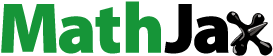
ABSTRACT
Crop residue burning is a major issue in farmers’ fields of Indo-Gangetic Plain and Central India. It emits air pollutants and GHGs into the atmosphere, adversely affects soil, crop yields, human health and the environment. Weed infestation is also a major problem and affects overall crop yield. Therefore, a 2-year farm experiment was conducted at farmers’ fields with three treatments – current farmers’ practice (FP), conservation agriculture with improved weed management (CAW) and conservation agriculture without any weed management (CA) – from kharif (monsoon) 2017 to summer 2019 to manage crop residue and weeds. A happy seeder and tractor-operated boom sprayer were also introduced in CA and CAW. Total 51 t of crop residue was generated from 2 ha of rice-wheat-greengram cultivation area. In FP this crop residue was burned, but it was utilized as mulch in CA and CAW, thus, emission of 34,400 kg CO2e was avoided and energy potential of 100.1 × 104 MJ was created. The CAW produced percent higher crop yield of 21, 22.5 and 44.4%in first year and 20.6, 20 and 30% in second year of crop cycle respectively in rice (4.6 & 4.1 t ha−1), wheat (4.9 & 4.1 t ha−1) and greengram (1.3 & 1.3 t ha−1).
Introduction
The rice–wheat–greengram system (RWGS) is a dominant cropping system in the Indo-Gangetic Plain of India, cultivated over an area of about 10 million hectares [Citation1,Citation2]. It also occupies a considerable area (1.064 M ha) of Central India, particularly in the central and eastern parts of Madhya Pradesh [Citation2]. Farmers’ practices for crop cultivation in this region of Madhya Pradesh include extensive conventional tillage, removal or burning of crop residues, limited use of organic manure, fixed rotations of the crops, and injudicious or unscientific use of fertilizers and other agrochemicals [Citation3]. In recent years, the mechanization of agricultural activities has increased. In Northwestern India about 91% and 82% of rice and wheat crops, respectively, were harvested using combine harvesters [Citation4], leaving the residues in the field. The same trend has been followed in other parts of India, such as the central Vertisols [Citation2,Citation3], further; combine harvesters are also being extensively used to harvest pulse crops. Parts of wheat residue and whole amounts of greengram residue are collected for animal feed and other domestic purposes. However, rice straw is left on the field as it makes a poor animal feed due to its high silica content [Citation5]. Therefore, leftover residues create problems during field preparation and for the timely sowing of wheat [Citation3]. Madhya Pradesh alone generates about 33.18 Mt of residues from various crops, of which 2.0–3.86 Mt is burned annually [Citation6]. Mostly, farmers feel that burning of crop residues is the easiest method of residue management and field preparation for timely sowing of succeeding crops, and do not realize its adverse impacts on soil, environment and human health. The practice of residue burning has increased with the introduction of combine harvesters [Citation4,Citation5,Citation7,Citation8].
Agricultural crop residue burning contributes to the GHGs – carbon dioxide (CO2), nitrous oxide (N2O) and methane (CH4) – causing global warming [Citation9,Citation10]. It also contributes air pollutants –namely, carbon monoxide (CO), oxides of sulfur (SOx), oxides of nitrogen (NOx), ammonia (NH3), non-methane volatile organic compounds (NMVOCs), polycyclic aromatic hydrocarbons (PAHs), elemental carbon (EC), organic carbon (OC) and particulate matter (PM10 and PM2.5) and smoke –causing air pollution and thereby causing threats to human health [Citation9,Citation11–13]. To reduce the GHG emissions from the agricultural sector, various solutions are available [Citation14]. These solutions involve minimizing the use of fossil fuels, minimum tillage operations and management [Citation15], conservational agriculture [Citation16], alternative nitrogen management [Citation17–19], utilization of crop residue as mulch, etc. [Citation20].
In RWGS, farmers generally prepare land for sowing following the burning of previous crop residue using 3–6 energy intensive tillage operations by investing 10–15 days under conventional tillage practice. This period is most valuable during the sowing period in the post-rainy season under Indian agriculture. In the Vertisols of central India, seed bed preparation for crop sowing in winter and summer crops poses serious problem and consumes a considerable amount of time, energy and farm inputs [Citation21]. Conventional tillage in the region was done using plows, cultivators, disc harrows and rotavators, which added USD 42.18–56.25 ha−1 to operational costs [Citation3]. Crop residue burning along with the conventional tillage practices led to a reduction in crop yield, factor productivity and sustainability of the system [Citation2,Citation22].
With the onset of the green revolution in India, agriculture became an energy-intensive production system [Citation23]; thus, for efficient use of agricultural inputs [Citation24] and effective management of crop residues, conservation agriculture is a better option [Citation25–27]. Conservation agriculture is based on three principles: minimum tillage, crop rotation and use of crop residue as mulching [Citation28]. Its use improves crop productivity, increases carbon sequestration, and reduces GHG emissions [Citation29]. Previous studies reported that cultivation of RWGS or maize–wheat–greengram system (MWGS) by adopting zero-tillage (ZT; a principal concept of conservation agriculture) reduced the global warming potential (GWP) by 15–30% over crop cultivation under conventional tillage (CT) practices [Citation30]. The CT system had 17.4 and 18.1% higher GWP compared to zero-tilled permanent beds and the ZT system when MWGS, maize–chickpea–sesbania, maize–mustard–greengram and maize–maize–sesbania systems were cultivated under CT [Citation31].
Conservation agriculture is a mechanized system in which most farm operations are performed by high-precision, advanced farm machinies such as zero-till seed drills, slit drillss, happy seeder, tractor-operated sprayers, combine harvesters, and super straw management systems (SMSs), etc. The farm machinery under ZT operations consumes 58 and 81% less fuel compared to the CT system for cultivating pigeonpea and castor; thus, a reduction in GHG emissions of 21% and 23%, respectively, was observed in ZT-based crop cultivation [Citation32,Citation33]. Recently, the happy seeder has become popular for sowing of crops in the Indo-Gangetic Plain and Central India. Crop cultivation using a happy seeder is 50 to 60% cheaper than CT [Citation4,Citation5,Citation7]. At the same time, the grain yield of wheat is statistically on par with that under CT [Citation8]. Additionally, using a happy seeder for farm activities reduces GHG emissions by more than 78% by avoiding crop residue burning [Citation16]. Therefore, crop cultivation with a happy seeder is a better option to reduce GHG emissions and air pollutants.
In addition to residue management, weeds are an issue, as weeds compete with agricultural crops for nutrients and water, causing biotic stress. In India, weeds alone cause a 37% loss in crop production, equivalent to about 11 billion USD, from 10 major crops [Citation34,Citation35]. Furthermore, weed management is one of the costliest operations, accounting for nearly 25% of the total labor requirements in crop production [Citation36]. The average cost of weed control is about USD 84.35 ha−1 in kharif (monsoon) season and USD 56.24 ha−1 for rabi (winter) season crops, contributing 33% and 22%, respectively, to the total cost of cultivation [Citation37,Citation38]. Traditionally, farmers have done manual weeding using khurpi or sickles. Weeding with these tools must be performed in a bending/squatting posture that consumes 30–50% more energy than standing or sitting does [Citation39–41]. Farmers are losing 15–20% crop yield due to this traditional manual weeding [Citation34]. Nearly one third of the cost of cultivation can be saved and crop yield can be increased by adopting mechanized weed management using wheel hoes, mechanical power weeders, effective application of herbicides though sprayers, etc. [Citation42,Citation43].
Given this, it was hypothesized that conservation agriculture with proper weed management practices can solve the problem of residue burning in the region, while producing higher crop yields and lower GHG emissions. Therefore, this study was undertaken with the objective of managing the crop residue, enhancing crop yields and minimizing GHG emissions through conservation agriculture and mechanized farming in RWGS in farmers’ fields on the central Vertisol of India.
Materials and methods
Study site selection
Five villages were selected – Pondi, Pauniya, Khaira, Boriya and Ramkhiriya of Patan block, district Jabalpur, Madhya Pradesh (MP) – to conduct on-farm research (OFR) trials for Indian Council of Agricultural Research (ICAR) – Directorate of Weed Research (DWR), Jabalpur (). These villages were selected on the basis of cultivation practices, availability of farm machinery, current crop residue management, and awareness of the ill effects caused by residue burning. The soils of the experimental villages are clay loam in texture and the percent clay value ranged from 26 to 30% with low soil organic carbon content (0.52–0.63%). The soil at 0–15 cm depth has a bulk density of ∼1.3 gcm−3 and a pH of 7.6 (1:2 soil/water). The climate of the region is humid subtropical. The average annual rainfall is about 1390 mm, 80% of which occurs during June to September (monsoon season). The annual rate of evaporation is about 1502 mm, which is higher than the annual rainfall.
Figure 1. Base map of farm trial sites (Source: Google Maps [Citation44]).
![Figure 1. Base map of farm trial sites (Source: Google Maps [Citation44]).](/cms/asset/31cc99ed-8cd0-42d9-8043-f052ff5b71ac/tcmt_a_1835387_f0001_c.jpg)
Experiment details and residue management
Two years of OFR trials were conducted under RWGS during 2017–2018 and 2018–2019 in farmers’ fields. The trials were conducted in an area of about 0.25 ha (4000 m2) at each location. The field experiment consisted of three treatments, namely (1) current farmers’ practice (FP); (2) conservation agriculture with recommended herbicide application (CAW); and (3) conservation agriculture without any weed management practices (CA). The details of the experimental treatments are given in . To improve the spraying efficiency and spray uniformity, a standard flat-fan nozzle with tractor boom sprayer was used under CAW. A survey was conducted in farmers’ fields in selected villages at the beginning of each cropping season to record the amount of crop residue left for burning to prepare the land for the succeeding crop. A quadrat (2 × 5 m2) was placed randomly in the field to record the residues of previous crops left for burning.
Table 1. Details of experimental treatments.
The happy seeder includes a rotary flail and inverted T-type furrow opener system, where the rotary flail only cuts and clears the standing and loose stubble present in front of the furrow opener without disturbing the soil, and the furrow opener opens a narrow slit in the soil for seed and fertilizer placement. The remaining portion of the crop residue present elsewhere in the field was untouched and left as such to act as mulch.
Estimation of energy potential and GHG emissions
To estimate the energy potential, emissions of GHGs and air pollutants from crop residue burning under FP, various parameters were used, which are described in .
Table 2. Coefficients used for preparation of emissions inventory from crop residue burning.
Table 3. Emission coefficients for residue burning of various crops.
Table 4. Lower heating value of various crop residues.
GHGs were estimated using EquationEquations (1)(1)
(1) and Equation(2)
(2)
(2) [Citation9,Citation13,Citation45,Citation46]:
(1)
(1) where TMCRB = total mass of crop residue burned in situ, in tonnes (t); and CSEF = crop-specific emission factor, in g kg−1[Citation13].
The crop-specific production-to-residue ratio and dry matter-to-crop ratio was used to estimate the total crop residue generated [Citation9]:(2)
(2) where CP = the crop production during a specific year; R = the ratio of crop-specific production to residue; D = the ratio of dry matter to crop; E = the burning efficiency; and B = the percentage of dry matter residue burned in the fields (%).
The coefficients of various parameters used in EquationEquations (1)(1)
(1) and Equation(2)
(2)
(2) are given in and . According to the IPCC, 2006 [Citation46] a value of 25% was taken as the fraction of crop residues burned. The value for rice varies from 8 to 80% across the various states in India; however, a fraction of 0.8 was taken as the best estimate in the region [Citation9]. The CO2 equivalent (CO2e) GHG emission, in Mg, was estimated using EquationEquation (3)
(3)
(3) . GWP values of 25 and 298 for CH4 and N2O, respectively, were used [Citation13,Citation53].
(3)
(3) where EGHG is the total CO2 equivalent GHG emissions (Mg); ECH4 and EN2O are the emissions of CH4 and N2O; and GWPCH4 and GWPN2O are the global warming potentials of CH4 and N2O, respectively.
The theoretical energy potential (TEP) was estimated using EquationEquation (4)(4)
(4) . It is the product of the lower heating value (LHV) of each crop and the amount of residue correspondingly generated.
(4)
(4)
The LHV values (in MJkg−1) used for various crop residues are listed in .
Crop performance and weed management
The rice (Oryza sativa) cultivar Kranti in FP and CA, wheat (Triticum aestivum L.) cultivar JW2851 in FP and GW-273 in CA and greengram (Vigna radiata L.) local variety in FP and cultivar Samrat in CA and CAW were used in this study. Rice seed was dry-seeded at 125 kg ha−1 in FP and 50 kg ha−1 in CA and CAW. Rice was sown during the last week of June and harvested in the last week of October during both years. Sowing of wheat seed was done by direct drilling at 250 kg ha−1 in FP and at 100 kg ha−1 under CA and CAW. Wheat was sown in mid-November and harvested during the first week of March in both years. Greengram was sown by direct drilling at 50 kg ha−1 in FP and 25 kg ha−1 in CA and CAW during the last week of March and harvested in mid-June. The recommended doses of fertilizers were maintained in all treatments. The weed management practices followed under different crops and tillage practices is given in . The weed density and weed dry biomass were measured at 60 days after sowing (DAS) using a quadrat with an area of 0.25 m2. Data on grain yield was recorded using quadrat (2 × 5 m2) randomly placed at three different locations within the experimental field and converted to a hectare basis. The weed control efficiency (WCE) was calculated using EquationEquation (5)(5)
(5) [Citation54]:
(5)
(5) where WDC and WDT are weed dry weight in control and herbicide-treated plots, respectively. Similarly, the yield attributes of each treatment were worked out based on the standard protocol.
Statistical analysis
The data on actual weed density and dry weight was transformed by square root transformation ), due to high variance, for statistical analysis. The statistical analysis of variance (ANOVA) of data was done using the ICAR-Indian Agricultural Statistics Research Institute (IASRI), New Delhi, online statistical portal (https://iasri.icar.gov.in/online-analysis-of-data/). Treatment means were separated with the use of Tukey’s honest significant difference test at the 5% level of significance.
Results and discussion
Crop residue generation and management
The results showed that farmers from study villages were not well aware of the adverse impacts of residue burning. Farmers have not adopted farm chinoiseries for crop cultivation and are using old machinery. The generated crop residues left for burning in famers’ fields are listed in . The maximum load of about 6.7 t ha−1 left for burning was seen in rice residue, followed by wheat (5.0 t ha−1). The whole amount of rice residue was left to burn was because of its high silica content and lower economic value, whereas about 40% of wheat residue was used for animal feeding and other domestic purposes [Citation5]. During the study period (2017–2019), about 51 t of total crop residue was generated from 2 ha of RWGS-cultivated land at farmers’ fields, of which 25.4 t was from rice followed by 20.0 t from wheat and 5.6 t from greengram. The indicated rice and wheat crop residue amounts were burned to prepare the land for the succeeding crops, whereas the whole amount of greengram crop residue was used for animal feed and/or mixed with the soil during tillage because of its easy decomposition. The burned crop residues lead to emission of GHGs and air pollutants, and loss of organic matter and nutrients [Citation4,Citation5,Citation7]. It was observed that the major reasons behind residue burning in farmers’ fields were their lack of knowledge about the adverse effects caused by residue burning and the non-availability of advanced residue-managing machinery.
Table 5. Maximum crop residue load recorded in farmers’ fields before straw burning.
In CA and CAW treatments, to manage leftover crop residue and sowing operations, the happy seeder, an advanced and improved residue management-cum-seed sowing machine, was used. The happy seeder requires straw to be uniformly spread in the field before sowing, which was done using an SMS along with a combine harvester. The 51 t of crop residue generated in the farmers’ fields was effectively utilized as crop mulch instead of being burned as in the FP treatments. Thus, emission of GHGs and air pollutants and nutrient losses through crop residue burning were eliminated. In addition to this eliminated crop residue burning, the crop mulch enhanced the soil organic carbon content and suppressed weed infestation [Citation55].
Estimation of energy potential and GHG emissions
The estimation of GHG and air pollutant emissions was carried out on the basis of actual crop cultivated area (i.e. 2 ha crop−1 yr−1). Later, this estimation could be extrapolated to the village, block, district or other level to determine the emission rate of GHGs and air pollutants. The estimated results are given in . The table shows that a total of 27,008.05 kg CO2, 11.07 kg N2O and 164.9 kg CH4 was released due to burning of rice and wheat residues during the 2-year study period (i.e. from 2017 to 2019). The GWP of these GHGs was 34,429.5 kg CO2e. The total amount of air pollutants released from rice and wheat crop residue burning was 2216.61 kg. In total, 165.4 kg PM2.5, 168.15 kg PM10, 4.77 kg SO2, 1566.9 kg CO, 43.49 kg NOX, 69.41 kg NH3, 142.45 kg NMVOC, 8.63 kg EC, 47.32 kg OC and 0.1 kg PAH were released. These results show that the FP currently used in the region is not environmentally friendly, necessitating an immediate intervention for effective crop residue management. Therefore, relative to FP, a happy seeder combined with SMS in CA and CAW can be used to avoid the emission of the abovementioned GHGs and air pollutants.
Table 6. Emissions of greenhouse gas and air pollutants from burning of rice and wheat crop residues in farmers’ fields (values are sums of residues from five farmers).
The TEP values for residue burning are shown in . A total energy of 100.1 × 104 MJ can be generated using the crop residues generated in the farmers’ fields. This value for TEP is from only 2 ha of cultivated area. If the total residue generated from the village was used, this would be a much greater source of energy production, and different modes of energy production units such as thermal, electrical, etc. could be developed at the village level. For the collection of crop residues, advanced machines such as straw-balers, straw combines and others can be used.
Crop performance and weed management
Rice
The weed flora observed in direct seeded rice (DSR) comprised jungle rice (Echinochloa colona), barnyard grass (Echinochloa crus-galli), viper grass (Dinebra retroflexa), variable flatsedge (Cyperus difformis), ricefield flatsedge (Cyperus iria), sunberry (Physalis minima), smooth joyweed (Alternanthera paronychioides), false daisy (Eclipta alba) and blistering ammannia (Ammania baccifera). The dominant weed species were jungle rice (Echinochloa colona), and smooth joyweed (Alternanthera paronychioides). The dominance of grassy weeds can be attributed to simultaneous germination of these species along with rice seeds under favorable soil moisture and temperature. These results agree with the findings of Saini and Angiras [Citation56] and Singh et al. [Citation57], who reported the dominance of grassy weeds over other species in DSR. The particular tillage operations followed, the burning of crop residue instead of keeping it as mulch, and the improper herbicide application practices followed under FP lead to less control over major weeds such as viper grass (Dinebra retroflexa) and jungle rice (Echinochloa colona). The maximum density and dry biomass of weeds were recorded in the un-weeded condition, whereas different weed management practices significantly diminished the total weed density and dry biomass in DSR [Citation3] (; ).
Figure 3. Two year pooled weed density and weed dry biomass under various crops.
FP - Farmer Practice; CAW - Conservation agriculture with improved weed management practices; CA - Conservation agriculture without any weed management practices; no. - number; g - gram; sq. m - square meter
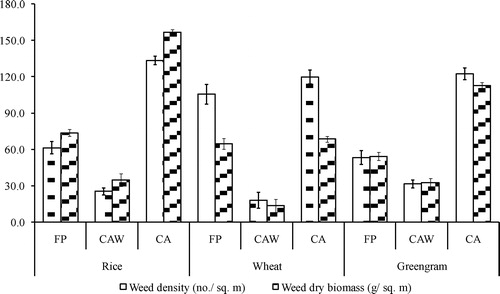
Table 7. Weed parameters observed under different treatments.
Among different tillage-cum-weed management methods, CAW i.e. recommended fertilizer dose (RFD) + pyrazosulfuron 25 g fb bispyribac-Na 25 g ha−1 produced the maximum rice grain yield of 4.6 and 4.1 t ha−1 during 2017 and 2018, respectively (). The lowest rice grain yield was recorded in CA (i.e. un-weeded plots). Even though crop mulch was maintained, the probable accumulation of a weed seed bank in the top layer of the soil leads to more weed flushes. Thus, under this weedy environment crops face severe competition for water, nutrients, space and solar radiation, which results in much lower yields. The application of pyrazosulfuron 25 g fb bispyribac-Na 25 g ha−1 effectively reduced the weed growth with its 77.7% WCE, whereas weed management practices followed under FP produced only 53% WCE ().
Figure 4. Weed control efficiency (WCE) of recommended herbicides in various crops.
FP - Farmer Practice; CAW - Conservation agriculture with improved weed management practices; WCE - Weed control efficiency
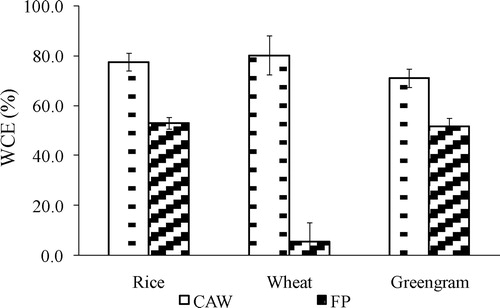
Table 8. Crop yield parameters under different treatments.
Wheat
The weed flora observed in the wheat crop were yellow pea (Lathyrus aphaca), common vetch (Vicia sativa), common lambsquarter (Chenopodium album), rough medik (Medicago polymorpha) and white sweet clover (Melilotus alba) among broad-leaved and wild oat (Avena ludoviciana) and little seed canary grass (Phalaris minor) among grasses. The grassy weeds (Avena ludoviciana and Phalaris minor) and rough medik (Medicago polymorpha) among broad leaved weeds were the most dominant weed species. A significant difference was observed between CAW and FP in terms of weed control, grain and straw yield. However, no significant difference was observed between CA and FP. The two treatments produced the same results ( and ; ). The highest grain yields of 4.9 and 4.8 t ha−1 were observed in the CAW treatment with the lowest weed density (17.2 and 18.9 no. m−2) and weed dry biomass (12.7 and 14.2 g m−2) during the winter season of 2017–2018 and 2018–2019. The combination effect of crop residue and improved weed management practices suppresses the weeds effectively [Citation58]. The application of clodinofop + metsulfuron 60 + 4 g ai ha−1 significantly reduced weed growth, with a WCE of 80.4% [Citation3], whereas the application of 2,4-D, 1 kg ai ha−1 at 35 DAS under FP produced only 5.6% WCE. This shows that the weed management practices followed by farmers at present are not effective. This is because farmers are using faulty spraying techniques with the wrong nozzle combination, not using proper safety measures and not practicing the proper spray path. All of this results in non-target deposition of herbicides, escaping of weeds from herbicide application, repeated and erratic application of herbicides and poor control of weeds. These problems were addressed in CAW treatments by using advanced machinery.
Greengram
The weed flora in greengram was knot grass (Paspalidium distichum), wild poinsettia (Euphorbia geniculata), stonebreaker (Phyllanthus niruri), obscure morning glory (Ipomea obscura), goosegrass (Eleusine indica), spreading dayflower (Cyanotis axillaris), purple nutsedge (Cyperus rotundus) and congress grass (Parthenium hysterophorus). Lower germination and growth of the weeds were observed in CAW compared to FP (; ). The weed seed germination, growth and dry biomass were effectively diminished by the CAW practice, thus producing 1.3 t ha−1 of grain during the study period [Citation3]. The second plus weed appearance and heavy weed infestation following one hand weeding under FP produced lower grain yields (0.9 and 1.0 t ha−1 during 2018 and 2019) compared to CAW. However, the lowest yield of 0.5 t ha−1 was observed in CA, in which weed management operations were not practiced at al. The application of imazethapyr 100 g ha−1 significantly controlled the weeds, with a WCE of 71.2%.
On the whole, the wrong nozzle combination and un-mechanized herbicide spraying operations under FP yield non-uniform application of herbicides, poor control of weeds and inefficacy of the spraying systems to control weeds. These effects can be clearly seen in , which shows the yield reduction in FP over CAW. Thus, mechanized operation with improved weed management practices should be followed to obtain higher grain yield and profitability.
Figure 5. Percentage reduction in crop yields under farmers’ practice (FP) over conservation agriculture with improved weed management practices (CAW).
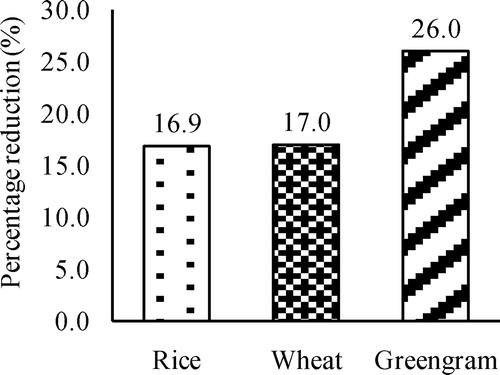
The economics of the various cropping systems were calculated by accounting the pooled values from both years (given in ). Practicing good weed controlling measures along with minimum tillage operations does have an impact on economic returns to the farmers. However, under CA treatments, only one minimum tillage operation was followed to sow the crop, and the heavy infestation of weeds reduced the crop yield and thus the economic return to the farmers was reduced. Under the FP treatment, because of using 3–6 tillage operations, the cost of cultivation (CoC) increases gradually by 30–48% over conservation agricultural practices. In addition to this increase in CoC due to tillage operations, the poor management of weeds also contributed to reduce economic returns. However, the situation was entirely different under CAW, for the entire cropping system. Practicing minimum tillage operations and good weed controlling measures through mechanized operations resulted in good benefit–cost ratios (BCRs) of 3.82 for rice, 3.98 for wheat and 2.71 for greengram. Singh et al. [Citation3] reported similar results. Further, the CoC was reduced by 24–32% for rice, 26–33% for wheat and 23–27% for greengram under conservation agricultural practices over FP. Therefore, practicing CAW was not only an environmentally friendly operation but also a crop cultivation practice capable of providing good economic returns [Citation59].
Table 9. Economic analysis of the cropping systems.
Conclusions
Adoption of mechanized conservation agricultural practices in farmers’ fields will eliminate the problem of crop residue burning and provide a better solution for crop residue management. In the cultivation of RWGS through conservation agricultural practices in farmers’ fields, a total of 51 tonnes of crop residues was generated from only 2 ha of farm area during the study period. This created an energy potential of 100.1 × 104 MJ and reduced emissions by 27,008.1 kg CO2, 11.1 kg N2O, 164.9 kg CH4, 34429.5 kg GWP (CO2e) and different air pollutants because the residue was used as crop mulch instead of burning it. The practice of CAW suppresses weeds effectively and increases yields by 21.1% in rice (4.3 t ha−1), 20.6% in wheat (4.8 t ha−1) and 35.1% in greengram (1.3 t ha−1) over FP. The cost of cultivation was also reduced in CAW over FP by 23.8% in rice, 26.2% in wheat and 22.5% in greengram, with highest BCR values of 3.8, 4.0 and 2.7 for rice, wheat and greengram, respectively. Therefore, the adoption of CAW at a larger scale will generate an energy source for thermal industries, giving the highest economic return to farmers with an environmentally friendly crop production.
Acknowledgement
The authors acknowledge the Indian Council of Agricultural Research, New Delhi for financial support and permission to conduct experimental trials at farmer fields.
Disclosure statement
No potential conflict of interest was reported by the authors.
References
- Ghosh D, Singh UP, Ray K, et al. Weed management through herbicide application in direct-seeded rice and yield modeling by artificial neural network. Span J Agric Res. 2016;14(2):e1003. doi:10.5424/sjar/2016142-8773.
- Mishra JS, Singh VP. Tillage and weed control effects on productivity of a dry seeded rice– wheat system on a Vertisol in Central India. Soil Till Res. 2012;123:11–20. doi:10.1016/j.still.2012.02.003.
- Singh PK, Sondhia S, Dubey RP, et al. Adoption and impact assessment of weed management technologies in wheat and greengram under conservation agriculture system in central India. Ind Jour Weed Scie. 2017;49(1):23–28. doi:10.5958/0974-8164.2017.00006.5.
- Singh H, Raheja A, Sharma R, et al. Happy seeder - a conservation agriculture technology for managing rice residue for central Punjab conditions. Int J Agri Eng. 2013;6(2):355–358.
- Sidhu HS, Singh M, Singh Y, et al. Development and evaluation of the Turbo Happy Seeder for sowing wheat into heavy rice residues in NW India. Field Crops Res. 2015;184:201–212. doi:10.1016/j.fcr.2015.07.025.
- Tripathi S, Singh RN, Sharma SK. Quantification and characterization of soil physico-chemical properties influence by wheat (Triticum aestivum) residue burning in India. J Glob Ecol Env. 2015;2(3):155–160.
- Sidhu HS, Singh M, Humphreys E, et al. The Happy Seeder enables direct drilling of wheat into rice stubble. Aus J Exp Agri. 2007;47(7):844–854. doi:10.1071/EA06225.
- Gupta R. Agro-environmental revolution in Punjab: case of the happy seeder technology. New Delhi, India: Indian Statistical Institute; 2011. (Discussion paper 11-11; p-30).
- Jain N, Bhatia A, Pathak H. Emission of air pollutants from crop residue burning in India. Aerosol Air Qual Res. 2014;14(1):422–430. doi:10.4209/aaqr.2013.01.0031.
- Fagodiya RK, Pathak H, Kumar A, et al. Global temperature change potential of nitrogen use in agriculture: A 50-year assessment. Sci Rep. 2017a;7:44928. doi:10.1038/srep44928.
- Carlton R, West J, Smith P, et al. A comparison of GHG emissions from UK field crop production under selected arable systems with reference to disease control. Eur J Plant Pathol. 2012;133(1):333–351. doi:10.1007/s10658-012-9961-0.
- Vetter SH, Sapkota TB, Hillier J, et al. Greenhouse gas emissions from agricultural food production to supply Indian diets: Implications for climate change mitigation. Agri Eco Env. 2017;237:234–241. doi:10.1016/j.agee.2016.12.024.
- Ravindra K, Singh T, Mor S. Emissions of air pollutants from primary crop residue burning in India and their mitigation strategies for cleaner emissions. J Clnr Prod. 2019;208:261–273. doi:10.1016/j.jclepro.2018.10.031.
- Smith P, Martino D, Cai Z, et al. Greenhouse gas mitigation in agriculture. Phil Tran R Soc B. 2008;363(1492):789–813. doi:10.1098/rstb.2007.2184.
- Gupta DK, Bhatia A, Kumar A, et al. Mitigation of greenhouse gas emission from rice–wheat system of the Indo-Gangetic plains: through tillage, irrigation and fertilizer management. Agric Eco Env. 2016;230:1–9. doi:10.1016/j.agee.2016.05.023.
- Shyamsundar P, Springer NP, Tallis H, et al. Fields on fire: alternatives to crop residue burning in India. Science. 2019;365(6453):536–538. doi:10.1126/science.aaw4085.
- Fagodiya RK, Pathak H, Bhatia A, et al. Simulation of maize (Zea mays L.) yield underalternative nitrogen fertilization using InfoCrop-maize model. Bioch Cell Arch. 2017b;17(1):65–71.
- Fagodiya RK, Pathak H, Bhatia A, et al. Nitrous oxide emission and mitigation frommaize-wheat rotation of upper Indo-Gangetic plains. Carbon Manag. 2019;10(5):489–499. doi:10.1080/17583004.2019.1650579.
- Malyan SK, Bhatia A, Kumar SS, et al. Mitigation of greenhouse gas intensity bysupplementing with Azolla and moderating the dose of nitrogen fertilizer. Biocatal Agri Biotech. 2019;20:101266. doi:10.1016/j.bcab.2019.101266.
- Glendining MJ, Dailey AG, Williams AG, et al. Is it possible to increase the sustainability of arable and ruminant agriculture by reducing inputs? Agri Sys. 2009;99(2-3):117–125. doi:10.1016/j.agsy.2008.11.001.
- Prasad J. A comparison between a rotavator and conventional tillage equipment for wheat-soybean rotations on a vertisol in Central India. Soil Til Res. 1996;37(2–3):191–197. doi:10.1016/0167-1987(95)00510-2.
- Gupta R, Kienzler K, Martius C, et al. Research prospectus: a vision for sustainable land management research in Central Asia. CGIAR-PFU, Tashkent, Uzbekistan: ICARDA Central Asia and Caucasus Program (Sustainable Agriculture in Central Asia and the Caucasus Series No. 1); 2009.
- Khambalkar V, Pohare J, Katkhede S, et al. Energy and economic evaluation of farm operations in crop production. J Agri Sci. 2010;2(4):191–200.
- Fagodiya RK, Pathak H, Bhatia A, et al. Global warming impacts of nitrogen use in agriculture: an assessment for India since 1960. Carbon Manage. 2020;11(3):291–301. doi:10.1080/17583004.2020.1752061.
- Melander B, Rasmussen IA, Barberi P. Integrating physical and cultural methods of weed control examples from European research. Weed Sci. 2005;53(3):369–381. doi:10.1614/WS-04-136R.
- Sharma AR, Singh VP. Integrated weed management in conservation agriculture systems. Ind J Weed Sci. 2014;46:23–30.
- Bajwa AA. Sustainable weed management in conservation agriculture. Crop Prot. 2014;65:105–113. doi:10.1016/j.cropro.2014.07.014.
- Jat ML, Saharawat YS, Gupta R. Conservation agriculture in cereal systems of south Asia: nutrient management perspectives. Kar J Agri Sci. 2011;24(1):100–105.
- Friedrich T, Josef K, Amir K. Conservation agriculture in developing countries: the role of mechanization. Paper presented at: Innovation for Sustainable Agricultural Mechanization, Hannover, Germany, November 8, 2009.
- Kumar V, Jat HS, Sharma PC, et al. Can productivity and profitability be enhanced in intensively managed cereal systems while reducing the environmental footprint of production? Assessing sustainable intensification options in the breadbasket of India. Agri Ecos Env. 2018;252:132–147. doi:10.1016/j.agee.2017.10.006.
- Parihar CM, Parihar MD, Sapkota TB, et al. Long-term impact of conservation agriculture and diversified maize rotations on carbon pools and stocks, mineral nitrogen fractions and nitrous oxide fluxes in inceptisol of India. Scie Total Env. 2018;640–641:1382–1392. doi:10.1016/j.scitotenv.2018.05.405.
- Pratibha G, Srinivas I, Rao KV, et al. Impact of conservation agriculture practices on energy use efficiency and global warming potential in rainfed pigeonpea– castor systems. Europ J Agronomy. 2015;66:30–40. doi:10.1016/j.eja.2015.02.001.
- Prathibha G, Srinivas I, Rao KV, et al. Net global warming potential and greenhouse gas intensity of conventional and conservation agriculture system in rainfed semi arid tropics of India. Atm Env. 2016;145:239–250. doi:10.1016/j.atmosenv.2016.09.039.
- Kumar S, Dubey RP, Sondhia S, et al. Annual report 2016–17. Jabalpur, India: ICAR-Directorate of Weed Research; 2017.
- Gharde Y, Singh PK, Dubey RP, et al. Assessment of yield and economic losses in agriculture due to weeds in India. Crop Prot. 2018;107:12–18. doi:10.1016/j.cropro.2018.01.007.
- Chethan CR, Krishnan AD. Dynamic push–pull strength data generation for agricultural workers to develop manual dryland weeders. Cur Sci. 2017;113(08):1601–1605. doi:10.18520/cs/v113/i08/1601-1605.
- , Yaduraju NT, Mishra JS. Enhancing farmers’ income through smart weed management. In: Kumar S, Choudhary PP, Bodake P, editors. Proceedings of the Biennial Conference on Doubling Farmers’ Income by 2022: The Role of Weed Science; March 1–3; Udaipur (India). Jabalpur (India): Indian Society of Weed Science; 2017. p. 5–6.
- Tewari VK, Chethan CR. Mechanization in weed management: Global review. In: Kumar S, Mishra JS, editors. Fifty years of Weed Research in India. Jabalpur (India): Indian Society of Weed Science, Jabalpur; 2018. p. 215–237.
- Tewari VK, Datta RK, Murthy ASR. Field performance of weeding blades of a manually operated push-pull weeder. J Agril Eng Res. 1993;55(2):129–141. doi:10.1006/jaer.1993.1038.
- Chethan CR, Chander S, Kumar SP. Dynamic strength based dryland weeders – ergonomic and performance evaluation. Ind Jour Weed Scie. 2018a;50(4):382–387. doi:10.5958/0974-8164.2018.00081.3.
- Chethan CR, Singh PK, Chander S, et al. Use of efficient weeding tools to reduce farmers’ drudgery. Ind Farm. 2018b;68(11):24–28.
- Chethan CR, Singh PK, Dubey RP, et al. Herbicide application in agriculture: nozzle selection and effective application. Ind Farm. 2018c;68(12):28–30.
- Singh PK, Dubey RP, Chethan CR, et al. Weed management in pulses under mechanized farming. In: Khare D, Nahatkar SB, Shrivastava AK, et al. editors. Farm mechanization for production. Jodhpur (India): Scientific publishers; 2018. p. 147–162.
- Google.co.in [Internet]. India: Google maps; 2020. Available from: https://www.google.com/maps/d/edit?mid=1FBXzUYimPILCz_oN1Fx-ajmDp0WbASMz&ll=23.664626483169968%2C79.55686642223554&z=10
- Streets DG, Bond TC, Carmichael GR, et al. An inventory of gaseous and primary aerosol emissions in Asia in the year 2000. J Geophys Res. 2003;108(D21):8809. doi:10.1029/2002JD003093.
- Eggleston HS, Buendia L, Miwa K, et al. IPCC Guidelines for National Greenhouse Gas Inventories. IGES, Japan: Institute for Global Environmental Strategies, The Intergovernmental Panel on Climate Change (IPCC); 2006. (IPCC 2006, ISBN 4-88788-032-4).
- Yang S, He H, Lu S, et al. Quantification of crop residue burning in the field and its influence on ambient air quality in Suqian, China. Atmos Env. 2008;42(9):1961–1969. doi:10.1016/j.atmosenv.2007.12.007.
- Kanabkaew T, Oanh NTK. Development of spatial and temporal emission inventory for crop residue field burning. Environ Model Assess. 2011;16(5):453–464. doi:10.1007/s10666-010-9244-0.
- Li X, Wang S, Duan L, et al. Particulate and trace gas emissions from open burning of wheat straw and corn stover in China. Env Sci Tech. 2007;41(17):6052–6058. doi:10.1021/es0705137.
- Sahai S, Sharma C, Singh DP, et al. A study for development of emission factors for trace gases and carbonaceous particulate species from in situ burning of wheat straw in agricultural fields in India. Atmos Env. 2007;41(39):9173–9186. doi:10.1016/j.atmosenv.2007.07.054.
- Zhang H, Hu D, Chen J, et al. Particle size distribution and polycyclic aromatic hydrocarbons emissions from agricultural crop residue burning. Environ Sci Technol. 2011;45(13):5477–5482. doi:10.1021/es1037904.
- Koopmans A, Koppejan J. Agricultural and forest residues - Generation, utilization and availability. Paper presented at: Regional Consultation on Modern Applications of Biomass Energy, Kuala Lumpur, Malaysia, January 6–10; 1997.
- Gadde B, Menke C, Wassmann R. Rice straw as a renewable energy source in India, Thailand, and the Philippines: overall potential and limitations for energy contribution and greenhouse gas mitigation. Biomass Bioenergy. 2009;33(11):1532–1546. doi:10.1016/j.biombioe.2009.07.018.
- Saran G, Ahlawat IPS, Yaduraju NT. Agronomic terminology. 5th rev. ed. New Delhi: Indian Society of Agronomy; 2009.
- Mahmood A, Ihsan MZ, Khaliq A, et al. Crop residues mulch as organic weed management strategy in maize. Clean – Soil Air Water. 2015;43(9999):1–8.
- Saini JP, Angiras NN. Evaluation of ethoxysulfuron against broad-leaved weeds and sedges in direct seeded puddled rice. Ind J Weed Sci. 2002;34:36–38.
- Singh S, Singh H, Narwal S, et al. Evaluation of Alkomba and tank mixture of Almix C Bulachlor for control of weeds in transplanted rice. Ind J Weed Sci. 2003;35:24–26.
- Chhokar RS, Sharma RK, Jat GR, et al. Effect of tillage and herbicides on weeds and productivity of wheat under rice-wheat growing system. Crop Prot. 2007;26(11):1689–1696. doi:10.1016/j.cropro.2007.01.010.
- Bhattacharyya R, Das TK, Sudhishri S, et al. Conservation agriculture effects on soil organic carbon accumulation and crop productivity under a rice–wheat cropping system in the western Indo-Gangetic Plains. Eur J Agr. 2015;70:11–21. doi:10.1016/j.eja.2015.06.006.
- Google.co.in [Internet]. India: Google maps; 2020. Available from: https://www.google.com/maps/d/edit?mid=1FBXzUYimPILCz_oN1Fx-ajmDp0WbASMz&ll=23.664626483169968%2C79.55686642223554&z=10.