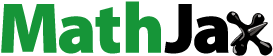
Abstract
The study aimed to compare the effect of individual or combined supplementation of condensed tannins (Tamarindus indica) and saponins-containing (Sapindus mukorossi) phyto-sources on enteric methane emission in crossbred cattle. Twenty-four animals were divided into four groups of six each and fed on a finger millet straw and concentrate (70:30) based diet as follows: C (no tannins/saponins), T (tamarind seed husk supplemented group), S (soapnut supplemented group) and TS (tamarind seed husk and soapnut combined supplementation in 60:40). Individual (T, S) or combination (TS) of tamarind seed husk and soapnut constituted 51 g/kg of the basal diet. Present in vivo study revealed a significant reduction (∼20%) in enteric methane emission (g/d) with tamarind seed husk (T) or combined supplementation of tamarind seed husk and soapnut (TS). Furthermore, the comparison of methane emission on uniform basis (g/kg dNDF) also revealed a significant decrease with the individual supplementation of tamarind seed husk or combined supplementation. Therefore, tamarind seed husk alone or in combination with soapnut at above level can be used to formulate low methane emission diet. However, the efficacy of such diet in reducing enteric methane emission should be confirmed by conducting long duration trials in ruminants.
Introduction
Methanogenesis is an integral part of the fermentation processes in the rumen. Methane (CH4) emission through rumen fermentation contributes to global warming. Additionally, rumen methanogenesis accounts for a loss of biological energy (6–12%). The loss of each litre of CH4 as a result of methanogenic digestion represents a reduction of 39.5 kJ feed energy [Citation1]. Therefore, extensive efforts are being made globally to develop effective CH4 mitigating interventions that would be acceptable to the livestock farmers [Citation2]. A feasible approach for reducing enteric CH4 emission could the use of plant secondary metabolites because they possess anti-methanogenic properties [Citation3].
Tannins are the most explored plant secondary metabolites as a modulator of the digestion processes in ruminants. Condensed tannins (CT) have been used widely for altering the protein flow from the rumen to lower digestive tract [Citation4,Citation5]. However, an adverse impact of tannins on feed intake and degradation is evident in many studies. The anti-methanogenic properties of tamarind (Tamarindus indica) seed husk, a tanniniferous agricultural waste have recently been explored both in vitro and in vivo [Citation6]. Similarly, saponins as such or in the form of phyto-sources have been explored as a possible intervention for reducing enteric CH4 emission [Citation7–9]. Soapnut (Sapindus mukorossi) is a valuable medicinal plant that is mostly found in the tropical and sub-tropical countries. Soapnut fruits are known for their high saponin content (∼10%). Although, saponins from different sources have been assessed previously for their possible inhibitory effect on methanogenesis process, the results are inconclusive [Citation10]. A significant reduction in CH4 production (in vitro) is reported, when various extracts of soapnut have been used [Citation11,Citation12]. In contrast, McSweeney and Ramirez-Restrepo [Citation13] have found no significant impact of tea seed saponin supplementation on CH4 emissions in Belmont Red Composite steers. Tannins primarily reduce CH4 emissions through a direct inhibition of rumen methanogens [Citation14]. On the other hand, saponins inhibit CH4 emissions through an indirect mechanism of reducing protozoa that are associated with methanogens [Citation15,Citation16]. Currently, limited information is available in the public domain whether combined supplementation of phyto-sources possessing two different biomolecules (tannins and saponins) has any synergistic impact on enteric CH4 emission. It was hypothesized that the combined supplementation of tamarind seed husk (tannins) and spaonut fruit pulp (saponins) would have a synergistic negative impact on the rumen methanogenesis process due to their different mechanism of action.
Material and methods
Experimental animals and management
The study was conducted on 24 male adult crossbred (HF × Hallikar) cattle (331 ± 25.7 kg body weight, 5–6 years old). The experimental animals were maintained at the Experimental Livestock Unit of ICAR-National Institute of Animal Nutrition and Physiology (ICAR-NIANP), Bangalore (12.97°N, 77.56°E), India. The use of the experimental animals conformed to the guidelines of the Institutional Animal Ethics Committee of ICAR-NIANP (Approval No. 25/14/2017-CPSCEA).
The animals were divided into four groups (N = 6 in each group) in a completely randomized design and were fed basal diet comprising finger millet (Eleusine coracana) straw (70%) and concentrates (30%) according to the ICAR standards [Citation17]. No tanniniferous (tamarind seed husk) or saponiferous (soapnut fruit pulp) phyto-sources were added in the basal diet of the control group (C). In contrast, in the experimental groups tanniniferous (T), saponiferous (S) or combination of the both (TS; tanniniferous: saponiferous 60: 40) phyto-sources were added in the basal diet. The phyto-sources following drying and grinding were added into concentrates (170 g/kg of the concentrates or 51 g/kg of the total diet) by partially replacing wheat bran (). The dosage (5.1%) for the supplementation in this study was decided on the basis of the previous in vitro and in vivo studies carried out by our group [Citation6]. The details of feed components, proportion of individual ingredient in concentrates and chemical composition of the diets are presented in .
Table 1a. Proportions (DM basis) of dietary and concentrate ingredients.
Table 1b. Chemical composition of basal diet, finger millet straw and concentrate (DM basis).
The experimental animals were housed individually in the adjacent concrete holding pens in a well ventilated shed and had free access to clean drinking water throughout the experimental period. The animals were fed control or test diets respectively for a period of 30 days and the diets were offered in the morning at 09.00 hrs. Finger millet straws were chopped to 2 mm size and mixed with concentrates as indicated in the . The measurement of CH4 emission was performed during the last nine days of the feeding-period.
Estimation of enteric CH4 emission
Sulfur hexafluoride (SF6) tracer technique of Johnson et al. [Citation18] was used for measuring the in vivo enteric CH4 emission from the experimental animals. Briefly, long brass permeation tubes (32 mm) were filled with SF6 gas (99.9% purity; Chemix specialities gases, Bangalore) and placed at 39 °C for calibration. The tubes were monitored and weighed daily to determine the SF6 release rate and when the release became steady (3.07 to 4.82 mg/d), the tubes were placed into the rumen for measuring the emission. The halters (teflon tube,capilary tube 1/8” OD; Supelco, USA), air filter (15 µm; Swagelok, USA), capillary tube (0.005″ ID, 1/16″ OD, 100 cm long; Supelco, USA) and QC4 quick connects (QC4-B-200 and QC4-D-200; Swagelok, USA) were assembled following the standard guidelines [Citation19] and tied to the animals. The exhaled air (breath) samples were collected for a complete feeding cycle and diluted with N2 gas. The diluted samples from canister were withdrawn using a Hamilton airtight syringe and injected into a gas chromatograph (GC 2010 plus, Shimadzu, Japan) fitted with electron capture detector (ECD) and flame ionization detector (FID) for the analysis of SF6 and CH4, respectively. Daily CH4 emission was calculated by using the formula given below [Citation18].
QCH4 = daily CH4 emission in mole/animal/d; QSF6 = daily release rate of SF6 from the permeation tube; CH4/SF6 = mixing ratio of CH4 and SF6 in collected breath samples.
Digestion trial and chemical analyses
A digestion trial was conducted simultaneously during the period of estimation of enteric CH4 emission, to study the impact of different diets on nutrient intake and digestibility. During the digestion trial, feed intake, residues and faeces were recorded daily. Samples of feed offered, refusals and faeces voided were collected and weighed in the morning daily and brought to the laboratory. One hundredth of the voided faeces was used for dry matter (DM) determination. The DM of feeds, concentrates, refusals and faeces was determined by drying the samples overnight at 80 °C. Dried samples were weighed and pooled for the individual animal. The dried samples were grounded, sieved (1 mm) and preserved for chemical analyses. All the samples were analyzed in triplicate for crude protein (CP) and ash by following the standard procedures of AOAC [Citation20]; while the neutral detergent fiber (NDF) and acid detergent fiber (ADF) were determined using the procedure describe by Van Soest et al. [Citation21]. The fiber fractions (NDF and ADF) were analyzed by using an automatic fiber analyzer (Fibretherm, Gerhardt) and recommended fiber bags (10-0127/177 ST 100). The content of CT in tamarind seed husk was analyzed by using the butanol-HCl-iron method of Makkar [Citation22] and expressed as leucocyanidin equivalent (g/kg DM). The content of saponin in soapnut fruit pulp was determined as described previously [Citation23].
Collection and analyses of rumen fluid
Rumen fluid (60 mL) was collected from each experimental animal using a stomach tube on the last day of CH4 measurement and digestibility trial. The rumen fluid samples were divided equally into three aliquots. The first aliquot was centrifuged and the supernatant was collected and divided into two equal parts that were subjected to the estimation of volatile fatty acid (VFA) fraction and ammonia-nitrogen (NH3-N). VFA fractions were determined using gas chromatograph following the method of Filípek and Dvořák [Citation24]. NH3-N was determined according to the method described previously by Conway [Citation25]. The second aliquot of the fluid was used for protozoa enumeration immediately. Protozoa in rumen fluid samples were enumerated and classified into entodinimorphs and holotrich according to the method described previously by Hungate [Citation26]. The third aliquot of the fluid was stored at −80 °C until used for DNA isolation.
Isolation of DNA and quantitative PCR (qPCR)
Total genomic DNA was isolated from 6 mL of rumen fluid for each animal. The samples were centrifuge at 500 × g for 5 min and the supernatant was collected and subjected to the isolation of genomic DNA according to the method described previously by Yu and Morrison [Citation27]. The relative abundance of total methanogens, protozoa and fibrolytic microbes (Fibrobacter succinogens, Ruminococcus albus and Ruminococcus flavefaciens) in the treatment (T, S and TS) as compared to control (C) groups was determined by qPCR analysis in a Quant Studio® 3 qPCR System (Thermo Fisher Scientific, CA, USA) using SsoFast™ Eva Green® Supermix (Bio-Rad Laboratories Inc, CA, USA). Briefly, 1 ng of total genomic DNA, 0.25 µM of each primer and 1X Eva Green mix were used in a total volume of 10 µL. The qPCR conditions were as follows: initial denaturation at 95 °C for 3 min and 40 cycles of 95 °C for 15 sec and 60 °C for 30 sec. The identity of amplified products was confirmed by agarose gel electrophoresis and melting curve analysis (continuous from 65 to 95 °C; increment 0.15 °C/sec). The details of the primer pairs used in the study are provided in . The relative changes in the abundance of total methanogens, protozoa and fibrolytic microbes were determined using 2−ΔΔCT method and the CT value of total bacteria was used as endogenous reference for the calculation. The qPCR assays were performed in three replicates.
Table 2. Primers employed for q-PCR.
Statistical analyses
Statistical analyses were performed using the SPSS 21 software package [Citation31]. All the data except those obtained from qPCR experiment were analyzed by one-way analysis of variance (ANOVA) with a model that included treatment effect and experimental error. Individual animals were considered as experimental units. Differences between means were compared by Tukey's method and considered significant at p value < 0.05. The values expressed in fold change from the qPCR assays were subjected to log2 transformation. The transformed values were analyzed by student’s t-test and considered significant at p value < 0.05. The superscripts were placed wherever mean values found significant.
Results
Feed intake, digestibility and rumen fermentation
Data pertaining to feed intake and apparent nutrient digestibility are presented in . The intake of DM did not differ significantly with the supplementation of tamarind seed husk (T), soapnut (S) or their combination (TS) as compared to control (). Similarly, the intake of organic matter (OM) was not affected (p > 0.05) by the supplementation in test groups as compared to control; while higher (p < 0.05) OM intake in TS group than T or S groups was recorded. In contrast, a significant (p < 0.05) decrease in CP intake was evident with the supplementation of S, but it was not different among the T, TS and control groups. Significantly (p < 0.05) greater NDF was recorded in the TS group, but it did not vary significantly among the T, S and control groups. No significant difference was observed in the digestibility of different nutrients (DM, OM, CP, NDF and ADF) among the control and treatment groups.
Table 3. Effect of supplementation of tannins, saponins and their combination on dry matter intake and nutrient digestibility.
The variations in the concentration of NH3-N and TVFA and its different fractions (acetate, propionate, butyrate, isobutyrate, valerate and isovalerate) in rumen fluid among the control and treatment groups are presented in . The concentration of NH3-N was found significantly (p < 0.05) lesser in all the treatment as compared to control groups (). No significant difference in the concentration of TVFA was observed between the T and control groups, but it was significantly (p < 0.05) greater in the S and TS groups than the control. Significantly lower (p < 0.05) proportion of acetate was recorded in the control than T group, but it was not significantly different among the T, S and TS groups. The propionate proportion in the test groups (T, S or TS) was not different than the control group. However, propionate production in the S group was significantly (p < 0.05) higher than the T group. In contrast, the proportions of butyrate, valerate and isovalerate did not differ significantly among the treatment and control groups. No measurable change in the acetate: propionate (A: P) ratio in any of the test groups as compared to control was observed. However, in the group S and TS a narrow (p < 0.05) A: P ratio was evident.
Table 4. Effect of tannins, saponins and their combination on rumen fermentation.
Enteric CH4 emission
A significant (p < 0.05) reduction (19.2%) in the enteric CH4 emission (g/d) was recorded with the supplementation of tamarind seed husk (T) either individually or in combination with soapnut (TS, 14.4%) as compared to the control (). Further, the comparison of enteric CH4 emission on a uniform basis (g/100 kg BW) also revealed a significant (p < 0.05) reduction in the T (19.8%) and TS (14.0%) groups as compared to the control (). Similarly, the supplementation of tamarind seed husk (T) or the combination of tamarind seed husk and soapnut (TS) significantly (p < 0.05) reduced the enteric CH4 emission (g) per kg intake of DM (19.3 and 20.6%) and OM (19.3 and 22.2%) or per kg intake of digestible DM (22.1 and 24.1%), OM (21.2 and 24.5%) and NDF (16.5 and 22.4%) as compared to the control group (). In contrast, the enteric CH4 emissions (derived based on the above parameters) did not differ significantly between the T and TS groups or between the control and S groups ().
Table 5. Effect of supplementation test sources on enteric methane emission and rumen protozoal population.
Effect on rumen microbes
A significant (p < 0.05) reduction in the population of total rumen total protozoa, entodinomorphs and holotrich was observed with the supplementation of tamarind seed husk and soapnut either individually (T and S) or in combination (TS) as compared to the control group ().
The results of the q-PCR-based relative quantification of the selected rumen microbes in the experimental groups are depicted in . It was observed that the population of total rumen methanogen was decreased significantly (p < 0.05) with the supplementation tamarind seed husk (T), but not with the supplementation of soapnut (S) or the combination of tamarind seed husk and soapnut, as compared to control. The population of Fibrobacter succinogens was increased significantly (p < 0.05) in the T and TS groups as compared to control. In contrast, the population of Ruminococcus albus and Ruminococcus flavifaciens was decreased significantly (p < 0.05) in the T and S (Ruminococcus albus) and T (Ruminococcus flavifaciens) groups respectively, as compared to control.
Figure 1. Relative abundance (log2 fold change) of different rumen microbes as affected with the supplementation.
C: control group; T: tamarind seed husk supplemented group; S: soapnut supplemented group; TS: tamarind seed husk and soapnut supplemented group; Met: methanogens; FS: Fibrobacter succinogenes; RA: Ruminicoccus albus; RF: Ruminicoccus flavifaciens.
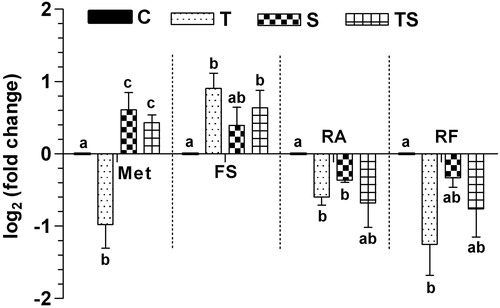
Discussion
Reduction in enteric CH4 emission to stabilize global warming and saving biological energy that otherwise would be lost in the form of CH4 remains a prioritize area of research for animal nutritionist. In recent past, various phyto-sources as such or their extracts have been incorporated in diet and ascertained their impact on rumen methanogenesis [Citation32–36]. Previous studies [Citation4,Citation6,Citation37] confirmed that tamarind (Tamarindus indica) seed husk has tremendous potential for altering rumen fermentation and inhibiting CH4 emission.
Supplementation of tamarind seed husk (group T) established a significant (p < 0.05) reduction in enteric CH4 emission. The CH4 emission in-group T was recorded 19.2 and 16.5% lower than control when compared on g/d and g/kg dNDF, respectively. Supplementation of CT-containing sources inhibits CH4 emission through direct as well indirect mechanisms [Citation38]. Direct inhibition is achieved through a reduction in rumen methanogens [Citation5], while indirect inhibition is primarily due to the reduction in rumen protozoal population. Results from this study confirmed that the reduction in methanogenesis was a combined action of both direct and indirect mechanism. Reduction in CH4 emission with individual supplementation of tamarind seed husk corroborates with previous findings of Malik et al. [Citation6], who also reported 17% reduction in CH4 emission with 5.0% tamarind seed husk supplementation in cattle. These results are in agreement with the earlier findings [Citation39,Citation40] that reported substantial decrease in CH4 emission with CT-containing phyto-sources supplementation. Feeding of phyto-sources that contain tannins gives an unpleasant taste [Citation41] and therefore reduction in feed consumption could be one of the reasons for less CH4 emission. However, condensed tannins equivalent to 40.6 (g/d) or 7.01 (g/kg DM) in-group T not shown any adverse impact on feed intake. Thus, it can be inferred that reduction in methane emission was not attributed to lower feed consumption. Similarly, no adverse impact of tamarind seed husk supplementation was evident in previous studies [Citation6,Citation37] with almost similar levels of supplementation. In another study, Malik et al. [Citation36] also did not find any reduction in DM digestibility with a condensed tannins level of 7.15 to 10.8 g/kg DM supplied through tropical tree leaves supplementation. Impact of tannins on feed intake and digestibility per se dose dependent and a level below 20 g/kg DM barely shows any adverse action [Citation42]. However, Gerlach et al. [Citation43] have recorded decreased intake and digestibility of nutrient in sheep with a much lower (2.3–10.2 g/kg DM) condensed tannins level. Malik et al. [Citation6] also reported an adverse impact with tamarind seed husk supplementation at higher level (10% of diet) equivalent to ∼15 g condensed tannins per kg of DM. This variable impact of tannins to exert adverse impact with lower doses than general recommendation (<20 g/kg DMI) can be justified on the basis of structural differences among tannins [Citation44].
Incorporation of saponin-rich sources often arrived at contrasting results from none to substantial reduction in CH4 emission. However, the reduction in CH4 emission with saponins is source and level dependent [Citation10]. Previous in vitro studies confirmed a reduction in CH4 production with soapnut supplementation [Citation11,Citation45]. In this study, soapnut supplementation at 5.1% equivalent to 5.15 g/kg dry matter or 29.8 g/d in the group S did not reduce CH4 emission and only a non-significant reduction of 6.4 (g/d) or 5.5% (g/kg dNDF) was recorded. The effectiveness of saponins depends on the biological activity that in turn is affected by the nature, number and sequence of sugars molecule in its structures. Soapnut saponins are mostly triterpenoid derived from hederagenin [Citation46]. Monodesmosidic saponins are comparatively more active than bidesmosidic saponins [Citation47]. Soapnut saponins are mostly bidesmosid and therefore their comparative lower biological activity [Citation48] could be one of the reasons for non-significant reduction in methane emission. Host physiological response to supplementation [Citation49] and variations in triterpenoid saponins structure [Citation50] could be the possible reasons for variable response of saponins on CH4 emission. It is well documented that 9 to 25% of the rumen methanogens are associated with protozoa [Citation51,Citation52] and accountable for 37% of CH4 emission [Citation15]. This can be an authentic explanation for lesser CH4 reduction in spite of a significant reduction in protozoal population. Soapnut saponins probably have the limited ability to target archaea-associated protozoa. There was no adverse effect of soapnut individual supplementation on feed intake in this study. Hristov et al. [Citation53] also reported no significant impact of saponins on intake and digestibility of nutrient. In contrast, others [Citation7,Citation8,Citation54,Citation55] have reported a significant reduction in DM digestibility with medium (30 g/kg DM) and high (45 g/kg DM) level of saponins supplementation. In this study, the lower level of saponins (5.13 g/kg DM) supplied through saponut was not adequate to induce any adverse impact on the intake or digestibility of nutrient. These results are in agreement with the previous findings [Citation56].
The interactive effect of tannins and saponins on CH4 emission is not well documented at present. Therefore, an attempt was made in this study to investigate the effect of combined supplementation of tamarind seed husk and soapnut at 5.1% (60:40) on enteric CH4 emission. The results confirmed that combined supplementation in TS group resulted nearly equal reduction in CH4 emission (g/d) as in the group T. The comparison of CH4 emission (g) on the basis of DM intake, digestible DM intake and digestible NDF revealed similar CH4 reduction in the TS and T groups as well. In a previous study in cattle [Citation6], tamarind seed husk at a similar level as in the TS group has been found ineffective in reducing the CH4 emission. In contrast, the same level of tamarind seed husk was found effective in combination with 2% soapnut in this study. Conversely, this study revealed that even individual supplementation of soapnut at higher level (5.1%) was not effective. These findings indicated that there was a synergistic effect of soapnut even at the lower level, in the presence of tamarind seed husk in reducing CH4 emission.
In this study, the reduction in the population of rumen protozoa primarily appears to be accountable for reduced CH4 emission due to the combined supplementation. Soapnut supplementation mostly likely targeted the protozoa that were not associated with rumen archaea as evident in the group S. Nevertheless, it appears that soapnut complimented the tamarind seed husk mediated reduction in the population of archaea associated protozoa in the TS group. The results of the TS group are in agreement with the findings of Yogianto et al. [Citation57] and Yuliana et al. [Citation58], who have demonstrated a significant decrease in CH4 production. Makkar et al [Citation59] have also reported that supplementation of hydrolysable or condensed tannins with saponins at three variable concentrations results additive effect on decreasing gas production. Gunun et al. [Citation60] have also demonstrated that condensed tannins and saponins from rambutan peel powder (RPP) decreased in vitro methane production beyond a level of 16 mg/0.5 g. Anantasook and Wanapat [Citation61] and Anantasook et al. [Citation62,Citation63] studied the combined effect of condensed tannins and saponins containing rain tree (Samanea saman) pod supplementation and reported no adverse impact of pod supplementation at 60 g/kg DM on the rumen fermentation and feed intake.
A significant decrease in ammonia-N in the combined supplementation group (TS) was attributed to the formation of tannin-protein complexes that resulted in less protein degradation in the rumen. The supplementation of condensed tannins sources has more binding affinity to protein than carbohydrates [Citation64]. A shift in fermentation pattern toward more propionate could be another reason for lower CH4 emission; however, in this study, we did not observe any significant change in propionate production in the combined supplementation group. These findings suggested that the CH4 reduction was independent to the shift in fermentation.
The lower methanogenesis in test group T could be attributed to the significant reduction in methanogens numbers, which are ecto or endo symbiotically associated to the protozoa. However, this rationalization did not appear complete as this failed to explain the methane reduction in TS group where rumen methanogens were not altered with the supplementation. From these results, it can be inferred that the reduction in rumen methane is a cumulative result of the reduction in rumen methanogens and protozoa. Previous reports [Citation65,Citation66] also confirmed a weak correlation between methanogens density and methane emission. Fibrolytic microbes exhibit different response to the supplementation of tannin-rich plants [Citation67] and Fibrobacter succinogenes attached more firmly to the cellulose than any other cellulolytic bacteria [Citation68]. Nevertheless, we did not find any adverse impact of tannins or saponins phyto-source Individual or combined supplementation on F. succinogenes. This was certainly due to the moderate level of tannins and saponins used in this study. F. succinogenes do not have syntrophic relations with methanogens and also not compete for hydrogen as an electron donor [Citation69]. Therefore, no concurrent decrease F. succinogenes with methanogens numbers was reported in this study. Findings of Kurihara et al. [Citation70] strengthen our results reported differential response of fibrolytic microbes to tannins and saponins supplementation. In our study, Ruminococcus albus and R. flavifaciens found more susceptible to the tannins. The similar fiber digestibility among control and test groups could be explained by the fluctuating numbers of fibrolytic microbes which lead to a balance within fibrolytic microbial consortia and therefore fiber digestibility in this was not affected.
In conclusion, the combined supplementation of tamarind seed husk and soapnut at 5.1% (60:40) achieved significant reduction in enteric CH4 emission and was found equally effective as that of the individual supplementation of tamarind seed husk at similar level. In contrast, soapnut supplementation at similar level in short duration was not effective in reducing CH4 emission to a significant extent. In order to confirm the persistency of inhibitory effect, long-term studies with the combined supplementation of tamarind seed husk and soapnut are warranted.
Conflict of interest
No conflict of interest is declared
Acknowledgements
The authors are grateful to the Department of Biotechnology (DBT), Govt. of India, New Delhi for providing the financial support to carry out this research work under the project (BT/PR8750/AAQ/1/555/2013) entitled “Livestock methane reduction through immunization based approach”. The first author is also thankful to the Director, National Institute of Animal Nutrition and Physiology, Bangalore for extending facilities for the study.
References
- Guan H, Wittenberg KM, Ominski KH, et al. Efficacy of ionophores in cattle diets for mitigation of enteric methane. J Anim Sci. 2006;84:1896–1906. doi: 10.2527/jas.2005-652.
- Malik PK, Bhatta R, Soren NM, et al. Feed based approaches in enteric methane amelioration. In: Malik PK, Bhatta R, Takahashi J, Kohan RA, Prasad CS editors. Livestock production and climate change, CABI climate change series. CABI, Nosworthy Way, Wallingford, Oxfordshire, UK. Vol. 6, 2015. p. 336–359.
- Bhatta R, Saravanan M, Baruah L, et al. Effects of graded levels of tannin-containing tropical tree leaves on in vitro rumen fermentation, total protozoa and methane production. J Appl Microbiol. 2015;118:557–564. doi: 10.1111/jam.12723.
- Bhatta R, Krishnamoorthy U, Mohammed F. Effect of tamarind (Tamarindus indica) seed husk tannins on in vitro rumen fermentation. Anim Feed Sci Tech. 2001;90:143–152. doi: 10.1016/S0377-8401(01)00204-8.
- McSweeney CS, Palmer B, Bunch R, et al. Effect of the tropical forage Calliandra on microbial protein synthesis and ecology in the rumen. J Applied Microbiol. 2001;90:78–88. doi: 10.1046/j.1365-2672.2001.01220.x.
- Malik PK, Kolte AP, Bakshi B, et al. Effect of tamarind seed husk supplementation on ruminal methanogenesis, methanogen diversity and fermentation characteristics. Carbon Manage. 2017;8:319–329. doi: 10.1080/17583004.2017.1357403.
- Lila ZA, Mohammed N, Kanda S, et al. Effect of sarsaponin on ruminal fermentation with particular reference to methane production in vitro. J Dairy Sci. 2003;86:3330–3336. doi: 10.3168/jds.S0022-0302(03)73935-6.
- Malik PK, Singhal KK. Influence of supplementation of wheat straw based total mixed ration with saponins on total gas and methane production in vitro. Indian J Anim Sci. 2008;78:987–990.
- Sirohi SK, Goel N, Singh N. Utilization of saponins, a plant secondary metabolite in enteric methane mitigation and rumen modulation. Ann Res Rev Biol. 2014;4:1–19. doi: 10.9734/ARRB/2014/5323.
- Jayanegara A, Wina E, Takahashi J. Meta-analysis on methane mitigating properties of saponin-rich sources in the rumen: influence of addition levels and plant sources. Asian Australas J Anim Sci. 2014;27:1426–1435. doi: 10.5713/ajas.2014.14086.
- Agarwal N, Kamra DN, Chaudhary LC, et al. Effect of Sapindus mukorossi extracts on in vitro methanogenesis and fermentation characteristics in buffalo rumen liquor. J Appl Anim Res. 2006;30:1–4. doi: 10.1080/09712119.2006.9706814.
- Malik PK, Singhal KK. Effect of Lucerne (Medicago sativa) fodder supplementation on nutrient utilization and enteric methane emission in male buffalo calves fed on wheat straw based total mixed ration. Indian J Anim Sci. 2009;79:416–421.
- McSweeney CS, Ramirez-Restrepo CA. Supplementation with tea saponins and statins to reduce methane emissions from ruminants. North Sydney (NSW): Meat & Livestock Australia Limited; 2015. (Project report (B.CCH.6430)).
- Bhatta R, Uyeno Y, Tajima K, et al. Difference in the nature of tannins on in vitro ruminal methane and volatile fatty acid production and on methanogenic archaea and protozoal populations. J Dairy Sci. 2009;92:5512–5522. doi: 10.3168/jds.2008-1441.
- Finlay DJ, Esteban G, Clarke KJ, et al. Some rumen ciliates have endosymbiotic methanogens. FEMS Microbiol Lett. 1994;117:157–162. doi: 10.1111/j.1574-6968.1994.tb06758.x.
- Hess HD, Kreuzer M, Dı́az TE, et al. Saponin rich tropical fruits affect fermentation and methanogenesis in faunated and defaunated rumen fluid. Anim Feed Sci Technol. 2003;109:79–94. doi: 10.1016/S0377-8401(03)00212-8.
- ICAR. Nutrient requirements of cattle and buffalo. New Delhi (India): Indian Council of Agricultural Research; 2013. p. 1–59.
- Johnson K, Huyler M, Westberg H, et al. Measurement of methane emissions from ruminant livestock using a sulfur hexafluoride tracer technique. Environ Sci Technol. 1994;28:359–362. doi: 10.1021/es00051a025.
- Lassey K. Workshop report: SF6 tracer technique guidelines. Palmerston North (New Zealand). Ministry for Primary Industries, Wellington, New Zealand; 2011.
- AOAC. Official methods of analysis. 17th ed. Arlington (VA): Association of Official Analytical Chemists; 1990.
- Van Soest PJ, Robertson JB, Lewis BA. Methods for dietary fiber, neutral detergent fiber, and nonstarch polysaccharides in relation to animal nutrition . J Dairy Sci. 1991;74:3583–3597. doi: 10.3168/jds.S0022-0302(91)78551-2.
- Makkar HPS. Quantification of tannins in tree and shrub foliage—a laboratory manual. Dordrecht (The Netherlands): Kluwer Academic Publishers; 2003.
- Upadhyay A, Singh DK. Pharmacological effects of Sapindus mukorossi. Rev Inst Med Trop Sao Paulo. 2012;54:273–280. doi: 10.1590/s0036-46652012000500007.
- Filípek J, Dvořák R. Determination of the volatile fatty acid content in the rumen liquid: comparison of gas chromatography and capillary isotachophoresis. Acta Vet Brno. 2009;78:627–633. doi: 10.2754/avb200978040627.
- Conway EJ. Micro-diffusion analysis and volumetric error. 4th ed. London: Crossby Lockwood and Son Ltd.; 1957. p. xviii + 465.
- Hungate RE. The rumen and its microbes. New York (NY): Academic Press; 1966. p. 1–533.
- Yu Z, Morrison M. Improved extraction of PCR-quality community DNA from digesta and fecal samples. BioTechniques. 2004;36:808–812. doi: 10.2144/04365ST04.
- Denman SE, Tomkins NW, McSweeney CS. Quantitation and diversity analysis of ruminal methanogenic populations in response to the antimethanogenic compound bromochloromethane. FEMS Microbiol Ecol. 2007;62:313–322. doi: 10.1111/j.1574-6941.2007.00394.x.
- Denman SE, McSweeney CS. Development of a real-time PCR assay for monitoring anaerobic fungal and cellulolytic bacterial populations within the rumen. FEMS Microbiol Ecol. 2006;58:572–582. doi: 10.1111/j.1574-6941.2006.00190.x.
- Wang B, Neue HU, Samonte HP. Effect of cultivar difference (’IR72’, “IR65598” and “Dular”) on methane emission. Agric Ecosyst Environ. 1997;62:31–40. doi: 10.1016/S0167-8809(96)01115-2.
- IBM Corp. IBM SPSS statistics for windows, version 21.0. Armonk (NY): IBM Corp. 2012.
- Alves TP, Dall-Orsoletta AC, Ribeiro-Filho H. The effects of supplementing Acacia mearnsii tannin extract on dairy cow dry matter intake, milk production and methane emission in a tropical pasture. Trop Anim Health Prod. 2017;49:1663–1668. doi: 10.1007/s11250-017-1374-9.
- Gemeda BS, Hassen A. The potential of tropical tannin rich browses in reduction of enteric methane. Approaches Poult Dairy Vet Sci. 2018;2: 1-9. doi: 10.31031/APDV.2018.02.000538.
- Widiawati Y, Puastuti W. The effect of condensed tannin and saponin in reducing methane produced during rumen digestion of agricultural byproducts. Proceedings of International Seminar on Livestock Production and Veterinary Technology. Ministry of Agriculture, Republic of Indonesia, Bali, Indonesia; 2016. DOI:http://dx.doi.org/10.14334/Proc.Intsem.LPVT-2016-p.139-146.
- Malik PK, Singhal KK, Deshpande SB. Effect of saponin rich Lucerne fodder supplementation on rumen fermentation, bacterial and protozoal population in buffalo bulls. Indian J Anim Sci. 2009;79:912–916.
- Malik PK, Kolte AP, Baruah L, et al. Enteric methane mitigation in sheep through leaves of selected tanniniferous tropical tree species. Livstock Sci. 2017;200:29–34. doi: 10.1016/j.livsci.2017.04.001.
- Bhatta R, Krishnamoorthy U, Mohammed F. Effect of feeding tamarind (Tamarindus indica) seed husk as a source of tannin on dry matter intake, digestibility of nutrients and production performance of crossbred dairy cows in mid-lactation. Anim Feed Sci Tech. 2000;83:67–74. doi: 10.1016/S0377-8401(99)00118-2.
- Tavendale MH, Meagher LP, Pacheco D, et al. Methane production from in vitro rumen incubations with lotus pedunculatus and Medicago sativa, and effects of extractable condensed tannin fractions on methanogenesis. Anim Feed Sci Tech. 2005;123–124:403–419. doi: 10.1016/j.anifeedsci.2005.04.037.
- Carulla JE, Kreuzer M, Machmüller A, et al. Supplementation of acacia mearnsii tannins decreases methanogenesis and urinary nitrogen in forage-fed sheep. Aust J Agric Res. 2005;56:961–970. doi: 10.1071/AR05022.
- Puchala R, Min BR, Goetsch AL, et al. The effect of a condensed tannin-containing forage on methane emission by goats. J Anim Sci. 2005;83:182–186. doi: 10.2527/2005.831182x.
- Becker K, Makkar H. Effects of dietary tannic acid and quebracho tannin on growth performance and metabolic rates of common carp (Cyprinus carpio L.). Aquaculture. 1999;175:327–335. doi: 10.1016/S0044-8486(99)00106-4.
- Waghorn GC, Ulyatt MJ, John A, et al. The effect of condensed tannins on the site of digestion of amino acids and other nutrients in sheep fed on Lotus corniculatus L. Br J Nutr. 1987;57:115–126. doi: 10.1079/BJN19870015.
- Gerlach K, Pries M, Sudekum KH. Effect of condensed tannin supplementation on in vivo nutrient digestibilities and energy values of concentrates in sheep. Small Rumin Res. 2018;161:57–62. doi: 10.1016/j.smallrumres.2018.01.017.
- Mueller-Harvey I. Unravelling the conundrum of tannins in animal nutrition and health. J Sci Food Agric. 2006;86:2010–2037. doi: 10.1002/jsfa.2577.
- Kamra DN, Pawar M, Singh B. Effect of plant secondary metabolites on rumen methanogens and methane emissions by ruminants. In: Patra AK, editors. Dietary phytochemicals and microbes. Dordrecht (The Netherlands): Springer Netherlands; 2012. p. 351–370.
- Saxena D, Pal R, Dwivedi AK, et al. Characterization of sapindosides in Sapindus mukorosii saponin (reetha saponin) and quantitative determination of sapindoside B. J Sci Res. 2003;63:181–186.
- Voutquenne L, Lavaud C, Massiot G, et al. Structure-activity relationships of haemolytic saponins. Pharma Biol. 2002;40:253–262. doi: 10.1076/phbi.40.4.253.8470.
- Sharma A, Sati SC, Sati OP, et al. Triterpenoid saponins from the pericarps of Sapindus mukorossi. J Chem. 2013;2013:1–5. doi: 10.1155/2013/613190.
- Ramirez-Restrepo CA, O’Neil CJ, Lopez VN, et al. Tropical cattle methane emissions: the role of natural statins supplementation. Anim Prod Sci. 2014;54:1294–1299. doi: 10.1071/AN14246.
- Dinda B, Debnath S, Mohanta BC, et al. Naturally occurring triterpenoid saponins. Chem Biodivers. 2010;7:2327–2580. doi: 10.1002/cbdv.200800070.
- Newbold CJ, Lassalas B, Jouany JP. The importance of methanogens associated with ciliate protozoa in ruminal methane production in vitro. Lett Appl Microbiol. 1995;21:230–234.
- Belanche A, Fuente G, de la Newbold CJ. Study of methanogen communities associated with different rumen protozoal populations. FEMS Microbiol Ecol. 2014;90:663–677. doi: 10.1111/1574-6941.12423.
- Hristov AN, McAllister TA, Van Herk FH, et al. Effect of Yucca schidigera on ruminal fermentation and nutrient digestion in heifers. J Anim Sci. 1999;77:2554–2563. doi: 10.2527/1999.7792554x.
- Malik PK, Singhal KK, Deshpande SB. Effect of Lucerne fodder (first cut) supplementation on in vitro methane production, fermentation pattern and protozoal counts. Indian J Anim Sci. 2010; 80:998–1002.
- Holtshausen L, Chaves AV, Beauchemin KA, et al. Feeding saponin-containing Yucca schidigera and Quillaja saponaria to decrease enteric methane production in dairy cows. J Dairy Sci. 2009;92:2809–2821. doi: 10.3168/jds.2008-1843.
- Jayanegara A, Goel G, Makkar HPS, et al. Reduction in methane emissions from ruminants by plant secondary metabolites: effects of polyphenols and saponins. In: Odongo NE, Garcia M, Viljoen GJ, editors. Sustainable improvement of animal production and health. Rome (Italy): Food and Agriculture Organization of the United Nations; 2010. p. 151–157.
- Yogianto Y, Sudarman A, Wina E, et al. Supplementation effects of tannin and saponin extracts to diets with different forage to concentrate ratio on in vitro rumen fermentation and methanogenesis. J Indones Trop Anim Agric. 2014;39:144–151.
- Yuliana P, Laconi EB, Wina E, et al. Extraction of tannins and saponins from plant sources and their effects on in vitro methanogenesis and rumen fermentation. J Indones Trop Anim Agri. 2014;39:91–97.
- Makkar HPS, Blummel M, Becker K. In vitro effects of and interactions between tannins and saponins and fate of tannins in the rumen. J Sci Food Agric. 1995;69:481–493. doi: 10.1002/jsfa.2740690413.
- Gunun P, Gunun N, Cherdthong A, et al. In vitro rumen fermentation and methane production as affected by rambutan peel powder. J Appl Anim. Res. 2018;46:626–631. doi: 10.1080/09712119.2017.1371608.
- Anantasook N, Wanapat M. Influence of rain tree pod meal supplementation on rice straw based diets using in vitro gas fermentation technique. Asian Australas J Anim Sci. 2012;25:325–334. doi: 10.5713/ajas.2011.11131.
- Anantasook N, Wanapat M, Cherdthong A. Manipulation of ruminal fermentation and methane production by supplementation of rain tree pod meal containing tannins and saponins in growing dairy steers. J Anim Physiol Anim Nutr. 2014;98:50–55. doi: 10.1111/jpn.12029.
- Anantasook N, Wanapat M, Cherdthong A, et al. Effect of tannins and saponins in Samanea saman on rumen environment, milk yield and milk composition in lactating dairy cows. J Anim Physiol Anim Nutr. 2015;99:335–344. doi: 10.1111/jpn.12198.
- Barry TM. Condensed tannins: their role in ruminant protein and carbohydrate digestion and possible effects upon the rumen ecosystem. In: Nolan JV, Leng RA, Demeyer DI, editors. Roles of protozoa and fungi in ruminant digestion. Armidale (NSW): Penambul Books; 1989. p. 102–145.
- Morgavi DP, Martin C, Jouany J-P, et al. Rumen protozoa and methanogenesis: not a simple cause-effect relationship. Br J Nutr. 2012;107:388–397. doi: 10.1017/S0007114511002935.
- Zhou M, Chung YH, Beauchemin KA, et al. Relationship between rumen methanogens and methane production in dairy cows fed diets supplemented with a feed enzyme additive. J Appl Microbiol. 2011;111:1148–1158. doi: 10.1111/j.1365-2672.2011.05126.x.
- Rira M, Morgavi DP, Archimède H, et al. Potential of tannin-rich plants for modulating ruminal microbes and ruminal fermentation in sheep. J Anim Sci. 2015;93:334–347. doi: 10.2527/jas.2014-7961.
- Shinkai T, Kobayashi Y. Localization of ruminal cellulolytic bacteria on plant fibrous materials as determined by fluorescence in situ hybridization and real-time PCR. Appl Environ Microbiol. 2007;73:1646–1652. doi: 10.1128/AEM.01896-06.
- Asanuma N, Iwamoto M, Hino T. Effect of the addition of fumarate on methane production by ruminal microorganisms in vitro. J Dairy Sci. 1999;82:780–787. doi: 10.3168/jds.S0022-0302(99)75296-3.
- Kurihara Y, Eadie JM, Hobson PN, et al. Relationship between bacteria and ciliate protozoa in the sheep rumen. J Gen Microbiol. 1968;51:267–288. doi: 10.1099/00221287-51-2-267.