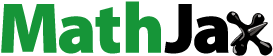
Abstract
The nature of carbon input to soil, its transformation to soil organic matter (SOM), and the degree of protection from decomposition are important for long-term persistence of SOM. The effect of exogenous addition of organic matter on SOM build-up is well known; however, changes in quality vis-à-vis composition of added organic matter have been less investigated. It is necessary to ascertain the form under which C accumulates and how the composition of added organic matter influences the stabilization of soil organic C (SOC). Changes in composition of SOM were studied by fractionating the soil into coarse (cPOC; >250 µm) and fine particulate organic C (fPOC; 53–250 µm) and mineral-associated organic C (MinOC; <53 µm) and measuring the acid non-hydrolyzable C (NHC) as well as mineralizable C following repeated applications of farmyard manure (FYM), rice straw compost (RSC) and vermicompost (VC) in a rice (Oryza sativa L.)–wheat (Triticum aestivum L.) system field experiment. Addition of organic amendments for 10 years significantly increased SOC stocks and the increase was greatest with RSC (12.2 t ha−1) followed by FYM (9.1 t ha−1), and lowest with VC (8.5 t ha−1), compared with solitary application of NPK. The C accumulation in separated pools was influenced by the chemical composition of the organic source. Compost applications accumulated C preferentially in the MinOC pool, whereas the FYM accumulated a greater proportion of C as POC, particularly as fPOC. The differences were attributed to the lower C/N ratio and higher lignin content of RSC than FYM. The RSC application increased NHC stocks to a significantly greater extent than FYM and VC, indicating recalcitrance of the accumulated C. This was substantiated by the significantly lower C mineralization potential of RSC-amended soils compared to the FYM- and VC-amended soils. The results showed that addition of compost, besides leading to C build-up, improved C stabilization by imparting recalcitrance to SOC.
Introduction
Carbon (C) sequestration in soil has an important role in climate regulation [Citation1]. Net C storage in soil depends on the transformation of C input to soil organic matter (SOM) and the mineralization of SOM via microbial decomposition [Citation2]. Soil acts as a C sink when formation of SOM exceeds decomposition. Formation of SOM can be enhanced by the application of organic amendments (compost, manure, crop residues), reduced or zero tillage, improved crop rotations and balanced fertilization [Citation3–5]. Although much is known about the effect of different organic amendments on soil C build-up [Citation6,Citation7], the mechanism of SOM stabilization in soils amended with organic sources that differ in composition is not well understood. The traditional kinetic theory proposes that stabilization of SOM is controlled by the formation of chemically complex C components resistant to microbial degradation [Citation8]. However, recent developments in understanding SOM stabilization support the importance of SOM–mineral associations and the inaccessibility of occluded SOM to decomposition [Citation9–12]. Therefore, evaluating the effect of organic amendments on chemically recalcitrant and mineral-associated C pools could help in understanding the mechanism of SOM stabilization in organically amended soils.
The chemical composition of C input to soil, such as the C/N ratio and lignin, cellulose and phenol contents, influences the decomposition of SOM [Citation12]. Benbi and Khosa [Citation13] reported, from a laboratory incubation study, that SOM decomposed to a greater extent when organic matter with relatively lower C/N and lignin/N ratios was added to the soil. Besides molecular complexity, persistence of SOM is also influenced by ecological and environmental factors [Citation14]. Berti et al. [Citation15] found that the persistence of SOM was influenced by the quality of added C, which in turn was a function of physicochemical and biological characteristics of the surrounding environment. Though several studies have quantified the effect of manure application in rice–wheat system on changes in soil organic C (SOC) stocks [Citation16–18], the effect of organic amendments varying in chemical composition on C accumulation in labile and recalcitrant forms of SOM is relatively less studied. From a long-term perspective on the persistence of accumulated organic C in soil, it is imperative to determine under which form the C accumulates and how the composition of added organic matter influences the stabilization of organic C in soil. Therefore, studying the distribution of accumulated C in different pools with varying turnover rates would help in devising strategies for enhancing SOM stabilization.
Rice–wheat is a major cropping system in India and occupies an area of 12.3 Mha [Citation19]. In the north Indian state of Punjab, rice–wheat is grown on 3.16 Mha, constituting 75% of the cropped area [Citation20]. Annually, huge quantities of rice (20.2 Mt) and wheat (14 Mt) residues are produced in the state. While most of the wheat residue is used as animal fodder, the majority of rice residue is subjected to open field burning. An estimated 9.7 Mt of rice residue was burnt in situ during 2018 [Citation21]. Besides leading to a loss of C and other essential nutrients (N, P, K and S), the burning of rice residues leads to emission of greenhouse gases and causes adverse environmental and human health effects [Citation22]. Therefore, it is imperative to devise practices for on-farm rice residue management. A number of on-farm residue management options, such as incorporating the residue in the soil, seeding the following crop with zero-till machines in standing stubble or leaving the residue as surface mulch are currently being evaluated and advocated for adoption. Incorporation of rice straw per se is constrained by the high C/N ratio of the residue and the difficulty of seedbed preparation for the following crop. Seeding of wheat crop using zero tillage with rice residue retention, however, has obvious benefits in terms of timely sowing and labor saving, yet it has limited adoption because of large financial investment, greater energy consumption and lower crop yields [Citation23]. Another eco-friendly and cost-effective option could be to prepare rice straw compost (RSC) by subjecting rice straw to decomposition prior to application in the field. Besides rice residue, surplus agricultural biomass from other crops along with animal manure could be subjected to vermicomposting. Composting has been reported to increase the content of recalcitrant humified components [Citation24,Citation25] and stable C components such as lignin, aromatics and O-alkyl in its initial composition, which could influence SOM stabilization [Citation26,Citation27]. Therefore, it is hypothesized that the chemical composition of C input, besides influencing the quantity of SOC, will impact the quality of organic C and its stabilization in soil. The specific objectives were to evaluate the impact of long-term addition of farmyard manure (FYM), RSC, vermicompost (VC) and the integrated use of FYM and mineral fertilizers in a rice–wheat system on C accumulation and quality of SOM in terms of lability and recalcitrance.
Materials and methods
Experimental site
A long-term field experiment with a rice (Oryza sativa L.)–wheat (Triticum aestivum L.) sequence was started in 2006–07 on a sandy loam soil (70% sand and 12% clay) at Punjab Agricultural University research farm, Ludhiana, India. The region, located at 30°56′ N latitude and 75°52′ E longitude, 247 m above sea level, is classified as semiarid subtropical. During the experimental period (2006–17), the site received 779 ± 285 mm rainfall annually, of which more than 75% occurred during monsoon season (June to September). The mean monthly maximum and minimum air temperature averaged 33 ± 0.4 °C and 22 ± 0.4 °C during rice season (June–November) and 24 ± 0.6 °C and 11 ± 0.7 °C during wheat season (December–April), respectively. At the start of the experiment, the surface (15 cm) soil was characterized as near neutral in reaction (pH 7.4), non-saline (electrical conductivity; 0.28 dS m−1), with total organic C in soil 4.8 g kg−1, available N 141 kg ha−1, Olsen extractable P 21.8 kg ha−1 and neutral ammonium acetate extractable K 189.6 kg ha−1.
Experimental details
The experiment consisted of six treatments: (i) NPK: application of recommended amounts of N, P and K fertilizers to both rice and wheat; (ii) INM: joint application of recommended amounts of NPK along with FYM to supply 200 kg N ha−1 to wheat; (iii) FYM: application of farmyard manure to supply 400 kg N ha−1 to wheat; (iv) RSC: application of rice straw compost to supply 400 kg N ha−1 to wheat; (v) VC: application of vermicompost to supply 400 kg N ha−1 to wheat; and (vi) unfertilized control. Each treatment was replicated 3 times (plot size 10 × 4 m2) in a randomized block design. Since the organic amendments were applied to wheat only, the nutritional requirement of rice was met through green manuring. A green manure crop of sunhemp (Crotalaria juncea L.) was grown in situ during the third week of May on the plots receiving FYM, RSC and VC. After 45 days of growth, the crop was plowed into the field before transplanting rice. Nitrogen (125 kg ha−1) was applied in the form of urea (46% N) in three equal splits in NPK and INM plots: at puddling, and 3 and 6 weeks after transplanting rice. Fertilizer K (30 K2O kg ha−1), in the form of muriate of potash (60% K2O), was applied as a basal dose to the rice crop in the relevant treatments. Before transplanting rice, the field was plowed twice with a tine cultivator under water-inundated conditions, followed by planking to create puddled conditions. Rice seedlings (aromatic rice variety PB-3) about 40 days old were transplanted during July 7–10 each year with 20 cm row spacing and 15 cm plant spacing. The rice field was kept under water for the first 15 days after transplanting. Thereafter, irrigation was supplied 2 days after the ponded water had infiltrated the soil. The crop was harvested manually during the third week of November each year. After rice harvest, organic amendments were applied 10–15 days prior to seeding wheat. The field was disked twice at the field capacity moisture. Nitrogen (125 kg ha−1) was applied through urea (46% N) in two equal splits (at sowing and with first irrigation). Fertilizer P (30 kg ha−1) was applied as basal dose in relevant plots in the for of single superphosphate (16% P2O5), and fertilizer K was omitted as soil had sufficient available K. Wheat (variety HD-2967) was sown during the first week of December each year in rows 22.5 cm apart. Irrigation was applied as per requirements based on visual examination of the field. Wheat was harvested manually in the first week of May. Except for the source of nutrients, organic and inorganic, the experiment was maintained without the use of pesticides or any other chemical.
Root and stubble C input
Root samples were collected at 50% panicle emergence in rice and at 50% heading in wheat with a tube auger (6 cm inner diameter) to a depth of 60 cm, as per the method described by Kumar et al. [Citation28]. The root samples were washed in nylon net bags (250 µm pore size) under running water and were cleaned of dead roots, weed seeds and other visible organic matter. Samples of stubble plus leaf litter were collected from a 2 m2 area at harvest of both crops. The samples were cleaned to remove sticking soil particles. The root and stubble plus leaf litter samples were oven dried at 60 °C and ground to pass a 250-µm sieve. Total C content was determined by dry combustion using a CHN analyzer (Elementar model Vario EL III cube). The C input to soil was calculated by multiplying root and stubble plus leaf litter biomass with measured C contents of 40.4% and 42.1% (wheat) and 39.8% and 40.2% (rice), respectively. Rhizodeposition was calculated by multiplying root-C by a factor of 0.63 [Citation29].
Chemical composition of organic amendments
The samples of FYM, RSC and VC were analyzed for chemical composition using standard methods. The samples were brought to the laboratory and oven dried at 60 °C. The dried samples were finely ground and passed through a 250-µm sieve. Total C and N contents were determined by dry combustion using a CHN analyzer (Elementar model Vario EL III cube). Lignin, cellulose and hemicellulose were determined by the neutral detergent fraction (NDF) and acid detergent fraction (ADF) methods described by Goering and Van Soest [Citation30].
Soil sampling and analysis
After wheat harvest (May 2017), soil samples were collected from each plot at 0–7.5, 7.5–15, 15–30, 30–45 and 45–60 cm soil depths using a tube auger (7.5 cm inner diameter). Each sample, a composite of 10 sites within a plot, was air-dried and ground with a wooden pestle and mortar to pass through a 2-mm sieve, and stored in plastic containers prior to analysis.
Soil organic carbon
Soil samples were cleaned of any visible plant residue and ground to pass through a 250-µm sieve. Total C in soils was analyzed by dry combustion using a CHN analyzer (Elementar model Vario EL III). Inorganic C in soils, analyzed using dilute HCl [Citation31], was absent; therefore, soil organic carbon (SOC) was equal to total C. Organic carbon stocks for each soil depth were calculated using measured bulk density (Db) by the core method, and SOC concentration (EquationEquation 1(1)
(1) ). For practical reasons, Db below 30 cm could not be measured; therefore, the Db values for the 15–30 cm layer were used to calculate SOC stocks at 30–45 and 45–60 cm soil depths.
(1)
(1)
For calculating C accumulation in response to management, the NPK treatment was taken as a reference as it is the most prevalent practice among the farmers in the experimental region. The amount of C accumulated in each treatment over NPK in progressively increasing profile depths (i.e. 0–7.5, 0–15, 0–30, 0–45 and 0–60 cm) was calculated as per EquationEquation (2)(2)
(2) :
(2)
(2)
where SOCtreatment = SOC stock (t ha−1) in treated plot at a given depth and SOCNPK = SOC stocks (t ha−1) in NPK plots at the corresponding depth.
Fractionation of soil organic matter
Size fractions of SOM, viz. coarse particulate (cPOM), fine particulate (fPOM), and mineral-associated organic matter (MinOM), in 0–7.5 and 7.5–15 cm soil layers were separated as per the method described by Benbi et al. [Citation18]. The size fraction >250 µm comprised cPOM and sand, the 250–53 µm size category comprised fPOM and fine sand and <53 µm was MinOM and silt plus clay. Total C in each fraction was analyzed by dry combustion with a CHN analyzer (Elementar model Vario EL III).
Non-hydrolyzable C (NHC) in 0–7.5 cm and 7.5–15 cm soil layers was determined as per the method proposed by Silveira et al. [Citation32]. One gram of air-dried sample was reacted with 50 mL of 6 M HCl at 105 °C for 4 h. After hydrolysis, the residue was separated from the supernatant by centrifugation (5 min at 5000 rpm). The residue was made chloride free by washing with de-ionized water and was dried at 60 °C in a hot-air oven. The NHC in the residue was analyzed by dry combustion with a CHN analyzer (Elementar model Vario EL III). The NHC stocks were calculated using EquationEquation (1)(1)
(1) .
Carbon mineralization kinetics
Carbon mineralization was studied by incubating soil samples at field capacity moisture and 25 °C temperature in a BOD (Bio-Oxygen Demand) incubator for 62 days. Fifty grams of soil from each treatment was brought to field capacity moisture and placed in 500-mL conical flask. Vials containing 10 mL of 0.1 M NaOH were placed inside the flasks to trap evolved CO2. The alkali in the traps was replaced daily during the first 10 days of incubation and every 2–3 days thereafter. The amount of CO2 evolved at a given time was measured by titrating alkali in the traps against 0.1 M HCl using phenolphthalein as indicator. After 62 days of incubation, the cumulative amount of CO2-C evolved was considered mineralizable C (Cmin).
Statistical analysis and model fitting
Treatment effects on C input, Db, SOC, particulate organic C (cPOC, fPOC), MinOC, NHC and mineralizable C were statistically analyzed in a complete randomized block design using Duncan’s multiple range test in SAS for Windows 64.0 (SAS 9.4 Incv. Cary, North Carolina, USA). The mean values of treatments were tested for statistical significance at a 5% (p < 0.05) level of probability. Regression analyses were performed using SPSS software v. 20 (SPSS Inc., Chicago, IL, USA).
The time course of C mineralization was described by fitting a first-order kinetic model [Citation33] between cumulative C mineralization (Ct, mg kg−1) and time (t, days) using EquationEquation (3)(3)
(3) :
(3)
(3)
where C0 represents the carbon mineralization potential (mg kg−1) and k is the first-order rate coefficient (d−1). The kinetic model was fitted by a non-linear least squares technique using SPSS software.
Results
Carbon input to soil
Annual plant-mediated C input through stubble plus leaf litter, roots and rhizodeposition ranged between 1.79 and 3.52 t ha−1 in different treatments (). Total C input through rice and wheat roots and the corresponding rhizodeposition C and stubble plus leaf litter C was significantly (p < 0.05) higher in organically amended plots compared to NPK plots. The root-mediated C input in both rice and wheat was highest in RSC, followed by FYM, and lowest in VC-treated plots. The annual exogenous input of C amounted to 5.8–15.2 t C ha−1 through different sources.
Table 1. Annual exogenous and plant-mediated C input in a rice–wheat system in unamended control plots and plots amended with fertilizer NPK, farmyard manure (FYM), vermicompost (VC), rice straw compost (RSC) and NPK plus FYM (INM). Numbers in parentheses indicate standard error.
Chemical composition of organic sources
The organic amendments varied in C, N, C/N, lignin/N ratios and lignin content (). Among organic amendments, FYM had the highest C/N ratio followed by RSC, while VC had the lowest C/N ratio. On the other hand, RSC had the highest lignin/N ratio and FYM the lowest.
Table 2. Chemical composition of farmyard manure (FYM), vermicompost (VC) and rice straw compost (RSC). Numbers in parentheses indicate standard error.
Carbon accumulation in soil
Soil organic C concentration and stocks in the 0–7.5 and 7.5–15 cm soil layers were significantly (p < 0.05) higher in organically amended and INM plots than in those treated with fertilizer NPK (). The highest SOC concentration was observed in FYM plots; RSC plots were similarly high, and the lowest values were found in VC-amended plots. Addition of organics significantly (p < 0.05) decreased Db in 0–7.5 and 7.5–15 cm soil layers compared to mineral fertilized treatment. The lowest Db resulted with the addition of FYM, followed by RSC- and VC-treated plots, in 0–7.5 and 7.5–15 cm soil layers (). Application of FYM, RSC and VC increased SOC stocks in 0–7.5 cm soil layer by 40.9, 41.2 and 24.7%, respectively, over NPK. The corresponding increase in SOC stocks in the 7.5–15 cm layer was 43.8, 42.4 and 28.6%, respectively. Joint application of FYM and NPK fertilizers (INM) significantly (p < 0.05) increased SOC stocks by 17.9 and 11.8% in 0–7.5 and 7.5–15 cm soil layers, respectively, compared to NPK treatment. A significant (p < 0.05) effect of organic amendments and INM on SOC concentration and stocks was also observed for the 15–30 cm soil layer. The effect of organics on SOC concentration as well as stocks was not significant below 30 cm soil depth. Considering 0–7.5 and 7.5–15 cm soil layers together, the addition of organics significantly (p < 0.05) increased SOC stocks by 4.7 to 7.1 t ha−1 (). In the entire 60-cm profile, RSC resulted in the highest (12.2 t ha−1) C accumulation, followed by FYM (9.1 t ha−1), VC (8.5 t ha−1) and INM (4.3 t ha−1). The unamended control resulted in a decline in SOC accumulation at all profile depths.
Figure 1. Carbon accumulation (over fertilizer NPK) in soil at different profile depths after 10 years of management with farmyard manure (FYM), vermicompost (VC), rice straw compost (RSC) and integrated use of NPK and FYM (INM) and unamended control in a rice–wheat system. Vertical line bars show standard error. Bars labeled with different letters indicate significant (p < 0.05) difference by Duncan’s multiple range test.
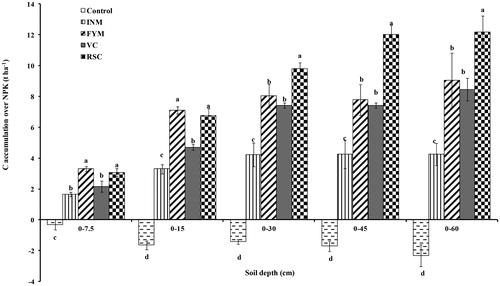
Table 3. Soil organic carbon (SOC) concentration, bulk density and C stocks in the 60-cm profile after 10 years in a rice–wheat system in unamended control plots and plots amended with fertilizer NPK, farmyard manure (FYM), vermicompost (VC), rice straw compost (RSC) and NPK plus FYM (INM). Numbers in parentheses indicate standard error.
Particulate and mineral-associated organic carbon
Organic amendments significantly (p < 0.05) increased cPOC stocks in 0–7.5 and 7.5–15 cm soil layers (). The cPOC stocks in the 0–7.5 cm soil layer were higher by 22.1–33.8%, with a greater increase in RSC (33.1%) and FYM (33.8%) compared to VC and INM. In the 7.5–15 cm soil depth, the increase in the cPOC stocks ranged between 23.1 and 56.4%, the highest being with FYM, and the other three treatments were similar. The increase in fPOC with reference to NPK at both soil depths was highest in FYM followed by RSC, and the lowest in VC- and INM-treated plots. The increase in fPOC stocks with different treatments ranged from 23.2 to 68.9% in the 0–7.5 cm layer and 28.6 to 100% in the 7.5–15 cm layer. The MinOC, in both soil layers, responded significantly (p < 0.05) to the application of organics and was higher by 21.9–31.1%, 36.2–51.3% and 10.7–32.8% in FYM-, RSC- and VC-amended plots, respectively compared to NPK (2.81–3.16 g kg−1) treatment (). The INM-treated plots showed a small increase (3.4–11.3%) in MinOC over NPK treatment. The unfertilized control invariably resulted in a decline in all the three physical pools of SOC.
Figure 2. Response of coarse particulate (cPOC), fine particulate (fPOC) and mineral-associated organic C (MinOC) in 0–7.5 and 7.5–15 cm soil depths to 10 years management with farmyard manure (FYM), vermicompost (VC), rice straw compost (RSC), integrated use of NPK and FYM (INM) and unamended control compared to fertilizer NPK in rice–wheat system. Vertical line bars indicate standard error. Bars with different letters differ significantly (p < 0.05) by Duncan’s multiple range test.
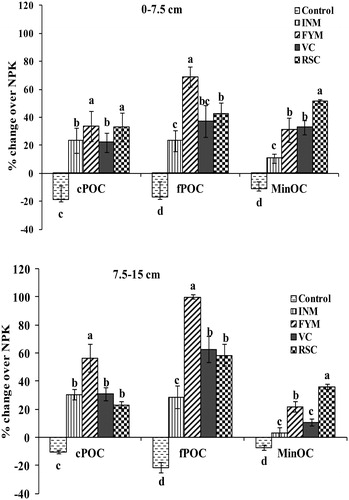
In RSC-amended soils, MinOC constituted the highest proportion (46.2–47.4%) of SOC, followed by fPOC (31.3–31.5%), at 0–7.5 and 7.5–15 cm soil depths (). However, in FYM plots the fPOC comprised the largest proportion (40.5–42.3% of SOC), followed by MinOC (34.4–34.9%). In VC-amended soils, MinOC was the dominant pool (40.9%) at 0–7.5 cm, whereas the fPOC pool was larger (39.4% of SOC) in the 7.5–15 cm layer. In INM plots, MinOC and fPOC accounted for similar proportions of SOC in both soil depths. Irrespective of the treatment, cPOC comprised the smallest pool of SOC. A small amount of C might have been lost while separating cPOM from sand by dry panning; as a result, the sum of cPOC, fPOC and MinOC did not make up 100%.
Figure 3. Influence of 10 years of management with fertilizer NPK, farmyard manure (FYM), vermicompost (VC), rice straw compost (RSC), integrated use of NPK and FYM (INM) and unamended control in a rice–wheat system on distribution of soil organic C among physical pools, viz. coarse particulate (cPOC), fine particulate (fPOC) and mineral-associated organic C (MinOC), as proportion of soil organic C in 0–7.5 and 7.5–15 cm soil depths. Numbers inside the bars labeled with different letters for a given pool indicate significant (p < 0.05) differences between treatments by Duncan’s multiple range test. Vertical line bars show standard error.
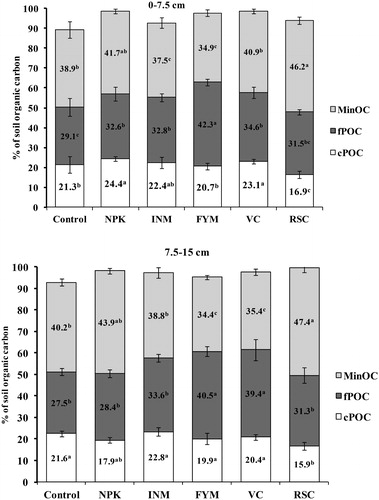
Non-hydrolyzable carbon
Application of organic amendments and INM resulted in significantly (p < 0.05) higher NHC stocks than in the NPK-treated plots at both soil depths (). The increase in NHC stocks over NPK in the 0–7.5 cm soil layer was highest in FYM-amended plots (4.3 t ha−1), followed by RSC (3.5 t ha−1), VC (1.5 t ha−1) and INM (1.4 t ha−1). In the 7.5–15 cm soil layer, the increase in NHC stocks was highest (4.3 t ha−1) with the application of RSC followed by VC (2.9 t ha−1), and lowest in FYM (1.8 t ha−1) treated plots. The increase in NHC stocks with the application of FYM was similar to INM (1.3 t ha−1). In the NPK treatment, the NHC constituted 39.6% of SOC (average of 0–7.5 and 7.5–15 cm soil layers; see ).The NHC as a proportion of SOC improved significantly (p < 0.05) with the application of organic amendments in both soil layers. Among the organic amendments, the proportion of NHC over NPK increased by 40.6, 58.6 and 33.4% (average of 0–7.5 and 7.5–15 cm soil layers) with the application of FYM, RSC and VC. In the INM treatment, the proportion of NHC grew by 25.2% over NPK.
Table 4. Non-hydrolyzable carbon (NHC) in 0–7.5 and 7.5–15 cm soil depths after 10 years in a rice–wheat system in unamended control plots and plots amended with fertilizer NPK, farmyard manure (FYM), vermicompost (VC), rice straw compost (RSC) and NPK plus FYM (INM). Numbers in parentheses indicate standard error.
Carbon mineralization kinetics
Mineralizable C in both soil layers (0–7.5 and 7.5–15 cm) increased significantly (p < 0.05) with the application of organic amendments compared to NPK (). The cumulative amount of C mineralized in 62 days of incubation was significantly (p < 0.05) higher in FYM-amended soils than in RSC and VC soils (). As a proportion of SOC, the amount mineralized ranged from 4.5 to 5.1% in FYM-amended soils, 3.9 to 4.9% in VC-amended soils and 3.5 to 4.3% in RSC-amended soils. The time course of C mineralization followed first-order kinetics as indicated by high R2 (0.995–0.999) and low root mean square error (4.4–8.6 mg kg−1). The carbon mineralization potential (C0) and the associated rate coefficient (k) were highest for FYM- followed by VC- and the lowest for RSC-amended soils (). The C0 of soils (0–7.5 and 7.5–15 cm layers) from different long-term treatments was significantly (p < 0.01) related to different pools of SOC (). Linear regression best described the relationship of C0 with fPOC and cPOC, and a second-degree polynomial best described the relationship between C0 and MinOC ().
Figure 4. Temporal trends of C mineralization in soils drawn from field plots (0–7.5 cm and 7.5–15 cm depth) amended with fertilizer NPK, farm yard manure (FYM), vermicompost (VC), rice straw compost (RSC), integrated use of NPK and FYM (INM) and unamended control for 10 years in a rice–wheat system. Points represent measured C mineralization and lines are fitted to the first-order kinetics model. Vertical line bars on points indicate standard error. Vertical line bars on the X-axis indicate least significant difference (p < 0.05) values for each sampling day.
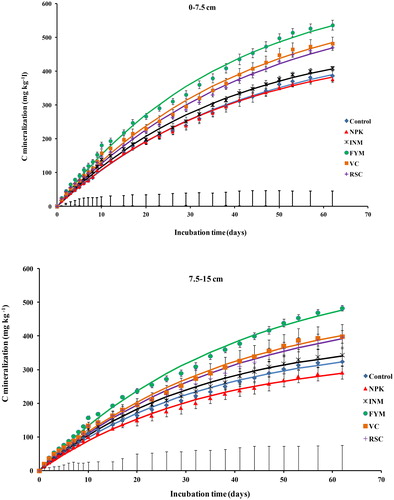
Figure 5. Relationship between C mineralization potential (C0) and coarse particulate (cPOC), fine particulate (fPOC) and mineral-associated organic C (MinOC) in 0–7.5 and 7.5–15 cm soil depths.
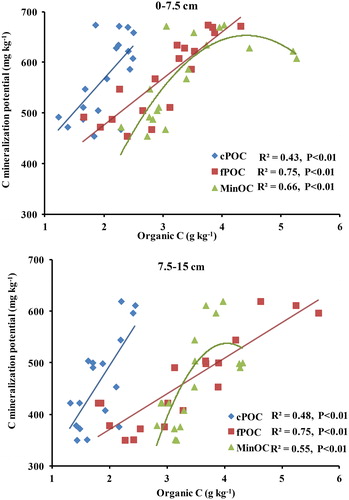
Table 5. Parameter (C0 and k) estimates for the first-order kinetic model (Equation 3) fitted to cumulative carbon mineralization (Cmin) vs. time data for 0–7.5 and 7.5–15 cm soil depths in unamended control and plots amended with fertilizer NPK, farmyard manure (FYM), vermicompost (VC), rice straw compost (RSC) and NPK plus FYM (INM).
Discussion
Carbon accumulation in soil
Consistent with published research, the addition of organic materials resulted in C accumulation in the soil compared with NPK-fertilized plots [Citation34–36]. A meta-analysis of several long-term experiments showed greater C accumulation in organically managed sites compared with those treated with mineral fertilizers [Citation37]. Organic sources improve soil C storage by acting as sources of C [Citation34,Citation38], enhancing crop-mediated C input through greater crop yields [Citation39], and improving macroaggregation and aggregate stability which reduces SOC loss [Citation40–42]. In the present study, organic amendments, besides supplying 5.8–15.2 t C ha−1 yr−1, increased crop-mediated C input by 0.48–1.31 t ha−1 yr−1 compared to NPK-fertilized plots. Differences in SOC accumulation among FYM, RSC and VC managed soils could be attributed to the variation in the amount of C input and the chemical composition of the organic substrates, particularly C/N ratio. Lower C accumulation in VC than FYM and RSC managed plots was due to less C input through VC over the 10-year period and a lower C/N ratio. Microorganisms preferentially use substrates with a low C/N ratio which are decomposed more rapidly than substrates with a higher C/N ratio [Citation26,Citation43]. The effect of the chemical composition of the organic source on C stabilization in soil was also noticeable when comparing FYM and RSC. Farmyard manure, despite supplying a higher amount of C (15.2 t ha−1 yr−1) than RSC (9.5 t ha−1 yr−1), resulted in lower C accumulation (9.1 t ha−1) compared to RSC (12.2 t ha−1), suggesting that the higher lignin/N ratio of RSC had an overriding effect on C stabilization in soil. Earlier studies also showed that application of RSC alone or in combination with mineral fertilizer in a rice–wheat system resulted in greater C sequestration through improved aggregation [Citation41].
Changes in SOC stocks below 15 cm depth (plow layer) indicate that organic amendments led to C accumulation beyond the plow layer. This could be due to puddling (wet tillage) of soil prior to transplanting rice, resulting in mixing of surface soil and organic C with the sub-surface layers. As a result, C sequestration estimates in response to management were higher by 2.1–5.5 t ha−1 when the entire 60-cm profile was considered, compared to the 15-cm profile as is usually done. These results are consistent with earlier reports that showed that addition of organic amendments resulted in SOC accumulation in the sub-surface layers (> 40 cm) [Citation35,Citation44] emphasizing the need to include lower soil depths in sampling plans for quantifying management effects on C accumulation in soil.
Particulate and mineral-associated organic C
Consistent with previous studies [Citation17,Citation18,Citation45], application of organic sources enlarged cPOC and fPOC pools. However, the magnitude of increase varied with the composition of the organic source. Particulate organic C is mainly composed of lightweight, partly decomposed plant and animal fragments [Citation46]. The proportion of POC (sum of cPOC and fPOC) increased from 46.3–56.9% of SOC (NPK) to 60.4–63.1% and 57.7–59.8% with application of FYM and VC, respectively; however, addition of RSC reduced the proportion of POC to 47.2–48.4%. The higher POC in FYM was ascribed to the higher C/N and lower lignin/N ratios compared to RSC with its lower C/N and higher lignin/N ratios. Studies have documented that low-quality (higher C/N and lower lignin/N ratios) C input favors the formation of POC [Citation11,Citation12].
Improvement in MinOC with the application of organic amendments compared with solitary application of NPK, which is in agreement with published research [Citation18,Citation47], could be ascribed to the chemical stabilization of SOM as a result of the formation of new organo-mineral complexes on the previously free mineral surfaces [Citation11]. The higher MinOC in organically amended soil could also be due to higher rhizodeposition than in mineral fertilized plots. Root mucilage plays an important role in the formation of MinOC by secreting hydroxy groups that are very reactive, to form strong organo-mineral complexes [Citation48]. Preferential accumulation of C as MinOC in RSC-amended soils was attributable to its higher N content (1.48%) [Citation49], higher concentration of N-rich compound lignin and the presence of chemically recalcitrant components in the composted material [Citation24,Citation25]. Nano-scale secondary ion mass spectrometry (NanoSIMS) revealed that the addition of N-rich organic substrates favors the formation of new organo-mineral associations on previously organic matter-free mineral surfaces [Citation11,Citation49]. Besides the formation of organo-mineral complexes, the addition of composted materials results in an increased proportion of high-molecular-weight compounds [Citation24] and an increase in aromatic and carboxyl functional groups [Citation50], suggesting the recalcitrant character of the compost-accumulated C in soil. The results suggest that RSC addition not only leads to greater C accumulation in soil but also imparts recalcitrance to the accumulated C.
Non-hydrolyzable C
Besides the formation of organo-mineral stable C compounds, the mechanism of SOM stabilization occurs though the formation of chemically non-biodegradable bonds [Citation48]. The chemical stabilization is mainly attributed to the presence of inherently stable compounds such as lignin and condensed tannins [Citation51]. The increase in NHC with the application of FYM, RSC and VC, over mineral-fertilized plots, could be associated with the recalcitrant nature of C components or the degradation of labile organic components and accrual of recalcitrant compounds such as lignin and condensed tannins during preparation of manure or compost [Citation26,Citation52]. The NHC comprises C complexes formed through condensation and complexation reactions during decomposition over time [Citation53], or through the inherent recalcitrant nature of the C input [Citation54]. Therefore, application of decomposed FYM, RSC and VC improved the hydrophobic alkyl-and aromatic-C contents and consequently the recalcitrance of SOC [Citation26]. The increase in NHC with application of organic amendments is in line with published studies [Citation35,Citation55,Citation56]. The higher NHC in the surface than sub-surface soil is consistent with earlier reports [Citation57,Citation58]; however it contradicts a study on Molisols [Citation35] which was due to an increase in silt content with depth. The greater proportion of NHC in RSC, confirming the recalcitrance of SOC, was ascribed to the higher lignin/N ratio and lower cellulose contents compared with FYM and VC, with a lower lignin/N ratio and higher cellulose contents [Citation59]. The lignin/N ratio has been proposed as a good indicator of SOM stabilization and is used to distinguish between organic substrates resistant to decomposition (high lignin/N ratio) and easily decomposed (low lignin/N ratio) organic substrates [Citation48]. This was further substantiated by the results on mineralizable C in soil.
Carbon mineralization kinetics
Greater C mineralization in soils amended with organic amendments indicates higher microbial activity because of greater substrate availability. The higher C0 and the associated rate coefficient (k) in FYM-amended soils indicate a relatively larger decomposable C pool. The lower Cmin in RSC-amended soils could be attributed to the presence of recalcitrant C components. These results agree with those of Benbi and Yadav [Citation60], who reported that RSC had a lower decomposition rate than FYM. The similar C0 and rate coefficients for RSC and VC could be due to the accumulation of resistant C components in VC other than lignin, such as aromatic components acquired through composting [Citation26]. The linear relationship between C0 and cPOC and fPOC shows the labile character of the POC, whereas the curvilinear relationship with MinOC suggests that beyond a threshold value, MinOC may not significantly influence soil C mineralization. The results are in congruence with previous studies [Citation18,Citation61] showing that short-term C mineralization is positively related to POC. Further, Benbi et al. [Citation18] reported that C mineralization is better related to cPOC than fPOC.
Conclusions
Long-term addition of composted organic matter and FYM in a rice–wheat cropping system improved SOC and its stabilization compared to mineral fertilizer treatment. Carbon stabilization in soil depended on the composition of the organic source brought about by composting. Despite lower exogenous C input through RSC than FYM, the C accumulation in soil was greater with RSC, and the accumulated C was preferentially stored in chemically recalcitrant forms characterized as MinOC and NHC with a lower amount of mineralizable C. The results suggest that composting of rice straw prior to its application has the potential to enhance soil C stabilization, with the co-benefit of protecting the environment.
Acknowledgements
This research was supported by the Indian Council of Agricultural Research (ICAR) National Professor project.
Disclosure statement
No potential conflict of interest was reported by the authors.
References
- IPCC. Intergovernmental plan on climate change-AR5 climate change 2014: summary mitigation of climate change — IPCC; 2014. Available from: https://www.ipcc.ch/report/ar5/wg3/.
- Baldock JA, Skjemstad JO. Role of the soil matrix and minerals in protecting natural organic materials against biological attack. Org Geochem. 2000;31(7–8):697–710. doi:10.1016/S0146-6380(00)00049-8.
- Lal R. Promoting “4 per thousand” and “adapting African agriculture” by south-south cooperation: conservation agriculture and sustainable intensification. Soil Till Res. 2019;188:27–34. doi:10.1016/j.still.2017.12.015.
- Minasny B, Malone BP, McBratney AB, et al. Soil carbon 4 per mille. Geoderma. 2017;292:59–86. doi:10.1016/j.geoderma.2017.01.002.
- Benbi DK, Brar JS. A 25-year record of carbon sequestration and soil properties in intensive agriculture. Agron Sustain Dev. 2009;29(2):257–265. doi:10.1051/agro:2008070.
- Jiang G, Zhang W, Xu M, et al. Manure and mineral fertilizer effects on crop yield and soil carbon sequestration: a meta-analysis and modeling across China. Global Biogeochem Cycles. 2018;32(11):1659–1672. doi:10.1029/2018GB005960.
- Gattinger A, Muller A, Haeni M, et al. Enhanced top soil carbon stocks under organic farming. Proc Natl Acad Sci USA. 2012;109(44):18226–18231. doi:10.1073/pnas.1209429109.
- Ågren GI, Bosatta E. Reconciling differences in predictions of temperature response of soil organic matter. Soil Biol Biochem. 2002;34(1):129–132. doi:10.1016/S0038-0717(01)00156-0.
- Dungait JAJ, Hopkins DW, Gregory AS, et al. Soil organic matter turnover is governed by accessibility not recalcitrance. Glob Change Biol. 2012;18(6):1781–1796. doi:10.1111/j.1365-2486.2012.02665.x.
- Lehmann J, Kleber M. The contentious nature of soil organic matter. Nature. 2015;528(7580):60–68. doi:10.1038/nature16069.
- Kopittke PM, Dalal RC, Hoeschen C, et al. Soil organic matter is stabilized by organo-mineral associations through two key processes: the role of the carbon to nitrogen ratio. Geoderma. 2020;357:113974. doi:10.1016/j.geoderma.2019.113974.
- Cotrufo MF, Wallenstein MD, Boot CM, Denef K, et al. The microbial efficiency-matrix stabilization (MEMS) framework integrates plant litter decomposition with soil organic matter stabilization: do labile plant inputs form stable soil organic matter? Glob Chang Biol. 2013;19(4):988–995. doi:10.1111/gcb.12113.
- Benbi DK, Khosa MK. Effects of temperature, moisture, and chemical composition of organic substrates on C mineralization in soils. Commun Soil Sci Plant Anal. 2014;45(21):2734–2753. doi:10.1080/00103624.2014.950423.
- Schmidt MWI, Torn MS, Abiven S, et al. Persistence of soil organic matter as an ecosystem property. Nature. 2011;478(7367):49–56. doi:10.1038/nature10386.
- Berti A, Morari F, Dal Ferro N, et al. Organic input quality is more important than its quantity: C turnover coefficients in different cropping systems. Eur J Agron. 2016;77:138–145. doi:10.1016/j.eja.2016.03.005.
- Anantha KC, Majumder SP, Badole S, et al. Pools of organic carbon in soils under a long-term rice–rice system with different organic amendments in hot, sub-humid India. Carbon Manage. 2020;11(4):331–339. doi:10.1080/17583004.2020.1783624.
- Benbi DK, Singh P, Toor AS, et al. Manure and fertilizer application effects on aggregate and mineral-associated organic carbon in a loamy soil under rice-wheat system. Commun Soil Sci Plant Anal. 2016;47(15):1828–1844. doi:10.1080/00103624.2016.1208757.
- Benbi DK, Toor AS, Kumar S. Management of organic amendments in rice-wheat cropping system determines the pool where carbon is sequestered. Plant Soil. 2012;360(1–2):145–162. doi:10.1007/s11104-012-1226-3.
- Bhatt R, Kukal SS, Busari MA, et al. Sustainability issues on rice–wheat cropping system. Int Soil Water Conserv Res. 2016;4(1):64–74. doi:10.1016/j.iswcr.2015.12.001.
- Government of India-Ministry of Agriculture. Punjab farmers guide. Mechanisation & Technology Division, Department of Agriculture & Cooperation Government of India. 2018. http://farmech.dac.gov.in/FarmerGuide/PB/index1.html.
- Ministry of Agriculture-Farmers Welfare. Promotion of agricultural mechanisation for in-situ management of crop residue in states of Punjab, Haryana, Uttar Pradesh and NCT of Delhi. 2019.
- Benbi DK. Carbon footprint and agricultural sustainability nexus in an intensively cultivated region of Indo-Gangetic Plains. Sci Total Environ. 2018;644:611–623. doi:10.1016/j.scitotenv.2018.07.018.
- Thind HS, Sharma S, Singh Y, et al. Rice–wheat productivity and profitability with residue, tillage and green manure management. Nutr Cycl Agroecosyst. 2019;113(2):113–125. doi:10.1007/s10705-018-09967-8.
- Silva JR, Silva DJ, Gava CAT, et al. Carbon in humic fractions of organic matter in soil treated with organic composts under mango cultivation. Revista Brasileira de Ciencia Do Solo. 2016;40:e0150095. doi:10.1590/18069657rbcs20150095.
- Spaccini R, Piccolo A. Soil organic carbon stabilization in compost amended soils. In: Global symposium on soil organic carbon, March 21–23. Vol. 2. Rome Italy: FAO, 2017.
- Leifeld J, Siebert S, Kögel-Knabner I. Kögel-Knabner I. Changes in the chemical composition of soil organic matter after application of compost. Eur J Soil Sci. 2002;53(2):299–309. doi:10.1046/j.1351-0754.2002.00453.x.
- Ngo PT, Rumpel C, Doan TT, et al. Effect of earthworms on carbon storage and soil organic matter composition in tropical soil amended with compost and vermicompost. Soil Biol Biochem. 2012;50:214–220. doi:10.1016/j.soilbio.2012.02.037.
- Kumar K, Prihar SS, Gajri PR. Determination of root distribution of wheat by auger sampling. Plant Soil. 1993;149(2):245–253. doi:10.1007/BF00016615.
- Hirte J, Leifeld J, Abiven S, et al. Below ground carbon inputs to soil via root biomass and rhizodeposition of field-grown maize and wheat at harvest are independent of net primary productivity. Agric Ecosyst Environ. 2018;265:556–566. doi:10.1016/j.agee.2018.07.010.
- Goering HK, Van Soest P. Forage fiber analyses: (apparatus, reagents, procedures, and some applications). Washington, DC: Agricultural Research Service, U.S. Dept. of Agriculture; 1970. Available from: https://catalyst.library.jhu.edu/catalog/bib_4172452.
- Jackson M. Soil chemical analysis. Englewood Cliffs, NJ: Prentice-Hall, INC; 1964.
- Silveira ML, Comerford NB, Reddy KR, et al. Characterization of soil organic carbon pools by acid hydrolysis. Geoderma. 2008;144(1–2):405–414. doi:10.1016/j.geoderma.2008.01.002.
- Benbi DK, Richter J. A critical review of some approaches to modelling nitrogen mineralization. Biol Fertil Soils. 2002;35(3):168–183. doi:10.1007/s00374-002-0456-6.
- Benbi DK, Brar K, Toor AS, et al. Sensitivity of labile soil organic carbon pools to long-term fertilizer, straw and manure management in rice-wheat system. Pedosphere. 2015;25(4):534–545. doi:10.1016/S1002-0160(15)30034-5.
- Abrar MM, Xu M, Shah SAA, et al. Variations in the profile distribution and protection mechanisms of organic carbon under long-term fertilization in a Chinese Mollisol. Sci Total Environ. 2020; 723:138181. doi:10.1016/j.scitotenv.2020.138181.
- Singh P, Benbi DK. Nutrient management effects on organic carbon pools in a sandy loam soil under rice-wheat cropping. Arch Agron Soil Sci. 2018;64(13):1879–1891. doi:10.1080/03650340.2018.1465564.
- Dai S, Wang J, Cheng Y, et al. Effects of long-term fertilization on soil gross N transformation rates and their implications. J Integr Agric. 2017;16(12):2863–2870. doi:10.1016/S2095-3119(17)61673-3.
- Malhi SS, Nyborg M, Solberg ED, et al. Long-term straw management and N fertilizer rate effects on quantity and quality of organic C and N and some chemical properties in two contrasting soils in Western Canada. Biol Fertil Soils. 2011;47(7):785–800. doi:10.1007/s00374-011-0587-8.
- Seufert V, Ramankutty N, Foley JA. Comparing the yields of organic and conventional agriculture. Nature. 2012;485(7397):229–232. doi:10.1038/nature11069.
- Six J, Paustian K. Aggregate-associated soil organic matter as an ecosystem property and a measurement tool. Soil Biol Biochem. 2014;68:A4–A9. doi:10.1016/j.soilbio.2013.06.014.
- Sodhi GPS, Beri V, Benbi DK. Soil aggregation and distribution of carbon and nitrogen in different fractions under long-term application of compost in rice-wheat system. Soil till Res. 2009;103(2):412–418. doi:10.1016/j.still.2008.12.005.
- Benbi DK, Senapati N. Soil aggregation and carbon and nitrogen stabilization in relation to residue and manure application in rice-wheat systems in northwest India. Nutr Cycl Agroecosyst. 2010;87(2):233–247. doi:10.1007/s10705-009-9331-2.
- Dignac MF, Houot S, Francou C, et al. Pyrolytic study of compost and waste organic matter. Org Geochem. 2005;36(7):1054–1071. doi:10.1016/j.orggeochem.2005.02.007.
- Bell LW, Sparling B, Tenuta M, et al. Soil profile carbon and nutrient stocks under long-term conventional and organic crop and alfalfa-crop rotations and re-established grassland. Agric Ecosyst Environ. 2012;158:156–163. doi:10.1016/j.agee.2012.06.006.
- Chai Y, Ma S, Zeng X, et al. Long-term fertilization effects on soil organic carbon stocks in the irrigated desert soil of NW China. J Plant Nutr Soil Sci. 2015;178(4):622–630. doi:10.1002/jpln.201400172.
- Christensen BT. Physical fractionation of soil and structural and functional complexity in organic matter turnover. Eur J Soil Sci. 2001;52(3):345–353. doi:10.1046/j.1365-2389.2001.00417.x.
- Tong X, Xu M, Wang X, et al. Long-term fertilization effects on organic carbon fractions in a red soil of China. Catena. 2014;113:251–259. doi:10.1016/j.catena.2013.08.005.
- Rasse DP, Rumpel C, Dignac MF. Is soil carbon mostly root carbon? Mechanisms for a specific stabilisation. Plant Soil. 2005;269(1-2):341–356. doi:10.1007/s11104-004-0907-y.
- Kopittke PM, Hernandez-Soriano MC, Dalal RC, et al. Nitrogen-rich microbial products provide new organo-mineral associations for the stabilization of soil organic matter. Glob Chang Biol. 2018;24(4):1762–1770. doi:10.1111/gcb.14009.
- Jindo K, Chocano C, Melgares de Aguilar J, et al. Impact of compost application during 5 years on crop production, soil microbial activity, carbon fraction, and humification process. Commun Soil Sci Plant Anal. 2016;47(16):1907–1919. doi:10.1080/00103624.2016.1206922.
- Tegelaar EW, De Leeuw JW, Holloway PJ. Some mechanisms of flash pyrolysis of naturally occurring higher plant polyesters. J Anal Appl Pyrolysis. 1989;15(C):289–295. doi:10.1016/0165-2370(89)85041-7.
- Chefetz B, Hatcher PG, Hadar Y, et al. Chemical and biological characterization of organic matter during composting of municipal solid waste. J Environ Qual. 1996;25(4):776–785. doi:10.2134/jeq1996.00472425002500040018x.
- Kögel-Knabner I. Analytical approaches for characterizing soil organic matter. Org Geochem. 2000;31(7–8):609–625. doi:10.1016/S0146-6380(00)00042-5.
- Yan D, Wang D, Yang L. Long-term effect of chemical fertilizer, straw, and manure on labile organic matter fractions in a paddy soil. Biol Fertil Soils. 2007;44(1):93–101. doi:10.1007/s00374-007-0183-0.
- Tian J, Lou Y, Gao Y, et al. Response of soil organic matter fractions and composition of microbial community to long-term organic and mineral fertilization. Biol Fertil Soils. 2017;53(5):523–532. doi:10.1007/s00374-017-1189-x.
- Jha P, Lakaria BL, Biswas AK, et al. Effects of carbon input on soil carbon stability and nitrogen dynamics. Agric Ecosyst Environ. 2014;189:36–42. doi:10.1016/j.agee.2014.03.019.
- Paul EA, Collins HP, Leavitt SW. Dynamics of resistant soil carbon of midwestern agricultural soils measured by naturally occurring 14C abundance. Geoderma. 2001;104(3–4):239–256. doi:10.1016/S0016-7061(01)00083-0.
- Schwendenmann L, Pendall E. Response of soil organic matter dynamics to conversion from tropical forest to grassland as determined by long-term incubation. Biol Fert Soils. 2008;44(8):1053–1062. doi:10.1007/s00374-008-0294-2.
- Skjemstad JO, Le Feuvre RP, Prebble RE. Turnover of soil organic matter under pasture as determined by 13C natural abundance. Soil Res. 1990;28(2):267–276. doi:10.1071/SR9900267.
- Benbi DK, Yadav SK. decomposition and carbon sequestration potential of different rice-residue-derived by-products and farmyard manure in a sandy loam soil. Commun Soil Sci Plant Anal. 2015;46(17):2201–2211. doi:10.1080/00103624.2015.1069322.
- Fließbach A, Mäder P. Microbial biomass and size-density fractions differ between soils of organic and conventional agricultural systems. Soil Biol Biochem. 2000;32(6):757–768. doi:10.1016/S0038-0717(99)00197-2.