be clinically bioequivalent to those of many synthetic medicines (Citation31). Diabetes (Citation32), often known as diabetes mellitus or just diabetes, is a syndrome of excessively high blood sugar levels (Citation32) brought by a malfunctioning metabolism and also due to inherited environmental factors (hyperglycemia) (Citation33). According to data from the Centers for Disease Control (CDC), diabetes is the sixth largest cause of disease-related mortality in the U.S. (Citation34) and the third major cause among some ethnic communities. It is observed that its prevalence is growing at an alarming rate around the globe (Citation35). Diabetes was expected to be the fifth leading cause of death in the Americas in 2019 among persons aged 20 or older, which accounted for 409,000 deaths (Citation36).
One in six fatalities (or 9.6 million people) was caused by cancer in 2018, also helps in making it one of the top causes of death (Citation37). However, 70% of cancer deaths take place in middle- and low-income nations (Citation38). The most popular methods for treating and managing cancer are surgery (Citation39), chemotherapy (Citation40), radiation therapy (Citation41), and hormone therapy (Citation42). Diabetes mellitus patients are more likely to develop malignancies (Citation43) such as pancreatic (Citation44), colon (Citation45), liver (Citation46), kidney (Citation47), bladder (Citation48), and breast (Citation49). According to the estimates of WHO, 12.4 million individuals received a cancer diagnosis in 2008 (Citation50) and additionally, about 6.6% of people worldwide have diabetes. Patients who have both DM and cancer are more common and have several risk factors (Citation51,Citation52). Rapid insulin and insulin-like growth factor 1 (IGF-1) receptors (Citation53) are found in cancer cells (Citation54), and these receptors can help in cell survival (Citation55), proliferation (Citation56), mitogenesis (Citation57), and metastasis (Citation58). The discovery of treatments for cancer and diabetes has progressed over time, but access and the expense of treatment remain key issues.
Nanotechnology-based therapeutics (Citation59) and diagnostic methods (Citation60) have recently shown effective results for increasing cancer treatment (Citation61) and are also one of the most promising research topics that are currently seen as a frontier in medicine (Citation62). Since ancient times, the valuable metal silver has been employed extensively in medicine (Citation63) and the founder of modern medicine (Citation64) Hippocrates thought that silver could heal wounds (Citation65) and alleviate sickness (Citation66).
This review covered green nanotechnology its significance, phytochemicals effective against diabetes and cancer, synthesis of gold/silver nanoparticles along their anti-diabetic and anti-cancerous potential, and their future prospects.
2. Green synthesis of nanoparticles (NPs)
Plants are inexpensive, low-maintenance chemical factories of the nature (Citation67). There have been reports of the synthesis of AgNPs and AuNPs utilizing biological sources such as plants (Citation68), bacteria (Citation69), fungus (Citation70), lichen (Citation71), seaweed (Citation72), and algae (Citation73). This environmentally benign technique might offer an alternative to the chemical and physical synthesis of AgNPs (Citation74). In recent years, many plants have been employed effectively for the production of silver and gold nanoparticles outside the cells (Citation75). The synthetic yield of plant-assisted nanoparticle synthesis is much greater than those of other biosynthetic methods (Citation76). Due to the presence of various phytochemicals in different plant parts such as fruit, leaves, stems, and roots, they have been widely exploited for the green production of nanoparticles (Citation77). Chemical-reducing agents, such as hydrazine, sodium citrate, and sodium borohydride, were used to reduce the respective precursor salts to produce homogenous suspensions in the most typical production of metallic nanoparticles (Citation78).
The procedure for the synthesis of nanoparticles starts with gathering the desired plant's parts from accessible locations, cleaning them thoroughly twice or three times using tap water to get rid of dirt and soil, and then washing them again in sterile distilled water to get rid of any leftover particles (Citation79). These crisp, clean plant parts are dried under shade for 5–7 days before being ground in a home blender and the powder is stirred with distilled water to make the plant broth. A few mL of plant extracts and a 10−3 M AgNO3 solution allow for the reduction of pure Ag+ ions to Ag (Citation80) which can be observed by taking periodic readings of the solution's UV-visible spectra (Citation81). Plant extracts and microorganisms also function as reducing agents and capping or stabilizing agents, preventing the nanoparticles from aggregating (Citation82). The general method for the synthesis of nanoparticles by green technology is presented in .
3. Significance of green synthesis
Nanoparticle (NP) production mediated by plants is favored over synthesis by microorganisms (Citation83). The latter is impractical since it calls for more aseptic circumstances, a laborious procedure, and a longer incubation period (Citation84). The green production of silver and gold nanoparticles is now a developing and significant area of nanotechnology. It has become more popular since it is less harmful when compared to chemical risks (Citation85) and is economical along environment-friendly. Green synthesis compared to other methods, offers numerous advantages in the production of nanoparticles, such as ecologically safe, high yield, needs less time, easy to synthesize, and uses cheaper and less toxic solvents (Citation86). They also consume less energy and perform at moderate operating temperatures. According to this claim, silver nanoparticles produced by plants are more biologically active than those produced by other chemical processes (Citation87).
Green synthesis is straightforward and typically involves a one-pot reaction (Citation88). It is scaleable, toxic chemicals are removed, increasing the product's biocompatibility with healthy tissues for in vivo applications (Citation89, Citation90). This procedure is also economical, having several possibilities for other metallic NPs in biological and industrial applications. The uses of green-synthesized metallic NPs have been extensively reviewed (Citation91). Some of the most important significant factors that assist green synthesis are shown in .
4. Phytochemicals as potent antidiabetes and anticancerous agents
Plants contain high concentrations of secondary metabolites called phytochemicals that are thought to have little or no impact on the growth and nutrient requirements of plants. The chemical structure of the plant-based diabetes drugs i.e. berberine (Citation92), procyanidins (Citation93), calycosin (Citation94), nigellicimine (Citation95), thymohydroquinone (Citation96), isoquinoline (Citation97), camptothecin (Citation98), ferulic acid (Citation99), strychinine (Citation100), asaronic acid, ellagic acid (Citation101), cassiaside (Citation102), caffeic acid (Citation103), and apigenin (Citation104), are given in .
Many of these drugs have undergone modifications to produce more effective analogs in terms of activity, toxicity, or solubility. Phytochemicals that have been used against cancer such as vincristine (Citation105), vinblastine (Citation106), taxol (Citation107), podophyllotoxin (Citation108), combretastatin (Citation109), xanthatin (Citation110), geraniol (Citation111), curcumin (Citation112), flavonoids (Citation113), withanolide (Citation114), honokiol (Citation115), indrubin (Citation116), quercetin (Citation117), caffeic acid (Citation118), piperine (Citation113), emodin (Citation119), resveratrol (Citation120), catechins (Citation121), and phenethyl isocyanate (Citation122) have been reported several times by the researchers. Chemicals structures of anticancerous phytochemicals are presented in .
An extensive variety of secondary metabolites with various chemical structures are produced by plants that are essential for a variety of physiological processes and interactions with the environment. The likelihood of discovering substances is rising with particular anticancer characteristics. Compared to other synthesized anticancer medications, many plant-based secondary metabolites are potentially more biocompatible and less harmful to healthy human cells (Citation123). Plant-based secondary metabolites synergistically act with other chemical elements to enhance the medicinal benefits of the plant as a whole (Citation124). Some secondary metabolites may be more bioavailable than synthesized substances as to absorb more effectively in the body and interact with cancer cells. Secondary metabolites (>800), including norstictic acid, usnic acid, salazinic acid, chloroatranorin, atranorin, and stictic acid, are present only in lichen species that have potential pharmacological effects including anticancer, antibacterial, antiviral, analgesic, anti-inflammatory, and antigenotoxic activities (Citation125).
Although many bioactive secondary metabolites are often found in very low quantities, they make extraction of these compounds difficult and laborious (Citation126). Depending on variables including climatic circumstances, plant age, and geographic location, the composition of secondary metabolites in plants can change dramatically. The regularity and dependability of their anticancer action may be impacted by this fluctuation. In contrast to manufactured medications, plant extracts lack standardized compositions, which might lead to differences in therapeutic effectiveness and dose management (Citation127). Some secondary metabolites with anticancer effects could potentially be harmful to healthy cells and also finding a dosage that is both safe and effective might be difficult. Petroselinum crispum, Apium graveolens, and Matricaria recutita are only a few examples of the plant species that comprise apigenin that belongs to the flavone class and its effective formulations are difficult to obtain due to lower bioavailability and specificity (Citation128). The use of reserpine has decreased significantly, most likely as a result of its unclear mechanism of action and several deceptive side effects brought on by large doses of the drug (Citation129).
5. Green synthesis of AuNPs /AgNPs nanoparticles
A variety of plants are used for the synthesis of gold and silver nanoparticles, as reported by various researchers. A literature survey regarding the green synthesis of NPs will be helpful for the readers to get a deep insight into methodology, reaction conditioning, and optimization in a summarized way. Phytochemicals with reducing properties can facilitate the conversion of metal ions, such as silver ions (Ag+) or gold ions (Au+), into their corresponding metallic forms (Ag⁰ or Au⁰) (Citation130). These phytochemicals can donate electrons to the metal ions, thereby reducing them and leading to the formation of metallic particles (Citation131). This reduction process is often aided by the presence of appropriate reaction conditions, such as temperature, pH, and the presence of other compounds. Phytochemicals can also help stabilize the particles formed during the synthesis or reduction processes. Different phytochemicals may exhibit varying degrees of effectiveness in metal reduction and stabilization processes (Citation132). Metallic nanoparticles tend to aggregate or oxidize due to their high surface energy and reactivity. Phytochemicals can act as capping agents or surface stabilizers, preventing the particles from agglomerating or undergoing further oxidation (Citation133). By binding to the particle surfaces, these phytochemicals can form a protective layer and enhance the stability and dispersibility of the nanoparticles. Some examples of phytochemicals commonly studied in relation to metal reduction and stabilization include flavonoids, phenolics, terpenoids, and proteins (Citation134).
The green production of silver nanoparticles was accomplished using silver nitrate (AgNO3), normalized saline, Tween-80, and alcoholic extracts of lichens: Flavopunctelia flaventior, Xanthoria parietina, and deionized water by stirring at 80°C for 2 hrs. The synthesized nanoparticles were characterized by XRD technique (Citation71). Cumin oil, extracted from the seeds of the Nigella sativa L plant, has anti-diabetic potential and was used to synthesize AgNPs using 1 mM AgNO3 (90 mL) by combining with cumin oil (10 mL), and characterized using UV/Visible spectroscopy (Citation135). The Solanaceae family member; Datura stramonium seed extract (1 mL) along with 1 mM HAuCl3 (40 mL) employed for AuNPs synthesis and characterized with UV/Vis, FTIR, and SEM that disclosed that AuNPs were agglomerated in the form and were spherical (Citation136). The ethanolic extract of Bauhinia variegate was used for AuNP synthesis by the chemical reduction method using tetrachloroauric acid (HAuCl4) and trisodium citrate (Na3C6H5O7.2H2O). In the second step cetyltrimethylammonium bromide was used to promote stabilization (CTAB), TEM and XRD were used to characterize the AuNPs (Citation137). Moringa oleifera (MO) leaves were used to synthesize gold nanoparticles (AuNPs). The HAuCl4.3H2O (90 mL of 1.0 mM) aqueous solution was mixed with MO extract (10 mL) dropwise, and a shift in color from light yellow to ruby red, indicates the bio-reduction of Au3+. The SEM, EDS, and UV/Vis spectroscopy were used for characterization (Citation19). AuNPs were synthesized from the Asteraceae family using 3-(4,5-dimethylthiazol-2-yl)-2,5-diphenyltetrazolium bromide, and auric chloride (HAuCl4.3H2O). Characterization was done with the help of UV/Visible spectroscopy, XRD, FTIR, SEM, TEM, DLS, and AFM (Citation138). Solvents, such as ethanol, ethyl acetate, and hexane, were used for the crude extraction of Leucosida sericea followed by the purification of procyanidins via column chromatography. The purified phytochemical was used for the production of gold nanoparticles (Citation139). From the Fabaceae family, Au/AgNPs were synthesized using aqueous Bauhinia tomentosa leaf extract (5 mL), AgNO3 (1 mM), and HAuCl4. H2O (1 mM), color shifts from pale yellow to ruby red and from pale yellow to reddish brown indicated the synthesize of Au and Ag nanoparticles, respectively (Citation140). The family Moringaceae includes the plant Moringa oleifera was used to synthesize NPs using silver nitrate (AgNO3) and tetrachloroauric acid (HAuCl4) 2:3 ratio (Citation141). Gold chloride (HAuCl4) 4 mM, Marsdenia tenacissima leaf extract was mixed and diluted to 20 mL followed by incubation for 12 hrs resulting in the formation of the targeted compounds. Characterization techniques, such as XRD, SAED, EDAX, UV/Visible spectroscopy, and FTIR, were used to examine nanoparticles (Citation142). Abelmoschus esculentus (L) extract was prepared using triple-distilled water, chloroauric acid (4 mL) was added and stirred continuously for 6 hrs and left for 18 hrs. The color changed from colorless to light purple served as a proof for the synthesis of nanoparticles (Citation143). Taxus baccanta dried needles were used to make ethanolic (80%) extract, treated with chloroauric acid (4 mM) at 24oC for 24 hrs to synthesize nanoparticles (Citation144). Couroupita guianensis flowers were extracted by adding 50 mL of double-distilled water and mixed with chloroauric acid solutions in various ratios (1:9, 2:8, 3:7, 4:6, and 5:5) for 5 min. Utilizing a variety of cutting-edge techniques such as UV-vis spectroscopy, FTIR, XRD, SEM, and TEM, the nanoparticles were analyzed (Citation145). Dendropanax morbifera leaf (5 g) was boiled in 1000 mL of deionized water to make the leaf extract, and used for the synthesis of gold nanoparticles by mixing gold (III) chloride trihydrate. Centrifuged nanoparticles were then cleaned with deionized water and TEM, EDX, JEM, DLS, and XRD, as well as UV-vis spectroscopy were used for characterization (Citation146). Solanum xanthocarpum leave extract (5 mL) was added to auric chloride (45 mL of 1 mM solution), and the mixture was constantly agitated. In this process, Au + 3 is reduced to Au0, resulting in the formation of AuNPs (Citation147). The synthesis of colloidal mono-dispersed AuNPs (20 nm) with Withanolide-A from Withania somnifera plant AuCl3 (0.05%) with TSC (1%), TA (2.5 mM) and aq. K2CO3 (150 mM) was stirred for 5 min at 25oC. For conjugation, Withanolide-A (10 μg) was dissolved in AuNPs (1 mL) incubated overnight in cold room with gentle shaking and for stability, NaCl (10 µL of 10%) solution was added (Citation148). Plant leaves of Indigofera tinctoria were first washed, chopped, and cooked for 20 min in distilled water. To synthesize the AuNPs, the leaf extract (10 mL) was combined with the HAuCl4 (90 mL of a 1 mM) solution. A microwave oven with operating power and a frequency of 800 W and 2450 MHz, respectively, was used to heat the reaction mixture. UV-vis spectroscopy was used to keep track of the synthesis of AuNPs (Citation149). 10 gram of Mangifera indica (dry seeds) was extracted with water for 5 hrs and the extract was mixed with an auric chloride tetrahydrate (1 mM) solution in a 6:4 ratio. The appearance of ruby red to purple red color suggested the synthesis of nanoparticles. The techniques, such as TEM, EDAX, SEM, FTIR, and DLS, were used for the confirmation of the synthesized nanoparticles (Citation150). para-aminobenzoic acid-quat188-pullulan (PABA-QP) solution (700 μL of 6%) was mixed with HAuCl4 (280 μL of 5 mM) and stirred at 80°C for 1 h. After synthesis, the effects of pH (4–10), PABA-QP concentration (1–5%) and type of pullulan derivatives (P, QP and PABA-QP) were also evaluated to check the morphology and particle size of AuNP (Citation151). Gold nanoparticles were synthesized by combining 250 mL of 1 mM HAuCl4 with 50 ml of Stigmaphyllon ovatum leaf extract while stirring continuously for 1 h at 80°C. For further examination and characterization, the prepared nanoparticles were centrifuged, collected, and dried. Transmission electron microscopy (TEM), energy-dispersive X-ray (EDX), and UV/Vis spectroscopy were used to characterize the nanoparticles (Citation152). Crocin (700 mM) was extracted from the stigma of Iranian saffron, which was mixed with gold ions (0.5 mM) by varying concentrations, temperaturess (25 to 75°C), and reaction times (2 hrs 8 weeks), at constant pH (7.5) for the synthesis of gold nanoparticles. However, the morphologies were confirmed using XRD and TEM (Citation153). Musa acuminata flower extract (ethanol and water) was prepared by a Soxhlet apparatus. The hydrogen tetraaurochlorate (III) trihydrate (1 mM) reacted with extract (10 mL) at room temperature and synthesis of NPs was subsequently centrifuged, air-dried and characterized by XRD, SEM, FTIR, EDAX, and UV/Vis spectroscopy (Citation154). Aegle marmelos, Eugenia jambolana, and soursop extract were made from fruits (100 mg) mixed with hydrogen tatraauric (5 mM) separately. The mixture was agitated and the visual inspection proved that nanoparticle production had occurred (Citation155). A leaf extract of Antigonon leptopus was obtained from 8 g of the plant material at a boiled temperature for 30 min in 100 mL of deionized water. A hydrogen tetrachooro-aurate (60 mL of a 1 mM) solution and 8 mL of leaf extract were combined, and the resultant mixture was centrifuged and vacuum-dried at 30oC. AuNPs were studied utilizing the JEOL JEM 2100, EDX, XRD, FTIR, and UV-100 spectrophotometer (Citation156). A leaf extract of Stigmaphyllon ovatum (100 mL) was mixed with hydrogen tetrarchloroaurate (III) (500 mL of a 1 mM), the reaction mixture was stirred continuously at 85°C for 1 h to synthesize AuNPs. Transmission electron microscopy (TEM), X-ray diffraction (XRD), and Zetasizer Nano-ZS90 were used to analyze the nanoparticles (Citation157). Genipa americana fruit extract was combined with an auric chloride solution (10 ml of 0.5 mM) and a visual confirmation of gold nanoparticles came from color changes from pinkish red to ruby red. Transmission electron microscopy, UV-vis spectroscopy, electron ionization mass spectrometry, dynamic light scattering, quadrupole time-of-flight QTOF mass spectrometry, X-ray diffraction, and Fourier transform infrared spectroscopy were used to characterize the nanoparticles (Citation30). The extract of Juglans regia was mixed with iron nanoparticles, suspended in an aqueous solution of hydrogen tetrachooroaurate (III) in a 1:1 molar ratio to synthesize AuNPs. Numerous techniques such as HR-TEM, JEM-2100F, XRD, UV/Vis, vibrating sample magnetometer VSM, TGA, and FE-SEM were used to characterize the AuNPs (Citation158). The first step in the synthesis of AuNPs is the synthesis of a saffron extract, attained by boiling 50 mg of saffron in 50 mL of water for 15 min. The extract was mixed with HAuCl4 (20 mL of 0.5 mM) solution in an orbital shaker at 25oC. The confirmation of NPs synthesis came from a yellow to purple-color shift. Several analytical methods, such as TEM, SEM, UV/Vis, FTIR, XRD, were used to characterize the NPs (Citation159). Tanacetum vulgare fruit extract was made by boiling fruits (50 g) with water (250 mL) followed by mixing extract (1.8 mL) with an auric acid (50 mL of a 1 mM) solution. AuNP characterization with surface plasmon resonance (SPR) was achieved and cubic lattice of NPs was revealed (Citation160). Cathranthus roseus (CR) and Carica papaya (CP) leaves were washed, dried, pulverized, and extracted using the soxhlet equipment. Leaf extract in diverse concentrations (5–200 μL) treated with chloroauric acid (1 mM). XRD, HR-TEM, FTIR, and UV/Vis spectroscopy were used to analyze NPs (Citation161). Anacardium occidentale dried, powdered leaves (10 g), and double-distilled water (50 mL) were stirred overnight. The resulting extract was combined with a chloroauric acid (0.01M) solution and incubated overnight on a magnetic stirrer. Nanoparticles were characterized by UV-vis spectroscopy, PSA, TEM, XRD, SPR, and FTIR spectroscopy (Citation162). Dracocephalum kotschyi leaves (5 g) were extracted with deionized water (75 mL) and mixed with chloroauric acid (10 ml of a 1 mM) solution. The coloration change in the reaction mixture indicated the formation of the targeted particles (Citation163). A dried plant material of Rosa damascena (0.40 g) was extracted and treated with an aqueous solution of hydrogen tetrachlorocuprate (2 mL of 5 mM) that resulted in the synthesis of the targeted compounds. Various analytical methods, including TEM, SEM, UV/Vis spectroscopy, FTIR, X-ray diffraction XRD, and XPS, were used to characterize the nanoparticles (Citation164). The green synthesis of nanoparticles from different plant extracts is listed in .
Table 1. Green synthesis of silver and gold nanoparticles.
6. Antidiabetic potential of Au/AgNPs
The inhibition of alpha-amylase and glucosidase, two crucial enzymes in glucose metabolism, is one of the most essential approaches in the management of diabetes. The primary cause of the rise of glucose levels in blood, is the inhibition of amylase and glucosidase, which stops the breakdown of polysaccharides into monosaccharides. Along with starchy meals, an amylase inhibitor reduces the blood sugar spike that occurs regularly. In various investigations conducted both in vitro and in vivo, AgNPs are referred to be alpha-amylase inhibitors. Silver nanoparticles synthesized with leaf extract of Lonicera japonica were employed for α-amylase and α-glucosidase inhibition by DNSA method and IC50 value of α-amylase and α-glucosidase was found to be 54.56 and 37.86 µg, respectively in a dose-dependent manner. The %age inhibition of α-amylase with AgNPs at the dose of 20, 40, 60, 80 and 100 µg/mL was 40, 60, 70, 80 and 90% respectively. At the same concentration, α-glucosidase shows 40, 60, 80, 90 and 100% inhibition, respectively (Citation176). The activity of polyherbal formulation-mehani-based synthesized Ag and Fe (NPs) were compared using ascorbic acid as a standard. The 50 to 250 µg/mL of Ag, Fe and ascorbic acid was utilized for inhibition of α-amylase. At highest concentration (250 µg/ml), % inhibition of Ag, Fe and ascorbic acid was 60, 70.48 and 73.87% respectively. The results showed that FeNPs show high inhibition to the standard, as compared to AgNPs (Citation177).
Sargassum swartzii was used to synthesize gold NPs and evaluated as an anti-diabetic agent. The diabetes was introduced in rats via injection of alloxan (100 mg/kg bodyweight) and BGL was measured on 7th day of injection to confirm the presence of diabetes. AuNPs were inoculated around 0.5 mg/Kg body weight. It was found that blood glucose was lowered to 98 ± 7.2% in diabetic rats administered with AuNPs (Citation178). Cinnamomum tsoi extract-based AgNPs were used to inhibit the activity of α-amylase and α-glucosidase enzymes. The %age inhibition of Cinnamomum tsoi AgNPs was higher than simple extract (). The 10 and 75 µg/mL of AgNPs showed inhibition of 45 ± 0.83%, 93 ± 1.09% against α-amylase, respectively, while against α-glucosidase was 57 ± 1.35% and 80 ± 1.54%, respectively (Citation191). AuNPs were synthesized from Padina boergesenii extract and diabetic potential was evaluated. Control group contains DMSO, enzyme (α-glucosidase), extract NPs, while the positive group has acarbose. IC50 of AuNPs was 24 μg/mL while for acarbose, it was 39 μg/mL and AuNPs exhibit high potential than standard acarbose (Citation197). Ocimum basilicum and Ocimum sanctum were used to synthesize AgNPs and their inhibition against α-amylase was 59.79 ± 6.91% and 59.57 ± 3.72%, respectively, although for acarbose it was 48.27 ± 1.79% and their IC50 values were 0.016, 0.070, and 0.022 mg/mL, respectively. However against α-glucosidase, it was 89.31 ± 5.32%, 89.31 ± 5.32% and 73.75 ± 12.86%, respectively along with their IC50 values as 0.009, 1.533, and 0.001 mg/mL, respectively (Citation198). AgNPs were synthesized using seed extract of Alyssum homalocarpum and employed for the inhibition of α-amylase and α-glucosidase enzymes using acarbose as standard drug. IC50 value of acarbose and AgNPs against α-glucosidase was 16.9 and 18.01 µg/mL, respectively. However, AgNPs against α-amylase were moderately active with IC50 value 221.87 μg/mL in 100 mg/mL (Citation199). The dose-dependent inhibitory action of the cumin oil-mediated silver nanoparticles on the α-amylase enzyme was checked. The %age inhibition of the α-amylase with doses of 10–100 μg/mL was 30–90% (Citation135). AuNPs in Moringa oliefera extract were synthesized and their in-vitro antidiabetic activity suppressed the amylase activity in a concentration-dependent manner. It was found that by increasing the concentration, the activity was also increased and the IC50 value was 130 μg/mL (Citation19). Dimers, trimers procyanidins fractions, and Leucosidea sericea total extract (LSTE) were used for the synthesis of AuNPs having respective sizes of 24, 21 and 6 nm. The dimers of procyanidins-Au NPs showed the minimum IC50 value of 1.88 µg/mL and trimers of procyanidins-AuNPs showed the highest α-glucosidase activity with IC50 at 4.5 µg/mL (Citation139).
Table 2. Green synthesis of silver and gold nanoparticles and their antidiabetic activity.
7. Anticancerous potential of Au/AgNPs
The MTT test was performed to investigate the in vitro anticancer activity of the synthesized AgNPs, AuNPs, and aqueous leaf extract of Bauhinia tomentosa exhibited IC50 values for lung A-549 cell lines; 28.125, 46.875, and 50 μg/mL and for MCF-7 cell lines 62.5, 23.4, and 13.26 μg/ mL, and HEp-2 cell lines; 103.125, 34.375, and 53.125 μg/ mL, respectively (Citation140). The nanoparticles used to check the cytotoxicity of cell lines HepG2, MDA-MB-231, and MCF-7 were kept alive in a 5% CO2 and 37°C in CO2 incubators. However, AuNPs is found with higher cytotoxic probability having IC50 value ranged between 9.20 and 21.46 µg/mL in contrast to bimetallic (Ag-Au NPs) having IC50 value ranged from 37.22 to 49.94 µg/mL. However, the cytotoxic potential of AgNPs was not observed up to 60 µg/mL against all cell lines (Citation141). Nanoparticles prepared using A. hirsutus leave extract and effective conjugation of activated folic acid (FA) and chlorambucil (CHL) to AuNPs produced AuNPs-FA-CHL. IC50 value of AuNPs-FA-CHL on cancerous (RKO, HeLa, and A549) and normal cells was 170 and 130 g/mL, respectively. However, using lowered concentrations of AuNPs–FA–CHL is found to have higher anti-cancerous ability than CHL or AuNPs (Citation200). Cytotoxic potential of the gold nanoparticles from Marsdenia tenacissima (2.5–25 ug) in prostate cancer A549 cell and following the treatment, 20 mL of a dye combination containing ethidium bromide and acridine orange was applied to the well plates. After that, a fluorescent microscope was used to analyze the cells utilizing green and red fluorescence. The IC50 of AuNPs was 15 ug/mL. While at 25 ug/mL cell viability was only 25% (Citation142). Different cell lines, such as PBL, Jurkat (human acute myeloid leukemia), K562 and DL cells, were exposed to AuNPs. The AuNP-exposed Jurkat cell viability significantly decreased by 45.1% to 87.2% at 5-50 ug/mL doses. In the cases of K562 cells, viability was significantly decreased by 38.38% and 50.165% at 25 and 50 μg/mL doses while it was observed 28.51% and 48.16% for DL cells, respectively. On the other hand, no significant reduction of PBL viability was noted with doses of AuNPs up to 25 μg/mL (Citation143). Anticancer potential of AuNPs was observed by cytomorphological alterations of the cancer cell using optical microscopy, which disclosed that AuNPs induce the caspase-independent apoptotic route of cell death. The maximum cell mortality of 80%, 92%, and 98% was observed for Caov-4, MCF-7, and HeLa cancer cells, respectively, using 20 µg mL−1 ethanolic AuNPs for 72 hrs, while for aqueous extract it was 76%, 77%, and 84% respectively (Citation144). To evaluate the cytotoxic effects of nanoparticles by the MTT assay synthesized by C. guianensis, HL-60 cells were used. They were supplemented with 2 mg/L of NaHCO3, 4.5 mg/L of glucose, 100 mg/L each of streptomycin sulfate, gentamycin, and penicillin, as well as 10% of half-inactivated fetal calf serum in a humid environment with 5% CO2. AuNP flower extract in a concentration-dependent manner showed the (cytotoxicity concentration) CC50 value of 5.14 μM whereas for PBMC it was 113.25 μM (Citation145). Gold and silver nanoparticles using Dendropanax morbifera leaf had little to no cytotoxic impact on HaCaT and cancer cells but had a greater synergistic effect on A549 lung cancer cells. The results indicated that D-AgNPs exhibited less cytotoxicity in the human keratinocyte cell line at 100 µg/mL after 48 h while potent cytotoxicity in the lung cancer cell. However, both D-AgNPs and D-AuNPs at 50 µg/mL enhanced the cytotoxicity of ginsenoside compounds at 25 µM after 48 h of treatment as a compared compound alone (Citation146). Solanum xanthocarpum extract was used to synthesize AuNPs and cell viability was checked by MTT assay. Control C666-1 cells (100% viability) treated with NPs exhibited a round morphology and decreased in the formation of colonies found using different concentrations of 5, 10, and 15 µg/mL but just 20% colony formation was reported at high concentrations (Citation147). AuNPs cytotoxicity toward SKBR3 was compared to that of anticancer drugs using the MTT test in a dose-dependent manner. The anticancer compound with its varying concentration 10–40 µg/mL along with the same nanoconjugate concentration was applied and higher antiproliferative effects were observed. Cell viability was 30% in conjugation while alone it was 45% at the concentration of 40 µg/mL followed by 60% and 80% at 20 µg/mL, which might be due to higher uptake of the complex mixture in nucleus or cytoplasm functionalized AuNP carrier (Citation148). The MTT test on a lung cancer cell line (A549) was used to evaluate the anticancer effects of nanoparticles, signs of cell shrinkage found, and the sample inhibits cancer cell growth in a dose-dependent way. IC50 values of Indigofera tinctoria leaf extract, AgNP-tinctoria, and AuNP-tinctoria, respectively, were 71.92 ± 0.76 ug/mL, 56.62 ± 0.86 μg/mL, and 59.33 ± 0.57 μg/mL (Citation149). The ability of AuNPs to stop the proliferation of AGS cells and biocompatibility with rBM-MSCs was assessed. The metabolic activity was to be reduced by AuNPs for cancerous cells and normal cells beyond 10 ppb and by 25 ppb, respectively. AGS cell line treated with AuNPs for 24-72 hrs and MTT assay depicted a significant dose-dependent reduction in metabolic activity of 25–100 μg/mL. The normal cells (bone marrow-derived mesenchymal stem cells) were incubated with AuNPs and a reduction in metabolic function beyond 25 μg/mL was observed (Citation150).
Cell viability was studied on cervical carcinoma using MTT assay and synthesized AuCuO NPs 25 (μg/mL) was further evaluated as potential anticancer agents in HeLa cells. The IC50 values for CuO, Au-CuO nanomaterials, and the control drug (5-fluorouracil) were 12.3, 112, and 40 µM, respectively (Citation152). AuNPs with crocin embedded are effective against breast cancer cell lines using the MTT test. The IC50 values of crocin-AuNPs after 24 and 48 hrs of incubation were reported 1.8 ± 0.08 mg/mL and 1.2 ± 0.04 mg/mL, respectively (Citation153). Musa acuminata flower-based AuNPs and AgNPs were tested against anticancer activity by MTT using 200, 100, 50, 25, 12.5, 6.25, and 3.12 µg/mL concentrations. Normal vero cell line 50% viability was calculated as 55.0, 37.5, 27.5, and 35 µg/mL for AuNPs, aqueous, ethanol, and AgNPs, respectively (Citation154). NPs in varied quantities were applied to the MCF-7 cells. The human breast cancer cell showed 60% cell death at 120 µg/mL concentrations of AuNPs (Citation155). Cytotoxicity study of the synthesized AuNPs exhibited the growth inhibitory property at the concentration (GI50) 40 of 257.8 μg/mL in human adenocarcinoma breast cancer (MCF-7) cells after 48 hrs (Citation156). Stigmaphyllon ovatum leaf extract-based AuNPs were synthesized to reveal cytotoxic effects toward the growth of the HeLa cell carcinoma cells with IC50 values of 5.75 × 10−16 µM, 9.1 × 10−9 and 0.0027 µM for the Ag-Au BNPs, AgNPs and AuNPs, respectively in contrast to the standard drug, 5-fluorouracil with an IC50 value of 40 µM (Citation157). Catharanthus roseus and Carica papaya and the combination of these two extracts (CPCRM)-based AuNPs were used against the cell death of MCF-7 and HepG2 cells that gradually increases as the concentration increases. Doxorubicin (250 g/mL) was used as a positive control and significantly cell death was found at about 97.5% and 98.1% against MCF-7 and HepG2 cells, respectively (Citation161). Peripheral blood mononuclear cells (PBMC), the MCF-7 cell line, and control cells were placed in 24-well plates, and left to incubate for 24 hrs. Cell viability of 80.3% and 71.5% against PBMC cells and MCF-7 cells was seen at 100 μg. The IC50 values for PBMC and MCF-7 cells were about 600 and 6 µg/mL, respectively (Citation162). The MTT test was used to determine the cyto-survival assay using pure verbascoside- and VB-loaded gold nanoparticles in a medium containing 2% fetal bovine serum and the cell survival rate was 80%, 70%, and 57% for AuNPs, VB, and Au-VB, respectively. According to in-vivo investigation, VB-Au significantly slowed the growth of mouse tumors and caused the tumor cells to die (Citation201). Rosa damascene-based AuNPs (50 and 100 μg/mL) were used against A549 and HL60 cells to check cell viability which was determined after 72 hrs and the cell viability was reduced by almost 22% and 33% against A549 and HL60, respectively. The cytotoxicity effects toward HL60 cells (acute leukemia) are more sensitive than those of the A549 (adenocarcinoma) cells within the same test concentrations and incubation times which demonstrated that the nanoparticles activity was dose-dependent (Citation164). The anticancer potential of the polymer-based nanoparticles (PCL-F68-D-NPs) was evaluated using in-vitro models (Citation202). The green synthesis of gold and silver particle from different biological sources along their anticancer activity has been documented in .
Table 3. Green synthesis of silver and gold nanoparticles and their anticancer activity.
Gold and silver nanoparticles have received a lot of attention in cancer research due to their distinctive physicochemical properties and potential anticancer activities. Examples of typical mechanisms include the induction of apoptosis, or a method for planned cell death, by gold and silver nanoparticles, which have been studied (Citation225). These nanoparticles can activate signaling pathways, which in turn induce caspases to activate, break DNA, and ultimately lead to the controlled death of cancer cells (Citation226). Both gold and silver nanoparticles have the ability to produce reactive oxygen species (ROS) inside cancer cells (Citation227). Oxidative stress, which is brought on by elevated ROS levels and damages cellular components such as proteins, lipids, and DNA, can lead to apoptosis or other forms of cell death.
Gold and silver nanoparticles can interfere with mitochondrial activity, reducing energy output and triggering the release of pro-apoptotic proteins (Citation228). According to certain research, autophagy, a cellular process involved in the breakdown and recycling of cellular components, may be modulated by gold and silver nanoparticles. Particularly, the ability of gold nanoparticles to prevent angiogenesis is the growth of new blood vessels that feed tumors (Citation229). The immune system may interact with gold and silver nanoparticles, changing the tumor microenvironment. Gold and silver nanoparticles’ functionalization with organic moieties improves distinctive surface characteristics enabling them to interact with targeted ligands. As a result, the nanoparticles can more effectively transport drugs to cancer cells while delivering less damage to healthy cells (Citation230).
8. Challenges and future perspectives of green synthesis of NPs
The use of silver and gold nanoparticles as potential anticancer and antidiabetic agents holds promise, but there are several challenges and future perspectives that need to be considered. One of the primary challenges is ensuring the biocompatibility of silver and gold nanoparticles. While these nanoparticles exhibit therapeutic potential, their interactions with biological systems need to be carefully studied to avoid any toxicity or adverse effects. The efficient and targeted delivery of nanoparticles to specific cells or tissues remains a challenge. The development of strategies to specifically target cancer cells or diabetic tissues while minimizing off-target effects is crucial for effective therapeutic applications. Silver and gold nanoparticles can be prone to aggregation or instability in physiological conditions. To maintain their stability and preventing unwanted aggregation during storage and administration is important for ensuring their efficacy. The understanding of pharmacokinetics and bio-distribution of nanoparticles is essential for optimizing their therapeutic use. Factors, such as their size, surface properties, and clearance mechanisms, influence their distribution in the body and affect their efficacy. The introduction of silver and gold nanoparticles as therapeutic agents requires rigorous safety evaluation and regulatory approval. Demonstrating their efficacy and safety through preclinical and clinical studies is essential for their translation into clinical practice.
Silver and gold nanoparticles can be used in combination with existing therapies to enhance their efficacy. For example, nanoparticles can be used as drug delivery vehicles to improve the delivery and bioavailability of anticancer or antidiabetic drugs. To explore the synergistic effects of silver and gold nanoparticles with other therapeutic agents, such as natural compounds or other nanoparticles, may enhance their therapeutic potential. Combined treatments can potentially provide complementary mechanisms of action and improved outcomes. To tailor nanoparticle-based therapies to individual patients based on their unique genetic, molecular, or physiological characteristics could improve treatment outcomes. Personalized medicine approaches may involve nanoparticle formulations specific to patient subgroups or the use of nanoparticles for the targeted therapy. The advancements in characterization techniques, such as imaging modalities and spectroscopic methods, can provide valuable insights into the behavior and interactions of nanoparticles in biological systems. This knowledge can contribute to the optimization of nanoparticle-based therapies. The development of biodegradable or bioresorbable nanoparticles can address concerns related to long-term accumulation and potential toxicity. Biodegradable nanoparticles can be designed to degrade or be eliminated from the body after exerting their therapeutic effects. In summary, while there are challenges associated with the use of silver and gold nanoparticles as anticancer and antidiabetic agents, ongoing research, and advancements in various areas hold promise for their future applications. To understand the effects of temperature, concentration of the reducing agent, light, time, etc., on the synthesis of AuNPs, several tests must be carried out to optimize the synthesis of nanoparticles. The interaction and quadratic impacts of the different variables should be taken into account throughout the optimization process. Additionally, there are still unanswered problems regarding chemical composite knowledge and the process underlying the transformation and stability of AuNPs during bio-reduction. To address the mechanism of AuNP synthesis and its impact on the shape and size of AuNPs for a variety of applications, more studies and analyses are needed of this era.
9. Conclusions
Medicinal plants are rich source of the different bio-active compounds which can be used in the bio-synthesis of metallic nanoparticles by green approaches. With increasing the population of the global, diseases are also increasing with rapid speed and curing such disease in a challenge to the medicinal chemist. The green synthesis is more safe, more secure, and more economical than other approaches. Among the metallic nanoparticles, silver and gold are most studied due to their great potential for anticancer and antidiabetic. These nanomaterials have even comparative results toward the standard drugs. The exact mechanism about the mode of action for diabetic or cancer is still needed in detail.
Disclosure statement
No potential conflict of interest was reported by the author(s).
Additional information
Funding
References
- Milojević, S. Multidisciplinary Cognitive Content of Nanoscience and Nanotechnology. J. Nanopart. Res. 2012, 14 (1), 1–28.
- Zhu, S.; Meng, H.; Gu, Z.; Zhao, Y. Research Trend of Nanoscience and Nanotechnology–A Bibliometric Analysis of Nano Today. Nano. Today. 2021, 39, 101233.
- Kirtane, A.R.; Verma, M.; Karandikar, P.; Furin, J.; Langer, R.; Traverso, G. Nanotechnology Approaches for Global Infectious Diseases. Nat. Nanotechnol. 2021, 16 (4), 369–384.
- Farooq, B.; Anjum, S.; Farooq, M.; Rather, G.A.; Nazir, A.; Nayak, B.K.; Nanda, A. Role of Nanotechnology in Soil Microbiome and Agricultural Development. In Core Microbiome: Improving Crop Quality and Productivity, Wiley. 2022; pp 230–248.
- Borm, P.J.; Kreyling, W. Toxicological Hazards of Inhaled Nanoparticles—Potential Implications for Drug Delivery. J. Nanosci. Nanotechnol. 2004, 4 (5), 521–531.
- Najeeb, J.; Farwa, U.; Ishaque, F.; Munir, H.; Rahdar, A.; Nazar, M.F.; Zafar, M.N. Surfactant Stabilized Gold Nanomaterials for Environmental Sensing Applications–A Review. Environ. Res. 2022, 208, 112644.
- Bharadwaj, K.K.; Rabha, B.; Pati, S.; Sarkar, T.; Choudhury, B.K.; Barman, A.; Bhattacharjya, D.; Srivastava, A.; Baishya, D.; Edinur, H.A.; Abdul Kari, Z.; Mohd Noor, N.H. Green Synthesis of Gold Nanoparticles Using Plant Extracts as Beneficial Prospect for Cancer Theranostics. Molecules 2021, 26 (21), 6389.
- Zhao, Y.; Zhang, Z.; Pan, Z.; Liu, Y. Advanced Bioactive Nanomaterials for Biomedical Applications. Exploration. 2021, 1 (3), 0089.
- Hammami, I.; Alabdallah, N.M. Gold Nanoparticles: Synthesis Properties and Applications. J. King Saud Univ-Sci. 2021, 33 (7), 101560.
- Khan, I.; Saeed, K.; Khan, I. Nanoparticles: Properties, Applications and Toxicities. Arab. J. Chem. 2019, 12 (7), 908–931.
- Kalpana, V.; Devi Rajeswari, V. A Review on Green Synthesis: Biomedical Applications, and Toxicity Studies of ZnO NPs. Bioinorg. Chem. Appl. 2018, 3569758.
- Lou-Franco, J., Das, B.; Elliott, C.; Cao, C. Gold Nanozymes: From Concept to Biomedical Applications. Nano-micro Lett. 2021, 13 (1), 1–36.
- Shah, M.; Fawcett, D.; Sharma, S.; Tripathy, S.; Poinern, G. Green Synthesis of Metallic Nanoparticles via Biological Entities. Materials 2015, 8 (11), 7278–7308.
- Ullah, S.; Shah, S.W.A.; Qureshi, M.T.; Hussain, Z.; Ullah, I.; Kalsoom, U.-e.; Rahim, F.; Rahman, S.S.u.; Sultana, N.; Khan, M.K. Antidiabetic and Hypolipidemic Potential of Green AgNPs Against Diabetic Mice. ACS Appl. Bio. Mater. 2021, 4 (4), 3433–3442.
- Benko, A.; Truong, L. B.; Medina-Cruz, D.; Mostafavi, E.; Cholula-Díaz, J. L.; Webster, T. J. Green Nanotechnology in Cardiovascular Tissue Engineering, in Tissue Engineering. 2022; pp. 237–281.
- Yap, J.K.; Sankaran, R.; Chew, K.W.; Halimatul Munawaroh, H.S.; Ho, S.-H.; Rajesh Banu, J.; Show, P.L. Advancement of Green Technologies: A Comprehensive Review on the Potential Application of Microalgae Biomass. Chemosphere 2021, 281, 130886.
- Yayayürük, A.E.; Yayayürük, O. Recent Advances in Environmental Analysis Towards Green Nanomaterials. Curr. Anal. Chem. 2021, 17 (4), 449–460.
- Zubair, M.; Azeem, M.; Mumtaz, R.; Younas, M.; Adrees, M.; Zubair, E.; Khalid, A.; Hafeez, F.; Rizwan, M.; Ali, S. Green Synthesis and Characterization of Silver Nanoparticles from Acacia nilotica and Their Anticancer, Antidiabetic and Antioxidant Efficacy. Environ. Pollut. 2022, 304, 119249.
- Kiran, M., et al. Green Synthesis and Characterization of Gold Nanoparticles from Moringa oleifera Leaves and Assessment of Antioxidant, Antidiabetic and Anticancer Properties: 2021, 33. p. 100714.
- Hosny, M.; Fawzy, M.; El-Fakharany, E.M.; Omer, A.M.; El-Monaem, E.M.A.; Khalifa, R.E.; Eltaweil, A.S. Biogenic Synthesis, Characterization, Antimicrobial, Antioxidant, Antidiabetic, and Catalytic Applications of Platinum Nanoparticles Synthesized from Polygonum salicifolium Leaves. J. Env. Chem. Eng. 2022, 10 (1), 106806.
- Gulbagca, F.; Aygün, A.; Gülcan, M.; Ozdemir, S.; Gonca, S.; Şen, F. Green Synthesis of Palladium Nanoparticles: Preparation, Characterization, and Investigation of Antioxidant, Antimicrobial, Anticancer, and DNA Cleavage Activities. Appl. Organomet. Chem. 2021, 35 (8), e6272.
- Koutavarapu, R.; Tamtam, M.R.; Rao, M.C.; Peera, S.G.; Shim, J. Recent Progress in Transition Metal Oxide/Sulfide Quantum Dots-Based Nanocomposites for the Removal of Toxic Organic Pollutants. Chemosphere 2021, 272, 129849.
- Abdussalam-Mohammed, W. Comparison of Chemical and Biological Properties of Metal Nanoparticles (Au, Ag), with Metal Oxide Nanoparticles (ZnO-NPs) and Their Applications. Adv. J. Chem. Sect. A 2020, 3 (2), 111–236.
- Kamel, S.; Khattab, T.A. Recent Advances in Cellulose Supported Metal Nanoparticles as Green and Sustainable Catalysis for Organic Synthesis. Cellulose 2021, 28 (8), 4545–4574.
- Yang, S.-Z., et al. Recent Progress in the Optical Detection of Pathogenic Bacteria Based on Noble Metal Nanoparticles. Microchim. Acta 2021, 188 (8), 1–23.
- Montes-García, V.; Squillaci, M.A.; Diez-Castellnou, M.; Ong, Q.K.; Stellacci, F.; Samorì, P. Chemical Sensing with Au and Ag Nanoparticles. Chem. Soc. Rev. 2021, 50 (2), 1269–1304.
- Liu, M.; Anderson, R.; Lan, X.; Conti, P.S.; Chen, K. Recent Advances in the Development of Nanoparticles for Multimodality Imaging and Therapy of Cancer. Med. Res. Rev. 2020, 40 (3), 909–930.
- Liu, L.; Jiang, H.; Wang, X. Functionalized Gold Nanomaterials as Biomimetic Nanozymes and Biosensing Actuators. TrAC, Trends Anal. Chem. 2021, 143, 116376.
- Luan, S.; Xie, R.; Yang, Y.; Xiao, X.; Zhou, J.; Li, X.; Fang, P.; Zeng, X.; Yu, X.; Chen, M.; Gao, H.; Yuan, Y. Acid-Responsive Aggregated Gold Nanoparticles for Radiosensitization and Synergistic Chemoradiotherapy in the Treatment of Esophageal Cancer. Small 2022, 18 (19), 2200115.
- Dewanjee, S.; Chakraborty, P.; Mukherjee, B.; De Feo, V. Plant-based Antidiabetic Nanoformulations: The Emerging Paradigm for Effective Therapy. Int. J. Mol. Sci. 2020, 21 (6), 2217.
- Rautray, S.; Rajananthini, A.U. Therapeutic Potential of Green, Synthesized Gold Nanoparticles. BioPharm. Int 2020, 33, 30–38.
- Farwa, U.; Raza, M.A. Heterocyclic Compounds as a Magic Bullet for Diabetes Mellitus: A Review. RSC Adv. 2022, 12 (35), 22951–22973.
- Galicia-Garcia, U.; Benito-Vicente, A.; Jebari, S.; Larrea-Sebal, A.; Siddiqi, H.; Uribe, K.B.; Ostolaza, H.; Martín, C. Pathophysiology of Type 2 Diabetes Mellitus. Int. J. Mol. Sci. 2020, 21 (17), 6275.
- Barfield, W.D.; Warner, L. Preventing Chronic Disease in Women of Reproductive Age: Opportunities for Health Promotion and Preventive Services. Prev. Chronic Dis. 2012, 9.
- Sivaprasad, S.; Gupta, B.; Crosby-Nwaobi, R.; Evans, J. Prevalence of Diabetic Retinopathy in Various Ethnic Groups: A Worldwide Perspective. Surv. Ophthalmol. 2012, 57 (4), 347–370.
- Cousin, E., et al. Burden of Diabetes and Hyperglycaemia in Adults in the Americas, 1990–2019: A Systematic Analysis for the Global Burden of Disease Study 2019. Lancet Diabetes Endocrinol. 2022, 10 (9), 655–667.
- Ferreira, L.L.; Andricopulo, A.D. Cancer Estimates in Brazil Reveal Progress for the Most Lethal Malignancies. Curr. Top. Med. Chem. 2020, 20 (22), 1962–1966.
- Saygili, A. Classification and Diagnostic Prediction of Breast Cancers via Different Classifiers. Int Sci Vocational Stud J. 2018, 2 (2), 48–56.
- Liu, H.; Yang, D.; Chen, X.; Sun, Z.; Zou, Y.; Chen, C.; Sun, S. The Effect of Anticancer Treatment on Cancer Patients with COVID-19: A Systematic Review and Meta-Analysis. Cancer. Med. 2021, 10 (3), 1043–1056.
- Behranvand, N.; Nasri, F.; Zolfaghari Emameh, R.; Khani, P.; Hosseini, A.; Garssen, J.; Falak, R. Chemotherapy: A Double-Edged Sword in Cancer Treatment. Cancer Immunol. Immunother. 2022, 71 (3), 507–526.
- Aslonov, S. Modern Approaches to Oropharyngeal Cancer Therapy. Int. J. Discoveries Innov. Appl. Sci. 2021, 1 (3), 38–39.
- Gasparri, M.L.; Gentilini, O.D.; Lueftner, D.; Kuehn, T.; Kaidar-Person, O.; Poortmans, P. Changes in Breast Cancer Management During the Corona Virus Disease 19 Pandemic: An International Survey of the European Breast Cancer Research Association of Surgical Trialists (EUBREAST). The Breast 2020, 52, 110–115.
- Ramos-Garcia, P.; Roca-Rodriguez, M.d.M.; Aguilar-Diosdado, M.; Gonzalez-Moles, M.A. Diabetes Mellitus and Oral Cancer/Oral Potentially Malignant Disorders: A Systematic Review and Meta-Analysis. Oral Dis. 2021, 27 (3), 404–421.
- Zheng, Z.; Liu, Y.; Yang, J.; Tan, C.; Zhou, L.; Wang, X.; Xiao, L.; Zhang, S.; Chen, Y.; Liu, X. Diabetes Mellitus Induced by Immune Checkpoint Inhibitors. Diabetes Metab. Res. Rev. 2021, 37 (1), e3366.
- Bragagnoli, A.C.; Araujo, R.L.C.; Ferraz, M.W.; dos Santos, L.V.; Abdalla, K.C.; Comar, F.; Santos, F.A.; Oliveira, M.A.; Carvalheira, J.B.C.; Cárcano, F.M.; da Silveira Nogueira Lima, J.P. Metformin Plus Lrinotecan in Patients with Refractory Colorectal Cancer: A Phase 2 Clinical Trial. Br. J. Cancer 2021, 124 (6), 1072–1078.
- Vieira Barbosa, J.; Lai, M. Nonalcoholic Fatty Liver Disease Screening in Type 2 Diabetes Mellitus Patients in the Primary Care Setting. Hepatol. Commun. 2021, 5 (2), 158–167.
- Duan, Y.R.; Chen, B.; Chen, F.; Yang, S.; Zhu, C.; Ma, Y.; Li, Y.; Shi, J. LncRNA lnc-ISG20 Promotes Renal Fibrosis in Diabetic Nephropathy by Inducing AKT Phosphorylation Through miR-486-5p/NFAT5. J. Cell. Mol. Med. 2021, 25 (11), 4922–4937.
- Dobruch, J.; Oszczudłowski, M. Bladder Cancer: Current Challenges and Future Directions. Medicina 2021, 57 (8), 749.
- Shikata, K.; Ninomiya, T.; Kiyohara, Y. Diabetes Mellitus and Cancer Risk: Review of the Epidemiological Evidence. Cancer Sci. 2013, 104 (1), 9–14.
- Giovannucci, E.; Harlan, D.M.; Archer, M.C.; Bergenstal, R.M.; Gapstur, S.M.; Habel, L.A.; Pollak, M.; Regensteiner, J.G.; Yee, D. Diabetes and Cancer: A Consensus Report. Diabetes Care 2010, 33 (7), 1674–1685.
- Rawla, P.; Sunkara, T.; Gaduputi, V. Epidemiology of Pancreatic Cancer: Global Trends, Etiology and Risk Factors. World. J. Oncol. 2019, 10 (1), 10–27.
- Alam, S.; Hasan, M.K.; Neaz, S.; Hussain, N.; Hossain, M.F.; Rahman, T. Diabetes Mellitus: Insights from Epidemiology, Biochemistry, Risk Factors, Diagnosis, Complications and Comprehensive Management. Diabetology 2021, 2 (2), 36–50.
- Gram, M.; Ekström, C.; Holmqvist, B.; Carey, G.; Wang, X.; Vallius, S.; Hellström, W.; Ortenlöf, N.; Agyemang, A.A.; Smith, L.E.H.; Hellström, A.; Mangili, A.; Barton, N.; Ley, D. Insulin-Like Growth Factor 1 in the Preterm Rabbit Pup: Characterization of Cerebrovascular Maturation Following Administration of Recombinant Human Insulin-Like Growth Factor 1/Insulin-Like Growth Factor 1-Binding Protein 3. Dev. Neurosci. 2021, 43 (5), 281–295.
- Poreba, E.; Durzynska, J. Nuclear Localization and Actions of the Insulin-Like Growth Factor 1 (IGF-1) System Components: Transcriptional Regulation and DNA Damage Response. Mutat. Res./Rev. Mutat. Res. 2020, 784, 108307.
- Chughtai, S. The Nuclear Translocation of Insulin-Like Growth Factor Receptor and its Significance in Cancer Cell Survival. Cell Biochem. Funct. 2020, 38 (4), 347–351.
- Shirakawa, J.; Tajima, K.; Okuyama, T.; Kyohara, M.; Togashi, Y.; De Jesus, D.F.; Basile, G.; Kin, T.; Shapiro, A.M.J.; Kulkarni, R.N.; Terauchi, Y. Luseogliflozin Increases Beta Cell Proliferation Through Humoral Factors That Activate an Insulin Receptor-and IGF-1 Receptor-Independent Pathway. Diabetologia 2020, 63 (3), 577–587.
- Siech, C.; Rutz, J.; Maxeiner, S.; Grein, T.; Sonnenburg, M.; Tsaur, I.; Chun, F.K.-H.; Blaheta, R.A. Insulin-like Growth Factor-1 Influences Prostate Cancer Cell Growth and Invasion Through an Integrin α3, α5, αV, and β1 Dependent Mechanism. Cancers 2022, 14 (2), 363.
- Christopoulos, P.F.; Msaouel, P.; Koutsilieris, M. The Role of the Insulin-Like Growth Factor-1 System in Breast Cancer. Mol. Cancer 2015, 14 (1), 1–14.
- Krishnan, S.; Thirunavukarasu, A.; Jha, N.K.; Gahtori, R.; Roy, A.S.; Dholpuria, S.; Kesari, K.K.; Singh, S.K.; Dua, K.; Gupta, P.K. Nanotechnology-based Therapeutic Formulations in the Battle Against Animal Coronaviruses: An Update. J. Nanopart. Res. 2021, 23 (10), 1–16.
- Al-Joufi, F.A.; Setia, A.; Salem-Bekhit, M.; Sahu, R.; Alqahtani, F.; Widyowati, R.; Aleanizy, F. Molecular Pathogenesis of Colorectal Cancer with an Emphasis on Recent Advances in Biomarkers, as Well as Nanotechnology-Based Diagnostic and Therapeutic Approaches. Nanomaterials 2022, 12 (1), 169.
- Caracciolo, G.; Vali, H.; Moore, A.; Mahmoudi, M. Challenges in Molecular Diagnostic Research in Cancer Nanotechnology. Nano. Today. 2019, 27, 6–10.
- Zhang, R.; Liu, F.; Tian, Y.; Cao; W.; Wang, R. Nanotechnology in Traditional Medicines and Natural Products. Front. Media SA. 2021, 9, 633419.
- Medici, S.; Peana, M.; Nurchi, V.M.; Lachowicz, J.I.; Crisponi, G.; Zoroddu, M.A. Noble Metals in Medicine: Latest Advances. Coord. Chem. Rev. 2015, 284, 329–350.
- Tyrer, P., Mulder, R.; Newton-Howes, G.; Duggan, C. Galenic Syndromes: Combinations of Mental State and Personality Disorders Too Closely Entwined to be Separated. Br. J. Psychiatry 2022, 220 (6), 309–310.
- Toczek, J.; Sadłocha, M.; Major, K.; Stojko, R. Benefit of Silver and Gold Nanoparticles in Wound Healing Process After Endometrial Cancer Protocol. Biomedicines 2022, 10 (3), 679.
- Beers, E.H. Palliative Wound Care: Less is More. Surg. Clin. 2019, 99 (5), 899–919.
- Ojo, O.A., Olayide, I.I.; Akalabu, M.C.; Ajiboye, B.O.; Ojo, A.B.; Oyinloye, B.E.; Ramalingam, M. Nanoparticles and Their Biomedical Applications. Biointerface Res. Appl. Chem 2021, 11 (1), 8431–8445.
- Hassanisaadi, M.; Bonjar, G.H.S.; Rahdar, A.; Pandey, S.; Hosseinipour, A.; Abdolshahi, R. Environmentally Safe Biosynthesis of Gold Nanoparticles Using Plant Water Extracts. Nanomaterials 2021, 11 (8), 2033.
- Huq, M.A. Green Synthesis of Silver Nanoparticles Using Pseudoduganella eburnea MAHUQ-39 and Their Antimicrobial Mechanisms Investigation Against Drug Resistant Human Pathogens. Int. J. Mol. Sci. 2020, 21 (4), 1510.
- Clarance, P.; Luvankar, B.; Sales, J.; Khusro, A.; Agastian, P.; Tack, J.-C.; Al Khulaifi, M.M.; AL-Shwaiman, H.A.; Elgorban, A.M.; Syed, A.; Kim, H.-J. Green Synthesis and Characterization of Gold Nanoparticles Using Endophytic Fungi Fusarium solani and its in-Vitro Anticancer and Biomedical Applications. Saudi J. Biol. Sci. 2020, 27 (2), 706–712.
- Alqahtani, M.A.; Al Othman, M.R.; Mohammed, A.E. Bio Fabrication of Silver Nanoparticles with Antibacterial and Cytotoxic Abilities Using Lichens. Sci. Rep. 2020, 10 (1), 1–17.
- Kaithavelikkakath Francis, P.; Sivadasan, S.; Avarachan, A.; Gopinath, A. A Novel Green Synthesis of Gold Nanoparticles Using Seaweed Lobophora variegata and its Potential Application in the Reduction of Nitrophenols. Part. Sci. Technol. 2020, 38 (3), 365–370.
- El-Sheekh, M.M.; Hassan, L.H.; Morsi, H.H. Evaluation of Antimicrobial Activities of Blue-Green Algae-Mediated Silver and Gold Nanoparticles. Rendiconti Lincei. Scienze Fisiche e Naturali 2021, 32 (4), 747–759.
- Wahab, S.; Khan, T.; Adil, M.; Khan, A. Mechanistic Aspects of Plant-Based Silver Nanoparticles Against Multi-Drug Resistant Bacteria. Heliyon 2021, 7 (7), e07448.
- Amina, S.J.; Guo, B. A Review on the Synthesis and Functionalization of Gold Nanoparticles as a Drug Delivery Vehicle. Int. J. Nanomed. 2020, 15, 9823–9857.
- Lade, B.D.; Shanware, A.S., Phytonanofabrication: Methodology and Factors Affecting Biosynthesis of Nanoparticles, in Smart Nanosystems for Biomedicine, Optoelectronics and Catalysis. 2020, IntechOpen.
- Jadoun, S.; Arif, R.; Jangid, N.K.; Meena, R.K. Green Synthesis of Nanoparticles Using Plant Extracts: A Review. Environ. Chem. Lett. 2021, 19 (1), 355–374.
- Lyalina, T.; Lunkov, A.; Varlamov, V. Obtaining of Metal Nanoparticles Using Reducing Agents and Chitosan. Appl. Biochem. Microbiol. 2022, 58 (2), 97–104.
- Sikiru, S., Abiodun, O. A.; Sanusi, Y. K.; Sikiru, Y. A.; Soleimani, H.; Yekeen, N.; Haslija, A. A. A Comprehensive Review on Nanotechnology Application in Wastewater Treatment a Case Study of Metal-Based Using Green Synthesis. J. Env. Chem. Eng. 2022, 10 (4), 108065.
- Mehta, K.; Balaraman, R.; Amin, A.H.; Bafna, P.A.; Gulati, O.D. Effect of Fruits of Moringa oleifera on the Lipid Profile of Normal and Hypercholesterolaemic Rabbits. J. Ethnopharmacol. 2003, 86 (2-3), 191–195.
- Singh, R.K.; Behera, S.S.; Singh, K.R.; Mishra, S.; Panigrahi, B.; Sahoo, T.R.; Parhi, P.K.; Mandal, D. Biosynthesized Gold Nanoparticles as Photocatalysts for Selective Degradation of Cationic dye and Their Antimicrobial Activity. J. Photochem. Photobiol., A 2020, 400, 112704.
- Albeladi, S.S.R.; Malik, M.A.; Al-thabaiti, S.A. Facile Biofabrication of Silver Nanoparticles Using Salvia Officinalis Leaf Extract and its Catalytic Activity Towards Congo red dye Degradation. J. Mater. Res. Technol. 2020, 9 (5), 10031–10044.
- Santos, T.S.; Silva, T.M.; Cardoso, J.C.; Albuquerque-Júnior, R.L.C.d.; Zielinska, A.; Souto, E.B.; Severino, P.; Mendonça, M.d.C. Biosynthesis of Silver Nanoparticles Mediated by Entomopathogenic Fungi: Antimicrobial Resistance, Nanopesticides, and Toxicity. Antibiotics 2021, 10 (7), 852.
- Kumari, S.; Selvakumar, V.; Padma, P.N.; Anuradha, K. Optimization Studies on Green Synthesis of Silver Nanoparticles from Different Plant Extracts Using Taguchi Design. Indian J. Sci. Technol 2021, 14, 2888–2898.
- Khan, S.H. Green Nanotechnology for the Environment and Sustainable Development, in Green Materials for Wastewater Treatment; Springer: 2020. p. 13–46.
- Sivakumar, T. A Modern Review of Silver Nanoparticles Mediated Plant Extracts and its Potential Bioapplications. Int. J. Bot. Stud 2021, 6 (3), 170–175.
- Castillo-Henríquez, L.; Alfaro-Aguilar, K.; Ugalde-Álvarez, J.; Vega-Fernández, L.; Montes de Oca-Vásquez, G.; Vega-Baudrit, J.R. Green Synthesis of Gold and Silver Nanoparticles from Plant Extracts and Their Possible Applications as Antimicrobial Agents in the Agricultural Area. Nanomaterials 2020, 10 (9), 1763.
- Zhuang, W.; Liu, X.; Chen, L.; Liu, P.; Wen, H.; Zhou, Y.; Wang, J. One-pot Hydrothermal Synthesis of Ultrafine Pd Clusters Within Beta Zeolite for Selective Oxidation of Alcohols. Green Chem. 2020, 22 (13), 4199–4209.
- Arabi, M.; Ostovan, A.; Li, J.; Wang, X.; Zhang, Z.; Choo, J.; Chen, L. Molecular Imprinting: Green Perspectives and Strategies. Adv. Mater. 2021, 33 (30), 2100543.
- Wibowo, A.; Tajalla, G.U.N.; Marsudi, M.A.; Cooper, G.; Asri, L.A.T.W.; Liu, F.; Ardy, H.; Bartolo, P.J.D.S. Green Synthesis of Silver Nanoparticles Using Extract of Cilembu Sweet Potatoes (Ipomoea batatas L var. Rancing) as Potential Filler for 3D Printed Electroactive and Anti-Infection Scaffolds. Molecules 2021, 26 (7), 2042.
- Parmar, M.; Sanyal, M. Extensive Study on Plant Mediated Green Synthesis of Metal Nanoparticles and Their Application for Degradation of Cationic and Anionic Dyes. Env. Nanotechnol. Monitor Manage. 2022, 17, 100624.
- Ma, X.; Chen, Z.; Wang, L.; Wang, G.; Wang, Z.; Dong, X.; Wen, B.; Zhang, Z. The Pathogenesis of Diabetes Mellitus by Oxidative Stress and Inflammation: Its Inhibition by Berberine. Front. Pharmacol. 2018, 9, 782.
- Osakabe, N.; Yamagishi, M.; Natsume, M.; Yasuda, A.; Osawa, T. Ingestion of Proanthocyanidins Derived from Cacao Inhibits Diabetes-Induced Cataract Formation in Rats. Exp. Biol. Med. 2004, 229 (1), 33–39.
- Han, L.; Song, J.; Yan, C.; Wang, C.; Wang, L.; Li, W.; Du, Y.; Li, Q.; Liang, T. Inhibitory Activity and Mechanism of Calycosin and Calycosin-7-O-β-D-Glucoside on α-Glucosidase: Spectroscopic and Molecular Docking Analyses. Process Biochem. 2022, 118, 227–235.
- Veeramani, S.; Narayanan, A.P.; Yuvaraj, K.; Sivaramakrishnan, R.; Pugazhendhi, A.; Rishivarathan, I.; Jose, S.P.; Ilangovan, R. Nigella sativa Flavonoids Surface Coated Gold NPs (Au-NPs) Enhancing Antioxidant and Anti-Diabetic Activity. Process Biochem. 2022, 114, 193–202.
- Butt, A.S.; Nisar, N.; Mughal, T. A Review: Therapeutics Potentials of Phytochemical Drugs and Their Loading in pH Specific Degradable Nano-Drug Carrier Targeting Colorectal Cancer. J. Pak. Med. Assoc. 2018, 68 (4), 607–614.
- Gaikwad, B.; Switi, G.; Mohan, K.; Sandhya Rani, M. Phytochemicals for Diabetes Management. Pharmaceutical Crops 2014, 5 (1), 11–28.
- Suhitha, S.; Devi, S.; Gunasekaran, K.; Pakyntein, H.; Bhattacharjee, A.; Velmurugan, D. Phytochemical Analyses and Activity of Herbal Medicinal Plants of North-East India for Anti-Diabetic, Anti-Cancer and Anti-Tuberculosis and Their Docking Studies. Curr. Top. Med. Chem. 2015, 15 (1), 21–36.
- Nankar, R.; Prabhakar, P.; Doble, M. Hybrid Drug Combination: Combination of Ferulic Acid and Metformin as Anti-Diabetic Therapy. Phytomedicine 2017, 37, 10–13.
- Maddisetty, P.P.; Doss, VA. HPLC and GC-MS: Identification and Quantification of Strychnine and Brucine in Strychnos nux-vomica Leaves. Pak. J. Pharm. Sci. 2017, 30 (6 (Supplementary)), 2369–2373.
- Jeevanandam, J.; Madhumitha, R.; Saraswathi, N. Identification of Potential Phytochemical Lead Against Diabetic Cataract: An Insilico Approach. J. Mol. Struct. 2021, 1226, 129428.
- Lee, G.Y.; Jang, D.S.; Lee, Y.M.; Kim, J.M.; Kim, J.S. Naphthopyrone Glucosides from the Seeds of Cassia tora with Inhibitory Activity on Advanced Glycation end Products (AGEs) Formation. Arch. Pharmacal Res. 2006, 29 (7), 587–590.
- Mousavi, L.; Salleh, R.M.; Murugaiyah, V. Phytochemical and Bioactive Compounds Identification of Ocimum Tenuiflorum Leaves of Methanol Extract and its Fraction with an Anti-Diabetic Potential. Int. J. Food Prop. 2018, 21 (1), 2390–2399.
- Shukla, R.; Kashaw, S.K.; Jain, A.P.; Lodhi, S. Fabrication of Apigenin Loaded Gellan gum–Chitosan Hydrogels (GGCH-HGs) for Effective Diabetic Wound Healing. Int. J. Biol. Macromol. 2016, 91, 1110–1119.
- Mohammad Nabavi, S., et al. Apigenin and Breast Cancers: From Chemistry to Medicine. Anti-Cancer Agents Med. Chem 2015, 15 (6), 728–735.
- Rajabalian, S. Methanolic Extract of Teucrium polium L Potentiates the Cytotoxic and Apoptotic Effects of Anticancer Drugs of Vincristine, Vinblastine and Doxorubicin Against a Panel of Cancerous Cell Lines. Exp. Oncol. 2008.
- Mishra, S.; Verma, S.S.; Rai, V.; Awasthee, N.; Chava, S.; Hui, K.M.; Kumar, A.P.; Challagundla, K.B.; Sethi, G.; Gupta, S.C. Long non-Coding RNAs are Emerging Targets of Phytochemicals for Cancer and Other Chronic Diseases. Cell. Mol. Life Sci. 2019, 76 (10), 1947–1966.
- Kusari, S.; Zühlke, S.; Spiteller, M. Chemometric Evaluation of the Anti-Cancer pro-Drug Podophyllotoxin and Potential Therapeutic Analogues in Juniperus and Podophyllum Species. Phytochem. Anal. 2011, 22 (2), 128–143.
- Choudhari, A.S.; Mandave, P.C.; Deshpande, M.; Ranjekar, P.; Prakash, O. Phytochemicals in Cancer Treatment: From Preclinical Studies to Clinical Practice. Front. Pharmacol. 2020, 10, 1614.
- Ramirez-Erosa, I.; Huang, Y.; Hickie, R.A.; Sutherland, R.G.; Barl, B. Xanthatin and Xanthinosin from the Burs of Xanthium strumarium L. as Potential Anticancer Agents. Can. J. Physiol. Pharmacol. 2007, 85 (11), 1160–1172.
- Gaonkar, R.; Singh, J.; Chauhan, A.; Avti, P.K.; Hegde, G. Geraniol and Citral as Potential Therapeutic Agents Targeting the HSP90 Activity: An in Silico and Experimental Approach. Phytochemistry 2022, 195, 113058.
- Barati, N.; Momtazi-Borojeni, A.A.; Majeed, M.; Sahebkar, A. Potential Therapeutic Effects of Curcumin in Gastric Cancer. J. Cell. Physiol. 2019, 234 (3), 2317–2328.
- Zheng, Z.; Zhang, L.; Hou, X. Potential Roles and Molecular Mechanisms of Phytochemicals Against Cancer. Food Funct. 2022, 13 (18), 9208–9225.
- Huang, M.; He, J.-X.; Hu, H.-X.; Zhang, K.; Wang, X.-N.; Zhao, B.-B.; Lou, H.-X.; Ren, D.-M.; Shen, T. Withanolides from the Genus Physalis: A Review on Their Phytochemical and Pharmacological Aspects. J. Pharm. Pharmacol. 2020, 72 (5), 649–669.
- Averett, C., Arora, S.; Zubair, H.; Singh, S.; Bhardwaj A.; Singh A.P. Molecular Targets of Honokiol: A Promising Phytochemical for Effective Cancer Management, in The Enzymes. 2014; pp. 175–193.
- Li, Y., et al. Anticancer Effects of Natural Phytochemicals in Anaplastic Thyroid Cancer. Oncol. Rep. 2022, 48 (3), 1–13.
- Tavana, E.; Mollazadeh, H.; Mohtashami, E.; Modaresi, S.M.S.; Hosseini, A.; Sabri, H.; Soltani, A.; Javid, H.; Afshari, A.R.; Sahebkar, A. Quercetin: A Promising Phytochemical for the Treatment of Glioblastoma Multiforme. BioFactors 2020, 46 (3), 356–366.
- Mirzaei, S.; Gholami, M.H.; Zabolian, A.; Saleki, H.; Farahani, M.V.; Hamzehlou, S.; Far, F.B.; Sharifzadeh, S.O.; Samarghandian, S.; Khan, H.; Aref, A.R.; Ashrafizadeh, M.; Zarrabi, A.; Sethi, G. Caffeic Acid and its Derivatives as Potential Modulators of Oncogenic Molecular Pathways: New Hope in the Fight Against Cancer. Pharmacol. Res. 2021, 171, 105759.
- Ramakrishnan, P.; Loh, W.M.; Gopinath, S.C.B.; Bonam, S.R.; Fareez, I.M.; Mac Guad, R.; Sim, M.S.; Wu, Y.S. Selective Phytochemicals Targeting Pancreatic Stellate Cells as new Anti-Fibrotic Agents for Chronic Pancreatitis and Pancreatic Cancer. Acta Pharm. Sin. B 2020, 10 (3), 399–413.
- Patra, S.; Pradhan, B.; Nayak, R.; Behera, C.; Rout, L., Jena, M.; Efferth, T.; Bhutia S.K.. Chemotherapeutic Efficacy of Curcumin and Resveratrol Against Cancer: Chemoprevention, Chemoprotection, Drug Synergism and Clinical Pharmacokinetics. in Seminars in Cancer Biology. 2021. Elsevier.
- Cheng, Z.; Zhang, Z.; Han, Y.; Wang, J.; Wang, Y.; Chen, X.; Shao, Y.; Cheng, Y.; Zhou, W.; Lu, X.; Wu, Z. A Review on Anti-Cancer Effect of Green tea Catechins. J. Funct. Foods 2020, 74, 104172.
- Adetuyi, B.O., et al. Preventive Phytochemicals of Cancer as Speed Breakers in Inflammatory Signaling. Res. J. Life Sci. Bioinform. Pharm. Chem. Sci. 2022, 8 (1), 30–61.
- Javed, B.; Ikram, M.; Farooq, F.; Sultana, T.; Mashwani, Z.-u.-R.; Raja, N.I. Biogenesis of Silver Nanoparticles to Treat Cancer, Diabetes, and Microbial Infections: A Mechanistic Overview. Appl. Microbiol. Biotechnol. 2021, 105, 2261–2275.
- Gorelick, J.; Bernstein, N. Elicitation: An Underutilized Tool in the Development of Medicinal Plants as a Source of Therapeutic Secondary Metabolites. Adv. Agron. 2014, 124, 201–230.
- Alavi, M.; Hamblin, M.R.; Kennedy, J.F. Antimicrobial Applications of Lichens: Secondary Metabolites and Green Synthesis of Silver Nanoparticles: A Review. Nano Micro Biosystems 2022, 1 (1), 15–21.
- Antonio, A.; Wiedemann, L.; Junior, V.V. The Genus Capsicum: A Phytochemical Review of Bioactive Secondary Metabolites. RSC Adv. 2018, 8 (45), 25767–25784.
- Oyenihi, A.; Smith, C. Are Polyphenol Antioxidants at the Root of Medicinal Plant Anti-Cancer Success? J. Ethnopharmacol. 2019, 229, 54–72.
- Javid, H.; Ahmadi, S.; Mohamadian, E. Therapeutic Applications of Apigenin and its Derivatives: Micro and Nano Aspects. Micro. Nano. Bio. Aspects 2023, 2 (1), 30–38.
- Verma, K.; Paliwal, S.; Sharma, S. Therapeutic Potential of Reserpine in Metabolic Syndrome: An Evidence Based Study. Pharmacol. Res. 2022, 186, 106531.
- Rana, A.; Yadav, K.; Jagadevan, S. A Comprehensive Review on Green Synthesis of Nature-Inspired Metal Nanoparticles: Mechanism, Application and Toxicity. J. Cleaner Prod. 2020, 272, 122880.
- Ahmad, T.; Bustam, M.A.; Irfan, M.; Moniruzzaman, M.; Asghar, H.M.A.; Bhattacharjee, S. Mechanistic Investigation of Phytochemicals Involved in Green Synthesis of Gold Nanoparticles Using Aqueous Elaeis guineensis Leaves Extract: Role of Phenolic Compounds and Flavonoids. Biotechnol. Appl. Biochem. 2019, 66 (4), 698–708.
- Yuliarto, B.; Septiani, N.L.W.; Kaneti, Y.V.; Iqbal, M.; Gumilar, G.; Kim, M.; Na, J.; Wu, K.C.-W.; Yamauchi, Y. Green Synthesis of Metal Oxide Nanostructures Using Naturally Occurring Compounds for Energy, Environmental, and bio-Related Applications. New J. Chem. 2019, 43 (40), 15846–15856.
- Restrepo, C.V.; Villa, C.C. Synthesis of Silver Nanoparticles, Influence of Capping Agents, and Dependence on Size and Shape: A Review. Environ. Nanotechnol. Monit. Manag. 2021, 15, 100428.
- Gebre, S.H. Bio-inspired Synthesis of Metal and Metal Oxide Nanoparticles: The key Role of Phytochemicals. J. Cluster Sci. 2023, 34 (2), 665–704.
- Preety, R.; Anitha, R.; Rajeshkumar, S.; Lakshmi, T. Anti-diabetic Activity of Silver Nanoparticles Prepared from Cumin Oil Using Alpha Amylase Inhibitory Assay. 2020.
- Oladipo, I.; Lateef, A; Azeez, M.A.; Asafa, T.B.; Yakeen, T.A.; Ogunsona, S.B.; Irshad, H.M.; Abbas S.H. Antidiabetic Properties of Phytosynthesized Gold Nanoparticles (AuNPs) from Datura Stramonium Seed. in IOP Conference Series: Materials Science and Engineering. 2020. IOP Publishing.
- Abdel-Halim, A.H., Fyiad, A.A.A.; Aboulthana, W.M.; El-Sammad, N.M.; Youssef, A.M., Ali, M.M. Assessment of the Anti-Diabetic Effect of Bauhinia variegata Gold Nano-Extract Against Streptozotocin Induced Diabetes Mellitus in Rats. J. Appl. Pharm. Sci. 2020, 10 (05), 077–091.
- Vijayakumar, S.; Vinayagam, R.; Anand, M.A.V.; Venkatachalam, K.; Saravanakumar, K.; Wang, M.-H.; Gothandam, K.M.; David, E. Green Synthesis of Gold Nanoparticle Using Eclipta alba and its Antidiabetic Activities Through Regulation of Bcl-2 Expression in Pancreatic Cell Line. J. Drug. Deliv. Sci. Technol. 2020, 58, 101786.
- Badeggi, U.M.; Ismail, E.; Adeloye, A.; Botha, S.; Badmus, J.; Marnewick, J.; Cupido, C.; Hussein, A. Green Synthesis of Gold Nanoparticles Capped with Procyanidins from Leucosidea sericea as Potential Antidiabetic and Antioxidant Agents. Biomolecules 2020, 10 (3), 452.
- Mukundan, D.; Mohankumar, R.; Vasanthakumari, R. Comparative Study of Synthesized Silver and Gold Nanoparticles Using Leaves Extract of Bauhinia tomentosa Linn and Their Anticancer Efficacy. Bull. Mater. Sci. 2017, 40 (2), 335–344.
- Gupta, S.; Hemlata, H.; Tejavath, K. Synthesis, Characterization and Comparative Anticancer Potential of Phytosynthesized Mono and Bimetallic Nanoparticles Using Moringa oleifera Aqueous Leaf Extract. Beilstein Arch 2020, 1, 95.
- Sun, B.; Hu, N.; Han, L.; Pi, Y.; Gao, Y.; Chen, K. Anticancer Activity of Green Synthesised Gold Nanoparticles from Marsdenia tenacissima Inhibits A549 Cell Proliferation Through the Apoptotic Pathway. Artif. Cells. Nanomed. Biotechnol. 2019, 47 (1), 4012–4019.
- Mollick, M.M.R.; Bhowmick, B.; Mondal, D.; Maity, D.; Rana, D.; Dash, S.K.; Chattopadhyay, S.; Roy, S.; Sarkar, J.; Acharya, K.; Chakraborty, M.; Chattopadhyay, D. Anticancer (in Vitro) and Antimicrobial Effect of Gold Nanoparticles Synthesized Using Abelmoschus esculentus (L.) Pulp Extract via a Green Route. RSC Adv. 2014, 4 (71), 37838–37848.
- Kajani, A.A.; Bordbar, A.-K.; Zarkesh Esfahani, S.H.; Razmjou, A. Gold Nanoparticles as Potent Anticancer Agent: Green Synthesis, Characterization, and in Vitro Study. RSC Adv. 2016, 6 (68), 63973–63983.
- Geetha, R.; Ashokkumar, T.; Tamilselvan, S.; Govindaraju, K.; Sadiq, M.; Singaravelu, G. Green Synthesis of Gold Nanoparticles and Their Anticancer Activity. Cancer. Nanotechnol. 2013, 4 (4), 91–98.
- Wang, C.; Wang, C.; Mathiyalagan, R.; Aceituno, V.C.; Singh, P.; Ahn, S.; Wang, D.; Yang, D.C. Rapid Green Synthesis of Silver and Gold Nanoparticles Using Dendropanax morbifera Leaf Extract and Their Anticancer Activities. Int. J. Nanomed. 2016, 11, 3691–3701.
- Zhang, P.; Wang, P.; Yan, L.; Liu, L. Synthesis of Gold Nanoparticles with Solanum xanthocarpum Extract and Their in Vitro Anticancer Potential on Nasopharyngeal Carcinoma Cells. Int. J. Nanomed. 2018, 13, 7047.
- Tabassam, Q.; Mehmood, T.; Raza, A.R.; Ullah, A.; Saeed, F.; Anjum, F.M. Synthesis, Characterization and Anti-Cancer Therapeutic Potential of Withanolide-A with 20 nm sAuNPs Conjugates Against SKBR3 Breast Cancer Cell Line. Int. J. Nanomed. 2020, 15, 6649.
- Vijayan, R.; Joseph, S.; Mathew, B. Indigofera tinctoria Leaf Extract Mediated Green Synthesis of Silver and Gold Nanoparticles and Assessment of Their Anticancer, Antimicrobial, Antioxidant and Catalytic Properties. Artif. Cells. Nanomed. Biotechnol. 2018, 46 (4), 861–871.
- Vimalraj, S.; Ashokkumar, T.; Saravanan, S. Biogenic Gold Nanoparticles Synthesis Mediated by Mangifera indica Seed Aqueous Extracts Exhibits Antibacterial, Anticancer and Anti-Angiogenic Properties. Biomed. Pharmacother. 2018, 105, 440–448.
- Laksee, S.; Puthong, S.; Kongkavitoon, P.; Palaga, T.; Muangsin, N. Facile and Green Synthesis of Pullulan Derivative-Stabilized Au Nanoparticles as Drug Carriers for Enhancing Anticancer Activity. Carbohydr. Polym. 2018, 198, 495–508.
- Elemike, E.E.; Onwudiwe, D.C.; Nundkumar, N.; Singh, M. CuO and Au-CuO Nanoparticles Mediated by Stigmaphyllon ovatum Leaf Extract and Their Anticancer Potential. Inorg. Chem. Commun. 2019, 104, 93–97.
- Hoshyar, R.; Khayati, G.R.; Poorgholami, M.; Kaykhaii, M. A Novel Green one-Step Synthesis of Gold Nanoparticles Using Crocin and Their Anti-Cancer Activities. J. Photochem. Photobiol., B 2016, 159, 237–242.
- Valsalam, S.; Agastian, P.; Esmail, G.A.; Ghilan, A.-K.M.; Al-Dhabi, N.A.; Arasu, M.V. Biosynthesis of Silver and Gold Nanoparticles Using Musa Acuminata colla Flower and its Pharmaceutical Activity Against Bacteria and Anticancer Efficacy. J. Photochem. Photobiol., B 2019, 201, 111670.
- Vijayakumar, S. Eco-friendly Synthesis of Gold Nanoparticles Using Fruit Extracts and in Vitro Anticancer Studies. J. Saudi Chem. Soc. 2019, 23 (6), 753–761.
- Balasubramani, G.; Ramkumar, R.; Krishnaveni, N.; Pazhanimuthu, A.; Natarajan, T.; Sowmiya, R.; Perumal, P. Structural Characterization, Antioxidant and Anticancer Properties of Gold Nanoparticles Synthesized from Leaf Extract (Decoction) of Antigonon leptopus Hook. & Arn. J. Trace Elem. Med. Biol. 2015, 30, 83–89.
- Elemike, E.E.; Onwudiwe, D.C.; Nundkumar, N.; Singh, M.; Iyekowa, O. Green Synthesis of Ag, Au and Ag-Au Bimetallic Nanoparticles Using Stigmaphyllon ovatum Leaf Extract and Their in Vitro Anticancer Potential. Mater. Lett. 2019, 243, 148–152.
- Kumar, B.; Smita, K.; Cumbal, L.; Camacho, J.; Hernández-Gallegos, E.; de Guadalupe Chávez-López, M.; Grijalva, M.; Andrade, K. One pot Phytosynthesis of Gold Nanoparticles Using Genipa americana Fruit Extract and its Biological Applications. Mater. Sci. Eng: C 2016, 62, 725–731.
- Vijayakumar, R.; Devi, V.; Adavallan, K.; Saranya, D. Green Synthesis and Characterization of Gold Nanoparticles Using Extract of Anti-Tumor Potent Crocus sativus. Physica E 2011, 44 (3), 665–671.
- Dubey, S.P.; Lahtinen, M.; Sillanpää, M. Tansy Fruit Mediated Greener Synthesis of Silver and Gold Nanoparticles. Process Biochem. 2010, 45 (7), 1065–1071.
- Muthukumar, T.; Sudhakumari; Sambandam, B.; Aravinthan, A.; Sastry, T.P.; Kim, J.-H. Green Synthesis of Gold Nanoparticles and Their Enhanced Synergistic Antitumor Activity Using HepG2 and MCF7 Cells and its Antibacterial Effects. Process Biochem. 2016, 51 (3), 384–391.
- Sunderam, V.; Thiyagarajan, D.; Lawrence, A.V.; Mohammed, S.S.S.; Selvaraj, A. In-vitro Antimicrobial and Anticancer Properties of Green Synthesized Gold Nanoparticles Using Anacardium Occidentale Leaves Extract. Saudi J. Biol. Sci. 2019, 26 (3), 455–459.
- Dorosti, N.; Jamshidi, F. Plant-mediated Gold Nanoparticles by Dracocephalum kotschyi as Anticholinesterase Agent: Synthesis, Characterization, and Evaluation of Anticancer and Antibacterial Activity. J. Appl. Biomed. 2016, 14 (3), 235–245.
- Kyzioł, A.; Łukasiewicz, S.; Sebastian, V.; Kuśtrowski, P.; Kozieł, M.; Majda, D.; Cierniak, A. Towards Plant-Mediated Chemistry–Au Nanoparticles Obtained Using Aqueous Extract of Rosa damascena and Their Biological Activity in Vitro. J. Inorg. Biochem. 2021, 214, 111300.
- Singh, H.; Du, J.; Singh, P.; Yi, T.H. Ecofriendly Synthesis of Silver and Gold Nanoparticles by Euphrasia officinalis Leaf Extract and its Biomedical Applications. Artif. Cells. Nanomed. Biotechnol. 2018, 46 (6), 1163–1170.
- Barai, A.C.; Paul, K.; Dey, A.; Manna, S.; Roy, S.; Bag, B.G.; Mukhopadhyay, C. Green Synthesis of Nerium oleander-Conjugated Gold Nanoparticles and Study of its in Vitro Anticancer Activity on MCF-7 Cell Lines and Catalytic Activity. Nano Convergence 2018, 5 (1), 1–9.
- Ayyoub, S.; Al-Trad, B.; Aljabali, A.A.A.; Alshaer, W.; Al Zoubi, M.; Omari, S.; Fayyad, D.; Tambuwala, M.M. Biosynthesis of Gold Nanoparticles Using Leaf Extract of Dittrichia viscosa and in Vivo Assessment of its Anti-Diabetic Efficacy. Drug. Deliv. Transl. Res. 2022, 12 (12), 2993–2999.
- Guo, Y.; Jiang, N.; Zhang, L.; Yin, M. Green Synthesis of Gold Nanoparticles from Fritillaria cirrhosa and its Anti-Diabetic Activity on Streptozotocin Induced Rats. Arab. J. Chem 2020, 13 (4), 5096–5106.
- Mahmoudi, F.; Mahmoudi, F.; Gollo, K.H.; Amini, M. M. Novel Gold Nanoparticles: Green Synthesis with Eryngium thyrsoideum Boiss Extract, Characterization, and in Vivo Investigations on Inflammatory Gene Expression and Biochemical Parameters in Type 2 Diabetic Rats. Biol. Trace Elem. Res. 2021, 1–10.
- Oves, M.; Aslam, M.; Rauf, M.A.; Qayyum, S.; Qari, H.A.; Khan, M.S.; Alam, M.Z.; Tabrez, S.; Pugazhendhi, A.; Ismail, I.M.I. Antimicrobial and Anticancer Activities of Silver Nanoparticles Synthesized from the Root Hair Extract of Phoenix Dactylifera. Mater. Sci. Eng: C 2018, 89, 429–443.
- Hosny, M.; Eltaweil, A.S.; Mostafa, M.; El-Badry, Y.A.; Hussein, E.E.; Omer, A.M.; Fawzy, M. Facile Synthesis of Gold Nanoparticles for Anticancer, Antioxidant Applications, and Photocatalytic Degradation of Toxic Organic Pollutants. ACS Omega 2022, 7 (3), 3121–3133.
- Narchin, F.; Larijani, K.; Rustaiyan, A.; Nejad Ebrahimi, S.; Tafvizi, F. Phytochemical Synthesis of Silver Nanoparticles by two Techniques Using Saturaja Rechengri Jamzad Extract: Identifying and Comparing in Vitro Anti-Proliferative Activities. Adv. Pharm. Bull. 2018, 8 (2), 235.
- Jini, D.; Sharmila, S. Green Synthesis of Silver Nanoparticles from Allium Cepa and its in Vitro Antidiabetic Activity. Mater. Today: Proc. 2020, 22, 432–438.
- Bhuvaneswari, R.; Xavier, R.J.; Arumugam, M. Facile Synthesis of Multifunctional Silver Nanoparticles Using Mangrove Plant Excoecaria agallocha L. for its Antibacterial, Antioxidant and Cytotoxic Effects. J. Parasit. Dis. 2017, 41 (1), 180–187.
- Suresh, U.; Murugan, K.; Benelli, G.; Nicoletti, M.; Barnard, D.R.; Panneerselvam, C.; Kumar, P.M.; Subramaniam, J.; Dinesh, D.; Chandramohan, B. Tackling the Growing Threat of Dengue: Phyllanthus Niruri-Mediated Synthesis of Silver Nanoparticles and Their Mosquitocidal Properties Against the Dengue Vector Aedes Aegypti (Diptera: Culicidae). Parasitol. Res. 2015, 114, 1551–1562.
- Balan, K.; Qing, W.; Wang, Y.; Liu, X.; Palvannan, T.; Wang, Y.; Ma, F.; Zhang, Y. Antidiabetic Activity of Silver Nanoparticles from Green Synthesis Using Lonicera japonica Leaf Extract. RSC Adv. 2016, 6 (46), 40162–40168.
- Sasidharan, J.; Meenakshi, R.; Sureshkumar, P. Green Synthesis, Characterization and Evaluation of in-Vitro Antioxidant & Anti-Diabetic Activity of Nanoparticles from a Polyherbal Formulation-Mehani. J. Environ. Nanotechnol 2018, 7 (3), 51–59.
- Dhas, T.S.; Kumar, V.G.; Karthick, V.; Vasanth, K.; Singaravelu, G.; Govindaraju, K. Effect of Biosynthesized Gold Nanoparticles by Sargassum swartzii in Alloxan Induced Diabetic Rats. Enzyme Microb. Technol. 2016, 95, 100–106.
- Mohammed, S.S.S.; Lawrance, A.V.; Sampath, S.; Sunderam, V.; Madhavan, Y. Facile Green Synthesis of Silver Nanoparticles from Sprouted Zingiberaceae Species: Spectral Characterisation and its Potential Biological Applications. Mater. Technol. 2022, 37 (8), 533–546.
- Bagyalakshmi, J.; Haritha, H. Green Synthesis and Characterization of Silver Nanoparticles Using Pterocarpus marsupium and Assessment of its in Vitro Antidiabetic Activity. American Journal of Advanced Drug Delivery ISSN 2017.
- Kong, Y.; Paray, B.A.; Al-Sadoon, M.K.; Fahad Albeshr, M. Novel Green Synthesis, Chemical Characterization, Toxicity, Colorectal Carcinoma, Antioxidant, Anti-Diabetic, and Anticholinergic Properties of Silver Nanoparticles: A Chemopharmacological Study. Arab. J. Chem 2021, 14 (6), 103193.
- Ponnanikajamideen, M.; Rajeshkumar, S.; Vanaja, M.; Annadurai, G. In Vivo Type 2 Diabetes and Wound-Healing Effects of Antioxidant Gold Nanoparticles Synthesized Using the Insulin Plant Chamaecostus cuspidatus in Albino Rats. Can. J. Diabetes. 2019, 43 (2), 82–89. e6.
- Kumar, V.; Singh, S.; Srivastava, B.; Bhadouria, R.; Singh, R. Green Synthesis of Silver Nanoparticles Using Leaf Extract of Holoptelea integrifolia and Preliminary Investigation of its Antioxidant, Anti-Inflammatory, Antidiabetic and Antibacterial Activities. J. Environ. Chem. Eng. 2019, 7 (3), 103094.
- Nagaraja, S.; Ahmed, S.S.; Bharathi, D.R.; Goudanavar, P.; Rupesh Kumar, M.; Fattepur, S.; Meravanige, G.; Shariff, A.; Shiroorkar, P.N.; Habeebuddin, M.; Telsang, M. Green Synthesis and Characterization of Silver Nanoparticles of Psidium guajava Leaf Extract and Evaluation for Its Antidiabetic Activity. Molecules 2022, 27 (14), 4336.
- Ul Haq, M.N.; Shah, G.M.; Gul, A.; Foudah, A.I.; Alqarni, M.H.; Yusufoglu, H.S.; Hussain, M.; Alkreathy, H.M.; Ullah, I.; Khan, A.M.; Jamil, S.; Ahmed, M.; Khan, R.A. Biogenic Synthesis of Silver Nanoparticles Using Phagnalon niveum and Its In Vivo Anti-Diabetic Effect Against Alloxan-Induced Diabetic Wistar Rats. Nanomaterials 2022, 12 (5), 830.
- Virk, P. Antidiabetic Activity of Green Gold-Silver Nanocomposite with Trigonella foenum graecum L. Seeds Extract on Streptozotocin-Induced Diabetic Rats. Pak. J. Zool. 2018, 50 (2.
- Saratale, R.G.; Saratale, G.D.; Ahn, S.; Shin, H.-S. Grape Pomace Extracted Tannin for Green Synthesis of Silver Nanoparticles: Assessment of Their Antidiabetic, Antioxidant Potential and Antimicrobial Activity. Polymers 2021, 13 (24), 4355.
- Das, S.K.; Behera, S.; Patra, J.K.; Thatoi, H. Green Synthesis of Sliver Nanoparticles Using Avicennia officinalis and Xylocarpus granatum Extracts and in Vitro Evaluation of Antioxidant, Antidiabetic and Anti-Inflammatory Activities. J. Cluster Sci. 2019, 30 (4), 1103–1113.
- Omolaja, A.A.; Pearce, B.; Omoruyi, S.I.; Badmus, J.A.; Ismail, E.; Marnewick, J.; Botha, S.; Benjeddou, M.; Ekpo, O.E.; Hussein, A.A. The Potential of Chalcone-Capped Gold Nanoparticles for the Management of Diabetes Mellitus. Surf. Interfaces 2021, 25, 101251.
- Yarrappagaari, S.; Gutha, R.; Narayanaswamy, L.; Thopireddy, L.; Benne, L.; Mohiyuddin, S.S.; Vijayakumar, V.; Saddala, R.R. Eco-Friendly Synthesis of Silver Nanoparticles from the Whole Plant of Cleome viscosa and Evaluation of Their Characterization, Antibacterial, Antioxidant and Antidiabetic Properties. Saudi J. Biol. Sci. 2020, 27 (12), 3601–3614.
- babu Maddinedi, S.; Mandal, B.K.; Maddili, S.K. Biofabrication of Size Controllable Silver Nanoparticles–a Green Approach. J. Photochem. Photobiol., B 2017, 167, 236–241.
- Mahmoudi, F.; Mahmoudi, F.; Gollo, K.H.; Amini, M.M. Biosynthesis of Novel Silver Nanoparticles Using Eryngium thyrsoideum Boiss Extract and Comparison of Their Antidiabetic Activity with Chemical Synthesized Silver Nanoparticles in Diabetic Rats. Biol. Trace Elem. Res. 2021, 199 (5), 1967–1978.
- Govindappa, M.; Hemashekhar, B.; Arthikala, M.-K.; Ravishankar Rai, V.; Ramachandra, Y.L. Characterization, Antibacterial, Antioxidant, Antidiabetic, Anti-Inflammatory and Antityrosinase Activity of Green Synthesized Silver Nanoparticles Using Calophyllum tomentosum Leaves Extract. Results Phys. 2018, 9, 400–408.
- Majeed, S., et al. In Vitro Evaluation of Antibacterial, Antioxidant, and Antidiabetic Activities and Glucose Uptake Through 2-NBDG by Hep-2 Liver Cancer Cells Treated with Green Synthesized Silver Nanoparticles. Oxid. Med. Cell. Longevity 2022, 2022.
- Ansari, S.; Bari, A.; Ullah, R.; Mathanmohun, M.; Veeraraghavan, V.P.; Sun, Z. Gold Nanoparticles Synthesized with Smilax glabra Rhizome Modulates the Anti-Obesity Parameters in High-fat Diet and Streptozotocin Induced Obese Diabetes rat Model. J. Photochem. Photobiol., B 2019, 201, 111643.
- Abd El-Moaty, H.I.; Soliman, N.A.; Hamad, R.S.; Ismail, E.H.; Sabry, D.Y.; Khalil, M.M.H. Comparative Therapeutic Effects of Pituranthos tortuosus Aqueous Extract and Phyto-Synthesized Gold Nanoparticles on Helicobacter Pylori, Diabetic and Cancer Proliferation. S. Afr. J. Bot. 2021, 139, 167–174.
- Senthilkumar, P., et al. Potent α-Glucosidase Inhibitory Activity of Green Synthesized Gold Nanoparticles from the Brown Seaweed Padina boergesenii. Int. J. Adv. Multidiscip. Res 2015, 2 (11), 0917–0923.
- Malapermal, V.; Botha, I.; Krishna, S.B.N.; Mbatha, J.N. Enhancing Antidiabetic and Antimicrobial Performance of Ocimum basilicum, and Ocimum sanctum (L.) Using Silver Nanoparticles. Saudi J. Biol. Sci. 2017, 24 (6), 1294–1305.
- Ghasemian Lemraski, E.; Valadbeigi, T. Evaluation of in Vitro Antimicrobial, Antidiabetic and Antioxidant Potential of Alyssum Homalocarpum and Green Synthesis of the Silver Nanoparticles. J. Med. Plants By-prod 2018, 7 (1), 1–8.
- Vijayashree, I.; Niranjana, P.; Prabhu, G.; Sureshbabu, V.V.; Manjanna, J. Conjugation of Au Nanoparticles with Chlorambucil for Improved Anticancer Activity. J. Cluster Sci. 2017, 28 (1), 133–148.
- Zhang, Y.; Liu, B.; Wu, H.; Li, B.; Xu, J.; Duan, L.; Jiang, C.; Zhao, X.; Yuan, Y.; Zhang, G.; Zeng, X. Anti-tumor Activity of Verbascoside Loaded Gold Nanoparticles. J. Biomed. Nanotechnol. 2014, 10 (12), 3638–3646.
- Rabha, B.; Bharadwaj, K.K.; Baishya, D.; Sarkar, T.; Edinur, H.A.; Pati, S. Synthesis and Characterization of Diosgenin Encapsulated Poly-ϵ-Caprolactone-Pluronic Nanoparticles and its Effect on Brain Cancer Cells. Polymers 2021, 13 (8), 1322.
- Gomathi, A.; Xavier Rajarathinam, S.R.; Mohammed Sadiq, A.; Rajeshkumar, S. Anticancer Activity of Silver Nanoparticles Synthesized Using Aqueous Fruit Shell Extract of Tamarindus indica on MCF-7 Human Breast Cancer Cell Line. J. Drug. Deliv. Sci. Technol. 2020, 55, 101376.
- Abdoli, M.; Arkan, E.; Shekarbeygi, Z.; Khaledian, S. Green Synthesis of Gold Nanoparticles Using Centaurea behen Leaf Aqueous Extract and Investigating Their Antioxidant and Cytotoxic Effects on Acute Leukemia Cancer Cell Line (THP-1). Inorg. Chem. Commun. 2021, 129, 108649.
- Kaplan, Ö; Gökşen Tosun, N.; Özgür, A.; Erden Tayhan, S.; Bilgin, S.; Türkekul, İ.; Gökce, İ. Microwave-assisted Green Synthesis of Silver Nanoparticles Using Crude Extracts of Boletus edulis and Coriolus Versicolor: Characterization, Anticancer, Antimicrobial and Wound Healing Activities. J. Drug. Deliv. Sci. Technol. 2021, 64, 102641.
- Al-Radadi, N.S. Green Biosynthesis of Flaxseed Gold Nanoparticles (Au-NPs) as Potent Anti-Cancer Agent Against Breast Cancer Cells. J. Saudi Chem. Soc. 2021, 25 (6), 101243.
- Hashemi, Z.; Mohammadyan, M.; Naderi, S.; Fakhar, M.; Biparva, P.; Akhtari, J.; Ebrahimzadeh, M.A. Green Synthesis of Silver Nanoparticles Using Ferula persica Extract (Fp-NPs): Characterization, Antibacterial, Antileishmanial, and in Vitro Anticancer Activities. Mater. Today Commun 2021, 27, 102264.
- Pallavi, S.; Rudayni, H.A.; Bepari, A.; Niazi, S.K.; Nayaka, S. Green Synthesis of Silver Nanoparticles Using Streptomyces hirsutus Strain SNPGA-8 and Their Characterization, Antimicrobial Activity, and Anticancer Activity Against Human Lung Carcinoma Cell Line A549. Saudi J. Biol. Sci. 2022, 29 (1), 228–238.
- Wang, L.; Xu, J.; Yan, Y.; Liu, H.; Karunakaran, T.; Li, F. Green Synthesis of Gold Nanoparticles from Scutellaria barbata and its Anticancer Activity in Pancreatic Cancer Cell (PANC-1). Artif. Cells. Nanomed. Biotechnol. 2019, 47 (1), 1617–1627.
- Zhang, X.; Tan, Z.; Jia, K.; Zhang, W.; Dang, M. Rabdosia rubescens Linn: Green Synthesis of Gold Nanoparticles and Their Anticancer Effects Against Human Lung Cancer Cells A549. Artif. Cells. Nanomed. Biotechnol. 2019, 47 (1), 2171–2178.
- Sibuyi, N.R.S., Thipe, V.C., Panjtan-Amiri, K., Meyer, M., Katti, K. V. Green Synthesis of Gold Nanoparticles Using Acai Berry and Elderberry Extracts and Investigation of Their Effect on Prostate and Pancreatic Cancer Cells. Nanobiomedicine. (Rij) 2021, 8, 1849543521995310.
- Rajeshkumar, S.; Lakshmi, T.; Tharani, M.; Sivaperumal, P. Green Synthesis of Gold Nanoparticles Using Pomegranate Peel Extract and its Antioxidant and Anticancer Activity Against Liver Cancer Cell Line. Alinteri J. Agric. Sci 2020, 35 (2), 164–169.
- Reyes-Becerril, M.; Ruvalcaba, F.; Sanchez, V.; López, M.G.; Silva-Jara, J.; Hernandez-Adame, L.; Angulo, C. Green Synthesis of Gold Nanoparticles Using Turnera diffusa Willd Enhanced Antimicrobial Properties and Immune Response in Longfin Yellowtail Leukocytes. Aquac. Res. 2021, 52 (7), 3391–3402.
- El-Borady, O.M.; Ayat, M.S.; Shabrawy, M.A.; Millet, P. Green Synthesis of Gold Nanoparticles Using Parsley Leaves Extract and Their Applications as an Alternative Catalytic, Antioxidant, Anticancer, and Antibacterial Agents. Adv. Powder Technol. 2020, 31 (10), 4390–4400.
- Mortazavi-Derazkola, S.; Ebrahimzadeh, M.A.; Amiri, O.; Goli, H.R.; Rafiei, A.; Kardan, M.; Salavati-Niasari, M. Facile Green Synthesis and Characterization of Crataegus microphylla Extract-Capped Silver Nanoparticles (CME@ Ag-NPs) and its Potential Antibacterial and Anticancer Activities Against AGS and MCF-7 Human Cancer Cells. J. Alloys Compd. 2020, 820, 153186.
- Chokkalingam, M.; Singh, P.; Huo, Y.; Soshnikova, V.; Ahn, S.; Kang, J.; Mathiyalagan, R.; Kim, Y.J.; Yang, D.C. Facile Synthesis of Au and Ag Nanoparticles Using Fruit Extract of Lycium chinense and Their Anticancer Activity. J. Drug. Deliv. Sci. Technol. 2019, 49, 308–315.
- Balasubramanian, S.; Kala, S.M.J.; Pushparaj, T.L. Biogenic Synthesis of Gold Nanoparticles Using Jasminum auriculatum Leaf Extract and Their Catalytic, Antimicrobial and Anticancer Activities. J. Drug. Deliv. Sci. Technol. 2020, 57, 101620.
- Hashemi, S.F.; Tasharrofi, N.; Saber, M.M. Green Synthesis of Silver Nanoparticles Using Teucrium polium Leaf Extract and Assessment of Their Antitumor Effects Against MNK45 Human Gastric Cancer Cell Line. J. Mol. Struct. 2020, 1208, 127889.
- Vallinayagam, S.; Rajendran, K.; Sekar, V. Green Synthesis and Characterization of Silver Nanoparticles Using Naringi crenulate Leaf Extract: Key Challenges for Anticancer Activities. J. Mol. Struct. 2021, 1243, 130829.
- Satpathy, S.; Patra, A.; Ahirwar, B.; Hussain, M.D. Process Optimization for Green Synthesis of Gold Nanoparticles Mediated by Extract of Hygrophila spinosa T. Anders and Their Biological Applications. Physica E 2020, 121, 113830.
- Alghuthaymi, M.A., et al. Green Synthesis of Gold Nanoparticles Using Polianthes tuberosa L. Floral Extract. Plants 2021, 10 (11), 2370.
- Anandan, M.; Poorani, G.; Boomi, P.; Varunkumar, K.; Anand, K.; Chuturgoon, A.A.; Saravanan, M.; Gurumallesh Prabu, H. Green Synthesis of Anisotropic Silver Nanoparticles from the Aqueous Leaf Extract of Dodonaea viscosa with Their Antibacterial and Anticancer Activities. Process Biochem. 2019, 80, 80–88.
- Vera-Nuñez, L.D.C.; Cornejo-Ruiz, J.O.; Arenas-Chávez, C.A.; de Hollanda, L.M.; Alvarez-Risco, A.; Del-Aguila-Arcentales, S.; Davies, N.M.; Yáñez, J.A.; Vera-Gonzales, C. Green Synthesis of a Novel Silver Nanoparticle Conjugated with Thelypteris glandulosolanosa (Raqui-Raqui): Preliminary Characterization and Anticancer Activity. Processes 2022, 10 (7), 1308.
- Hosny, M.; Fawzy, M.; Abdelfatah, A.M.; Fawzy, E.E.; Eltaweil, A.S. Comparative Study on the Potentialities of two Halophytic Species in the Green Synthesis of Gold Nanoparticles and Their Anticancer, Antioxidant and Catalytic Efficiencies. Adv. Powder Technol. 2021, 32 (9), 3220–3233.
- Austin, L.A.; Kang, B.; Yen, C.-W.; El-Sayed, M.A. Plasmonic Imaging of Human Oral Cancer Cell Communities During Programmed Cell Death by Nuclear-Targeting Silver Nanoparticles. J. Am. Chem. Soc. 2011, 133 (44), 17594–17597.
- Gurunathan, S.; Park, J.H.; Han, J.W.; Kim, J.-H. Comparative Assessment of the Apoptotic Potential of Silver Nanoparticles Synthesized by Bacillus tequilensis and Calocybe indica in MDA-MB-231 Human Breast Cancer Cells: Targeting p53 for Anticancer Therapy. Int. J. Nanomed. 2015, 4203–4223.
- Ullah, I., et al. Green-synthesized Silver Nanoparticles Induced Apoptotic Cell Death in MCF-7 Breast Cancer Cells by Generating Reactive Oxygen Species and Activating Caspase 3 and 9 Enzyme Activities. Oxid. Med. Cell. Longevity 2020, 2020.
- Akter, M.; Sikder, M.T.; Rahman, M.M.; Ullah, A.K.M.A.; Hossain, K.F.B.; Banik, S.; Hosokawa, T.; Saito, T.; Kurasaki, M. A Systematic Review on Silver Nanoparticles-Induced Cytotoxicity: Physicochemical Properties and Perspectives. J. Adv. Res. 2018, 9, 1–16.
- Jin, J.; Ovais, M.; Chen, C. Stimulus-responsive Gold Nanotheranostic Platforms for Targeting the Tumor Microenvironment. Nano. Today. 2018, 22, 83–99.
- Alavi, M.; Kowalski, R.; Capasso, R.; Douglas Melo Coutinho, H.; Rose Alencar De Menezes, I. Various Novel Strategies for Functionalization of Gold and Silver Nanoparticles to Hinder Drug-Resistant Bacteria and Cancer Cells. Micro. Nano. Bio. Aspects 2022, 1 (1), 38–48.