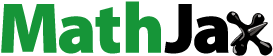
Abstract
To elucidate the differences of various sugar sources and doses on rice straw silage, the effect of molasses (M, direct substrate) and fibrolytic enzyme (E, indirect substrate) with four doses on fermentation dynamics, carbohydrates and in vitro digestibility was evaluated. Molasses (0, 2, 3 and 4% of fresh weight) or fibrolytic enzyme (0, 0.02, 0.04 and 0.06%) were applied to fresh rice straw ensiling for 3, 6, 9, 15, 30 and 60 days. All additives increased lactic acid content and lactic/acetic acid, reduced pH, NH3-N content of resulting silage (p < .05). The highest lactic acids, residual sugar and fibre degradation were observed in 0.06%E (p < .05). M-treated silage had higher dry matter (DM) content, in vitro digestibility of DM and neutral detergent fibre, and less DM loss, acetic acid, butyric acid and in vitro gas production (GP) than other treatments. Both sugar sources effectively improved fermentation quality. Fibrolytic enzyme induced free sugar release from fibre, especially from hemicellulose, while molasses showed a potential dose effect to decrease ruminal energy waste by increasing digestibility and decreasing GP in vitro. 3%M, 4%M and 0.04%E produced moderate silage based on V-score, and 4%M is recommended for rice straw silage, indicated by intermediate fermentation quality and higher in vitro digestibility.
Low WSC limited fermentation
Sugar sources reduced fibre with various mechanisms
Molasses decreased in vitro gas production with a dose effect
Highlights
Introduction
Rice (Oryza sativa, L.) harvesting produces the largest amount of crop residues worldwide each year. Approximately 21Mt yr−1 of rice straw was produced accounting for 47% of the total crop residue in China (Chen Citation2016). However, plenty of rice straw has been left unused or burnt directly resulting in environmental concerns, e.g. haze, which indicates an urgent need for new methodologies for rice straw disposal. Ensiling, as a promising processing technology, is applicable for various climates and has been used to treat straw waste and supply year-round availability of feeds (Kim Citation2006). However, rice straw is difficult to ensile due to low sugar and high fibre content. As such, great efforts have been made to improve the quality of straw silage by supplying fermented sugar by all means (Zhang et al. Citation2010; Li et al. Citation2017).
In general, sugar supplied in silage production, in its essence, can be divided into two main types: direct substrates and indirect substrates. Among them, molasses and fibrolytic enzymes are the most commonly used sugar sources in straw silage. Molasses is a by-product of sugar industries and rich in soluble carbohydrates, especially for sucrose, which directly provides a low-cost substrate for lactic acid bacteria (LAB) and compensates for the sugar deficiency of rice straw. Lignocellulose, especially cellulose and hemicellulose, is a potentially available carbon source since this polyose can be degraded into free sugars by enzymic hydrolysis (Yang et al. Citation2017; Blümmel et al. Citation2018). Thus, fibrolytic enzyme is applied to break down fibre and release soluble sugar, which provides fermentable substrate indirectly. Although both as sugar supplementation, the mechanisms of molasses and enzymes acting on silage differ. Possible differences between these two sugar sources are unclear and need to be studied, however, there is little available information until now.
The optimal addition doses of molasses and fibrolytic enzyme to rice straw silage is evaluated in the study respectively. Furthermore, comparative analysis of these two sugar sources on fermentation dynamics, carbohydrates and in vitro digestibility was also carried out.
Materials and methods
Animal care
The experimental protocol was approved by the Animal Care and Use Committee of the Shanxi Agriculture University and performed according to recommendations proposed by the European Commission (1997) to minimise the suffering of animals.
Sugar-source additives
Molasses (M, cane molasses) was obtained from JiaFurui Biological Technology Co., Ltd. (Nanjing, Jiangsu, China). Fibrolytic enzyme (E, the 1:1 mix of cellulase and hemicellulase, Solarbio Technology Co., Ltd., Beijing, China) was used with cellulase activity of 3000 U/g and hemicellulase activity of 1500U/g specified by the manufacturer.
Materials collection
Rice was cultivated in Nanjing Branch of Chinese National Centre for Rice Improvement in Jiangsu Academy of Agricultural Science (32.04°N, 118.88°E, 20 m asl, annual mean temperature 15.4 °C and average annual precipitation 1106.5 mm, Jiangsu, China). The pH of the paddy soil was 6.5, total N, K and P contents were 2.04, 2.75 and 1.01 g/kg, respectively. Fresh rice straw was collected after grain harvest on 21 October 2016, leaving the stubble of 10 cm.
Silage preparation
The straw was chopped into about 2 cm in length with a fodder chopper. After manual mixing, the chopped rice straw was treated with 0, 2, 3 and 4% fresh weight (FW) of molasses or 0, 0.02, 0.04 and 0.06% FW of fibrolytic enzyme, respectively. 20 mL kg−1 FW additives or deionised water was sprayed mixed into the chopped material. Then, approximately 520 g treated material was tightly packed into minisilos (1 L polyethylene bottle with a diameter of 9.5 cm and height of 18.7 cm, Lantian biological experimental instrument Co., Ltd, Jiangsu, China) and stored at the ambient temperature (22–28 °C) after being sealed with screw tops and plastic tape. Five silos per treatment were opened on 3, 6, 9, 15, 30 and 60 days after ensiling, respectively.
Chemical and microbial analyses
Analysis of raw material
The raw material was sampled for the determination of crude protein (CP), buffering capacity (BC) and the counts of epiphytic microorganisms immediately. Total nitrogen (TN) was determined by Kjeldahl nitrogen analyser (Kjeltec 8200; FOSS, Sweden), and the CP was calculated as TN × 6.25. The BC was determined following the method described by Playne and Mcdonald (Citation1966). The plate counting method and the colony-forming units (CFU) were used for the enumeration of epiphytic microorganism population. The samples (10 g) homogenised with 90 mL sterilised saline solution (8.50 g/L NaCl) was serially diluted from 10−1 to 10−6. The LAB, aerobic bacteria, moulds and yeasts were counted on de Man, Rogosa and Sharpe (MRS) agar medium, nutrient agar medium and potato dextrose agar medium at 37 °C for 2–3 days, respectively. The ensilability of rice straw was assessed by calculating the fermentation coefficient (FC) according to the formula described by Yitbarek and Tamir (Citation2014).
Analysis of extract samples
Fresh or ensiled rice straw was divided into two subsamples. The first subsample was homogenised with distilled water at a ratio of 1:3 and stored at 4 °C for 24 h. Then, the extracts were filtered through two layers of cheesecloth and a Whatman filter paper (pore size of 11 µm, Xinhua Co., Hangzhou, China). The pH was measured with a glass electrode pH metre (HANNA pH 211; Hanna Instruments Italia Srl, Villafranca Padovana, Italy). The ammonia nitrogen (NH3-N) was determined by the phenol-hypochlorite reaction method of Broderick and Kang (Citation1980). The contents of organic acids and ethanol were analysed in high performance liquid chromatography system (1260 HPLC, Agilent Technologies, Inc., Waldbronn, Germany) equipped with a refractive index detector (column: Carbomix® H-NP5, Sepax Technologies, Inc., Newark, DE, USA; eluent: 2.5 mM H2SO4, 0.5 mL/min; temperature: 55 °C).
The V-score method (Takahashi et al. Citation2005) was adopted to evaluate the silage quality using a 100-point scale as below: < 60 (bad), 60–80 (moderate) and 81–100 (good). V-score = YN + YA +YB, where YN is calculated from the NH3-N content (% TN), YA is calculated from the acetate + propionate contents (% DM), and YB is calculated from the butyrate content (% DM).
Analysis of solid samples
The second subsample was freeze-dried to determine dry matter (DM) content by a vacuum freeze dryer (Freeze Dryer-1A-50, Biocool Laboratory Instrument Co., Ltd, Beijing, China). DM loss was measured using the formula of Li et al. (Citation2017). The solid samples were ground to pass 1-mm screen with laboratory knife mills (FW100, Taisite Instrument Co., Ltd., Tianjin, China) and stored for later analysis of carbohydrate components. The contents of neutral detergent fibre (NDF), acid detergent fibre (ADF) and acid detergent lignin (ADL) were measured by ANKOM 200i fibre analyser (ANKOM Technologies, Inc., Fairport, NY, USA). The water-soluble carbohydrates (WSC) was determined via the modified method of the phenol-sulfuric acid method (Thomas Citation1977). The monosaccharide compositions (glucose, xylose and fructose) were determined according to the method of Desta et al. (Citation2016). Further analysis of CP and ash contents were carried out in 60-day silage samples. Crude ash was measured by incinerating in a muffle furnace at 550 °C for 4 h.
In vitro incubation of 60-day silage
The rumen fluid was collected from the rumens (different positions) of two Holstein cows before morning feeding. The cows were fed the diet based on 50% corn silage and 50% concentrate at 1.2 times of the maintenance level (Feng and Lu Citation2007). Rumen fluid was filtered, moved to the laboratory, and stored at 39 °C in a water bath immediately. Before use, the rumen fluid was mixed with a buffer solution at the ratio of 1:2 (v/v) as described by Menke (Citation1988). The whole operation process was carried out under continuous flushing with CO2.
In vitro fermentation was conducted in serum bottles following the Contreras-Govea et al. (Citation2011) method with some modifications. Ground samples (1 g) were placed in filter bags (F57; ANKOM Technology, Macedon, NY, USA) that were washed with acetone, dried at 55 °C for 24 h and weighted previously. Then each bag was heat-sealed and put into each preheated serum bottle (120 mL capacity) with 60 mL inoculum under CO2 at 39 °C. The blank was 3 serum bottles with the only inoculum added. The gas volume was measured at 4, 8, 12, 24, 48 and 72 h of incubation by pressure transducer technique and corrected with blank bottles. After incubation, the residual samples were gently rinsed with cold tap water and dried at 65 °C for 48 h to determine in vitro degradability of dry matter (IVDMD), neutral detergent fibre (IVNDFD) and acid detergent fibre (IVADFD). IVDMD, IVNDFD and IVADFD were calculated based on the differences in their respective weight before and after incubation.
Cumulative gas production (GP) data were fitted to the non-linear equation: Y = b (1 − e−ct), where Y is the volume of gas produced at time t, b is the potential GP (mL), and c is the rate constant of GP (Blümmel et al. Citation2003).
Statistical analysis
Microbial data was transformed to the log10 on a fresh weight basis. Data on fermentation dynamics and chemical composition were subjected to two-way analysis of variance (ANOVA) with the fixed effects of treatments, ensiling days and treatments × ensiling days, and the data of in vitro digestibility was subjected to one-way ANOVA using the general linear model (GLM) procedure of SAS rev. 9.1 (SAS Institute, Cary, NC, USA). Tukey’s multiple comparison was used to determine the statistical differences between means, and the level of significance was set at p < .05.
Results
Chemical and microbial composition of rice straw
The fresh rice straw had DM of 445.71 g/kg FW, CP of 62.59 g/kg DM, WSC of 53.23 g/kg DM and pH value of 6.59. The monosaccharide constituents were glucose of 13.86 g/kg DM and fructose of 15.08 g/kg DM. The cellulose and hemicellulose were 322.29 and 225.94 g/kg DM, respectively. The buffering capacity (BC) and fermentation coefficient (FC) was 41.22 mEq/kg DM and 54.90, respectively. The epiphytic LAB on rice straw were less than 1.0 105 cfu/g FW. The counts of aerobic bacteria (6.39 lg cfu/g FW) were higher than that for moulds and yeasts (total 4.65 lg cfu/g FW), which were more than 1.0
106 cfu/g FW (Table ).
Table 1. Chemical composition, pre-ensiled characteristics and bacterial population of raw materials.
Fermentation dynamics of rice straw silage
The fermentation dynamics of rice straw silage are presented in Table . Treatments, ensiling days and their interaction significantly affected the silage pH, organic acids and ethanol content (p < .05). The control silage always had high pH, acetic acid (AA) and butyric acid (BA) content, low LA and ratio of lactic acid to acetic acid (LA/AA). The pH values of additive-treated silage showed a significant reduction initially and then showed a slow increase trend. Molasses addition induced a more rapid decline in pH than enzyme during the first 9 days of ensiling, but then the pH of E-treated silage dropped to a lower value. Correspondingly, the LA content in all silage soared to reach a peak at day 30 then followed by a sharp drop (p < .05). The highest LA content was recorded in 0.06%E silage at day 30 with the value of 84.85 g/kg DM. All additives significantly (p < .05) decreased AA content except for 0.02%E and 0.04%E. E-treated silage had numerically or statistically higher AA content than M-treated silage during ensiling. Additives application significantly increased LA/AA of resulting silage, of these, LA/AA was further increased with increasing addition doses (p < .05). Negligible amounts of BA were observed in all silage, except for the control (2.19 g/kg DM). Propionic acid and valeric acid was not detected along the ensiling process (data not shown). High concentration of ethanol was detected in all silage, and M-treated silage always remained a relatively low level until the end of ensiling as compared with other treatments.
Table 2. Effect of additives and ensiling days on pH, organic acids and ethanol composition of rice straw silage.
Table illustrates the dynamic changes of DM, DM loss and NH3-N of rice straw silage. DM loss and NH3-N were significantly affected by additives, ensiling days and their interaction (p < .05). Both molasses and fibrolytic enzyme decreased DM loss of rice straw silage, and DM loss was further reduced with the increasing doses (p < .05). M-treated silage showed higher DM content and the lower DM loss than did the control and E-treated silage (p < .05). All silage produced high NH3-N content (>100 g/kg DM) at the end of ensiling. Furthermore, the NH3-N was even higher in 3%M or 4%M silage than in the control silage during the first 9 days of ensiling. As indicated by V-scores, the 3%M, 4%M and 0.04%E silage were moderate quality silage (Figure ).
Figure 1. V-score of 60-day rice straw silage (n = 5, bars indicate standard error of the means). Control, no additive; 2%M, 2% molasses; 3%M, 3% molasses; 4%M, 4% molasses; 0.02%E, 0.02% fibrolytic enzyme; 0.04%E, 0.04% fibrolytic enzyme; 0.06%E, 0.06% fibrolytic enzyme. Means with different small letters shows significant differences among treatments at p < .05.
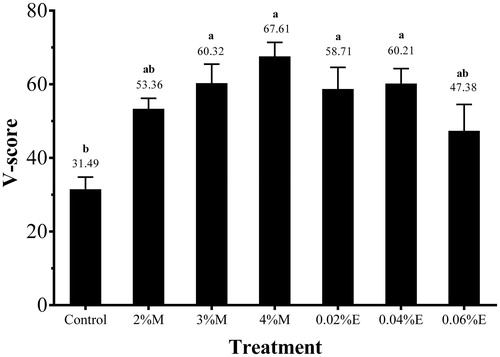
Table 3. Effect of additives and ensiling days on dry matter, dry matter loss and NH3-N content of rice straw silage.
Structural carbohydrate compositions of rice straw silage
Structural carbohydrate compositions of rice straw silage are listed in Table . The effects of treatments and ensiling days on structural carbohydrates were notable (p < .05), except ADL content. The interaction between treatments and ensiling days significantly affected the silage NDF and hemicellulose (p < .05). Except NDF and hemicellulose in 0.06%E, the other measured structural carbohydrate performed a continuously increase throughout the ensiling. All additive-treated silage exhibited numerically (p > .05) or statistically (p < .05) lower content of NDF, ADF, cellulose and hemicellulose than the control. The increased doses of molasses or fibrolytic enzyme resulted in further decreased proportion of structural carbohydrates in silage. After 60 days of ensiling, the minimum content of NDF, cellulose and hemicellulose were detected in 0.06%E silage.
Table 4. Effect of additives and ensiling days on structural carbohydrates composition of rice straw silage.
Non-structural carbohydrate compositions of rice straw silage
The dynamic changes of WSC, glucose, fructose and xylose are given in Figure . Treatments, ensiling days and their interaction significantly affected these soluble sugars in rice straw silage (p < .05). All non-structural carbohydrates declined substantially during ensiling (p < .05), except for xylose. Xylose content showed an upward and then downward tendency with always high value in E-treated silage (p < .05). Both molasses and fibrolytic enzyme increased non-structural carbohydrate content with a dose-effect (p < .05). The highest WSC content was recorded in 4%M silage at the end of ensiling. E-treated silage had significantly higher content of glucose, fructose and xylose than M-treated silage during ensiling (p < .05).
Figure 2. Dynamic changes of (A) water soluble carbohydrates, (B) glucose, (C) fructose and (D) xylose during fermentation with different treatments (n = 5, bars indicate standard error of the means). Control, no additive; 2%M, 2% molasses; 3%M, 3% molasses; 4%M, 4% molasses; 0.02%E, 0.02% fibrolytic enzyme; 0.04%E, 0.04% fibrolytic enzyme; 0.06%E, 0.06% fibrolytic enzyme. Treatments, ensiling days and their interaction were significant at p < .05.
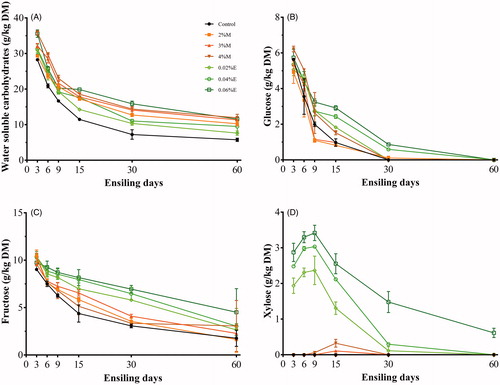
CP, ash and in vitro parameters of 60-day rice straw silage
As shown in Table , the E-treated silage had significantly higher (p < .05) CP content than other silage, with the highest value in 0.04%E followed by 0.02%E then 0.06%E. The ash content of M-treated silage was lower (p < .05) than that of other treatments, and there were no significant differences among 2%M, 3%M and 4%M silage. 4%M addition improved IVDMD and IVNDFD of resulting silage, while E addition reduced IVADFD (p < .05).
Table 5. Crude protein, crude ash, gas production kinetics and in vitro degradability of rice straw silage after 60 days of ensiling.
For GP24, significant differences were observed between molasses and fibrolytic enzyme treatments (p < .05). There were no significant differences among the control, 2%M and 0.02%E, while as the additive dose increased, the M-treated silage reduced GP24, and the E-treated silage increased GP24 (Table , Figure ). Similar trend could be found in potential GP with lower b value in 4%M while higher in 0.06%E. GP72 declined in M-treated silage and the value was less when molasses was added up to 4%.
Figure 3. Gas production profiles (mL/g DM) from in vitro fermentation of rice straw silage for 72 h (n = 5). DM: dry matter; Control, no additive; 2%M, 2% molasses; 3%M, 3% molasses; 4%M, 4% molasses; 0.02%E, 0.02% fibrolytic enzyme; 0.04%E, 0.04% fibrolytic enzyme; 0.06%E, 0.06% fibrolytic enzyme. Treatments, incubation time and their interaction were significant at p < .05.
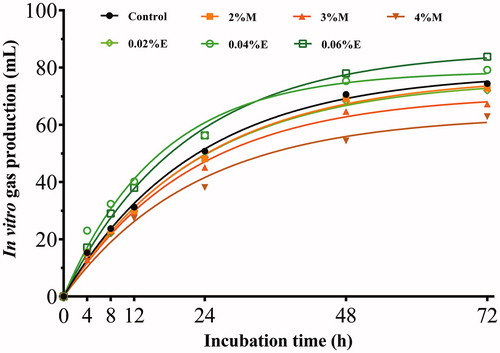
Discussion
Analysis of raw material
The rice straw used in this study contains high FC (54.90), low BC, relative high DM content, which is suitable for ensilage theoretically (Mcdonald et al. Citation1991). However, the epiphytic LAB on rice straw is too low (<1.0 × 105 cfu/g FW) to dominate fermentation (Cai et al. Citation1999). Furthermore, the rice straw had unsuitable carbohydrate proportion, with low WSC content accounting for approximately 5% of DM, and high NDF and ADF for approximately 60 and 38% of DM, respectively. Consequently, rice straw is of difficulty for long-term preservation through natural fermentation.
Analysis of fermentation quality of rice straw silage
Although molasses addition accelerated the production of lactic acid in the early stage of ensiling, the accumulation of lactic acid in the mid-late stage of ensiling was weak, leading to less final content than those observed in enzyme treatments. Therefore, pH value of M-treated silage was lower first then higher than that of E-treated silage. It is possible that molasses as direct substrate addition accelerated the proliferation of undesired microorganism and consumption of available sugar, which leads to insufficient sugar supply for LAB in the mid-late stages of ensiling. Differently, free sugar produced by enzyme hydrolysis supported enough carbon source for LAB to utilise. The higher AA content in E-treated silage may be explained by the pentose released from enzymolysis of hemicellulose (Niimi and Kawamura Citation1998). Generally, pentose can convert to D-xylos-5-phosphate which is then fermented to lactic and acetic acids (Mcdonald et al. Citation1991). LA/AA decreased after 9 days in M-treated silage and 15 days in E-treated silage. These differences could be speculated to the different sugar supply mechanism. The gentle but steady release of fibre-based sugar prolonged fermentation process. A more rapid shift from homofermentation to heterofermentation was occurred in M-treated silage than in E-treated silage.
High levels of ethanol were detected in all silage and this could be explained by the high DM content and yeasts counts of rice straw. Ethanol is commonly formed in high DM silage (Hengeveld Citation1983). Moreover, less ethanol in M-treated silage may be related to an inhibition of yeasts resulting from lower pH in M treatments during the early stage of ensiling (0–9 days). Trace amounts of BA were detected in all silage except for the control, suggesting that low pH in additive-treated silage inhibited the clostridial fermentation. Clostridial fermentation signified protein degradation, DM loss and energy wastage. Similarly, high NH3-N content and DM loss was also observed in the control silage (p < .05). M-treated silage, especially 4%M silage, had abnormally high NH3-N content during the first 9 days of ensiling. It could be speculated that direct sugar application promoted the growth of LAB as well as proteolytic bacterium during the early stage of ensiling. The DM content showed a continuous downtrend, with considerable DM loss recorded in control silage followed by E-treated silage. This could be attributed to the breakdown of nutrients caused by clostridial spoilage or heterofermentation (Mcdonald et al. Citation1991), as indicated by higher BA and AA in these silages. Jaakkola et al. (Citation1991) also reported that fibrolytic enzyme addition had greater DM loss than that of other additives.
Analysis of structural carbohydrates compositions of rice straw silage
The ensiled rice straw had higher structural carbohydrate contents than fresh rice straw (Table ), probably due to the DM loss, which primarily evolved from non-fibre fractions. Both sugar sources reduced the structural carbohydrate contents compared with the control in this study. This needed to be discussed separately. Molasses addition increased silage DM and thus relatively reduced the proportion of structured carbohydrates since molasses itself is high in dry matter and low in fibre. As for fibrolytic enzyme treatment, enzymatic degradation of cellulose and hemicellulose occurred during the early and middle stage of ensiling. Moreover, the hydrolysis evinced by organic acid (particularly lactic acid) during ensiling is also considered. Dewar et al. (Citation1963) concluded that after ensiling of 7–28 days, structural carbohydrates could be degraded by acid hydrolysis at low pH. The ADL content exhibited an uptrend over the course of ensiling, which could be explained by the high loss of DM. In fact, ensilage cannot affect the ADL content.
Analysis of non-structural carbohydrates compositions of rice straw silage
Within the initial 3 days of ensiling, the maximum consumption of WSC (Figure ) was accompanied by small amounts of fermentation products (Table ) in the control, suggesting that the readily available substrates in this treatment were mainly consumed by plant respiration and aerobic bacteria.
The significantly higher glucose and fructose in E-treated silage after 15 days of ensiling could be attributable to the low pH in these silages. Sufficient acid environment inhibited microbial consumption of sugar and caused acidolysis of available structural carbohydrates. Hemicellulose, also known as polyose, is formed by various components including a backbone of xylans and arabinose side chains (Yang et al. Citation2017), which can be decomposed into xylose mainly. Thus, the production of xylose during ensiling is a good indicator of hemicellulose degradation. Fibrolytic enzyme addition significantly increased xylose content during the first 9 days of ensiling, then decreased to near zero. This result indicated that the enzyme (hemicellulase) was functioned in this period until being inhibited under low pH. Trace xylose produced in 3%M and 4%M silage could attribute to slight acidolysis of hemicellulose. Similar to the study of Shao et al. (Citation2002), the larger reduction was observed in glucose than in other sugars. This result indicated that glucose could be the optimal fermentation substrate for LAB during ensiling.
Analysis of CP, ash and in vitro parameters of 60-day rice straw silage
Low CP content observed in the control could be attributed to the uncontrollable growth of proteolytic bacteria during ensiling. Higher CP observed in E-treated silage could ascribe to the high DM loss and relatively low NH3-N. The fluctuation of ash content could be related to DM loss since ash was expressed as a percentage of DM.
Digestibility has gained wide acceptance of the evaluation of feed nutritional value and intake (Huhtanen et al. Citation2007). Molasses addition, notably 4%M, enhanced IVDMD of rice straw silage. This might be due to the less DM loss occurred in M treatments. More easily digestible constituents, such as WSC and CP, as part of the DM, are remained before in vitro incubation. NDF comprises mainly cellulose, hemicellulose and lignin, and other minor components (silicate and protein), which is constituents of the plant cell wall. Previous study showed a positive correlation between hemicellulose content and cell wall digestibility (Riboulet et al. Citation2008). Hemicellulose is known to be easily digested than other cell wall components due to its amorphous structure and much lower polymerisation level. Similarly, higher hemicellulose content resulting in higher amounts of NDF digested was found in this study. Lower digested amount of NDF and ADF observed in 0.06%E silage could be explained that cellulose and hemicellulose were predegraded by the enzyme, and then less NDF and ADF was available for rumen microorganisms. This observation is in line with the result of our previous study that cellulose is increasing resistant after enzyme hydrolysis (Zhao et al. Citation2018).
In vitro gas production is commonly used as an indicator for efficiency of rumen degradability and predicts the metabolisable energy of animal feed (Contreras-Govea et al. Citation2011). In this study, GP24, GP72 and potential GP of E-treated silage were increased with the increasing doses of fibrolytic enzyme, probably due to higher dose of the enzyme, indirectly but effectively, released more fermentable sugars which were available for rumen microorganisms during in vitro incubation (Bayatkouhsar et al. Citation2012).
Interestingly, molasses addition decreased GP of resulting silage as compared with the control. Furthermore, molasses addition from 2% to 4% enhanced suppression effect on GP. This result is difficult to explain but probably due to the M-treated silage altered ruminal short-chain fatty acid (SCFA) production even ruminal fermentation patterns. Yulistiani et al. (Citation2007) reported that adding molasses increased ruminal propionic acid proportion, decreased acetic/propionic acid and GP of fermented fibrous material in an in vitro trial. Also, the study of Xia et al. (Citation2018) followed a similar result with higher propionic acid content and lower gas production in M-treated wheat silage. It is known that rumen gas, such as methane and hydrogen, can be reduced by the shift of ruminal fermentation pattern from acetic to propionic type (O'Mara Citation2004). Thus, further study on ruminal SCFA is needed to clarify and explain this phenomenon.
Conclusions
Molasses or fibrolytic enzyme with appropriate dose is necessary to avoid spoilage and enhance the fermentation stability of rice straw silage. The effects of these two sugar sources on fermentation quality in rice straw silage were comparable. Molasses addition enhanced digestibility while reducing ruminal gas production in vitro. Fibrolytic enzyme addition improved fibre degradation and utilisation but had high DM loss. Molasses was more effective than fibrolytic enzyme to improve the silage quality of rice straw, and 4% molasses is recommended for rice straw silage by comprehensive consideration. However, high level of NH3-N detected in all silage should also be considered when applied to practice.
Disclosure statement
No potential conflict of interest was reported by the authors.
Additional information
Funding
References
- Bayatkouhsar J, Tahmasbi AM, Naserian AA. 2012. Effects of microbial inoculant on composition, aerobic stability, in situ ruminal degradability and in vitro gas production of corn silage. Int J Agri Sci. 2:774–786.
- Blümmel M, Karsli A, Russell JR. 2003. Influence of diet on growth yields of rumen micro-organisms in vitro and in vivo: influence on growth yield of variable carbon fluxes to fermentation products. Br J Nutr. 90:625–634.
- Blümmel M, Teymouri F, Moore J, Nielson C, Videto J, Kodukula P, Pothu S, Devulapalli R, Varijakshapanicker P. 2018. Ammonia Fiber Expansion (AFEX) as spin off technology from 2nd generation biofuel for upgrading cereal straws and stovers for livestock feed. Anim Feed Sci Technol. 236:178–186.
- Broderick GA, Kang JH. 1980. Automated simultaneous determination of ammonia and total amino acids in ruminal fluid and in vitro media. J Dairy Sci. 63:64–75.
- Cai Y, Benno Y, Ogawa M, Kumai S. 1999. Effect of applying lactic acid bacteria isolated from forage crops on fermentation characteristics and aerobic deterioration of silage. J Dairy Sci. 82:520–526.
- Chen XG. 2016. Economic potential of biomass supply from crop residues in China. Appl Energ. 166:141–149.
- Contreras-Govea FE, Muck RE, Mertens DR, Weimer PJ. 2011. Microbial inoculant effects on silage and in vitro ruminal fermentation, and microbial biomass estimation for alfalfa, bmr corn, and corn silages. Anim Feed Sci Technol. 163:2–10.
- Desta ST, Yuan X, Li J, Shao T. 2016. Ensiling characteristics, structural and nonstructural carbohydrate composition and enzymatic digestibility of Napier grass ensiled with additives. Bioresour Technol. 221:447–454.
- Dewar WA, Mcdonald P, Whittenbury R. 1963. The hydrolysis of grass hemicelluloses during ensilage. J Sci Food Agric. 14:411–417.
- Feng YL, Lu ZN. 2007. The cow nutritional needs and feed ingredients. Beijing, China: China Agricultural Press.
- Hengeveld AG. 1983. Spores of butyric acid bacteria in silage. Wageningen, Holland: Wageningen University. Dutch.
- Huhtanen P, Rinne M, Nousiainen J. 2007. Evaluation of the factors affecting silage intake of dairy cows: a revision of the relative silage dry-matter intake index. Animal. 1:758–770.
- Jaakkola S, Huhtanen P, Hissa K. 1991. The effect of cell wall degrading enzymes or formic acid on fermentation quality and on digestion of grass silage by cattle. Grass Forage Sci. 46:75–87.
- Kim JG, Chung ES, Hum JS, Yoon SH, Lim YC, Seo S. 2006. Development of lactic acid bacteria inoculant for whole crop rice silage in Korea. In: International Symposium on Production and Utilization of Whole Crop Rice for Feed; Busan (Korea). pp. 77–82.
- Li P, Ji S, Wang Q, Qin M, Hou C, Shen Y. 2017. Adding sweet potato vines improve the quality of rice straw silage. Anim Sci J. 88:625–632.
- Mcdonald P, Henderson AR, Heron S. 1991. The biochemistry of silage. 2nd ed. Marlow, Bucks (UK): Chalcombe Publications.
- Menke KH. 1988. Estimation of the energetic feed value obtained from chemical analysis and in vitro gas production using rumen fluid. Anim Res Dev. 28:7–55.
- Niimi M, Kawamura O. 1998. Degradation of cell wall constituents of guineagrass (Panicum maximum Jacq.) during ensiling. Grassl Sci. 43:413–417.
- O'Mara F. 2004. Greenhouse gas production from dairying: reducing methane production. Adv Dairy Technol. 16:295–309.
- Playne MJ, Mcdonald P. 1966. The buffering constituents of herbage and of silage. J Sci Food Agric. 17:264–268.
- Riboulet C, Lefevre B, Denoue D, Barriere Y. 2008. Genetic variation in maize cell wall for lignin content, lignin structure, p-hydroxycinnamic acid content, and digestibility in set of 19 lines at silage harvest maturity. Maydica. 53:11–19.
- Shao T, Ohba N, Shimojo M, Masuda Y. 2002. Dynamics of early fermentation of Italian ryegrass (Lolium multiflorum Lam.) silage. Asian Australas J Anim Sci. 15:1606–1610.
- Takahashi T, Horiguchi K, Goto M. 2005. Effect of crushing unhulled rice and the addition of fermented juice of epiphytic lactic acid bacteria on the fermentation quality of whole crop rice silage, and its digestibility and rumen fermentation status in sheep. Anim Sci J. 76:353–358.
- Thomas TA. 1977. An automated procedure for the determination of soluble carbohydrates in herbage. J Sci Food Agric. 28:639–642.
- Xia C, Liang Y, Bai S, He Y, Muhammad AUR, Su H, Cao B. 2018. Effects of harvest time and added molasses on nutritional content, ensiling characteristics and in vitro degradation of whole crop wheat. Asian-Australas J Anim Sci. 31:354–362.
- Yang Y, Zhu N, Yang J, Lin Y, Liu J, Wang R, Wang F, Yuan H. 2017. A novel bifunctional acetyl xylan esterase/arabinofuranosidase from Penicillium chrysogenum P33 enhances enzymatic hydrolysis of lignocellulose. Microb Cell Fact. 16:166.
- Yitbarek MB, Tamir B. 2014. Silage additives: review. Open J of Appl Sci. 04:258–274.
- Yulistiani D, Jelan ZA, Liang JB, Yaakub H, Abdullah N. 2007. The use of in vitro gas production technique to evaluate molasses supplementation to mulberry (Morus alba) and rice straw mixed diets. Indonesian J Anim Vet Sci. 12:255–261.
- Zhang JG, Kawamoto H, Cai YM. 2010. Relationships between the addition rates of cellulase or glucose and silage fermentation at different temperatures. Anim Sci J. 81:325–330.
- Zhao J, Dong Z, Li J, Chen L, Bai Y, Jia Y, Shao T. 2018. Ensiling as pretreatment of rice straw: the effect of hemicellulase and Lactobacillus plantarum on hemicellulose degradation and cellulose conversion. Bioresour Technol. 266:158–165.