Abstract
In the experiment, the effects of maternal dietary energy restriction on carcase, meat quality and antioxidant capacity in broilers were evaluated. A total of 400 25-weeks-old Arbour Acres broiler breeder female birds were randomly assigned to four groups (T1, T2, T3 and T4) with different dietary metabolisable energy levels (11.70, 9.36, 8.19 and 5.85 MJ kg−1). Female birds were restrictively fed same intake. Broilers from different maternal dietary energy were fed diets similar in nutrients contents during deferent growth period. Low maternal energy decreased egg weight and offspring hatch weight. T2 group got a higher thigh muscle percentage of 28-d-old offspring and a higher body weight of 49-d-old offspring than T1 group. Abdominal fat percentage of 49-d-old offspring in T3 group was increased. Low maternal energy increased intramuscular fat (IMF) contents in 28-d-old offspring breast and 49-d-old offspring thigh. 49-d-old offspring in T2 and T3 groups got lower drip loss and higher meat colour value. T2 and T3 groups had higher total superoxide dismutase and glutathione peroxidase activities in offspring muscles and lower malondialdehyde level. A higher glutathione peroxidase mRNA expression in breast muscle was found in T2 group. Maternal dietary energy restriction altered egg weight and offspring hatch weight. Appropriate reduction of maternal dietary energy made chickens fattier and increased the antioxidant capacity of offspring muscles.
It is well known that embryo development and postnatal growth are influenced by maternal nutritional supply. This paper shows that 9.36 MJ ME/kg diet for hens during laying period is better for broiler to achieve greater slaughter weight and body antioxidant capacity.
This paper indicated that maternal dietary energy restriction affected fat accumulation and antioxidant capacity of offspring. Maternal nutritional management may become a way to regulate the quality of chickens through.
Highlights
Introduction
The quality of meat products has always been a common concern of consumers. Most researchers focus on the nutrition and breeding of animals after birth, but do not pay enough attention to the factors before birth. Extensive studies suggested that offspring performance could be influenced by maternal nutritional status at specific periods (Enting et al. Citation2007; Zhu et al. Citation2012; Koppenol et al. Citation2015). Maternal diets with high energy levels increased the body weight of male offspring and the number of eggs per hen (Attia et al. Citation1993, Citation1995b). High energy allotments for male broilers increased body weight of 42-d-old offspring (Attia et al. Citation1995a). The perinatal period is a critical stage for complex foetal growth. Changes in nutrition during this period can profoundly alter metabolism, endocrine (Rehfeldt et al. Citation2004), immune status (Enting et al. Citation2007), antioxidant Defence capacity (Tarry-Adkins et al. Citation2010) and muscle development (Dauncey and Harrison Citation1996). Maternal nutritional status can alter the epigenetic state of the foetal genome and foetal programming and even produce the genomic imprinting (Wu et al. Citation2006; Ramaiyan et al. Citation2016). High level of metabolisable energy allotment had a negative effect on feed per egg and feed per chick of feed conversion efficiency (Attia et al. Citation1995b). Eventually, the stress of maternal nutrition on the foetus may lead to changes in the phenotypic characteristics of the mature progeny, such as carcase traits, meat quality and antioxidant capacity (He et al. Citation2012; Goliomytis et al. Citation2016; Li, Shan, et al. Citation2019). For poultry, foetal growth is conducted in the egg for only 21 d. The developing embryo and the hatched chick are completely dependent for their growth and development on nutrients deposited in the egg. Maternal nutrition may alter the nutrient supply to the foetus and thereby affect the foetal production of growth factors and finally affect nutrient availability to the foetus (Rehfeldt et al. Citation2004). Maternal nutrition restriction had a negative influence on the offspring’s body weight at 6 weeks of age (Van der Waaij et al. Citation2011). Female offspring of female broilers with the low crude protein intake had lighter weight than female offspring of female broilers with high crude protein during 22–36 d of age (Moraes et al. Citation2014). Liver weight of day-old chicks was affected by hen’s energy intake, which was increased as energy intake be increased (Attia et al. Citation1995b). Evidently, the influence of maternal dietary energy on broiler development via the egg may have latent and long-term effects that become manifest later in the grow-out period (Peebles et al. Citation2002). Our previous study showed that low maternal dietary intake increased the deposition of Cys in egg yolks (Li, Shan, et al. Citation2019). The energy of diet was 30% lower than normal diet would increase 49-d-old abdominal fat percentage and subcutaneous fat thickness in offspring of breeders (Li et al. Citation2014). The offspring of broiler breeders fed low energy diet had slighter higher fat and protein content of breast and thigh muscle than normal energy diet (Xu et al. Citation2010). Low dietary feed intake of hens had a significant effect on weight of 1-d-old offspring and chest muscle rate of 28-d-old offspring (Li, Yang, et al. Citation2019). In order to study the effects of maternal nutrition on the later growth stage of offspring in broilers, Arbour Acres broiler breeder hens were fed with four dietary metabolisable energy levels in this study and the growth performance, carcase, antioxidant capacity and meat quality of their offspring were detected.
Materials and methods
Birds and experimental design
A total of 400 Arbour Acres broiler breeder female birds were used in this experiment. During the growing period (from 1 d to 24 weeks), all the birds were fed the same diet (0–6 weeks: 12.12 MJ ME kg−1, 180 g kg−1 CP, 10 g kg−1 Ca; 7–18 weeks: 11.91 MJ ME kg−1, 150 g kg−1 CP, 9 g kg−1 Ca; 19–24 weeks: 11.70 MJ ME kg−1, 160 g kg−1 CP, 20 g kg−1 Ca). When laying rate reached 5% (25 weeks), the birds were randomly assigned to four groups (T1, T2, T3 and T4) and fed with different dietary metabolisable energy levels (11.70, 9.36, 8.19 and 5.85 MJ kg−1). Each dietary treatment had 10 replicates with 10 female birds per replicate. The experimental diets were formulated to meet or exceed the nutrient requirements of broiler breeders (Leeson and Summers Citation2008). Composition and nutrient levels of the broiler breeder diets are presented in Table . Daily feed and energy allotments provided for hens from 25 to 40 weeks (Figure ). All feedstuffs used for the experiment were provided by the Xiang Fang Experimental Bases of the Northeast Agricultural University. Diets for four treatments were prepared separately in an experimental feed mill with high precision mixers. Diets were mash feeds. The procedures of feed processing and the experiment were approved and supervised by the Institute of Animal Nutrition in Northeast Agricultural University.
Figure 1. Daily feed and energy allotments provided for hens from 25 to 40 weeks. ME: Metabolizable energy.
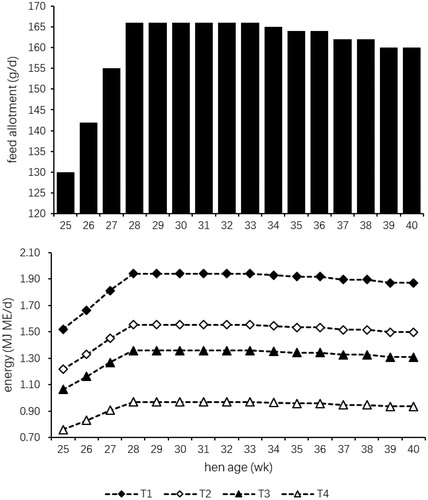
Table 1. Composition and nutrient levels of broiler breeder diets (g kg−1 or MJ kg−1 as-fed).
Management
During the experiment period, every two female birds were housed in an individual cage measuring 48 cm × 34 cm × 39 cm. All the cages are in an environmentally controlled house of the experiment station of College of Animal Science and Technology (Northeast Agricultural University, China) with temperature maintained at approximately 20 °C as possible.
All female birds were restrictively fed the same intake at 08:00 with freely access to water during the whole day (1–4 weeks: 29 g d−1; 5–8 weeks: 43.75 g d−1; 9–12 weeks: 55 g d−1; 13–17 weeks: 75 g d−1; 18–22 weeks: 103 g d−1; 23 weeks: 120 g d−1; 24 weeks: 125 g d−1; 25 weeks: 130 g d−1; 26 weeks: 142 g d−1; 27 weeks: 155 g d−1; 28–33 weeks: 166 g d−1; 34 weeks: 165 g d−1; 35–36 weeks: 164 g d−1; 37–38 weeks: 162 g d−1; 39–40 weeks: 160 g d−1). By combination of natural and artificial light, light was provided 8 h/d from 10 d to 20 weeks. At 21st week, the photoperiod was 12 h d−1 and increased by an average of 0.5 h wk−1 up to a maximum of 16 h d−1 and then maintained at 16 h d−1 thereafter. Female birds housing and handling procedures during experimentation were in accordance with the guidelines of Handbook of Modern Broiler Production (Shan Citation2001).
Artificial insemination was used in the experiment. Female birds were fertilised once in 5 d. At 40 weeks of age, 25 settable eggs per replicate were collected and then stored in a separated room at 10 °C for 4 d before incubation. The eggs of each group were placed evenly and hatched in each floor of an automatic incubator (FT-ZF 10, Chunmingfangtong Electronic Co., LTD, Beijing, China). After hatching, 400 male broiler chickens (10 replicates per treatment and 10 broilers per replicate according to broiler feeding schedule) were reared under the same normal conditions in cages in a same house. The environmental Humidity was around 65%. The environmental temperature was maintained at 32–34 °C for the first week and then decreased gradually to 21 °C by 2–3 °C per week and was maintained at 18–24 °C thereafter. Broilers housing and handling procedures during experimentation were in accordance with the guidelines of Handbook of Modern Broiler Production (Shan Citation2001). The whole experimental period lasted for 49 d. Birds were fed corn-soybean meal-based diets. The diet from 1 to 21 d contained 12.54 MJ ME kg−1 and 215.10 g kg−1 CP, 12.96 MJ ME kg−1 and 199.80 g kg−1 CP from 22 to 42 d, 13.17 MJ ME kg−1 and 180.00 g kg−1 CP from 43 to 49 d. The chickens had feed and water ad libitum during the experiment. By combination of natural and artificial light, light was provided 23 h/d at 1 week. At 2 week, the photoperiod was decreased by 1 h d−1 up to a minimum of 16 h d−1 and then maintained at 19h d−1 thereafter.
Data and sample collection
Egg weight, egg components and growth performance of broiler offspring
Before incubation, hatching egg weight was determined per replicate in an egg tray by an electronic balance (JTS-2H, JADEVER Co., LTD, Qingdao, China). Egg components were measured using three randomly selected eggs from each replicate at 40 weeks. Eggs components (egg yolk, egg white and egg shell) were measured by the method of Li et al (Li et al. Citation2011). At day 1, 28 and 49, the bird body weight was determined in a square plastic basket on a per-replicate basis by an electronic balance (JA50K-5, Sunny Hengping Scientific Instrument Co., LTD, Shanghai, China). The average body weight was calculated. Daily feed consumption of each replicate was recorded and the average daily feed intake (ADFI) of the broilers for 49 d was calculated.
Carcase traits
At 28 and 49 d of age, one bird per replicate were randomly selected and slaughtered. The broilers were fasted for 12 h before slaughtering and be killed by bleeding. When each broiler was killed, breast muscles and thigh muscles were removed immediately with a sharp scalpel and then weighed. The left and right sides of breast and thigh muscles were vacuum-packed and stored at 4 °C and −20 °C respectively for further analysis. Moisture content was determined with an oven drying method (5–8 g breast or thigh muscle tissues were dried at 103 °C ± 2 °C for 4–5 h). The lateral central part of the pectoral muscle and the central part of the long head of the biceps femoris muscle were used for determining the intramuscular fat (IMF) content and protein content of muscles by Soxhlet extractor method and the Kjeldahl nitrogen determination method (Zhang Citation2003). IMF and protein contents were shown on the basis of weight of fresh muscle tissue.
Meat quality
Ultimate pH was measured at 24 h in the left muscles of broilers after slaughter with a portable pH metre (SFK Meat Systems, Denmark) combined with a glass electrode. The electrode was inserted into the left muscles for the pH determination. Three measurements were recorded and averaged for each breast. Colour measurements were performed on the medial surface of the left muscles at 24 h after slaughter by an automatic colour difference metre (CR-400, Japan) with the CIELAB system, where L* represents lightness, a* redness and b* yellowness. Higher L *, a* and b* values correspond to paler, redder and more yellow meat, respectively. Each value was an average of at least six measurements. Drip loss was determined by the filter paper method (Kauffman et al. Citation1986). Pieces (2 cm × 2 cm × 1 cm) excised from the left muscles were weighed then placed in a polyethylene box and stored at 4 °C. The muscle was removed from the box then wiped and weighed to evaluate the drip loss, which was expressed as the percentage of the initial muscle weight. Shear force was determined on cooked muscle samples using a universal Warner-Bratzler testing machine (C-LM3, Northeast Agricultural University, China). To prepare cooked muscles, pieces (1.5 cm × 3.0 cm × 0.5 cm) of each muscle were cut, put in a tightly sealed plastic bag, and cooked in water bath at 80 °C until the internal temperature measured by a thermometer into the meat in the bag was 70 °C and then cooled to room temperature. Shear force was shown in Newton.
Total superoxide dismutase (T-SOD), glutathione peroxidase (GSH-Px) and malondialdehyde (MDA)
The activities of T-SOD, GSH-Px and the concentration of MDA in breast and thigh muscle of 49-d-old offspring were measured using colorimetric method with a UV–Vis spectrophotometer (UV-2401PC, Shimadzu Corporation, Japan). The assays were conducted using the assay kits (Nanjing Jiancheng Institute of Bioengineering, Nanjing, Jiangsu, China) according to the prescribed procedures.
RNA isolation, cDNA synthesis and real-time PCR
Based on published sequences of chicken GSH-Px (NM_001039329.1) and GAPDH (glyceraldehyde-3-phosphate dehydrogenase, NM_204305) mRNA on GenBank, oligonucleotide primer sets for the two genes were designed with the software programs Primer Premier 5.0 and Oligo 6 obtained from Shanghai Sangon Biological Engineering Technology & Services Co., Ltd (China). Primer sequences were as follows: GAPDH forward, ′-GCCATCACAGCCACACAGA-3′; GAPDH reverse, 5′-TTTCCCCACAGCCTTAGCA-3′; GSH-Px forward, 5′-GATGAGATCCTGAGAGTGGTGGAC-3′; GSH-Px reverse, 5′-TCCTGTGTCATCCCGATACC-3′. The mRNAs of GAPDH were used as an internal standard for the determination of targeted mRNA levels. Total RNA was extracted from 49-d-old broiler chicken’s breast muscle tissue using Trizol Reagent (Invitrogen, Carlsbad, CA) following the manufacturer’s protocol. RNA quality was assessed by electrophoresis on 12.0 g kg−1 agarose gel. The extracted RNA was then treated with RQ1 RNase-Free DNase (Promega, Madison, WI) to remove contaminated DNA. An amount of 100 mg of total RNA was used for cDNA synthesis. Reverse transcription was performed as described in the M-MLV Reverse Transcriptase protocol according to the manufacturer’s instructions. PCR amplification was performed in triplicate in an ABI PRISM 7500 Fast Real-time PCR system (Applied Biosystems, Foster City, CA) using the SYBR® PrimeScriptTM RT-PCR Kit (Takara Bio Inc., Japan, Takara code: DRR041A) following the manufacturer’s instructions. The 20 μL samples were treated in separate tubes. Initial PCR activation was performed at 95 °C for 30 s, followed by 40 cycles of 95 °C for 5 s and 60 °C for 20 s. The dissociation curves of PCR products were obtained at 62 °C for 15 s, and reaction specificity was determined when there was only one specific peak in the dissociation curve. Using the cycling number (Ct) of the comparative formwork quantitative method to study the GSH-Px gene expression in the muscular tissue. Comparing with the internal reference gene GAPDH, and the Ct’s relative amount: ΔCt = Ct (purpose gene) – Ct (18s GAPDH). The method of 2 − ΔΔCt was used to analyse the real-time PCR data (Livak and Schmittgen Citation2001). The mRNA levels were expressed as the fold change relative to the mean value of the 11.70 MJ ME kg−1 group (T1).
Statistical analysis
A completely randomised design, one-way ANOVA and the mathematical model described below were used in this study. Data were subjected to the GLM procedure of SAS (SAS Institute Inc., Cary, NC), followed by the Duncan’s test when significant differences at p ≤ .05 were realised: Yij = μ+αi+εij, where Yij = the value observed, μ = the overall mean, αi=the effect of treatment (energy intake level) and εij is the residual error. All the percentages were transformed to log10 to normalise data distribution.
Results
Egg weight, egg components, growth performance and carcase traits of broiler offspring
Hatching egg weights were lower in low-energy groups (T2, T3 and T4) compared to the 11.70 MJ ME kg−1 group (p < .05; Table ). The percentage of egg yolk decreased as the maternal dietary energy level decreased (p < .05; Figure ). However, the percentage of egg white increased as the maternal dietary energy level decreased (p < .05). Average body weight of 1-d-old chickens decreased significantly as maternal energy level decreased (p < .05). However, average body weight of 49-d-old chickens was significantly increased when the broiler breeders were fed with the diet of 9.36 MJ ME kg−1 (p < .05). A significant increase was found in thigh muscle as a percentage of body weight of 28-d-old offspring in 9.36 MJ ME kg−1 group, but a significant decrease in 5.85 MJ ME kg−1 group (p < .05). No significant difference was observed in breast muscle as a percentage of body weight (p>.05). Abdominal fat as a percentage of body weight of 49-d-old offspring was significantly increased in 8.19 MJ ME kg−1 group (p < .05). In addition, maternal low-energy diets remarkably increased IMF contents in breast muscle of 28-d-old offspring and thigh muscle of 49-d-old offspring from 8.19 to 5.85 MJ ME kg−1 groups (p < .05; Table ). No significant differences were observed in protein and moisture contents of muscles (p>.05). Low maternal energy level increased the ADFI level of offspring for 49 d (p < .05; Figure ).
Figure 2. Effects of maternal dietary energy level on egg components; abcd means with different letters are significantly different (p < .05); T1 = Treatment 1 (11.70 MJ ME kg−1); T2 = Treatment 2 (9.36 MJ ME kg−1); T3 = Treatment 3 (8.19 MJ ME kg−1); T4 = Treatment 4 (5.85 MJ ME kg−1); ME: Metabolizable energy.
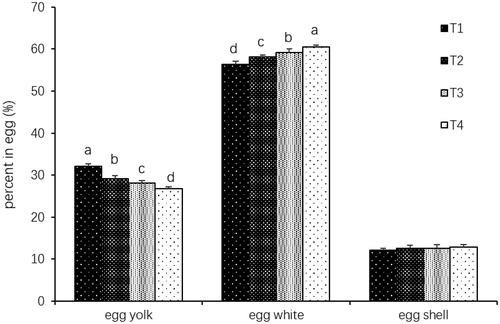
Figure 3. Effects of maternal dietary energy level on the average daily feed intake (ADFI) of broilers; ab means with different letters are significantly different (p<.05); T1 = Treatment 1 (11.70 MJ ME kg−1); T2 = Treatment 2 (9.36 MJ ME kg−1); T3 = Treatment 3 (8.19 MJ ME kg−1); T4 = Treatment 4 (5.85 MJ ME kg−1); ME: Metabolizable energy.
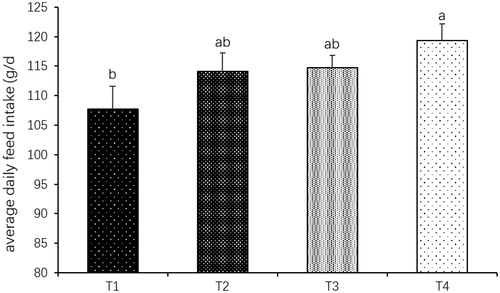
Table 2. Effects of maternal dietary energy level on egg weight, body weight, muscle weight and abdominal fat weight of offspring.
Table 3. Effects of maternal dietary energy level on muscle composition of offspring (g kg−1).
Meat quality of broiler offspring
No significant differences were found in pH (24 h) and shear force (p>.05; Table ). The drip loss of thigh muscle of chickens in 9.36 and 8.19 MJ ME kg−1 groups were lower than 11.70 MJ ME kg−1 group (p < .05). Offspring from 5.85 MJ ME kg−1 group (T4) had lower a* value of breast muscle than those from other groups (p < .05). Offspring from 9.36 to 8.19 MJ ME kg−1 groups (T2 & T3) had higher a* value of thigh muscle than those from other groups (p < .05). And all the chickens from broiler breeders fed low-energy diets had a higher b* value (p < .05).
Table 4. Effects of maternal dietary energy level on meat quality traits of breast muscle and thigh muscle of 49-d-old offspring.
Antioxidant capacity of muscle in broiler offspring
Compared to 11.70 MJ ME kg-1 group, the activities of T-SOD, GSH-Px were higher in 9.36 and 8.19 MJ ME kg-1 groups and lower in 5.85 MJ ME kg−1 group (p < .05; Table ). On the contrary, the concentration of MDA was significantly decreased when the broiler breeders were fed with the diets of 9.36 and 8.19 MJ ME kg−1 while significantly increased when the broiler breeders were fed with the diet of 5.85 MJ ME kg−1 (p < .05).
Table 5. Effects of maternal dietary energy level on breast and thigh muscle antioxidative properties of 49-d-old offspring.
GSH-Px mRNA expression in breast muscle of broiler offspring
Compared with 11.70 MJ ME kg−1 group, the relative expression of GSH-Px mRNA in breast muscle of 49-d-old offspring in 9.36 MJ ME kg−1 group was higher (p < .05; Figure ), but there was no significant difference in 8.19 and 5.85 MJ ME kg−1 groups.
Figure 4. Effect of maternal dietary energy level on relative expression level of GSH-Px mRNA in breast muscle of offspring; abc Means with different letters are significantly different (p<.05); T1: Treatment 1 (11.70 MJ ME kg−1); T2: Treatment 2 (9.36 MJ ME kg−1); T3: Treatment 3 (8.19 MJ ME kg−1); T4 : Treatment 4 (5.85 MJ ME kg−1); ME: Metabolizable energy; GSH-Px = Glutathione peroxidase.
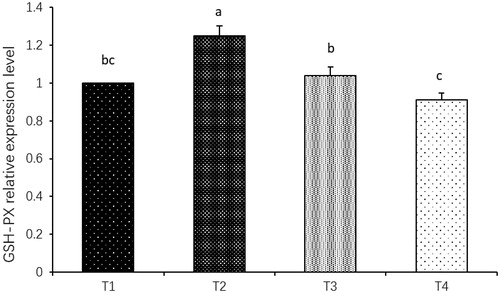
Discussion
Before transported to embryo, maternal nutrition could be deposited in egg yolk and egg albumen that provide the developing embryo and the hatched chick with nutrients, water and minerals for normal growth and development (Attia et al. Citation1995b; Nasr et al. Citation2011). Previous studies indicated that the initial weight of broiler chicks showed a positive correlation with the weight of hatched eggs (Pinchasov Citation1991). The results of this study indicated that maternal low-energy diets could decrease hatching egg weight and affect offspring growth performance significantly. This was similar to the results of Enting et al. (Citation2007), which indicated that low-density diets could decrease egg weight and 1-d-old chick weight. Decreased body weight of 1-d-old broiler offspring in this study was related to the decreased egg weight, the decreased serum triglycerides level and the increased serum low-density lipoprotein-cholesterol level in hatched eggs caused by breeder low-energy diets (Li et al. Citation2018). Zhan et al. (Citation2007) reported that early feed restriction during 1–21 d of age obviously altered the growth performance of broiler chickens, and near full compensatory growth was attained when feed restriction was cancelled from 22 to 63 d of age. In our research, maternal low-energy diet during the laying period led to low egg weight and low 1-d-old chick weight, but there was no significant difference on offspring body weight at 28 d of age, and higher weight in T2 group was found at 49 d of age. All of these suggested that low body weight at 1 d of age caused by low-energy-density broiler breeder diets could be completely compensated for in the growth process of the offspring, and even over compensatory growth. In this research, the ratio between egg weight and hatch weight varies between 72% (T1) and 65% (T4). The huge difference may be related to changes in egg composition in eggs. Low maternal dietary energy level decreased the percentage of egg yolk, which means lower residual yolk weight and lower hatch weight.
In this research, low-energy-density broiler breeder diets could affect carcase quality of offspring. Higher thigh muscle percentage was found in 28-d-old offspring from 9.36 MJ ME kg−1 group, and lower one was observed in the offspring of 5.85 MJ ME kg−1 group, but no difference was found in breast muscle of all groups which may be due to the different metabolic programming effects of maternal energy restriction on breast and thigh muscle. Abdominal fat percentage was significantly increased in 49-d-old offspring when broiler breeders were fed with the diet of 8.19 MJ ME kg−1. But the results of Zhu et al. (Citation2012) indicated that there were no significant effects of ME on abdominal fat percentage. The differences may be related to the different breeds used in trial. Additionally, in this study, it was observed that IMF contents in breast muscle of 28-d-old chicken and thigh muscle in 49-d-old chicken were significantly increased when broiler breeders were fed low-energy diets. Similarly, Zhan et al. (Citation2007) reported that early feed restriction during 1–21 d of age also altered lipid metabolism in the grower and finisher period of broiler chickens and broilers were much more fatty and obese in early feed-restricted group. Also, Attia et al. (Citation2017) found that feed restriction significantly decreased plasma cholesterol and growth rate, but feed intake and FCR were not affected. Expression of chick growth regulatory factors in body was affected by Energy restriction and then increasing the body fat deposition of their offspring by altering the nutrition deposition in eggs (Lippens et al. Citation2000). The result of Noble et Al. (Citation1993) showed that fat content of yolk was decreased continuously from 0 to 14 embryonic age, which indicates that the fat was decomposed and utilised from the beginning of hatching. The most of lipid was transferred to the embryonic heart, muscle and adipose tissue, the lipoprotein is taken up into the liver during the development period (Speake et al. Citation1998).Our previous studies also showed that maternal dietary energy restriction directly affected lipid metabolism in embryos at the end of the laying period and crude fat content of breast muscle tissues of embryos in 9.38 MJ kg−1 group were significantly higher than those in 11.72 MJ kg−1 group and yolk lipids were rapidly absorbed by embryos in 9.38 MJ kg−1 group during the last few days of incubation, and the lipid material was rapidly deposited in the liver and muscle (Li et al. Citation2018). Liver fat percentages and intermuscular fat width of offspring increased by lower energy diets given to broiler breeders (Li et al. Citation2014). These researches suggested that offspring growth and development were affected by restriction nutrition and whether maternal energy restriction or energy restriction in early growth phase altered fat metabolism and deposition in broilers and resulted in obesity in adult life.
Meat colour (L*, a* and b* values), an important visual factor for the buying behaviour, is perceived by consumers as indicate of freshness and wholesomeness (Mancini and Hunt Citation2005). It is due to the concentration of myoglobin and haemoglobin and their chemical states (Brewer Citation2004). Results of this study indicated that maternal low-density diets increased b* value of breast and thigh muscles and L* value of thigh muscle in 49-d-old broiler offspring. Similarly, Zhu et al. (Citation2012) found that broilers from breeders fed 11.09 MJ kg−1 ME diet had higher L* value of breast muscle than those from breeders fed 11.51 MJ kg−1 ME diet. The variation may be related to the fat deposition and feed intake. On the one hand, low maternal energy level enhanced the lipid deposits (such as higher IMF and abdominal fat levels) in body tissues. The higher the IMF level, the less water is lost, which means higher L* value. On the other, a higher corn intake for broilers was found from T2 to T4 groups, which is known to give a yellow colour to the meat by being stored in fat and increase the b* value.
The pH value is one of the most important physical parameters for the qualitative profile of meat and is widely used as a predictor of meat technological and sensory qualities. In this study, no significant differences were detected in pH of offspring breast muscle from different breeder treatments. The ultimate pH of the muscle is positively correlated with water holding capacity (WHC) (Jung et al. Citation2010). WHC is always linked to the juiciness, texture and flavour of meat (Abdullah and Musallam Citation2007). In this study, the drip loss of thigh muscle of 49-d-old chickens was significantly decreased when the broiler breeders were fed with the diets of 9.36 and 8.19 MJ ME kg−1. The variation may be related to the changes in muscle fibre (Huff-Lonergan and Lonergan Citation2005).
Shear force is a widespread indicator for meat tenderness which can be influenced by age, sex, deboning time and cooking method. Tenderness was affected by nutrient components of broiler feeds and be a major quality concern with boneless skinless broiler breast fillets (Attia et al. Citation2014). Main determinants of meat tenderness are connective tissue, IMF content and myofibrillar structure. Jeleníková et al. (Citation2008) reported that the shear force decreased with increasing IMF content. In this study, the diets of 8.19 and 5.85 MJ ME kg−1 significantly increased the IMF content of thigh muscle of 49-d-old offspring but not the shear force. We may be able to draw the conclusion that tenderness of thigh muscle may be not positively associated with IMF content.
Free radicals are produced during normal metabolism and in a dynamic balance but can in turn induce body damage when they are present in excessive levels. SOD and GSH-Px have been generally recognised the main antioxidant enzymes in scavenging the oxygen free radical. SOD is the antioxidant enzyme that catalyses the dismutation of the highly reactive superoxide anion into less reactive hydrogen peroxide. The GSH-Px can inactivate peroxides. MDA is formed as an end product of lipid peroxidation and, therefore, the extent of lipid peroxidation by reactive oxygen species can be monitored by MDA levels (Sumida et al. Citation1989). Antioxidants of embryonic development were obtained from maternal diet (Blout et al. Citation2002; Royle et al. Citation2003) . Antioxidants in the yolk were distributed in embryo tissue through yolk sac membrane during the development period (Surai et al. Citation1996). Gaál et al. (Citation1995) showed that SOD and GSH-Px levels in yolk had a decreasing trend during embryo development but SOD and GSH-Px levels in liver tissue had an increasing trend during embryo development. Surai’s (Citation1999) study showed that GSH-Px level of level was increased by the time of hatching. Yuan et al. (Citation2014) reported that more Se will be deposited in the chick embryo when organic selenomethionine was used in maternal diet, which increased GSH-Px activity and decreased MDA. It indicated that Se enhanced the ability of the developing chick embryo to protect from oxidation. So we can infer that antioxidants of offspring muscle tissues were increased by antioxidants deposition of embryo development. In this study, maternal low-energy diets (9.36 and 8.19 MJ ME kg−1) increased T-SOD and GSH-Px activity and decreased MDA contents in offspring muscles, which indicated that proper maternal low-energy diets might improve the anti-oxidation capability of broiler offspring. Similarly, Zhang et al. (Citation2011) reported that maternal high fat diet could induced to reduce the anti-oxidising ability of offspring in rats, which confirmed the inference.
GSH-Px gene expression in muscle tissue is regulated by multiple signal pathways. Nguyen et al. (Citation2004) reported that exogenous oxidative stress could activate Nrf2 through MAPK signal pathways, which regulated redox state of cells. The gene expression level of antioxidant enzymes in cells was significantly increased for the reason that these signal pathways were activated (Wang Citation2010). Dietary nutrition changes will greatly affect the body’s antioxidant system. When the proportion of energy and protein is extremely unbalanced, oxidative stress will be caused, and then intracellular antioxidant enzyme genes will be damaged (Li et al. Citation2002). This trial showed that compared with 11.70 MJ ME kg−1 group; GSH-Px mRNA relative expression level in the offspring breast muscle tissue of 9.36 MJ ME kg−1 group was significantly increased by 25%, and that of 8.19 MJ ME kg−1 Group was increased by 4%. This implied that broiler breeder dietary energy restriction affected relative expression of GSH-Px mRNA by activating one or multiple pathways of MAPK in body cells. The mitochondria are vulnerable organelles. Some studies have shown that early feed restriction can lead to significant reduction in antioxidant capacity (Pan et al. Citation2005). Mitochondrial membrane may be attacked by more free radicals, which result in higher peroxide degree of unsaturated fatty acid in mitochondrial membrane and the raise of membrane microviscosity (Pamplona Citation2008). Changes in mitochondrial structure and functional damage lead to changes in antioxidant enzyme activity, and this effect reduces the related genes expression of chicken meat for 63 d of age (Zhuang et al. Citation2007). In this trial, relative expression of GSH-Px mRNA in offspring breast muscle tissues of 5.85 MJ ME kg−1 group was reduced by 10%, which indicated that maternal energy restriction changed the expression process of the progeny GSH-Px gene. High content of crude fibre in diet can cause necrosis of jejunal epithelial cells (Yu and Peter Citation1996). The main components of rice hull include crude fibre and silica; large amounts of crude fibres and silicon in rice husk also affect the digestion and absorption of other nutrients (Ding Citation1991). Rice hull at a high level 40% was decreased the concentrations of cholesterol, triglyceride and T3 and increased the concentrations of uric acid and T4 in plasma at 21 d of age (Rezaei and Hajati Citation2010). Similarly, the result of this experiment showed that 37% rice hulls had a negative effect on the antioxidant activity of 49-d-old offspring. Intestines were longer and the mucosal yield higher by rats were injected of streptocitrate and was accompanied by an increase in the SOD activities (Bhor et al. Citation2004) . It can be inferred that diet composition and particularly using of rice hulls has a negative role in this due to its profile of silica and crude fibre that may cause intestinal injury, consuming large portion of antioxidants enzymes for tissue repair.
Conclusions
In summary, dietary energy restriction of broiler breeder female birds during the laying period altered egg weight and offspring hatch weight, but these effects could be compensated during the growth period. Low maternal energy level altered the carcase trait and meat quality of offspring, especially in the lipid deposition in body tissues which made broiler chickens more fatty and obese. Fat content of broiler chickens of lowest energy level with rice hulls at 37% was higher than other groups. In addition, appropriate reduction of maternal dietary energy level could increase the antioxidant capacity of the offspring muscles. Compared with 11.70, 8.19, 5.85 MJ ME kg−1 group, 9.36 MJ ME kg−1 group had higher 49-d-old body weight and mRNA expression of GSH-Px in breast muscle, better antioxidant performance of breast and thigh muscle of offspring. Compare with other groups, the activities of T-SOD, GSH-Px were lower in 5.58 MJ ME kg−1 group, which had poor antioxidant performance of breast and thigh muscle of offspring.
Ethical approval
The protocols used in this experiment were approved by the Northeast Agricultural University Institutional Animal Care and Use Committee. The Animal care and treatment were complied with the standards described in the “Laboratory Animal Management Regulations” (revised 2016) of Heilongjiang Province, China.
Disclosure statement
No potential conflict of interest was reported by the authors.
Additional information
Funding
References
- Abdullah AY, Musallam HS. 2007. Effect of different levels of energy on carcass composition and meat quality of male black goat kids. Livest Sci. 107:70–80.
- Attia YA, Abd-ElHamid AE, Mustafa M, Al-Harthi MA. 2017. Response of slow growing chickens to feed restriction and effects on growth performance, blood constituents and immune markers. Rev Mex Cienc Pecu 2017. 8:175–184.
- Attia YA, Burke WH, Yamani KA, Jensen LS. 1993. Energy allotments and performance of broiler breeder males. Poult Sci. 72:42–50.
- Attia YA, Burke WH, Yamani KA, Jensen LS. 1995a. Energy allotments and performance of broiler breeder 1. Males. Poult Sci. 74:247–260.
- Attia YA, Burke WH, Yamani KA, Jensen LS. 1995b. Energy allotments and performance of broiler breeder. 2. Females. Poult Sci. 74:261–270.
- Attia YA, El-Tahawy WS, Abd El-Hamid AE, Nizza A, El-Kelway MI, Al-Harthi AMA, Bovera F. 2014. Effect of feed form, pellet diameter and enzymes supplementation on carcass characteristics, meat quality, blood plasma constituents and stress indicators of broilers. Archiv Tierzucht. 57:1–14.
- Bhor VM, Raghuram N, Sivakami S. 2004. Oxidative damage and altered antioxidant enzyme activities in the small intestine of streptozotocin-induced diabetic rats. Int J Biochem Cell Biol. 36:89–97.
- Blout JD, Surai PF, Nager RG, Houston DC, Moller AP, Trewby ML, Kennedy MW. 2002. Carotenoids and egg quality in the lesser black backed gull Larus fuscus: a supplemental feeding study of maternal effects. Pro R Soc Lond B. 269:29–36.
- Brewer S. 2004. Irradiation effects on meat color-a review. Meat Sci. 68:1–17.
- Dauncey MJ, Harrison AP. 1996. Developmental regulation of cation pumps in skeletal and cardiac muscle. Acta Physiol Scand. 156:313–323.
- Ding M. 1991. Rice Husk Silicon and Its Application. Anhui Chem Ind. 4:31–36.
- Enting H, Boersma WJ, Cornelissen JB, Van Winden SC, Verstegen MW, Van Der Aar PJ. 2007. The effect of low-density broiler breeder diets on performance and immune status of their offspring. Poult Sci. 86:282–290.
- Gaál T, Mézes M, Noble RC, Dixon J, Speake BK. 1995. Development of antioxidant capacity in tissues of the chick embryo. Com Biochem Physiol. 112B:711–716.
- Goliomytis M, Skoupa EP, Konga A, Symeon GK, Charismiadou MA, Deligeorgis SG. 2016. Influence of gestational maternal feed restriction on growth performance and meat quality of rabbit offsprings. Animal. 10:157–162.
- He ZX, Sun ZH, Tan ZL, Tang SX, Zhou CS, Han XF, Wang M, Wu DQ, Kang JH, Beauchemin KA. 2012. Effects of maternal protein or energy restriction during late gestation on antioxidant status of plasma and immune tissues in postnatal goats. J Animal Sci. 90:4319–4326.
- Huff-Lonergan E, Lonergan SM. 2005. Mechanisms of water-holding capacity of meat: The role of postmortem biochemical and structural changes. Meat Sci. 71:194–204.
- Jeleníková J, Pipek P, Miyahara M. 2008. The effects of breed, sex, intramuscular fat and ultimate ph on pork tenderness. Eur Food Res Technol. 227:989–994.
- Jung S, Choe JH, Kim B, Yun HZ, Kruk A, Jo C. 2010. Effect of dietary mixture of gallic acid and linoleic acid on antioxidative potential and quality of chest meat from broilers. Meat Sci. 86:520–526.
- Kauffman RG, Eikelenboom GP, Van Der Wal G, Merkus G, Zaar M. 1986. The use of filter paper to estimate drip loss of porcine musculature. Meat Sci. 18:191–200.
- Koppenol A, Delezie E, Wang Y, Franssens L, Willems E, Ampe B, Buyse J, Everaert N. 2015. Effects of maternal dietary EPA and DHA supplementation and breeder age on embryonic and post-hatch performance of broiler offspring: age and n-3 pufa affect embryonic and post-hatch performance. J Anim Physiol Anim Nutr. 99:36–47.
- Leeson S, Summers JD. 2008. Commercial poultry nutrition. 3rd ed. Nottingham (England): Nottingham University Press.
- Li F, Mou SY, Liu Y, Jiang D, Wang C, Chen YL, Ren HL, Xu LM. 2018. Maternal dietary energy levels affected the lipid deposition of offspring embryos at the end of the laying period of broiler breeder hens. Ital J of Anim Sci. 17:180–183.
- Li F, Shan MX, Gao X, Yang Y, Yang X, Zhang YY, Hu JW, Shan AS, Cheng BJ. 2019. Effects of nutrition restriction of fat- and lean-line broiler breeder hens during the laying period on offspring performance, blood biochemical parameters, and hormone levels. Domwst Anim Endocrin. 68:73–82.
- Li F, Yang Y, Yang X, Shan MX, Gao X, Zhang YY, Hu JW, Shan AS. 2019. Dietary intake of broiler breeder hens during the laying period affects amino acid profiles in eggs. R Bras Zootec. 48:e20180292.
- Li F, Xu LM, Shan AS, Hu JW, Zhang YY, Li YH. 2011. Effect of daily feed intake in laying period on laying performance, egg quality and egg composition of genetically fat and lean lines of chickens. Br Poult Sci. 52:163–168.
- Li J, Wang H, Stoner GD, Bray TM. 2002. Dietary supplementation with cysteine prodrugs selectively restores tissue glutathione levels and redox status in protein malnourished mice. J Nutr Biochem. 13:25–633.
- Li JF, Xu LM, Zhang YY, Jiang D, Zhang J, Zhang H, Li J. 2014. Effects of maternal dietary energy restriction on fat deposition of offspring. J Northeast Agric Univ (Engl Ed). 21:46–52.
- Lippens M, Room G, De Groote G, Decuypere E. 2000. Early and temporary quantitative food restriction of broiler chickens. 1. Effects on performance characteristics, mortality and meat quality. Br Poult Sci. 41:343–354.
- Livak KJ, Schmittgen TD. 2001. Analysis of relative gene expression data using real-time quantitative PCR and the 2(-Delta delta C(T)) method. Methods. 25:402–408.
- Mancini RA, Hunt MC. 2005. Current research in meat color. Meat Sci. 71:100–121.
- Moraes TGV, Pishnamazi A, Mba ET, Wenger LL, Renema RA, Zuidhof MJ. 2014. Effect of maternal dietary energy and protein on live performance and yield dynamics of broiler progeny from young breeders. Poult Sci. 93:2818–2826.
- Nasr J, Yaghobfar A, Ebrahimnezhad Y, Nazeradl K. 2011. Effects of amino acids and metabolizable energy on egg characteristics and broiler breeder performance. Afr J Biotechnol. 10:10066–10071.
- Nguyen T, Yang CS, Pickett CB. 2004. The pathways and molecular mechanisms regulating Nrf2 activation in response to chemical stress. Free Radical Biol Med. 37:433–441.
- Noble RC, Mccartney R, Ferguson MWJ. 1993. Lipid and fatty acid compositional differences between eggs of wild and captive-breeding alligators (Alligator mississippiensis): an association with reduced hatchability. Proc Zool Soc London. 230:639–649.
- Pamplona R. 2008. Membrane phospolipids, lipoxidative damage and molecular integrity: a causal role in aging and longevity. Biochim Biophys Acta. 1777:1249–1262.
- Pan JQ, Sun WD, Tan X, Li JC, Wang XL. 2005. Effects of early feed restriction on lipid peroxidation and enzymatic antioxidant activity of broiler. Acta Vet Zootech Sin. 36:464–470.
- Peebles ED, Zumwalt CD, Gerard PD, Latour MA, Smith TW. 2002. Market age live weight, carcass yield, and liver characteristics of broiler offspring from breeder hens fed diets differing in fat and energy contents. Poult Sci. 81:23–29.
- Pinchasov Y. 1991. Relationship between the weight of hatching eggs and subsequent early performance of broiler chicks. Br Poult Sci. 32:109–115.
- Ramaiyan B, Bettadahalli S, Talahalli RR. 2016. Dietary omega-3 but not omega-6 fatty acids down-regulate maternal dyslipidemia induced oxidative stress: A three generation study in rats. Biochem Biophys Res Commun. 477:887–894.
- Rehfeldt C, Nissen PM, Kuhn G, Vestergaard M, Ender K, Oksbjerg N. 2004. Effects of maternal nutrition and porcine growth hormone (pgh) treatment during gestation on endocrine and metabolic factors in sows, fetuses and pigs, skeletal muscle development, and postnatal growth. Domest Anim Endocrinol. 27:267–285.
- Rezaei M, Hajati H. 2010. Effect of diet dilution at early age on performance, carcass characteristics and blood parameters of broiler chicks. Ital J Anim Sci. 9(1):93–100.
- Royle NJ, Surai PF, Hartle IR. 2003. The effect of variation in dietary intake on maternal deposition of antioxidants in zebra finch eggs. Funct Ecol. 17:472–481.
- Shan YL. 2001. Handbook of modern broiler production. Beijing (China): China Agriculture Press.
- Speake BK, Murray AMB, Noble RC. 1998. Transport and transformations of yolk lipids during development of the avian embryo. Prog Lipid Res. 37:1–32.
- Sumida S, Tanaka K, Kitao H, Nakadomo F. 1989. Exercise-induced lipid peroxidation and leakage of enzymes before and after vitamin E supplementation. Int J Biochem. 21:835–838.
- Surai PF. 1999. Tissue-specific changes in the activities of antioxidant enzymes during the development of the chicken embryo. Brit Poult Sci. 40:397–405.
- Surai PF, Noble RC, Speake BK. 1996. Tissue-specific differences in antioxidant distribution and susceptibility to lipid peroxidation during development of the chick embryo. Biochim Biophys Acta. 1304:1–10.
- Tarry-Adkins JL, Chen JH, Jones RH, Smith NH, Ozanne SE. 2010. Poor maternal nutrition leads to alterations in oxidative stress, antioxidant defense capacity, and markers of fibrosis in rat islets: potential underlying mechanisms for development of the diabetic phenotype in later life. FASEB J. 24:2762–2771.
- Van der Waaij EH, Van der Brang H, Van Arendonk JAM, Kemp B. 2011. Effect of match or mismatch of maternal-offspring nutritional environment on the development of offspring in broiler chickens. Animal. 5:741–748.
- Wang C. 2010. The effect of oxidative stress on main MAPK signal transduction pathway of chicken meat quality [dissertation]. Beijing (China): Chinese Academy of Agricultural Sciences.
- Wu G, Bazer FW, Wallace JM, Spencer TE. 2006. Board-invited review: intrauterine growth retardation: implications for the animal sciences. J Anim Sci. 84:2316–2337.
- Xu LM, Chen ZH, Li F, Cheng BJ, Shan AS. 2010. Effects of maternal dietary energy restriction on growth performance, carcass and meat quality of broilers. Chi J Anim Nutr. 22:894–903.
- Yu B, Peter WS. 1996. Effects of crude fibre level in the diet on the intestinal morphology of growing rabbits. Lab Anim. 30:143–148.
- Yuan D, Guo X, Shi M. 2014. Maternal dietary supplementation with two sources of selenium affects the mortality and the antioxidative status of chick embryo at different developmental periods. Int J Agric Biol. 16:629–633.
- Zhan XA, Wang M, Ren H, Zhao RQ, Li JX, Tan ZL. 2007. Effect of early feed restriction on metabolic programming and compensatory growth in broiler chickens. Poult Sci. 86:654–660.
- Zhang LY. 2003. Feed analysis and examination technology of feed quality. Beijing (China): China Agriculture Press.
- Zhang X, Strakovsky R, Zhou D, Zhang Y, Pan YX. 2011. A maternal high-fat diet represses the expression of antioxidant defense genes and induces the cellular senescence pathway in the liver of male offspring rats. J Nutr. 141:1254–1259.
- Zhu C, Jiang ZY, Jiang SQ, Zhou GL, Lin YC, Chen F, Hong P. 2012. Maternal energy and protein affect subsequent growth performance, carcass yield, and meat color in Chinese yellow broilers. Poult Sci. 91:1869–1878.
- Zhuang JY, Xia D, Yang XJ, Xu XP, Ni YD, Li X, Zhao RQ. 2007. Long-term effect of early feed restriction on lipid peroxidation and enzymatic antioxidant activity in broiler chicken. J Clin Rehabil Tissue Eng Res 11:7573–7576.