Abstract
An in vitro gas production study was conducted to test the effect of papaya leaf (PL) at different concentrations of 0 (CON), 10% (Low PL: LPL), 15% (Medium PL: MPL) and 25% (High PL: HPL) of replacement with alfalfa hay (AH) in substrate (50% AH + 50% concentrate) on rumen methane (CH4) production, rumen biohydrogenation (BH) of polyunsaturated fatty acid (PUFA) and rumen microbial population. PL at different concentrations were mixed with 30 ml of buffered rumen fluid and were incubated for 24 h. Methane production (ml/250 mg DM) declined at a decreasing rate (linear p = 0.03) with increasing levels of PL. The acetic/propionic ratio (1.80) was lowest (p < 0.05) for HPL compared with CON (2.04), LPL (1.95) and MPL (1.89). Supplementation of the diet with PL significantly (p < 0.05) decreased the rate of BH of C18:2n-6 (LA) and C18 PUFA after 24 h of incubation especially at the higher inclusion rate, resulting in an increased production of rumen BH intermediates such as conjugated linoleic acid (CLA), whereas saturated fatty acid (SFA) decreased in the rumen fluid after 24 h of incubation. Estimates of rumen microbial population using real-time PCR assay showed a reduction in total methanogens (p < 0.05) in MPL (−12%) and HPL (−16%) and increase in total bacteria population (HPL: +5%) and Butyrivibro fibrisolvens (MPL: +20; HPL: +23%) among PL treatment groups compared with the CON group. These results emphasise PL as a beneficial feed material for ruminants.
Introduction
Global warming and climate change are big issues with negative consequences on the environment and livestock production. Livestock production and its by-products are responsible for at least 51% of global warming gases in which CH4 comprises 15–20% of them (Wanapat et al. Citation2013). CH4 which has a heat trapping potential 23 times than that of CO2, accounts for 2–12% loss of dietary gross energy in ruminants (Bhatta et al. Citation2012). Therefore, mitigation of CH4 production by ruminants has been recognised as an important goal because it reduces the greenhouse gas (GHG) emission and improves feed efficiency (Bouchard et al. Citation2015). Enteric CH4 is a by-product of anaerobic fermentation in the rumen which is produced by methanogenic archaea for disposing metabolic hydrogen (H2) produced by their metabolic activity (Bhatta et al. Citation2014). In the rumen ecosystem, rumen protozoa are also involved in methanogenesis through a symbiotic relationship with methanogenic archaea which utilise H2 produced by the protozoa to produce CH4 (Tan et al. Citation2011).
The production of healthy food is an important issue in current ruminant nutrition research. Health promoting properties of ruminant products (meat and milk) have been attributed to PUFA, such as linoleic acid (LA) and conjugated linoleic acids (CLA; especially cis 9 and trans 11C18:2) (Jayanegara et al. Citation2011). Large amounts of PUFA originally present in feed rapidly disappeared in the rumen through microbial biohydrogenation (BH, transformation of unsaturated fatty acid to saturated fatty acid by rumen microbes) (Chilliard et al. Citation2007). But, many intermediates are produced during this process such as CLA mainly cis 9, trans 11 C18:2 and vaccenic acid (VA), which have been reported to prevent cancer, reduce atherosclerosis, improve immune response, reduce fat and increase lean deposition in muscle (Kim et al. Citation2010). Thus, reducing the extent of ruminal BH of PUFA, on one hand, and maximising the synthesis of intermediates such as cis 9, trans 11 CLA, on the other hand, are therefore desirable and are important goals of ruminant nutritionists.
The manipulation of the rumen microbial ecosystem to increase the efficiency of rumen metabolism and ultimately the productivity of animals has long been a pertinent goal for nutritionists and rumen microbiologists. Plants containing secondary metabolites (PSM), particularly the tannins, are able to reduce the extent of ruminal BH of PUFA at various steps of the pathways (Vasta et al. Citation2009; Cabiddu et al. Citation2010; Jayanegara et al. Citation2011, Citation2012). The PSM are also recommended as a means for reducing rumen methane (Wanapat et al. Citation2013). The papaya tree is a tree-like plant, a member of the small family Caricaceae and widely cultivated for its edible fruits and can be found in all tropical countries and many sub-tropical regions of the world (Canini et al. Citation2007). Ayoola and Adeyeye (Citation2010) showed that the papaya leaf (PL) is a source of essential nutrients, which could be used as potential source of useful elements for drug formulation. Quantitive analysis of PL has also shown the presence of phenolic acids as the main compound in PL extract (Canini et al. Citation2007). In a study by Maisarah et al. (Citation2014), PL exhibited the highest phenolic content among other parts of the papaya (unripe papaya fruit, ripe papaya fruit and seed) which shows the medicinal properties of PL.
The objectives of present study were to identify the effect of PL on in vitro gas production, fermentation characteristics, microbial population and processes involved in ruminal BH and ruminal methanogenesis to decrease the production of methane and to decrease BH of fatty acids in the rumen as well.
Materials and methods
Experimental plant materials and their chemical analyses
The experiment was conducted at the research farm of the faculty of Veterinary Medicine, Universiti Putra Malaysia, Serdang, Selangor. The experimental protocols were approved by the Animal Care Committee of Universiti Putra Malaysia. Samples of PL were harvested from the Malaysian Agriculture Research And Development Institute (MARDI, 3°00' 21.34″N, 101° 42'). Fresh PL samples (phonological stage: recently matured) were oven-dried at 60 °C for 48 h in and ground to pass a 1-mm sieve. Then, they were preserved in tightly closed plastic bags and stored in −20 °C until further analysis. The PL samples were analysed for the contents of dry matter (DM), ash, crude protein (CP), ether extract (EE), acid detergent fibre (ADF) and neutral detergent fibre (NDF) using standard methods. The DM content was determined according to AOAC (Citation1990) by weighing 5 g of sample to dry at 105 °C to constant weight for 24 h. The CP content of feed was determined using the Kjeldahl method (CP (%) = N, total nitrogen % × 6.25). The NDF and ADF were determined according to Van Soest et al. (Citation1991). The ash content was determined by furnace oven at 550 °C for 5 h. Lipids were extracted in a Soxhlet apparatus with petroleum ether at 40–60 °C for 1 h. Determination of phenolic contents was conducted by following the protocols of Makkar and Becker (Citation2003). Prior to determination, the phenol contents in PL were extracted twice with aqueous ethanol (700: 300 v/v) for 3 h at 80 °C. Total phenols (TP) and total tannins (TT) were determined by a modified Folin–Ciocalteu method using polyvinyl–polypyrrolidone (Sigma-Aldrich GmbH, Steinheim, Germany) to separate tannin phenols from non-tannin phenol (NTP); the values were calibrated against a gallic acid standard (Sigma-Aldrich GmbH, Steinheim, Germany) and expressed as gallic acid equivalents. Briefly, 0.5 mL of the extract was placed in a test tube, then 0.25 mL of Folin reagent (1 N; Sigma-Aldrich GmbH**) and 1.25 mL of sodium carbonate solution (40 g Na2CO3.10H2O) were added. The tube was vortexed and kept in the dark thereafter for 40 min. The absorbance was read at 725 nm using a UV-vis spectrophotometer (Shimadzu UV-160, Shimadzu Corporation, Kyoto, Japan). Condensed tannins (CT) were determined by the butanol–HCl–iron method according to Porter and Hrstich (Citation1986) as described by Jayanegara et al. (Citation2011) and expressed as catechin equivalents. In brief, a total of 0.5 mL of the extract was mixed with 0.1 ml of ferric reagent (2.0 g ferric ammonium sulphate in 100 mL of 2 N HCl), followed by 3 mL butanol–HCl (95:5 v/v), and the tube was vortexed. Subsequently, the tube was heated in a boiling water bath at 100 °C for 60 min. The absorbance was read at 550 nm. Hydrolysable tannins (HT) were estimated as the difference between TT and CT. Chemical composition and fatty acid content of substrates used for the in vitro incubation are shown in .
Table 1. Ingredients and chemical composition and fatty acid content of substrates used for the in vitro incubation.
In vitro incubation
Four rumen fistulated male goats (Kajang crossbred) with an average body weight of 39 ± 0.7 kg (body weight ± standard error) were used as rumen fluid donors. The goats were fed twice daily with a diet () containing 50% AH and 50% concentrate (W/W) twice daily at 08:00 and 17:00 h. Rumen fluid was sampled before the morning feeding at 08:00 h from the four goats and placed immediately in warm (39 °C) insulated flasks under anaerobic conditions. In the laboratory, samples were pooled in equal proportions and strained through four layers of cheesecloth under anaerobic conditions and used immediately. Strained rumen fluid were diluted 1:2 with two kinds of buffer phosphate buffer (1.4 g NH4Cl + 28.8 g Na2HPO4·12H2O + 6.2 g NaH2PO4 in 1 l of distilled water; pH = 7.0) and bicarbonate buffer (39.2 g NaHCO3 in 1 l of distilled water) according to Fievez et al. (Citation2005). Then 30 ml of buffered rumen fluid solution was dispensed into 100 ml calibrated syringes containing different concentrations of PL: 0 (CON, 50% AH + 50% concentrate), 15% of AH in substrate replaced by PL (18.75 mg/250 mg DM), 25% of AH in substrate replaced by PL (31.25 mg/250 mg DM) and 50% of AH in substrate replaced by PL (62.50 mg/250 mg DM). Linoleic acid (LA) was added to the incubations as a PUFA source for BH purposes. Additionally, the buffered rumen fluid samples were sampled before incubation (0 h) to determine the initial FA profiles, which later were used for calculating the BH of fatty acid. Syringes were incubated at 39 °C for 24 h. Volumes of the gas produced were determined at times of 0, 2, 4, 6, 8, 10, 12 and 24 h of incubation. Net gas production values were corrected by subtracting blank values from the samples.
Analysis after incubation
After 24 h of incubation, 1 ml of the gas phase was sampled from the syringe and analysed by gas–liquid chromatography (Agilent 5890 Series Gas Chromatograph, Wilmington, DE) equipped with flame ionisation detector (FID) for the determination of methane production. Calibration was completed using standard methane prepared by Scotty Specialty Gases (Supelco, Bellefonte, PA). All the procedures were repeated three times. One millilitre of syringe content was immediately collected and frozen (−20 °C) for DNA extraction and microbial population quantification. The pH of the contents of the syringes was determined using a pH electrode (Mettler-Toledo Ltd., England, UK). The samples were acidified with 25% metaphosphoric acid and centrifuged (10 min, 4 °C at 15 000×g), filtered, and the filtrate was used to determine volatile fatty acid (VFA) and ammonium nitrogen (NH3N). The VFA were determined using gas chromatography with Quadrex 007 Series (Quadrex Corporation, New Haven, CT) bonded phase fused silica capillary column (15 m, 0.32 mm ID, 0.25 μm film thickness) in an Agilent 7890A gas-liquid chromatography (Agilent Technologies, Palo Alto, CA) equipped with a FID. 4-Methyl-n-valeric acids (Sigma, St. Louis, MO) was used as an internal standard for VFA determination. The concentration of NH3N (mg/dl) was determined using the colorimetric method described by Solorzano (Citation1969). A standard curve using ammonium chloride (NH4Cl) was prepared to determine whether a linear relationship existed between the varying concentrations of an NH4Cl standard solution and the intensity of colour produced.
Fatty acid analysis and ruminal biohydrogenation calculation
Fatty acid content of substrates (PL, AH and concentrate) and whole syringe contents before (0 h) and after fermentation (24 h) were determined based on the method of Folch et al. (Citation1957) with some modifications by Rajion et al. (Citation1985) as described by Ebrahimi et al. (Citation2014) using chloroform:methanol 2:1 (v/v) containing butylated hydroxytoluene to prevent oxidation during sample preparations. The extracted fatty acids were transmethylated to their fatty acid methyl esters (FAME) using 0.66N KOH in methanol and 14% methanolic boron trifluoride (BF3) (Sigma Chemical Co. St. Louis, MO) according to the methods of AOAC (Citation1990). The FAME was separated using Agilent 7890A gas-liquid chromatography (Agilent Technologies, Palo Alto, CA) using a 100 m × 0.25 mm ID (0.20 μm film thickness) Supelco SP-2560 capillary column (Supelco, Inc., Bellefonte, PA). One microlitre (μl) of FAME was injected by an auto sampler into the chromatograph, equipped with a FID. The split ratio was 1:30 after injection of 1 μl of the FAME. The injector temperature was programmed at 250 °C and the detector temperature was 300 °C. The column temperature program initiated runs at 100 °C, for 2 min, warmed to 170 °C at 10 °C/min, held for 2 min, warmed to 220 °C at 7.5 °C/min and then held for 10 min to facilitate optimal separation. The identification of the peaks was made by comparison of equivalent chain lengths with those of authentic FAME (37 Component FAME mix, Supelco, Bellefonte, PA). Peak areas were determined automatically using the Agilent Gas Chromatography ChemStation software (Agilent Technologies, Palo Alto, CA). The fatty acid concentrations are expressed as g/100 g of identified fatty acid. A reference standard (mix C4-C24 methyl esters; Sigma-Aldrich, Inc., St. Louis, MO) and CLA standard mix (cis-9, trans-11 and trans 10, cis 12 CLA, Sigma-Aldrich, Inc., St. Louis, MO) was used to determine recoveries and correction factors for the determination of individual FA composition.
The BH percentage of PUFA was calculated as the decrease of PUFA at 24 h from the initial PUFA at 0 h of incubation as described by Jayanegara et al. (Citation2011):
PUFA (0 h): concentration (g/100g of FA) of PUFA at 0 h incubation; PUFA (24 h): concentration (g/100g of FA) of PUFA at 24 h of incubation.
Ruminal microbial quantification by real-time polymerase chain reaction
The DNA was extracted from the thawed rumen fluid using the QIAamp DNA Stool Mini Kit (Qiagen Inc., Valencia, CA) according to the protocol of the manufacturer. The concentration of DNA was measured by Nanodrop 2000 spectrophotometer (Thermo Scientific, Wilmington. DE). DNA concentrations ranged between 30 and 50 ng/μl. DNA samples were used as templates for real-time polymerase chain reaction (PCR) assay. The relative abundance of total bacteria, total methanogen, Butyrivibrio fibrosolvens and total protozoa in extracted samples from the goat was measured by real-time PCR and the SYBR Green PCR Master Mix Kit (Bio-Rad Laboratories, Hercules, CA). Real-time PCR was performed with the Bio-Rad CFX96 Touch (Bio-Rad Laboratories, Hercules, CA) using optical grade plates. The PCR reaction was performed on a total volume of 25 μl using the iQTMSYBR Green Supermix (Bio-Rad Laboratories, Hercules, CA). Each reaction included 12.5 μl SYBR Green Supermix (Bio-Rad Laboratories, Hercules, CA), 1 μl of each primer, 1 μl of DNA samples and 9.5 μl RNAse free waters. To confirm the specificity of amplification, a melting curve analysis was carried out after the last cycle of each amplification. PCR primers used for amplifying target bacteria in the rumen are shown in .
Table 2. Polymerase chain reaction primers used for amplifying target bacteria in the rumen.
Statistical analysis
All fermentation data and fatty acid data were analysed using the MIXED procedure of SAS ver. 9.1 (SAS Institute Inc., Cary, NC). For statistical analysis of in vitro gas production, sampling at different times were added to the model and analysed using repeated measures. The microbial data were normalised using the log10-transformation for the analysis. Means were separated using the “pdiff” option of the “lsmeans” statement of the MIXED procedure. Polynomial contrasts (linear and quadratic effects) were also used. Values of p < 0.05 were considered significant.
Results and discussion
Rumen fermentation parameters
The results of rumen fermentation characteristics are shown in . In general, rumen fermentation parameters were positively improved by the PL in the current study. Total gas production () was not affected by the experimental treatments. Total gas production for CON, LPL, MPL and HPL was 50.33, 47.17, 47.33 and 51.17 (ml/250 mg DM), respectively. Lack of significant difference in total gas production by increasing levels of PL represents minimal detrimental effect of PL on rumen fermentation characteristics. The CH4 production decreased linearly (linear p= 0.03) with increasing levels of PL. The maximum reduction in methane production with PL inclusion was recorded in HPL (−38%) followed by MPL (−35%) and LPL (−17%) compared with CON.
Figure 1. Cumulative gas production of control (CON) and different inclusion levels of papaya leaf at different times of incubation. CON: 50% concentrate + 50% AH, LPL: 15% replacement of AH substrate by PL, MPL: 25% replacement by PL, HPL: 50% replacement by PL. Vertical bars are standard error.
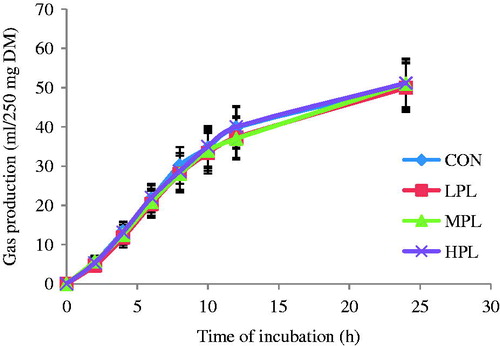
Table 3. Effect of the addition of different levels of papaya leaf on rumen fermentation parameters after in vitro incubation with rumen fluid from goats.
The phenolic content of PL is shown in . The PL contains TP (30.31 GAE/g), TT (28.36 GAE/g), CT (17.39 CAE/G) and HT (10.96 GAE/g). Reduced rumen CH4 production in this study could be linked to the role of tannins present in PL in reducing methanogenic archaea population (Bhatta et al. Citation2012). Tannins could reduce methane reduction because of its inhibition of fibre degradation, reduction in protozoa and methanogenic archaea population. It was also established that samples containing both hydrolysable tannin plus condensed tannin had additive effect in reducing in vitro methane production (Bhatta et al. Citation2009, Citation2012). Phenolic acids such as p-coumaric acids, ferulic acids and cinnamic acids, which were reported to be active compounds in PL by Canini et al. (Citation2007) were also effective in decreasing rumen CH4 (Bhatta et al. Citation2012). In a study by Tan et al. (Citation2011), 33% reduction in in vitro CH4 production at the inclusion of 10 mg of purified CT from Leucaena/500 mg DM had been observed. In a study by Bhatta et al. (Citation2013), it was shown that plant leaves containing secondary metabolites as their contents such as Rauvolfia serpentine, Indigofera tinctoria and Withania somnifera have great potential to suppress methanogenesis with minimal adverse effect on feedstuff fermentation. Zmora et al. (Citation2012) indicated that Mentha piperita (MP) leaves which contain different classes of secondary metabolites at a concentration of 16.34 and 23.35 mg of MP to the 233.30 mg of substrate decreased rumen methane production at 24 h of incubation by altering the rumen micro-organisms’ profiles without changing the profile of VFA and DM digestibility. As expected, reduced CH4 production in this study was also accompanied by reduced acetic/propionate ratio providing the sink for metabolic H2 during rumen fermentation. The acetic/propionic ratio was lowest in HPL (1.80) followed by LPL (1.89) and MPL (1.95). CON had the highest (p<0.05) acetic/propionic ratio (2.04) among treatment groups. Although there was not much difference in VFA production among diets, the addition of PL led to a decrease in the molar proportion of acetic acid and reduction of the acetic:propionic ratio. This might have been due to the fact that PL is a substrate that is more reduced than the substrate present in diets without PL. The effects of different treatments on in vitro fermentation characteristics are presented in . The proportion of acetate (mol/100 mol) was lower in LPL (56.91) and MPL (59.06) compared with HPL (68.07) and CON (67.31). The highest proportion of propionate (mol/100 mol) was recorded for CON (33.46) followed by HPL (30.09), MPL (30.25) and LPL (30.10). There was no significant difference (p>0.05) between CON and HPL in terms of propionate concentration. The pH in the treatment groups ranged from 7.35 to 7.38. There was significant (p<0.05) difference between CON (pH = 7.38) and MPL (pH = 7.35) in terms of pH. The mean pH was slightly lower (7.35) in MPL diets, a reduction that was probably not large enough to cause a disturbance in bacterial populations. The NH3N was higher (p<0.05) in the PL treatment group (23.05, 23.44 and 22.55 (mg/dl) for LPL, MPL and HPL, respectively) than CON (18.23 mg/dl). In the current study, the concentration of NH3N ranged from 22.55 to 23.44 mg/dl among the PL treatment groups. The higher concentrations of NH3N observed with PL treatment groups may have resulted from an increase in the deamination of amino acids by hyper-ammonia-producing bacteria (Gunal et al. Citation2014). It has been shown that the concentration of 15–20 mg/100 ml rumen fluid NH3N is required for maximum nutrient utilisation in goats (Samanta et al. Citation2003), which results obtained in this study are within the range of optimum microbial growth and nutrient utilisation.
Table 4. Phenolic compound present in papaya leaf.
Biohydrogenation of fatty acids
The process of BH reduces the rumen outflow of PUFA and contributes to accumulation of cis and trans isomers in ruminant products, including CLA and trans monoenes. Hence, the extent and the type of the rumen BH process will determine both the amounts and structures of fatty acids leaving the rumen. As the fatty acid structure determines its physiological features, interest has grown in the process of rumen BH (Fievez et al. Citation2007). The BH results of OA, C18:2n-6 (LA), C18:3n-3 (LNA) and C18 PUFA are presented in . Concentrations of rumen fatty acids at 0 h and 24 h of incubation are shown in . Increasing inclusion level of PL 15% (LPL), 25% (MPL) and 50% (HPL) decreased (p<0.05) the percentage BH of C18 PUFA at 24 h incubation. The percentage of BH of C18 PUFA for HPL, MPL, LPL and CON was 25.32, 30.65, 32.64 and 50.57, respectively. There was also a significant difference (p<0·05) in the percentage BH of LA between HPL (62.85%) and CON (79.27%). The percentage results of BH intermediates shows in . The production of cis 9, trans 11 CLA and C18:1t-11 were not different (p>0.05) among treatments. Interestingly, production of trans 10, cis 12 CLA was significantly (p<0.05) different between HPL and CON. There was a significant difference (p<0.05) between HPL (33.87 g/100 g) and CON (23.62 g/100 g) in terms of C18 PUFA. In this study, the BH of LA and C18 PUFA were significantly (p<0.05) affected by the inclusion of PL under in vitro conditions. The BH of LA and LNA resulted in an increase in the concentration of trans 10, cis 12 CLA reaching its maximum for HPL treatment groups at 24 h of incubation time. This means that the presence of secondary metabolites in PL, group B bacteria responsible for the final BH of PUFA were selectively inhibited hence favouring accumulation of one of the CLA isomers (trans 10, cis 12 CLA). In a study by Jayanegara et al. (Citation2011) on 27 different tropical plant species which were incubated with linseed oil under in vitro conditions, it was found that hydrolysed tannin (HT) and condensed tannin (CT) contributed to the inhibition of fatty acid BH but at different steps. Vasta et al. (Citation2009) reported that accumulation of BH intermediate C18:1 in vitro could be attributed to the effect of condensed tannins, a class of secondary metabolites. The CLA is a very beneficial FA when absorbed and incorporated into the animal tissues that are consumed by humans. The formation of CLA from LA is facilitated by group A bacteria such as Butyrivibrio species which are responsible for the conversion of LA to CLA. The increase in production of CLA in this study was also consistent with the increase in Butyrivibrio fibrisolvens species which confirms the finding of Vasta et al. (Citation2009). The actions of the group B bacteria were probably reduced at higher inclusion levels of PL favouring the production and accumulation of CLA in this study. Therefore, it can be suggested that there was selective inhibition of group B bacteria which are responsible for terminal step in BH process while group A which contains B. fibriosolvens was spared hence promoting the accumulation of CLA in this study. In an in vitro study (using a dairy cow as inoculum donor), Cabiddu et al. (Citation2010) showed that lipolysis and BH of PUFA could be affected by plant phenols, particularly tannic phenol (TP).
Figure 2. Effect of incremental levels of papaya leaf on the percentage of variation with respect to the control (i.e. without PL) of C18:1n-9, C18:2n-6, C18:3n-3 and C18 PUFA (C18:1n-9 + C18:2n-6 +C18:3n-3) content after 24 h in vitro incubation with rumen inoculum from goats. Differences (p < 0.05) compared with the control (CON). Vertical bars are standard error of the mean, a,bdifferent letters denote significant difference at p<0.05.
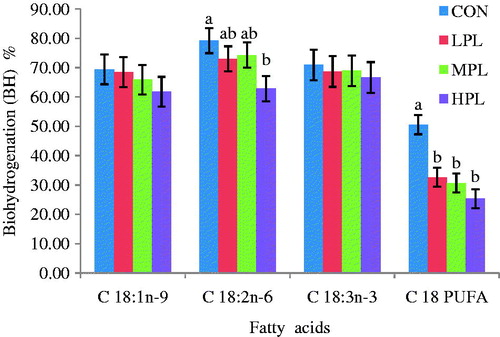
Figure 3. Production of biohydrogenation intermediates after 24 h of incubation. CON: 50% concentrate + 50% AH, LPL: 15% replacement of AH substrate by PL, MPL: 25% replacement by PL, HPL: 50% replacement by PL. Vertical bars are standard error of the mean; a,bdifferent letters denote significant difference at p<0.05; C18:1t-11, vaccenic acid; c9t11 CLA, rumenic acid.
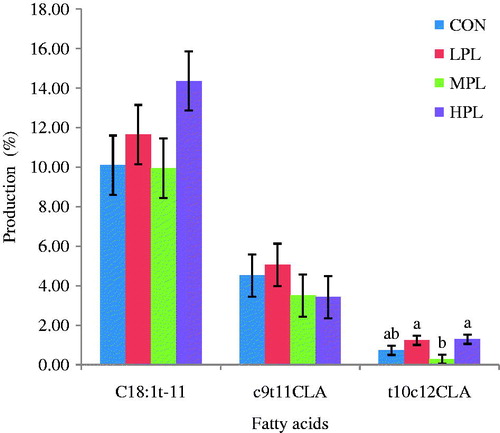
Table 5. Effect of the addition of different levels of papaya leaf on rumen concentration of fatty acids at 0 h and 24 h of in vitro incubation with rumen fluid from goat.
Rumen bacteria and protozoa population
Results of the relative abundance of total bacteria, B. fibrisolvens, total protozoa and total methanogens in rumen are presented in . The use of PL in the diets had no effects (p>0.05) on the relative abundance of total protozoa compared with the CON. The total protozoa population ranged from 5.67 to 6.52 (log10cell/l of rumen fluid). Interestingly, the use of PL in the diets affected (p<0.05) the relative abundance of methanogen bacteria in MPL (7.87) and HPL (7.67) compared with CON (9.06 log10cell/l of rumen fluid). There was a linear increase of the B. fibrisolvens population with increasing levels of PL especially in MPL and HPL compared with CON. The total bacterial population was also higher (linear p=0.06) in HPL (11.09) compared with MPL (10.57) and CON (10.53) log10cell/l of rumen fluid. In the current study, the population of protozoa ranged from 5.67 to 6.59 (log10cell/l) without any significant (p>0.05) difference among treatments. Interestingly, the population of total methanogens was affected (p<0.05) by PL treatment groups, MPL and HPL with, respectively (−12%) and (−16%) reduction in population of total methanogens compared with CON. Rumen protozoa is responsible for symbiotic transfers of H2 with methanogens, which is used to reduce CH4, producing over 25% of CH4 in the rumen (Newbold et al. Citation1995; Castagninoa et al. Citation2015). Meanwhile, Machmuller et al. (Citation2003) suggested that the association between protozoa and methanogens does not play an important role in rumen methanogenesis. From the results of methanogen and protozoa population obtained in this study, it could be concluded that PL may have had a direct effect on methanogenic archaea populations that were not associated with the protozoa and use of PL to induce defaunation as a CH4 reduction strategy in ruminants needs to be viewed with caution.
Table 6. Effect of papaya leaf on the population of bacteria and protozoa at 24 h of in vitro incubation.
Many ruminal bacteria species of the genera Butyrivibrio are known to participate in ruminal BH of fatty acids (Harfoot & Hazlewood Citation1997; Maia et al. Citation2007). Kemp (Citation1984) has classified bacteria involved in the different steps of the BH pathway into two groups, A and B.
**B. fibrisolvens is the most active species among the group A and plays a major role in biohydrogenating unsaturated fatty acids to vaccenic acid (VA), while few species of bacteria such as B. proteoclasticus (group B) hydrogenate the last step of the BH process. The MPL (+20%) and HPL (+23%) groups significantly (p<0.05) increased the rumen population of B. fibrisolvens compared with CON. The results obtained in the current study on the population of B. fibrisolvens are consistent with the findings of Vasta et al. (Citation2010) who reported that use of quebracho tannin (9.6% of DM intake) in the diets of sheep increased the relative abundance of B. fibrisolvens. It seems that PL-containing treatment groups could decrease the last step of the rumen BH process (conversion of CLA and VA to stearic acid) due to their ability to increase some intermediate products such as CLA and inhibit the activity of some ruminal bacteria and consequently less C18:0 (stearic acid) production. Moreover, in a study of 91 Australian plants containing bioactive compounds, most of which showed inhibitory effects towards B. proteoclasticum without affecting B. fibrisolvens (Durmic et al. Citation2008).
Conclusions
Supplementing different levels of PL suppressed CH4 emissions and BH percentage of LA and C18 PUFA at the same time in an in vitro condition. The PL addition improved fermentation characteristics without negative effect on total gas production in vitro. In addition, the use of PL in the diets affected the relative abundance of total bacteria and decreased rumen BH through increasing B. fibrisolvens and reduced rumen methanogenesis through reducing total mehanogens.
Acknowledgements
The authors are very grateful to the Faculty of Veterinary Medicine and Faculty of Agriculture, Universiti Putra Malaysia.
Disclosure statement
The authors report no conflicts of interest. The authors alone are responsible for the content and writing of this article.
References
- AOAC. 1990. Official method of analysis. 15th ed. Arlington, VA: Association of Analytical Chemists.
- Ayoola PB, Adeyeye A. 2010. Phytochemical and nutrient evaluation of Carica papaya (pawpaw) leaves. IJRRAS. 5:325–328.
- Bhatta R, Saravanan M, Baruah L, Prasad CS. 2014. Effects of graded levels of tannin-containing tropical tree leaves on in vitro rumen fermentation, total protozoa and methane production. J Appl Microbiol. 118:557–564.
- Bhatta R, Saravanan M, Baruah L, Sampath KT, Prasad CS. 2013. Effect of plant secondary compounds on in vitro methane, ammonia production and ruminal protozoa population. J Appl Microbiol. 115:455–465.
- Bhatta R, Saravanan M, Baruah L, TSampath K. 2012. Nutrient content, in vitro ruminal fermentation characteristics and methane reduction potential of tropical tannin-containing leaves. J Sci Food Agric. 92:2929–2935.
- Bhatta R, Uyeno Y, Tajima K, Takenaka A, Yabumoto Y, Nonaka I, Enishi O, Kurihara M. 2009. Difference in the nature of tannins on in vitro ruminal methane and volatile fatty acid production and on methanogenic archaea and protozoal populations. J Dairy Sci. 92:5512–5522.
- Bouchard K, Wittenberg KM, Legesse G, Krause DO, Khafipour E, Buckley KE, Ominski KH. 2015. Comparison of feed intake, body weight gain, enteric methane emission and relative abundance of rumen microbes in steers fed sainfoin and lucerne silages under western Canadian conditions. Grass Forage Sci. 70:116–129.
- Canini A, Alesiani D, Arkangelo G, Tagliatesta P. 2007. Gaschromatography–mass spectrometry analysis of phenolic compounds from Carica papaya L. leaf. J Food Comp Anal. 20:584–590.
- Cabiddu A, Salis L, Tweed JK, Molle G, Decandiaa M, Lee M. 2010. The influence of plant polyphenols on lipolysis and biohydrogenation in dried forages at different phenological stages: in vitro study. J Sci Food Agric. 90:829–835.
- Castagninoa PS, Messanaa JD, Fiorentini G, De Jesusa RB, San Vitoa E, Carvalhoa IPC, Berchielli TT. 2015. Glycerol combined with oils did not limit biohydrogenation of unsaturated fatty acid but reduced methane production in vitro. Anim Feed Sci Technol. 201:14–24.
- Chilliard Y, Glasser F, Ferlay A, Bernard L, Rouel J, Doreau M. 2007. Diet, rumen biohydrogenation and nutritional quality of cow and goat milk fat. Eur J Lipid Sci Technol. 109:828–855.
- Durmic Z, McSweeney CS, Kemp GW, Hutton PP, Wallace RJ, Vercoe PE. 2008. Australian plants with potential to inhibit bacteria and processes involved in ruminal biohydrogenation of fatty acids. Anim Feed Sci Technol. 145:271–284.
- Ebrahimi M, Rajion MA, Goh YM. 2014. Effects of oils rich in linoleic and α-linolenic acids on fatty acid profile and gene expression in goat meat. Nutrients. 6:3913–3928.
- Fievez V, Babayemi OJ, Demeyer D. 2005. Estimation of direct and indirect gas production in syringes: A tool to estimate short chain fatty acid production that requires minimal laboratory facilities. Anim Feed Sci Technol. 123:197–210.
- Fievez V, Vlaeminck B, Jenkins T, Enjalbert F, Doreau M. 2007. Assessing rumen biohydrogenation and its manipulation in vivo, in vitro and in situ. Eur J Lipid Sci Technol. 109:740–756.
- Folch J, Lees M, Sloane Stanely GH. 1957. A simple method for the isolation and purification of total lipids from animal tissues. J Biol Chem. 226:497–450.
- Gunal M, Ishlak A, Abu Ghazaleh AA, Khattab W. 2014. Essential oils effect on rumen fermentation and biohydrogenation under in vitro conditions. Czech J Anim Sci. 10:450–459.
- Harfoot G, Hazlewood GP. 1997. Lipid metabolism in the rumen. In: Hobson PN, editor. The rumen microbial ecosystem. London (UK): Elsevier. p. 382–426.
- Jayanegara A, Kreuzer M, Leiber F. 2012. Ruminal disappearance of polyunsaturated fatty acids and appearance of biohydrogenation products when incubating linseed oil with alpine forage plant species in vitro. Livestock Sci. 147:104–112.
- Jayanegara A, Goel G, Makkar HPS, Becker K. 2011. Reduction in methane emissions from ruminants by plant secondary metabolites: effects of polyphenols and saponins. Rome: FAO. 151–157.
- Kemp PLD. 1984. Hydrogenation in vitro of alpha-linolenic acid to stearic acid by mixed cultures of pure strains of rumen bacteria. J Genera Microbiol. 130:527–533.
- Kim JE, Sharon AH, Michael RF, Nigel DS. 2010. Dietary transformation of lipid in the rumen microbial ecosystem. Asian-Aust. J Anim Sci. 22:1341–1350.
- Koike S, Kobayashi Y. 2001. Development and use of competitive PCR assays for the rumen cellulolytic bacteria: Fibrobacter succinogenes, Ruminococcus albus and Ruminococcus flavefaciens. FEMS Microbiol Lett. 204:361–366.
- Liu H, Vaddella V, Zhou D. 2012. Effects of chestnut tannins and coconut oil on growth performance, methane emission, ruminal fermentation, and microbial populations in sheep. J Dairy Sci. 94:6069–6077.
- Machmuller A, Soliva CR, Kreuzer M. 2003. Methane-suppressing effect of myristic acid in sheep as affected by dietary calcium and forage proportion. Br J Nutr. 3:529–540.
- Maia MRG, Chaudhary LC, Figueres L, Wallace RJ. 2007. Metabolism of polyunsaturated fatty acids and their toxicity to the microflora of the rumen. Antonie Van Leeuwenhoek. 91:303–314.
- Maisarah AM, Asmah R, Fauziah O. 2014. Proximate analysis, antioxidant and antiproliferative activities of different parts of Carica papaya. J Nutr Food Sci. 4:2.
- Makkar HPS, Becker K. 2003. Nutrional value and whole and ethanol antinutritional components of extracted Moringa oleifera leaves. Anim Feed Sci Technol. 63:211–228.
- Newbold CJ, Lassalas B, Jouany JP. 1995. The importance of methanogens associated with ciliate protozoa in ruminal methane production in vitro. Lett Appl Microbiol. 21:230–234.
- Porter LJ, Hrstich BG. 1986. The conversion of proantocyadins and prodelphinidin stocyanidin and delphinidin. Phytochemtry. 25:223–230.
- Rajion MA, McLean JG, Cahill RNP. 1985. Essential fatty acids in the fetal and newborn lamb. Aust J Biol Sci. 38:33–40.
- Samanta AK, Singh KK, Das MM, Maity SB, Kundu SS. 2003. Effect of complete feed block on nutrient utilization and rumen fermentation in Barbari goats. Small Ruminant Res. 48:95–102.
- Solorzano L. 1969. Determination of ammonia in natural waters by the phenolhypochlorite method. Limnol Oceanogr. 14:799–801.
- Sylvester JT, Karnati SKR, Yu Z, Morrison M, Firkins JL. 2004. Development of an assay to quantify rumen ciliate protozoal biomass in cows using real-time PCR. J Nutr. 134:3378–3380.
- Tan HY, Sieo CC, Abdullah N, Liang JB, Huang JD, Ho YW. 2011. Effects of condensed tannins from Leucaena on methane production, rumen fermentation and populations of methanogens and protozoa in vitro. Anim Feed Sci Technol. 169:185–193.
- Vasta V, Makkar HPS, Mele M, Priolo A. 2009. Ruminal biohydrogenation as affected by tannins in vitro. Br J Nutr. 102:82–92.
- Vasta V, Yanez-Ruiz DR, Mele M, Serra A, Luciano G, Lanza M, Biondi L, Priolo A. 2010. Bacterial and protozoal communities and fatty acid profile in the rumen of sheep fed a diet containing added tannins. Appl Environ Microbiol. 76:2549–2555.
- Van Soest PJ, Robertson JB, Lewis BA. 1991. Methods for dietary fiber, neutral detergent fiber, and nonstarch polysaccharides in relation to animal nutrition. J Dairy Sci. 74:3583–3597.
- Wanapat M, Kang S, Polyorach S. 2013. Development of feeding systems and strategies of supplementation to enhance rumen fermentation and ruminant production in the tropics. J Anim Sci Biotechnol. 4:32.
- Zhang CM, Guo YQ, Yuan ZP, Wu YM, Wang JK, Liu JX, Zhu WY. 2008. Effect of octadeca carbon fatty acids on microbial fermentation, methanogenesis and microbial flora in vitro. Anim Feed Sci Technol. 146:259–269.
- Zmora P, Cieslak A, Pers-Kamczyc E, Nowak A, Szczechowiak J, Szumacher-Strabel M. 2012. Effect of Mentha piperita L. on in vitro rumen methanogenesis and fermentation. Acta Agric. 62:46–52.